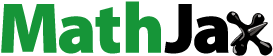
ABSTRACT
The oil content, fatty acid, oil quality and oxidation stability of seeds of four woody oil plants (pecan, Camellia oleifera, Torreya grandis and walnut) were analyzed. The results showed that pecan oil had the strongest antioxidant activity, the variation range of FFA, peroxide value(PV), UV absorption, carbonyl value(CV) and OSI were the lowest; C. oleifera oil and T. grandis oil were the next, and walnut oil had the greatest variation range; besides, pecan oil contained higher tocopherol and total phenol(TP) content. Correlation analysis showed that, OSI was significantly positively correlated with OA, tocopherol and TP, and significantly negatively correlated with PA, LA, LOA and PV. The oxidation stability from superior to inferior is pecan oil, C. oleifera oil, T. grandis oil and walnut oil. Meanwhile, the oil content of pecan kernel was the highest, the fatty acid composition of pecan oil fulfills human dietary needs, it’s an high-quality edible oil.
Abbreviations: PA: palmitic acid; POA: palmitoleic acid; SA: stearic acid; OA: oleic acid; LA: linoleic acid; LOA: linolenic acid; AA: arachidic acid; EA: cis-11-eicosenoic acid; UFA: unsaturated fatty acid;SFA: saturated fatty acid; FFA: free fatty acid; PV: peroxide value; CV: carbonyl value; TP: total phenols; OSI: oil stability index; UV: ultraviolet.
1. Introduction
Plant oil is extracted or pressed from seeds of oil plants. Plant oil is commonly consumed as an important source of energy and nutrition because it is rich in fat and nutrients. Under the current background of global food supply and demand is tight background, the development of woody oil industry is an effective means to cope with the shortage of edible oil. The fatty acid compositions and lipid contents of plant oils differ, with some plant oils more beneficial than others in terms of nutrition and human health (Liu et al., Citation2017). Oil plants are divided into herbaceous and woody plants. There are abundant woody plant oil germplasm resources in China, with more than 150 kinds of seeds with oil contents above 40% and more than 50 kinds of edible woody oils (Wang et al., Citation2017). Pecan (Carya illinoensis (Wangenh.) K. Koch.), native to the United States and Mexico, is a valuable dried fruit and woody oil tree species worldwide. Pecan was introduced into China more than 110 years ago. The shell of the pecan nut is thin and easy to peel, and the kernel contains more than 70% of the oil, in addition, it is rich in protein and carbohydrates, as well as amino acids, vitamins, and minerals, all of which are beneficial to human health (Yao et al., Citation2014). Thus, pecan is highly valued as a source of nutrition and health. C. oleifera (Camellia oleifera Abel.), which belongs to the camellia genus of the camellia family, is an edible woody oil plant species unique to China. The seed kernels contain about 47% oil and are also rich in microelements and active ingredients required for human health. C. oleifera oil is comparable or possibly superior to olive oil according to the main quality indexes and has extremely high nutritional and health value (Wang, Fei, et al., Citation2021). T. grandis (Torreya grandis cv. Merrilli) is an evergreen tree belonging to the Taxaceae family. It has been cultivated for more than 1,300 years and is a tree specie of economic importance in China. It contains about 50% oil in the kernels. The nuts are nutritious and a source of a variety of amino acids and mineral elements thought to play important roles in the prevention and treatment of cardiovascular and cerebrovascular diseases (Wang, Yao, et al., Citation2021). Walnut (Juglans regia L.) is one of the four major nuts cultivated worldwide. China is the largest producer of walnuts worldwide, with approximately 48% of the world’s total annual output (Gao et al., Citation2018). Walnut kernels contain a high oil content (65%). and it contain a variety of vitamins, mineral elements, and physiologically active substances, with high nutritional and health care value (Choi et al., Citation2016).
Plant oils contain mainly unsaturated fats. Unsaturated fats are vulnerable to oxidative rancidity as a result of complex free radical chain reactions induced by oxidation. Various factors cause oxidation of unsaturated fats, including temperature, light, air, and water. Small molecules, such as aldehydes, ketones, and acids, are broken down when fatty acids react with oxygen and lead to an increase in free fatty acid (FFA) content and peroxide value (PV). This leads to the production of a musky pungent odor and a change in oil color, with the oil turning dark and having a poor taste. Moreover, small molecules (e.g. aldehydes, ketones, acids, etc.) are difficult to metabolize and accumulate in the body, where they can cause liver damage (Shadyro et al., Citation2020).
Therefore, the anti-oxidation of lipids has long attracted attention in the field of lipid chemistry (Sun et al., Citation2017). The oxidation of nut oil was mainly auto-oxidation and thermal oxidation, followed by photo-oxidation and enzymatic oxidation (Gao et al., Citation2017). Many studies have shown that autoxidation is the main cause of oxidative deterioration of nuts (David & Eric, Citation2015). There are many studies on the oxidation stability of bulk edible plant oils (Cao et al., Citation2013; Huang et al., Citation2019). Oxidation stability is assessed by evaluating the degree of oxidation of oils during deterioration, focusing on changes in physicochemical and sensory propertiess (Li et al., Citation2020; Zhu et al., Citation2015). Thus far, research on C. oleifera oil and walnut oil in China has primarily addressed issues relating to oil processing, nutritional quality, and utilization of by-products (Wang et al., Citation2020; Zheng et al., Citation2020; Zhou & Pei, Citation2016). The research on T. grandis and pecan mainly focuses on breeding, cultivation technology, growth and development and chemical composition (Cao et al., Citation2022; Jia et al., Citation2012; Luo et al., Citation2017; Zhu et al., Citation2019). There are relatively few studies on the oxidative stability of T. grandis oil and pecan oil (Rudolph et al., Citation1992; Yang et al., Citation2018; Zhang et al., Citation2022). In recent years, the woody oil plant industry, including pecan, C. oleifera, T. grandis and walnut production, has grown rapidly, and the industry continues to expand. However, the research on the utilization and quality of oils from the seeds of these woody oil plants is lagging behind. This study investigated the oil oxidation stability of four woody oil plant species (pecan, C. oleifera, T. grandis, and walnut) to provide reference data needed for the processing of high-grade woody edible oils and the development of high-quality and stable edible plant oil products.
2. Materials and methods
2.1. Experimental material
Pecan nuts were obtained from Xin’anjiang Forest Farm, Jiande City, Zhejiang Province. C. oleifera seeds were picked from the camellia germplasm nursery of Dongfanghong Forest Farm, Jinhua City, Zhejiang Province. T. grandis nuts were obtained from a plantation in Fuyang City, Zhejiang Province. Walnuts were purchased from Aksu, Xinjiang Uygur Autonomous Region.
2.2. Methods
2.2.1. Oil extraction and oxidation stability test
About 3 kg seeds of the four woody oil plant species (pecan, C. oleifera, T. grandis, walnut) were weighed respectively and transported to the laboratory, then cleaned and dried to 7% kernel moisture content in fan-forced oven at 50°C. They were shelled and pressed using a screw oil press (ZYJ-9018, Guangdong, China). The plant oils were then dehydrated with anhydrous sodium sulfate and filtrated with a vacuum pump. For each of the four wood oil plant species, three separate oil extraction were performed from three different batches of seeds.
The autoxidation rate of oil is relatively slow at room temperature. To judge the oxidation stability of oils, low-temperature oven-accelerated oxidation is commonly used, as it reflects the autoxidation behavior of oils at room temperature (Huang et al., Citation2019; Miraliakbari & Shahidi, Citation2008). We placed samples of the four seed oils (total volume: 20 mL) in 84 uncovered brown glass sample vials (20 mL, e.d. 27 mm×h. 57 mm, with cap fitted with a PTEF inner pad). There were 21 bottles of each oil sample. The samples were placed in an oven at 60°C and sampled as follows: 0 days, 1 day, 3 days, 5 days, 7 days, 9 days, and 11 days. Each experiment was done in three replication of oil sample which was heated. On each sample day, three bottles of each oil sample were removed and stored in a refrigerator at −18°C for later analysis.
2.2.2. Determination of kernel oil content, free fatty acid (FFA) content, and peroxide value (PV)
The kernel oil contents of the four woody oil plant species were determined using Soxhlet standard extraction mode of a B811-Soxhlet apparatus (Buchi, Switzerland) by which the dried seed samples were finely grounded in a household grinder and extracted with petroleum ether (boiling range 30 − 60°C) at a constant temperature of 110°C for 8 hours. The methods of International Organization for Standardization were used to determine FFA (ISO 660:Citation2020) and PV (ISO 3950:Citation2017). Each treatment was analyzed in triplicates.
2.2.3. Determination of fatty acid composition
The fatty acid composition of oil samples was analyzed as methyl esters by gas chromatography. Methyl esters were prepared in compliance with the standard method (ISO 12966-2, Citation2017), and Samples were analyzed using a gas chromatograph (2010 Plus, Shimadzu, Japan) equipped with an autosampler, a split/splitless injector, and a flame ionization detector. A fused silica capillary column (30 m length × 0.32 mm internal diameter and 0.25 µm film thickness; FAMEWAX; Restek, U.S.A.) was used. An initial column temperature of 150°C for 1 minute, then programmed to 190°C at a rate of 5°C/minute and maintained for 20 minutes. Both the injector and detector were held at 220°C. The sample of 1 µL was injected and the split ratio was 1:10. Identification of fatty acid methyl esters was carried out using a mix of 37-component fatty acid methyl esters purchased from Sigma Aldrich (Shanghai) (Sigma-Aldrich, Milan, Italy). Each sample was analyzed in triplicates. Individual fatty acids were expressed as the percentage of total fatty acids.
2.2.4. Determination of tocopherols
The oil samples (1 g, accurated to 0.01 g) were briefly vortexed in 10 mL of n-hexane and filtrated (0.22 µm, PTFE) and analyzed using HPLC. The analysis was performed on an Agilent 1290 high-performance liquid chromatography (Agilent Technologies Inc., Santa Clara, CA, U.S.A.) equipped with a fluorescence detector (Agilent Technologies Inc., Santa Clara, CA, U.S.A.) using a silica column (250 mm × 4.6 mm i.d., 5 µm, Waters Co., Milford, MA, U.S.A.). The mobile phase was n-hexane:isopropanol (98.5:1.5) with 10 µL injection volume and flow rate of 1.2 mL/min. The column temperature was 30°C. The excitation wavelength and emission wavelength of the fluorescence detector were 295 nm and 333 nm respectively. The tocopherols were identified and quantified by comparing with the mixed standard (α-tocopherol, β-tocopherol, γ-tocopherol and δ-tocopherol) (MilliporeSigma, Billerica, MA). The results were expressed in milligrams of tocopherol per 100 grams of oil. Each treatment was analyzed in triplicates.
2.2.5. Determination of total polyphenols
Phenols from oil were extracted using a method adapted from conditions described in previous studies (Ojeda-Amador et al., Citation2018). Then 1 g of oil are extracted using 20 mL in two extraction (10 mL +10 mL) of MeOH:H2O (70:30), with 1 minutes vortex followed by 5 min ultrasound and centrifugation at 2000 × g for 10 minutes. Total phenolic compounds from the extracts above were quantified by the Folin−Ciocalteu method (Mahmood et al., Citation2011) using a calibration curve of gallic acid and expressed as milligram per kilogram of oil. 1 mL of extract above was added to 5 mL of Folin- Ciocalteu’s phenol reagent (5% w/v) and to 4 mL of sodium carbonate (7.5% w/v). The mixture was kept in the dark for 30 min after 1 min of vortex oscillation. The absorptions of the solution at 765 nm were measured. Each sample was analyzed in triplicates.
2.2.6. Determination of ultraviolet (UV) absorbance at 232 nm (K232) and 270 nm (K270) and carbonyl value (CV)
The UV absorbance and CV were analyzed by UV-2550 UV-visible spectrophotometry (Shimadzu Company, Japan). For determination of K232 and K270, 0.1 g of oil sample was weighed accurately and transferred to a 25 mL volumetric flask, dissolved with isooctane, and diluted to the scale line. Using the solvent isooctane as a reference, the absorbance at K232 and K270 was measured using a quartz cuvette, as below:
For determination of the CV, 0.1 g of oil sample was weighed accurately and transferred to a 25 mL test tube with a plug and dissolved with 5 mL of benzene, followed by the addition of 3 mL of trichloroacetic acid and 5 mL of 2,4-dinitrophenylhydrazine in order and mixed well. The test tube was sealed with a plug and heated in a 60°C water bath for 30 minutes and then cooled to room temperature under running water, after which potassium hydroxide ethanol solution (10 mL) was added, mixed evenly, and left for 10 minutes. The absorbance was then measured at 440 nm.
2.2.7. Determination of the oil oxidative stability
The oil oxidative stability is expressed as the induction period determined by the Rancimat method (Gharby et al., Citation2012). A Rancimat 743 oil oxidation stability tester (Metrohm Ltd., Herisau, Switzerland) was used for determination of the induction time. The detection temperature was 110°C, the airflow was 10 L/hour, and the sample amount was 3.00 ± 0.01 g. All determinations were carried out in triplicate.
2.3. Statistical analysis
All the experiments were performed in triplicate and all data were processed and analyzed using Microsoft Office Excel 2016 and IBM SPSS Statistics for Windows, version 19.0 (IBM Corp., Armonk, NY). To test the statistical significance of the results, a one-way analysis of variance was used. Differences were considered statistically significant at the P < .05 level.
3. Results and discussion
3.1. Kernel oil content and fatty acid composition of the four woody plant oils
The kernel oil content of the four woody plant oils was significantly different (P < .01) (), with pecan having the highest oil content (73.07%), followed by walnut (65.48%), T. grandis (55.28%) and C. oleifera (49.34%).
Fatty acid composition is an important indicator of the nutritional quality of plant oils. The fatty acid compositions and relative contents of the four oils are shown in . The results indicated that all four woody plant oils contain palmitic acid (PA), stearic acid (SA), oleic acid (OA), linoleic acid (LA), linolenic acid (LOA), and cis-11-eicosenoic acid (EA), among which OA and LA were the main fatty acids. In addition, there were small amounts of other fatty acids, such as palmitoleic acid (POA) and arachidic acid (AA) in pecan oil, POA in walnut oil, trans-6,11-eicosadienoic acid (2.89%), cis-5,11,14-eicosatrienoic acid (8.7%), and AA (0.16%) in T. grandis oil. Cis-5,11,14-eicosatrienoic acid, also known as sciadonic acid, is a characteristic fatty acid of T. grandis oil and has high positive functions in lowering triglycerides, reducing inflammation, preventing blood clots and regulating lipid metabolism (Lou et al., Citation2023; Wang, Yao, et al., Citation2021).
Table 1. The fatty acid compositions and contents of the four woody oil plant seeds.
Among the saturated fatty acids (SFAs), the PA relative content was highest (P < .01), followed by SA and EA relative contents. The total SFA relative content in the four oils was as follows: T. grandis oil > walnut oil > C. oleifera oil > pecan oil.
The total unsaturated fatty acids (UFAs) relative content of the four seed oils was about 90%, with pecan oil having the highest UFA relative content (91.19%), which was significantly higher than that of the other three oils (P < .05). There was no significant difference in the UFAs relative contents of C. oleifera, T. grandis, and walnut oils (P > .05). Among the four seed oils, the OA relative content was highest in C. oleifera oil (78.81%), followed by pecan oil (70.92%), with the OA relative contents of these oils markedly higher than those in the T. grandis and walnut oils (P < .01). Among the four woody plant seed oils, the highest OA relative content was found in C. oleifera oil (78.81%), followed by pecan oil (70.92%), with C. oleifera oil and pecan oil having markedly higher OA relative contents that those of either T. grandis or walnut oils (P < .01). The highest LA relative content was found in walnut oil (62.92%), followed by T. grandis oil (40.61%). Walnut oil had the highest LOA relative content (12.19%), which was much higher than that of the other three oils (P < .01).
UFAs are easily absorbed and digested by the human body and are indispensable nutrients for human growth. Instead of accumulating fat in the body, UFAs can lower low-density cholesterol and serum cholesterol levels. UFAs also have physiological functions, including immune-enhancing and antiaging properties (Liu et al., Citation2011). WHO recommends that adults limit total fat intake to 30% of total energy intake or less, adults and children reduce SFA intake to 10% of total energy intake. There is research that showed that dietary intake of PUFAs can prevent and reduce the risk of cardiometabolic diseases (Schulze et al., Citation2020). LA and LOA are essential fatty acids in the human body. Like UFAs, LA can reduce serum cholesterol, as well as inhibit arterial thrombosis and prevent atherosclerosis and osteoporosis (Wang et al., Citation2016). LOA has important physiological functions, such as enhancing intelligence, immunity, and eyesight. However, both LA and LOA are easily oxidized. The oxidation rate of LOA is twice that of LA and 25 times that of OA (Chu et al., Citation2013). The OA relative content is an important index to evaluate the quality of edible oil, and it is known as a safe fatty acid in the field of nutrition (Yuan et al., Citation2013). According to our results, pecan oil is an ideal edible plant oil based on its fatty acid composition, having the highest relative content of UFAs and OA among the four woody plant oils.
3.2. Tocopherol compositions and contents of the four woody plant seed oils
Tocopherol is present in plants in four forms (α-, β-, γ- and δ-) which possess different molecular structures and functions, the composition of tocopherol is an important characteristic feature have some nutritional importance because they are known to have an antioxidative activity, which protects the polyunsaturated fatty acids (PUFAs) against oxidative deterioration; additionally, a biological activity exists, which protects cells against oxidative stress (Hajib et al., Citation2021; Seker et al., Citation2022). lists the tocopherol compositions and contents of the four woody plant seed oils. The total tocopherol contents of T. grandis oil were 114.9 mg/100 g, which is much higher than that of pecan oil (22.04 mg/100 g), C. oleifera oil (19.3 mg/100 g) and walnut oil (20.07 mg/100 g) (P < .01). Among the four woody plant seed oils, α-tocopherol and γ-tocopherol were identified in pecan oil, and γ-tocopherol was the main component and represented about 21.6 mg/100 g; only α-tocopherol were present in C. oleifera oil, the content was 19.3 mg/100 g; α-tocopherol and β-tocopherol were identified in T. grandis oil, the contents were respectively 12.09 mg/100 g and 102 mg/100 g; there were three types of tocopherols in walnut oils which were α-tocopherol, γ-tocopherol and δ-tocopherol, of which γ-tocopherol content was the highest (15 mg/100 g), followed by δ-tocopherol(4.41 mg/100 g) and α-tocopherol (0.86 mg/100 g). Of the four wood plant oils, only T. grandis oil contains β-tocopherol, common vegetable oils often contain little or no β-tocopherol, however T. grandis oil is rich in β-tocopherol, which also can serve as a characteristic indicator of T. grandis oil.
Table 2. Tocopherol compositions and contents of the four woody plant seed oils.
3.3. Total pherols contents of the four woody plant seed oils
Phenolic compounds are well-known effective chain-breaking antioxidants for lipids and other organic compounds (Zhao & Lliu, Citation2009), they can not only be used as antioxidants, but also have various physiological functions such as anti-cancer, anti-radiation, and lowering blood pressure, which can play an important role in human health (Su et al., Citation2016). lists the of total phenol contents of the four woody plant seeds oil. The results showed that the total phenol content of pecan oil was the highest (12.6 mg/kg), followed by T. grandis oil and C. oleifera oil, with the total phenol content was 11.3 mg/kg and 9.65 mg/kg respectively, the lowest level was walnut oil, there were statistically significant difference between them (P < .05). There is research that showed that phenols are one of the main antioxidant substances in Carya kernel (Chen et al., Citation2013). Some studies have compared pecan with a variety of vegetables and nuts, and found that pecan had the most total phenol content and the strongest antioxidant capacity (Wu et al., Citation2004), which is consistent with the results of this study. Phenolic compounds inhibit lipid oxidation by interfering with either chain propagation or initiation by readily donating hydrogen atoms to lipid peroxyl radicals (Kortenska et al., Citation2002).
3.4. Changes in FFA contents and PVs during oxidation of the four woody plant seed oils
FFAs can reflect the degree of hydrolysis rancidity. As shown in , the order of initial FFA contents (day 0) in the four plant oils from low to high was as follows: walnut oil < pecan oil < C. oleifera oil < T. grandis oil. With the heating time increased, the FFA contents of all four oils showed an upward trend, which was due to oxidation and heating-induced cracking of fatty acids, especially UFAs, resulting in the formation of FFAs or other small molecular compounds, which were further oxidized to produce organic acids (Zhou & Li, Citation2015). In general, of the four oils, the trend in FFA formation was slowest in pecan oil in response to heating. As compared with their initial values before heating (day 0), FFA contents increased by 7.78% in pecan oil and by 8.23% and 8.48% in C. oleifera oil and T. grandis oil, respectively, on the 11th day of heating. The FFA content of walnut oil changed little in the first 9 days and then increased rapidly after 9 days. By the 11th day, it had increased more than two times compared with the initial value (day 0). These results indicated that among the four oils, the FFA content of pecan oil changed the least, whereas that of walnut oil changed the most (P < .01).
The PV is a measure of the concentration of peroxides and hydroperoxides formed in the initial stages of lipid oxidation. It is one of the most widely used tests for the measurement of oxidative rancidity in oils and fats (Guo et al., Citation2016). As shown in , the initial PVs of the four oils were in the order of pecan oil < T. grandis oil < C. oleifera oil < walnut oil. The PV of walnut oil was significantly higher than that of the other three oils (P < .01). The PVs of the four plant oils increased to varying degrees with an extension in the heating time. Among the four plant oils, the change in the trend in PVs of pecan oil with heating time was lowest (slope of the linear relationship: y = 0.0097 × + 0.0384, R2 = 0.9811), followed by C. oleifera oil (y = 0.0303 × + 0.2050, R2 = 0.9881) and T. grandis oil (y = 0.0605 × + 0.0581, R2 = 0.9029). Walnut oil showed the biggest trend, with PVs increasing rapidly after the 9th day and increasing 123.78% on the 11th day compared with the 9th day (y = 0.1525 × + 0.6462, R2 = 0.6334). Thus, pecan oil had the lowest change in PV and the best oxidation stability. By contrast, the PV of walnut oil increased the fastest (P < .01), and walnut oil was most likely to be oxidized.
3.5. Main fatty acids in the four woody plant oils during oxidation
The contents of OA and LA, which are essential fatty acids in the human diet, can serve as a marker of edible oil quality. As shown in , the order of OA and LA content in the four oil samples from high to low was as follows: pecan oill > C. oleifera oil > walnut oil > T. grandis oil, with a statistically significant difference in the OA/LA contents of the oils (P < .05). Therefore, in terms of their fatty acid compositions, of the four oils, pecan oil and C. oleifera oil have the highest nutritive and health values.
Table 3. The mainly fatty acid relative content during oxidation of the four woody oils.
With an extension in the heating time, the OA contents in the four oils increased slightly, whereas LA contents decreased slightly, but there was no significant difference (P > .05). And, there was no significant difference among the four oils in the total OA and LA contents (P > .05). The SFA and UFA contents did not change significantly. Oil oxidation stability is affected by various factors, including the total amount of UFAs, oxidation characteristics of single fatty acids, and the proportional model of fatty acids composition and amount (Leonardis & Macciola, Citation2012). Usually, the change of fatty acid contents is little during accelerated oxidation of oil, which is characterized mainly by a decrease in polyunsaturated fatty acids and an increase in SFA to different degrees (Pang et al., Citation2021), this is basically coincides with this study. This may be due to the oxidation of the double bonds of USFAs in the oils during heating, so that some or all of the double bonds of UFAs such as linoleic acid and linolenic acid are oxidized to form stearic acid or oleic acid, which reduces the relative content of linoleic acid and increases the relative content of oleic acid, and the total relative content of SFAs increases.
3.6. UV absorption (K232 and K270) during oxidation of the four woody plant oils
Lipid primary oxidation products, conjugated diene hydroperoxides, show absorption at 232 nm. Thus, K232 is often used to determine the degree of lipid oxidation during the initial stages of autoxidation when hydroperoxides is produced (Mohdaly et al., Citation2010). As shown in , K232 of the four oils showed an upward trend in accordance with an extension of the heating time. Among the four oils, K232 of walnut oil had the fastest rate of increase (P < .01), which increased 3.4 times on day 11 compared with day 0. K232 of pecan oil, C. oleifera oil, and T. grandis oil showed a relatively gentle upward trend, with values increasing 0.60, 1.3, and 1.53 times, respectively, on day 11 compared with day 0 (P < .01).
The presence of secondary oxidation products, especially dienones and conjugated trienes, in oil can be detected by measuring its absorbance at 270 nm. K270 is one indicator of the degree of secondary oxidation of oil: the lager K270 is, the more accumulation of secondary oxidation products (Conte et al., Citation2020). As shown in , K270 of all four oils showed an upward trend in accordance with an extension of the heating time, and the K270 on the 11th day were significantly higher than those on day 0 (P < .01). Compared with K270 values of the four woody plant oils on day 0, the growth rate with heating was slowest for pecan oil, with an increase of 2% on the 11th day, followed by C. oleifera oil and T. grandis oil, with increases of 3% and 10%, respectively, on the 11th day. The rate of increase with heating was fastest for walnut oil, with an increase of 16% on the 11th day. These findings suggested that pecan oil had the best oxidation stability, followed by C. oleifera oil and T. grandis oil. Walnut oil had the worst oxidation stability.
3.7. CVs during oxidation of the four woody plant oils
The CV refers to the amount of peroxides produced during oil oxidation that are further decomposed into fatty acids or glycerides containing carbonyl and ketone groups and their polymers. The value can reflect the content of oxidative rancidity products (e.g. ketones, aldehydes, and other carbonyl compounds) and the deterioration degree of oil (Chen et al., Citation2006). As shown in , before heating, the CVs of the four oils from low to high were as follows: C. oleifera oil < pecan oil < walnut oil < T. grandis oil. The CV of T. grandis oil was markedly higher than that of the other three oils (P < .01). With an increase in the heating time, the CVs of the four oils showed a rising trend to different degrees, and the CVs of the four oils on the 11th day were significantly higher than those on day 0 (P < .01). The CV of walnut oil increased the fastest, with the highest value (10.91 meq/kg) recorded on the 11th day of heating, followed by T. grandis oil. The CVs for pecan oil and C. oleifera oil increased at a relatively slow rate, with the CVs remaining low on the 11th day (2.61 meq/kg and 2.60 meq/kg, respectively) (P < .01). These findings indicate that with an increase in the heating time, the carbonyl compound production rate of pecan oil was lowest, followed by that of C. oleifera oil.
3.8. OSI during oxidation of the four woody plant seed oils
The oxidation rate of most oils is slow in the initial stage and then accelerate during oxidation. The initial slow oxidation stage is known as the induction period. Oxidation stability can be determined by measuring the oxidation induction time of oil (i.e. OSI). A higher OSI denotes better oxidation stability. Conversely, a lower OSI denotes worse oxidation stability (Zhang, Citation2001). As indicated in , before heating (day 0), of the four seed oil types, the OSI of pecan oil was markedly higher (15.06 hours) than that of the three other oils (P < .01). The next best OSI was that of T. grandis oil and C. oleifera oil, with no significant difference in the OSI of the two oils (P > .05). The OSI of walnut oil was the lowest (0.99 hours). During the heating process, the OSI of all the four oils showed a downward trend. On the 11th day of heating, the OSI of pecan oil decreased by 27.09% compared with that on day 0, but the OSI was still the highest (10.98 hours), followed by the OSI of T. grandis oil and C. oleifera oil, which decreased by 32.94% and 51.34%, respectively. Pecan oil had the best oxidation stability on day 11, followed by T. grandis oil and C. oleifera oil. On the 11th day of heating, the OSI of walnut oil was 0.15 hours, which was at the lowest level compared to the other three oils. Therefore, of the four oils, the oxidation stability of walnut oil was worst. Compared with the other three oils, walnut oil contains more polyunsaturated fatty acids such as linoleic and linolenic acids, which are highly susceptible to a free-radical chain reaction under oxygen and high temperature to form hydroperoxides and eventually break down into volatile compounds, resulting the perception of rancidity (Grilo & Wang, Citation2021; Guillen & Goicoechea, Citation2009). Thus, high polyunsaturated fatty acids result in low stability of walnut oil due to rancidity development from oxidation and lipolysis. On the contrary, among the four oils, pecan oil has the highest total amount of unsaturated fatty acids, and the content of oleic acid in monounsaturated fatty acids is higher, second only to that of C. oleifera oil, and significantly higher than that of T. grandis oil and walnut oil. Monounsaturated fatty acids are less susceptible to oxidation and cleavage than polyunsaturated fatty acids. In addition, the content of total phenols in pecan oil is much higher than that of other three oils, and total phenols has certain antioxidant properties. Therefore, pecan oil has strong oxidation stability.
3.9. Correlation between oil quality and OSI
presents the results of the correlation analysis of oil quality and oil stability. In terms of fatty acids, the OA content was negatively correlated with SA, LA and LOA contents (r = −0.68, r = −0.99 and r = −0.77, respectively). OSI was positively correlated with the OA content (r = 0.65) and negatively correlated with PA, LA and LOA contents (r = −0.62, r = −0.62 and r = −0.66, respectively). In addition, FFA were significantly positively correlated with K232, K270, CV, SA, EA, and total phenol contents (r = 0.61, r = 0.95, r = −0.87, r = 0.71, r = −0.85 and r = 0.93, respectively), PV were significantly positively correlated with LA and LOA contents (r = 0.78 and r = 0.97) and negatively correlated with the OA, EA, tocopherol contents and OSI (r = −0.74, r = −0.67, r = −0.71, r = −0.99 and r = −0.80, respectively). Therefore, with a reduction in the OA content, the PA, LA and LOA contents increased, and the oxidation induction time decreased. This finding indicated that higher PA, LA and LOA contents are not conducive to the maintenance of oxidation stability of oil, which is consistent with the results of previous research (Xu et al., Citation2016; Zhu et al., Citation2013). The OSI was positively correlated with tocopherol and total pherol content (r = 0.83, r = 0.76), that was, the higher the tocopherol and total pherol content, the longer the oxidation induction time, which showed that tocopherol and total pherol can effectively inhibit the oxidation of oil (Huang et al., Citation2022; Liu et al., Citation2017).
Table 4. The correlation between oil quality and OSI value.
4. Conclusion
The main fatty acids detected in the four woody plant oils (pecan, T. grandis, C. oleifera, and walnut) were largely similar and included PA, SA, OA, LA, LOA, and AA. T. grandis oil contains cis-5,11,14-eicosenotrienoic acid, also known as chrysanoic acid, which is a unique fatty acid with high physiological activity. Among the four woody plant oils, the fatty acid composition of pecan oil can be considered to offer optimum health benefits, as it contains the highest UFA and OA contents. During the heating process, pecan oil showed the smallest changes in FFA contents, PVs, UV absorption (K232 and K270), CVs, and OSI, followed by C. oleifera oil and T. grandis oil. Walnut oil showed the greatest changes in these parameters. The fatty acid compositions and contents of the four oils showed no significant changes in response to heating. In all four woody plant seed oils, OSI was significantly positively correlated with the OA content and negatively correlated with PA, LA and LOA contents. PVs were positively correlated with LA and LOA contents but negatively correlated with OA, EA, tocopherol contents and OSI. In all the four oils. FFA contents were significantly positively correlated with SA, EA contents, CVs, total pherol content, K232 and K270. The OSI was positively correlated with tocopherol and total pherol content. The order of oxidation stability of the four woody plant oils from high to low was as follows: pecan oil > C. oleifera oil > T. grandis oil > walnut oil. Among the four seed oils tested, the oil content of pecan kernel was the highest (73.07%), and its oxidation stability was better than that of the other oils. In addition, the fatty acid composition of pecan oil fulfills human dietary needs. Therefore, we conclude that pecan oil is high-quality edible oil, which offers nutritional benefits and can be used in the development of functional oils.
Disclosure statement
No potential conflict of interest was reported by the author(s).
Additional information
Funding
References
- Cao, Y. Q., Ren, H. D., Wang, K. L., & Yao, X. H. (2022). Comprehensive evaluation and analysis of kernel quality of Torreya grandis ‘Merrillii’ from different soil types. Journal of Fruit Science, 39(5), 836–11.
- Cao, W. M., Wang, X., Bao, J., Jin, Q. Z., & Wang, X. G. (2013). Research progresses on lipids rancidity and oxidation evaluation. Creal and Oil, 26(4), 1–5.
- Chen, H. J., Li, X. F., Gao, H. Y., & Fang, X. J. (2013). The analysis of chemical composition and antioxidant activities of phenolic compounds from carya (Carya cathayensis) kernel. Journal of Nuclear Agricultural Sciences, 27(1), 61–67.
- Chen, F. L., Wei, Y. M., & Zhong, G. (2006). Deterioration of soybean oil in deep-frying. China Oils & Fats, 31(8), 53–58.
- Choi, Y., Abdelmegeed, M. A., Akbar, M., & Song, B. J. (2016). Dietary walnut reduces hepatic triglyceride content in high-fat-fed mice via modulation of hepatic fatty acid metabolism and adipose tissue inflammation. The Journal of Nutritional Biochemistry, 30, 116–125. https://doi.org/10.1016/j.jnutbio.2015.12.005
- Chu, L. J., Wang, S. S., Zhang, R., Yin, X. H., Wang, J. L., Li, Q., & Li, H. Y. (2013). Investigation on oxidation stability of different kinds of vegetable oil. Cereal and Food Industry, 20(6), 3–5.
- Conte, L., Milani, A., Calligaris, S., Rovellini, P., & Nicoli, M. C. (2020). Temperature dependence of oxidation kinetics of extra virgin olive oil (EVOO) and shelf-life prediction. Foods, 9(3), 1–3. https://doi.org/10.3390/foods9030295
- David, R. J., & Eric, A. D. (2015). The role of oxygen on lipid oxidation reactions. Annual review of food science and technology. Annual Review of Food Science and Technology, 6(1), 171–190. https://doi.org/10.1146/annurev-food-022814-015532
- Gao, H. Y., Chen, H. J., Mu, H. L., Fang, X. J., & Zhou, Y. J. (2017). Research advances in oxidation and antioxidation of nut products. Journal of Chinese Institute of Food Science and Technology, 17(11), 1–8.
- Gao, P., Liu, R. J., Jin, Q. Z., & Wang, X. G. (2018). Comparison of different processing methods of iron walnut oils (Juglans sigillata): Lipid yield, lipid compositions, minor components, and antioxidant capacity. European Journal of Lipid Science & Technology, 120(9), 1800151. https://doi.org/10.1002/ejlt.201800151
- Gharby, S., Harhar, H., Monfalouti, H. E., Kartah, B., Maata, N., Guillaume, D., & Charrouf, Z. (2012). Chemical and oxidative properties of olive and argan oils sold on the Moroccan market. A comparative study. Mediterranean Journal of Nutrition and Metabolism, 5(1), 31–38. https://doi.org/10.1007/s12349-011-0076-5
- Grilo, F. S., & Wang, S. C. (2021). Walnut (Juglans regia L.) volatile compounds indicate kernel and oil oxidation. Foods, 10(2), 329. https://doi.org/10.3390/foods10020329
- Guillen, M. D., & Goicoechea, E. (2009). Oxidation of corn oil at room temperature: Primary and secondary oxidation products and determination of their concentration in the oil liquid matrix from 1H nuclear magnetic resonance data. Food Chemistry, 116(1), 183–192. https://doi.org/10.1016/j.foodchem.2009.02.029
- Guo, Q., Gao, S., Sun, Y. W., Gao, Y. F., Wang, X. R., & Zhang, Z. S. (2016). Antioxidant efficacy of rosemary ethanol extract in palm oil during frying and accelerated storage. Industrial Crops and Products, 94, 82–88. https://doi.org/10.1016/j.indcrop.2016.08.032
- Hajib, A., Nounah, I., Harhar, H., Gharby, S., Kartah, B., Matthäus, B., Bougrin, K., & Charrouf, Z. (2021). Oil content, lipid profiling and oxidative stability of “Sefri” Moroccan pomegranate (Punica granatum L.) seed oil. OCL-Oilseeds and Fats Crops and Lipids, 28(1), 1–7. https://doi.org/10.1051/ocl/2020069
- Huang, J. H., Chen, C. Y., Zhao, Z. M., Li, X., Wang, X. G., Jin, Q. Z., & Tang, J. J. (2022). Inhibition effect of oryzanol on the degradation of tocopherol and the oxidation kinetic of rice bran oils with different content of oryzanol and tocopherol. European Journal of Lipid Science & Technology, 124(5), 1–8. https://doi.org/10.1002/ejlt.202100155
- Huang, Y. Y., Qi, B. K., Zhao, C. B., Li, Y., & Zhang, S. (2019). Research on stability of different plant oils in the process of accelerated oxidation. Journal of Chinese Institute of Food Science and Technology, 19(1), 243–248.
- ISO 12966-2: 2017. Animal and vegetable fats and oils—gaschromatography of fatty acid methyl esters.
- ISO 3960: 2017. Animal and vegetable fats and oil—Determination of peroxide value—Iodometric (visual) endpoint determination.
- ISO 660: 2020. Animal and vegetable fats and oils—Determination of acid value and acidity.
- Jia, X. D., Wang, T., Zhang, J. Y., Wang, X., Liu, Y. Z., & Guo, Z. R. (2012). A review of progress in Carya illinoensis. Chinese Agricultural Science Bulletin, 28(4), 74–78.
- Kortenska, V. D., Yanishlieva, N. V., Kasaikina, O. T., Totzeva, I. T., & Russina, I. F. (2002). Phenol antioxidant efficiency in various lipid substrates containing hydroxy compounds. European Journal of Lipid Science & Technology, 104(8), 513–519. https://doi.org/10.1002/1438-9312(200208)104:8<513:AID-EJLT513>3.0.CO;2-9
- Leonardis, A. D., & Macciola, V. (2012). Heat-oxidation stability of palm oil blended with extra virgin olive oil. Food Chemistry, 135(3), 1769–1776. https://doi.org/10.1016/j.foodchem.2012.06.046
- Li, T. C., Hou, D. Y., Hui, R. H., & Diao, Q. P. (2020). Study on stability change of cooking oil during heating. Journal of Anshan Normal University, 22(2), 39–41.
- Liu, H., Gao, R. P., & Niu, X. (2011). Research advances in physiological nutritional function of polyunsaturated fatty acids and application. Acultural Food Products Science and Technology, 5(1), 49–52.
- Liu, Y., Wang, C. L., Cao, P. R., Li, J. W., & Liu, Y. F. (2017). Physicochemical properties and oxidative stability evaluation of seven kinds of edible vegetable oils. China Oils & Fats, 42(10), 63–68.
- Lou, H. Q., Song, L. L., Li, X. L., Zi, H. L., Chen, W. J., Gao, Y. D., Zheng, S., Fei, Z. J., Sun, X. P., & Wu, J. S. (2023). The Torreya grandis genome illuminates the origin and evolution of gymnosperm-specific sciadonic acid biosynthesis. Nature Communications, 14, 1315. https://doi.org/10.1038/s41467-023-37038-2
- Luo, H. T., Jia, X. D., Zhai, M., Yi, J. P., Zhang, J. Y., Wang, G., Jia, Z. H., Huang, S. N., Guo, Z. R., & Weng, W. X. (2017). A review of nutritional components in carya illinoinensis. Chinese Agricultural Science Bulletin, 33(8), 39–46.
- Mahmood, G., Mohamad, S., & Gholam, A. P. (2011). Variation in phenolic compounds, ascorbic acid and antioxidant activity of five coloured bell pepper (Capsicum annum) fruits at two different harvest times. Journal of Functional Foods, 3(1), 44–49. https://doi.org/10.1016/j.jff.2011.02.002
- Miraliakbari, H., & Shahidi, F. (2008). Oxidative stability of tree nut oils. Journal of Agricultural and Food Chemistry, 56(12), 4751–4759. https://doi.org/10.1021/jf8000982
- Mohdaly, A. A. A., Sarhan, M. A., Mahmoud, A., Ramadan, M. F., & Smetanska, I. (2010). Antioxidant efficacy of potato peels and sugar beet pulp extracts in vegetable oils protection. Food Chemistry, 123(4), 1019–1026. https://doi.org/10.1016/j.foodchem.2010.05.054
- Ojeda-Amador, R. M., Salvador, M. D., Gómez-Alonso, S., & Fregapane, G. (2018). Characterization of virgin walnut oils and their residual cakes produced from different varieties. Food Research International, 108(Jun), 396–404. https://doi.org/10.1016/j.foodres.2018.03.066
- Pang, X. H., Li, J. J., Wu, Q. J., Lin, L., Du, B., & Li, P. (2021). Comparison of oxidative stability of sacha inchi oil, linseed oil and perilla seed oil. China Oils & Fats, 46(1), 32–37.
- Rudolph, C. J., Odell, G. V., Hinrichs, H. A., Thomson, H. J., & Kays, S. J. (1992). Chemical changes in pecan oils during oxidation. Journal of Food Quality, 15(4), 279–293. https://doi.org/10.1111/j.1745-4557.1992.tb00992.x
- Schulze, M. B., Minihane, A. M., Saleh, R. N. M., & Ulf, R. (2020). Intake and metabolism of omega-3 and omega-6 polyunsaturated fatty acids: Nutritional implications for cardiometabolic diseases. The Lancet Diabetes & Endocrinology, 8(11), 915–930. https://doi.org/10.1016/S2213-8587(20)30148-0
- Seker, M., Engin, G., & Yesiloglu, T. (2022). Investigation of tocopherol composition and total amounts in pecan nut [Carya illinoinensis (Wangenh.) K. Koch] varieties. Journal of Food Agriculture & Environment, 20(2), 91–95.
- Shadyro, O., Sosnovskaya, A., & Edimecheva, I. (2020). Effect of biologically active substances on oxidative stability of flaxseed oil. Journal of Food Science and Technology, 57(1), 243–252. https://doi.org/10.1007/s13197-019-04054-4
- Sun, H., Xu, J., Liu, X. Y., Li, Y. X., & Lei, R. (2017). Fatty acid composition and oxidative stability of agrestal vegetable oils in lijiang. China Oils & Fats, 42(1), 40–42.
- Su, S. W., Wang, R. C., Guo, S. T., Zhang, C., Zhang, T., & Liang, M. (2016). Walnut phenolic compounds: Binding with proteins and antioxidant activities. Transactions of the Chinese Society of Agricultural Engineering, 32(22), 309–314.
- Wang, Y. P., Fei, X. Q., Yao, X. H., Guo, S. H., & Ren, H. D. (2021). Principal component analysis and cluster analysis of fatty acids and triglycerides in oil-tea camellia seeds from different origins. China Oils & Fats, 46(9), 112–119.
- Wang, X. G., Jin, Q. Z., & Chang, M. (2017). Nutrition and health of woody oils. Cereal and Food Industry, 24(1), 8–12.
- Wang, J. F., Tan, X. J., Wu, X. C., Li, Q. P., Zhong, Q. P., Yan, C., & Ge, X. N. (2020). Development status and suggestions of camellia industry in China. World Forestry Research, 33(6), 80–85.
- Wang, J. H., Wang, X., Chen, Q., & Wang, F. X. (2016). Study on oxidation degradation products of linoleic aid. Food and Machine, 32(5), 5–10.
- Wang, Y. P., Yao, X. H., Yang, L., Fei, X. Q., Cao, Y. Q., Wang, K. L., & Guo, S. H. (2021). Effects of harvest time on the yield quality and active substance of Torreya grandis nut and its oil. Journal of Oleo Science, 70(2), 175–184. https://doi.org/10.5650/jos.ess20155
- Wu, X., Beecher, G. R., Holden, J. M., Haytowitz, D. B., Gebhardt, S. E., & Prior, R. L. (2004). Lipophilic and hydrophilic antioxidant capacities of common foods in the United States. Journal of Agricultural and Food Chemistry, 52(12), 4026–4037. https://doi.org/10.1021/jf049696w
- Xu, F., Shi, A. M., Liu, H. Z., Liu, L., & Wang, Q. (2016). The content of fatty acids and endogenous antioxidant components of walnut oil and their correlation with oxidative stability index. Journal of the Chinese Cereals and Oils Association, 31(3), 53–58.
- Yang, K. M., Hsu, F. L., Chen, C. W., Hsu, C. L., & Cheng, M. C. (2018). Quality characterization and oxidative stability of camellia seed oils produced with different roasting temperatures. Journal of Oleo Science, 67(4), 389–396. https://doi.org/10.5650/jos.ess17190
- Yao, X. H., Chang, J., & Wang, K. L. (2014). The research proceeding of pecan in China (1st ed.). Science Press.
- Yuan, J. J., Wang, C. Z., Chen, H. X., Zhou, H., & Ye, J. Z. (2013). Prediction of fatty acid composition in Camellia oleifera oil by near infrared transmittance spectroscopy (NITS). Food Chemistry, 138(2/3), 1657–1662. https://doi.org/10.1016/j.foodchem.2012.11.096
- Zhang, R. M. (2001). The oxidation stability test of oil or fat. Science and Technology of Cereals, Oils and Foods, 9(4), 13–15.
- Zhang, J. F., Wu, Y. J., Zhou, S. M., Xu, L. R., Xie, L. L., Jin, J., Jin, Q. Z., Jiang, Y. R., & Wang, X. G. (2022). Oxidative stability, shelf-life and stir-frying application of Torreya grandis oil. Journal of Chinese Institute of Food Science and Technology, 57(3), 1836–1845. https://doi.org/10.1111/ijfs.15561
- Zhao, G. L., & Lliu, C. C. (2009). A review on phenolic compounds from edible plants and their protective activities in vivo through antioxidation. Biotechnology Bulletin, 25(S1), 40–45.
- Zheng, Y. W., Wu, T. S., Shen, D. Y., Mo, R. H., Tang, F. B., & Liu, Y. H. (2020). Comparison of the quality of ten walnut oils. China Oils & Fats, 45(10), 47–51.
- Zhou, T., & Li, J. Z. (2015). Influence of different medium heating treatment conditions on acid value and carbonyl value of oils. Science and Technology of Cereals, Oils and Foods, 23(2), 40–43.
- Zhou, Y., & Pei, D. (2016). Influence factors of quality and storage stability of walnut oil. China Oils & Fats, 41(1), 60–63.
- Zhu, J. L., Chai, Z. L., Wu, C. R., Huang, Y. T., & Wu, X. Y. (2019). Comprehensive research on the quality of Torreya grandis and its oil in Zhejiang province. Journal of the Chinese Cereals and Oils Association, 34(3), 67–73.
- Zhu, Z. B., Liu, M. Y., Yi, J. J., & Liu, N. (2015). Comparison of physicochemical property, fatty acid composition and oxidative stability of walnut oil from different origins. China Oils & Fats, 40(3), 87–90.
- Zhu, X. M., Wu, J. F., Hu, J. N., Zhao, S. Q., Hu, Z. Y., & Xiong, H. (2013). Antioxidative efficiency of α-tocopherol in peanut oil, sesame oil, and rapeseed oil. Food and Fermentations Industry, 39(10), 85–90.