ABSTRACT
To investigate the effect of adding high-dose docosahexaenoic acid (DHA) and arachidonic acid (ARA) on the stability of infant formula powders (IFs), IFs fortified with high-dose and low-dose DHA and ARA were compared and evaluated during storage at 37°C for six months. We specifically focused on the changes to IFs caking, amino acid content, and 5-hydroxymethylfurfural (HMF) content. We found that during the storage process, there was no significant change in the amino acids of IFs following supplementation with high- or low-doses of DHA and ARA. However, HMF and color difference (∆E) increased with the length of storage time. A comparison between the high-dose and low-dose groups revealed that the addition of high-dose DHA and ARA did not cause an additional increase in the HMF but exacerbated the caking of IFs. The addition of high-dose DHA and ARA did not exacerbate the non-enzymatic browning of IFs.
1. Introduction
Infant formula powders (IFs) are dairy-based products that are carefully designed to meet the nutritional needs of non-breastfed newborns. In most cases, IFs are produced using cow’s milk with the addition of other components, such as whey, lactose, long-chain polyunsaturated fatty acids, lecithin, vitamins, and minerals, to mimic breast milk (Walshe et al., Citation2021; Wu et al., Citation2022; Zou et al., Citation2016). In most IFs, lactose is the main reducing sugar, whereas proteins are found as casein and whey proteins. Due to their high lactose (35.4–51% w/w) and protein (10.5–16.1%, w/w) content, IFs are prone to initiating the Maillard reaction, a complex chemical reaction between amino acids and reducing sugars, when exposed to high temperatures or prolonged storage periods (Ferrer et al., Citation2005; Nasirpour et al., Citation2006; Perez-Locas & Yaylayan, Citation2010). The Maillard reaction is affected by several factors, including pH, temperature, water activity, type of reducing sugar, presence of metals, and lipid oxidation products. This can result in numerous consequences, such as the unavailability of amino acids, solubility loss, increased allergenicity of certain proteins, and even hindrance of mineral absorption (Nunes et al., Citation2019).
Long-chain polyunsaturated fatty acids are added to IFs as they are the main fatty acids in breast milk and are essential for the development of the infant’s central nervous system, retinas, and immune functions (Lien et al., Citation2018; Zou et al., Citation2016). Yuhas et al. (Citation2006) determined the content of docosahexaenoic acid (DHA) and arachidonic acid (ARA) in mature breast milk (>four weeks of lactation) from nine countries (Australia, Canada, Chile, China, Japan, Mexico, the Philippines, the UK, and the U.S.A.). The results showed that DHA content, expressed as weight percentage, ranged from 0.17% to 0.99%, and the ARA content ranged from 0.36% to 0.49%, with ARA levels in most cases being equal to or greater than DHA levels. Therefore, the supply of these fatty acids in IFs (typically using breast milk as a model with ARA greater than DHA is considered important (Lien et al., Citation2018). In China, the regulations on IFs specify that DHA and ARA are optional ingredients. The maximum amount of DHA added to infant formula (0–6-month-old) and follow-up infant formula (older infant formula, 6–12-month-old) was 3.6–9.6 mg/100 kJ, while the amount in young children formula (12–24-month-old) was less than 9.6 mg/100 kJ. At the same time, it is necessary to add at least the same amount of ARA to the formula as DHA (GB 10,765–2021 National food safety standard: Infant formula; GB 10,766–2021 Food Safety National Standard: Older Infant Formula; and GB 10,767–2021 Food Safety National Standard: Young Children Formula). In addition, mothers and caregivers recognize DHA and ARA as beneficial to infant development; therefore, it is rare to see IFs without DHA and ARA being added to the market in China. According to our laboratory’s investigation, currently, the amount of DHA and ARA added to most IFs on the market in China is 80–110 mg/100 g, and the DHA: ARA ratio is 1:1–1:1.7. Thus, the amounts of DHA and ARA added only met the minimum requirements specified by the regulations.
It should be noted that IFs fortified with polyunsaturated fatty acids (PUFAs) are prone to oxidative reactions (Chávez-Servín et al., Citation2009). Moreover, even when low doses of DHA and ARA were added to IFs, the IFs exhibited greater oxidation than the ones without DHA and ARA powders (Maduko & Akoh, Citation2006). However, few studies have reported that lipid oxidation products have catalytic effects on the Maillard reaction (Nunes et al., Citation2019). PUFAs can react with lysine during oxidation, thereby blocking lysine and promoting the Maillard reaction. In the advanced stages of the Maillard reaction, unwanted compounds such as furfural, including 5-hydroxymethyl furfural (HMF), can be generated (Chávez-Servín et al., Citation2009; Nasirpour et al., Citation2006). As an intuitive phenomenon of the Maillard reaction, color change has also been used in recent years to evaluate the degree of the Maillard reaction in IFs (Cheng et al., Citation2019; Jia et al., Citation2019; Nasirpour et al., Citation2006). The above-mentioned indicators can serve as valuable tools for assessing the extent of damage to IFs and for evaluating the degree of the Maillard reaction.
In recent years, most researchers has focused on the Maillard reaction of IFs during processing (i.e. heat treatment), different processing methods (Aalaei et al., Citation2019; Ma et al., Citation2022), and the Maillard reaction of IFs stored in non-commercial packaging (Cheng et al., Citation2019). Few studies have focused on the Maillard reaction during storage of IFs rich in long-chain PUFAs packaged in commercial environments. We investigated whether high doses of DHA and ARA could promote non-enzymatic browning of IFs. This study compared and analyzed the effects of low and high doses of DHA and ARA added to IFs, as well as the changes in milk powder caking, water content/activity, amino acid composition, formation of browning product HMF, and color, to clarify the impact of adding high doses of DHA and ARA on the Maillard reaction of IFs.
2. Materials and methods
2.1. Infant formula powders
Three types of IFs (0–6-month-old infant, 6–12-month-old infant, and 12–36-month-old infant) based on bovine milk, namely IF1, IF2 and IF3, were produced using a wet mixing-spray-drying process (in Bright Dairy & Food Co., Ltd.). IF1 contained (per 100 g of powder): 11.87 g proteins (whey protein: casein = 65:35), 25.6 g total fats (including linoleic acid, 4.18 g, and α-linolenic acid, 0.540 g), and 53.8 g carbohydrates (lactose constituted 96.5%). IF2 contained (per 100 g of powder): 12.98 g proteins (whey protein: casein = 49:51), 21.4 g total fats (including linoleic acid, 3.41 g, and α-linolenic acid, 0.442 g), and 55.1 g carbohydrates (lactose constituted 93.2%). IF3 contained (per 100 g of powder): 14.56 g proteins (whey protein: casein = 45:55), 20.6 g total fats (including linoleic acid, 3.26 g, and α-linolenic acid, 0.420 g), and 55.1 g carbohydrates (lactose constituted 93.1%). Next, through the dry mixing process, IF1, IF2, and IF3 were fortified with different doses of DHA and ARA to form a low-dose group (IF1-L, IF2-L, and IF3-L; additional fortified DHA, 105 mg; ARA, 130 mg per 100 g of milk powder per formula) and a high-dose group (IF1-H, IF2-H, and IF3-H; additional fortified DHA, 168 mg; ARA, 180 mg per 100 g of milk powder per formula). The six formulas were packaged in commercial airtight 800 g containers (sealed metal cans) in an N2-modified atmosphere.
Storage: Samples of six formulas were stored in a constant temperature and humidity storage chamber (37°C, 75% relative humidity) for six months and analyzed just after manufacturing (time zero) and after two, four and six months of storage. Samples were maintained in airtight containers until analysis. Each time, new independent packaging was opened for analysis.
2.2. Caking analysis
The milk powder was gently removed and stored in the storage chamber for zero, two, four, and six months to prevent violent vibrations, after which it was opened and the upper powder was gently shaken to observe caking.
2.3. Water content and water activity determination
The water content (or the dry matter) of the samples was determined using the standard method of the International Dairy Federation (Rückold et al., Citation2000).The water activities of the samples were determined using an Aqua Lab Series 4TE water activity meter (Aqua Lab, Pullman WA. U.S.A.). Before testing, the water activity meter, and the sample to be tested (in the unopened state) were equilibrated in a measuring room with a temperature of 25°C and a relative humidity of 40%-50% for 1–2 hours. All the measurements were performed in duplicate.
2.4. Amino acid analysis
4.5 g of IFs was added to 30 mL of deionized water at a temperature of 40-50°C to ensure complete dissolution, and then detect the content of 18 amino acids was detected according to the detection method described by Feng et al. (Citation2016). All the measurements were performed in duplicate.
2.5. 5-hydroxymethylfurfural (HMF) determination
The HMF content of the IFs was determined using a slightly modified version of the method described by Xing et al. (Citation2021). The powder was diluted 6.5 times with distilled water into 50 mL test tubes, followed by the addition of 5 mL 0.15 M oxalic acid solution (freshly prepared). After sealing with caps, the test tubes were heated in a water bath at 95°C for 25 min and cooled to room temperature. Next, 3 mL of 40% trichloroacetic acid (TCA) solution was added to the cooled sample, which was oscillated for 10 min. The mixture was centrifuged at 6000 rpm for 15 min. The supernatant phase was collected in a 25 mL volumetric flask. Next, 5 mL of 4% TCA solution was added to the solid residue. The solution was then oscillated for 10 min and then centrifuged again. The supernatant was added to the flask, and the solid phase was discarded. The solution was made up to 25 mL with 4% TCA solution in a volumetric flask. The mixture was then filtered through a 0.22-mm polyether sulfone filter before HPLC analysis. All the measurements were performed in duplicate.
2.6. Color analysis
The colors of the samples were determined after sampling according to the method described by Jia et al. (Citation2019). Hunter L, Hunter a and Hunter b values were measured using A ColorFlex EZ spectrophotometer (Hunter Lab, Reston, U.S.A). The results were expressed as L* (lightness ranging from 0% to 150%), a* (color axis ranging from greenness (−a*) to redness (+a*)) and b* (color axis ranging from blueness (−b*) to yellowness (+b*)). All the measurements were performed thrice.
Moreover, the color difference (ΔE) was calculated from the color values (ΔE = [(L0* – Lt*)2 + (a0* – at*)2 + (b0* – bt*)2]1/2), where, L0*, a0*, and b0* are the L*, a *, and b * values measured at 0 d for the milk powder sample and Lt*, at*, and bt* are the L*, a *, and b * values measured at other time points.
2.7. Statistical analysis
All results are presented as means±standard deviation. Statistical analysis was performed using a one-way ANOVA test or paired t-test with IBM SPSS Statistics 24 (IBM Software, Somers, NY, U.S.A.), and the level of significance was determined at p < .05.
3. Results and discussion
3.1. Caking
Caking of the six IFs at different storage times is shown in . Under the storage condition at 37°C, the IF1-L and IF3-L samples did not cake during the entire investigation period (0 months, two months, four months, six months); the samples remained as loose powders. For IF2-L, there was no caking at 0 months, two months and four months during the investigation period, and only slight caking occurred at six months of storage. However, for IF1-H, IF2-H, and IF3-H, caking occurred after two months of storage and continued to increase until six months of storage.
Figure 1. Caking of infant formula powders.
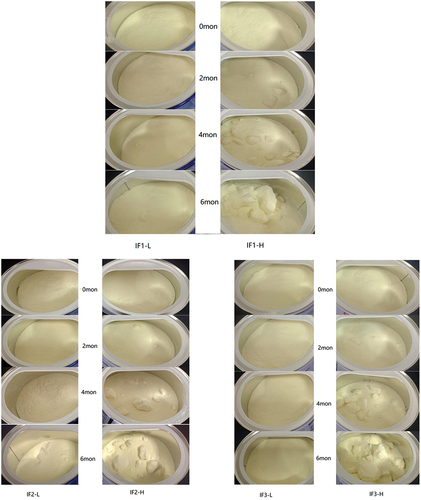
Research has shown that the content of lactose, water, fat, and protein contents affect the caking of milk powder (Fitzpatrick et al., Citation2007; Phosanam et al., Citation2020), and the common causes of caking in IFs include lactose crystallization, moisture absorption and fat bridging (Nasirpour et al., Citation2006; Tham et al., Citation2017). In this study, IF1-H, IF2-H, and IF3-H had higher DHA and ARA contents than the corresponding IF1-L, IF2-L and IF3-L, and the caking of IF1-H, IF2-H, and IF3-H occurred earlier (in the second month), possibly because the high content of PUFAs promoted fat bridging of the powder, thus making the powder more prone to caking. In addition, the protein in infant formula powder is hygroscopic (competes for water), which can slow down the crystallization of lactose, thus preventing the powder from caking (Fitzpatrick et al., Citation2007). Previous studies by Walshe et al. (Citation2021) showed that infant formula powder increased free fat content as protein content decreased. In this study, IF2-L and IF3-L had similar lactose content and fat contents, but the protein content of IF2-L was lower than that of IF3-L, which may explain the slight caking of IF2-L in the sixth month.
3.2. Water content and water activity
shows that the changes in water content are inconsistent during the storage of different IFs. For example, IF1-L and IF1-H show a trend of increasing, decreasing and increasing, respectively. The water content of IF2-L fluctuated slightly around 2.75 g/100 g throughout the storage period, while IF2-H decreased with the extension of the storage period. The water content of IF3-L and IF3-H showed an opposite trend of change. The water content of IF3-L initially decreased (having its lowest water content after two months of storage) and then increased, whereas the water content of IF3-H showed a trend of first increasing (having its highest water content after four months of storage) and then decreased. Unlike the phenomenon in which the water content in different milk powders varies with the storage period, the water activity in different formula milk powders exhibits a similar change trend. Except for IF1-H (which increased throughout the investigation period), the remaining five formula powders first increased and then decreased.
Figure 2. The water content (a) and water activity (b) of IFs storage.
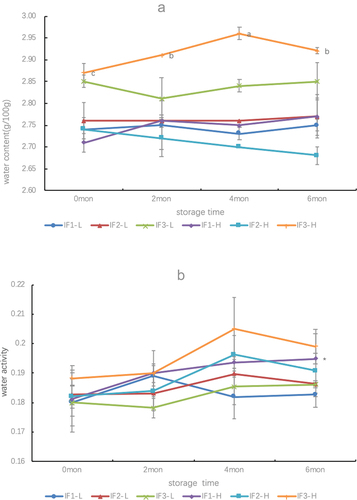
Because these six IFs were stored in closed iron cans, external moisture could not enter the formula through its container. Therefore, the fluctuations in water content and water activity shown in originate from the moisture fluctuations of the milk powder itself. As mentioned previously, IF1-L, IF2-L, IF3-L, IF1-H, IF2-H, and IF3-H are rich in lactose and unsaturated fats. Studies have shown that during long-term storage of milk powder, lactose is prone to lactose crystals (Nasirpour et al., Citation2006; Phosanam et al., Citation2020), unsaturated fats are prone to fat oxidation (Jia et al., Citation2019; Nasirpour et al., Citation2006), and lactose crystals and fat oxidation release water. Moreover, the six types of milk powder are rich in proteins, which, in turn, have water absorption properties (Fitzpatrick et al., Citation2007; Tham et al., Citation2017). Therefore, based on the above factors, the changing trends in water content and water activity may be inconsistent during storage for formulas with different protein, lactose, and fat contents. The above results show that the difference in the amount of DHA and ARA added to the same formula, resulting in seat differences in protein, lactose, and fat content, affects the changing trend of water content and water activity of the formula powder.
3.3. Amino acid
Changes in 18 amino acids during milk powder storage are presented in . During the six-month storage period, the 18 amino acids in the six formula powders did not show significant changes. However, the essential amino acid lysine showed a downward trend in all formulas, with a decreases of 2.8%, 1.9%, 1.7%, 3.2%, 1.1%, and 0.7% in IF1-L, IF2-L, IF3-L, IF1-H, IF2-H, and IF3-H, respectively, after six months of storage. These data are consistent with those of Hodson (Citation1950), who found that the loss of lysine during long-term storage of evaporated milk was less than 4% after 17 months of storage and 17% after 5 years of storage.
Table 1. Amino acid contents of infant formula (0–6-month old) stored at different storage time (mg/100 g).
Table 2. Amino acid contents of older infant formula (6–12- month old) stored at different storage time (mg/100 g).
Table 3. Amino acid contents of young children formula (12–24- month old) stored at different storage time (mg/100 g).
Studies have shown that during long-term storage of milk powder, lysine, histidine, arginine, methionine, and tryptophan can undergo Maillard reactions with reducing sugars (Hodson, Citation1950; Mehta & Deeth, Citation2016). Moreover, lysine is one of the most reactive amino acids in the Maillard reaction; both the α-amino group in the terminal amino acid and the free ɛ-amino group in the side chain can participate in the Maillard reaction. In IFs, lysine can undergo a Maillard reaction with lactose to form Schiff bases. Schiff bases are chemically unstable and can be subjected to additional isomerization, a reaction known as Amadori rearrangement, which leads to the production of lactoulosyl-lysine (Amadori product) (Mehta & Deeth, Citation2016). In addition, lactulosyl-lysine is biologically unavailable; hence, the Maillard reaction reduce the biological value of the protein (Nunes et al., Citation2019). However, the data in show that high-dose DHA and ARA fortified in IFs will not affect the stability of amino acids in milk powder, nor will they affect the Maillard reaction of IFs.
3.4. 5-hydroxymethylfurfural
The HMF content in the studied formulas were expressed as μg/100 g of powder sample (). The initial HMF content of IFs in the study was in the range of 542.1–690.8 μg/100 g; after six months of storage, the content of HMF was 736.8–1370.1 μg/100 g. Across the board, there is a clear tendency for the formation of HMF in IFs as the time of storage increases, and this formation is more pronounced two months after storage. At this timepoint, the HMF of IF2-L and IF2-H were significantly higher than at the starting point (0 months). All the six IFs samples showed a progressive increase in HMF content upon storage, which was consistent with the results of Chávez-Servín et al. (Citation2015) and Albalá-Hurtado et al. (Citation1998), who noted the formation of HMF as storage time increased.
Table 4. HMF contents of IFs stored at different storage time (μg/100 g).
Previous research suggested that whey protein contains higher lysine content than casein; therefore, high whey protein in infant formula can promote the Maillard reaction, leading to high HMF content (Contreras-Calderón et al., Citation2008). This is consistent with the 0-month data in for the low-dose (HMF content IF1-L>IF2-L>IF3-L) and high-dose (IF3-H, which had the lowest whey protein content and also had the lowest HMF content) groups. In addition, we found that when IFs are fortified with DHA and ARA, the HMF content in the high-dose group was lower than that in the low-dose group, especially in the IF2-L, whose HMF content was significantly higher than that in the IF2-H group after six months of storage (). This result differed slightly from that reported by Chávez-Servín et al. (Citation2006). Chávez-Servín showed that the initial HMF content of infant formula with DHA was lower than that of formula without DHA; however, during storage, the HMF content of infant formula with DHA was higher than that of formula without DHA. The slight differences in the above studies may be related to the different formulations selected. Owing to different formulations (e.g. different types of carbohydrates, protein types, and content), the intensity of Maillard reactions during storage may vary (Chávez-Servín et al., Citation2015).
3.5. Color
With respect to the color parameter, the L* values of IFs with low DHA and ARA content significantly decreased after two months of storage, whereas the decreases did not reach significance in the other samples (high-dose group). For the a* value, after storage for two months in the low-dose and the high-dose groups, except for IF2-H and IF3-L, all samples showed significant increases. However, the change trend of the b* value after two months of storage was irregular, showing a significant decrease in the IF1-L group and a significant increase in the IF2-L and IF3-L groups, whereas there was no significant change in the high-dose group (). Notably, for all three infant formula powders in each group, the overall change in the intensity of L*, a*, and b* was IF1>IF2>IF3, as shown in . As shown in , the color difference (ΔE) of the six types of infant formula milk powders showed a slight increase with storage time. However, IF1-L and IF3-L, which had low DHA and ARA levels, showed a rapid increase from the start of storage until the sixth month.
Table 5. The color changes of IFs stored at different storage time.
Previous studies have shown that the relative importance of lipid oxidation and browning reactions for IFs during storage of established formulations strongly depends on temperature and water activity (Cheng et al.,Citation2019, Citation2017; Zhu et al. Citation2017). At the higher storage water activity, lactose absorbs more water, promoting the amorphous lactose to undergo crystallization, further increasing non-enzymatic Maillard reactions, and leading to a brown color (Kim et al., Citation1981; Tham et al., Citation2017). In this study, we observed that the water activity of the formulas in the high-dose group was higher than that in the low-dose group, but the one-way ANOVA test showed that there was no significant difference in the water activity of all formulas throughout the entire observation period, and a paired t-test showed that at 0 month, there was no significant difference among the three groups (IF1-L vs. IF1-H, IF2-L vs. IF2-H, and IF3-L vs. IF3-H), which may be the reason why there was no significant difference in b* among the high-dose group formulas during the six-month storage period.
In addition, the color difference (∆E) of the six formulations was less than 1 during the entire storage period. This indicated that only slight browning reactions, difficult to distinguish by the human eye, had occurred in the six formulations. Ramirez-Jimenez et al. (Citation2004) found that for infant cereals packaged under industrial conditions (e.g. nitrogen atmosphere), the change in ∆E is less than 1 when stored at 32°C for 12 months. However, if the sample is stored at room temperature for one month under laboratory conditions (modified aw 0.65), its ∆E will be higher than 1, and when stored for four months, its ∆E will be higher than 3, indicating that nitrogen-filled, oxygen-isolated, and water-isolated packaging can help prevent the occurrence of the Maillard reaction. This is consistent with the change in low ∆E obtained in this study using industrial packaging (e.g. iron cans with nitrogen atmosphere) of infant formula milk powder.
4. Conclusion
In this study, we analyzed the effects of low and high doses of DHA and ARA added to IFs, along with changes in milk powder caking, water content/activity, amino acid composition, formation of browning product HMF, and color, to elucidate the impact of adding high doses of DHA and ARA on the Maillard reaction of IFs. Contrary to expectations, the addition of high-dose of DHA and ARA to IFs did not affect the stability of amino acids, and even the lysine involved in the Maillard reaction did not change significantly in the high-dose group. In addition, HMF, the indicator of Maillard reaction, increased significantly with the storage time (with the exception of IF3-H). However, the addition of high doses of DHA and ARA did not accelerate HMF formation. In contrast, after six months of storage, the HMF content of IF2-L was significantly greater than that of IF2-H. Similarly, the L *, a *, and b * values in the high-dose group of IFs are more stable. However, the results showed that the high-dose group of IFs was more prone to caking. Thus, the addition of high-dose DHA and ARA did not exacerbate the non-enzymatic browning of IFs but exacerbated the caking of IFs.
Author contributions
Jia Hong-xin and Su Mi-ya designed the study. Jia Hong-xin and Su Mi-ya wrote the manuscript and were primarily responsible for its final content. Liu Hai-an collected test data and discussed over the course of the study. All the authors have read and approved the final final version of the manuscript.
Acknowledgments
The authors thank Brightdairy (Shanghai, China) for providing samples of infant formula powders.
Disclosure statement
No potential conflict of interest was reported by the author(s).
Additional information
Funding
References
- Aalaei, K., Sjöholm, I., Rayner, M., Teixeira, C., Tareke, E., & Banoub, J. (2019). Early and advanced stages of Maillard reaction in infant formulas: Analysis of available lysine and carboxymethyl-lysine. PLOS ONE, 14(7), e0220138. https://doi.org/10.1371/journal.pone.0220138
- Albalá-Hurtado, S., Veciana-Nogués, M. T., Mariné-Font, A., & Vidal-Carou, M. C. (1998). Changes in furfural compounds during storage of infant milks. Journal of Agricultural and Food Chemistry, 46(8), 2998–9. https://doi.org/10.1021/jf980079f
- Chávez-Servín, J. L., Castellote, A. I., & Lopez-Sabater, M. C. (2006). Evolution of potential and free furfural compounds in milk-based Infant Formula during storage. Food Research International, 39(5), 536–543. https://doi.org/10.1016/j.foodres.2005.10.012
- Chávez-Servín, J. L., Castellote, A. I., Martin, M., Chifré, R., & Carmen López-Sabater, M. C. (2009). Stability during storage of LC-PUFA-supplemented infant formula containing single cell oil or egg yolk. Food Chemistry, 113(2), 484–492. https://doi.org/10.1016/j.foodchem.2008.07.082
- Chávez-Servín, J. L., de la Torre Carbot, K. D. L. T., Garcia-Gasca, T., Castellote, A. I., & Lopez-Sabater, M. C. (2015). Content and evolution of potential furfural compounds in commercial milk-based infant formula powder after opening the packet. Food Chemistry, 166(janvier 1), 486–491. https://doi.org/10.1016/j.foodchem.2014.06.050
- Cheng, H., Erichsen, H., Soerensen, J., Petersen, M. A., & Skibsted, L. H. (2019). Optimising water activity for storage of high lipid and high protein infant formula milk powder using multivariate analysis. International Dairy Journal, 93, 92–98. https://doi.org/10.1016/j.idairyj.2019.02.008
- Cheng, H., Zhu, R. G., Erichsen, H., Soerensen, J., Petersen, M. A., & Skibsted, L. H. (2017). High temperature storage of infant formula milk powder for prediction of storage stability at ambient conditions. International Dairy Journal, 73, 166–174. https://doi.org/10.1016/j.idairyj.2017.05.007
- Contreras-Calderón, J., Guerra-Hernández, E., & García-Villanova, B. (2008). Indicators of non-enzymatic browning in the evaluation of heat damage of ingredient proteins used in manufactured infant formulas. European Food Research and Technology, 227(1), 117–124. https://doi.org/10.1007/s00217-007-0700-2
- Feng, P., Gao, M., Burgher, A., Zhou, T. H., & Pramuk, K. (2016). A nine-country study of the protein content and amino acid composition of mature human milk. Food and Nutrition Research, 60(1), 31042. https://doi.org/10.3402/fnr.v60.31042
- Ferrer, E., Alegría, A., Farré, R., Abellán, P., & Romero, F. (2005). High-performance liquid chromatographic determination of furfural compounds in infant formulas during full shelf-life. Food Chemistry, 89(4), 639–645. https://doi.org/10.1016/j.foodchem.2004.05.040
- Fitzpatrick, J. J., Hodnett, M., Twomey, M., Cerqueira, P. S. M., O“flynn, J., & Roos, Y. H. (2007). Glass transition and the flowability and caking of powders containing amorphous lactose. Powder Technology, 178(2), 119–128. https://doi.org/10.1016/j.powtec.2007.04.017
- Hodson, A. Z. (1950). Amino acid content of evaporated milk on prolonged storage. Industrial and Engineering Chemistry, 42(4), 694–695. https://doi.org/10.1021/ie50484a036
- Jia, H. X., Chen, W. L., Qi, X. Y., & Su, M. Y. (2019). The stability of milk-based infant formulas during accelerated storage. CyTa–Journal of Food, 17(1), 96–104. https://doi.org/10.1080/19476337.2018.1561519
- Kim, M. N., Saltmarch, M., & Labuza, T. P. (1981). Non-enzymatic browning of hygroscopic whey powders in open versus sealed pouches. Journal of Food Pro Cessing and Preservation, 5(1), 49–57. https://doi.org/10.1111/j.1745-4549.1981.tb00619.x
- Lien, E. L., Richard, C., & Hoffman, D. R. (2018). DHA and ARA addition to infant formula: Current status and future research directions. Prostaglandins, Leukotrienes and Essential Fatty Acids, 128, 26–40. https://doi.org/10.1016/j.plefa.2017.09.005
- Ma, X., Ali, M. M., Liang, J., Du, Z., & Shen, Z. (2022). Analysis of the evolution of potential and free furfural compounds in the production chain of infant formula and risk assessment. Food Chemistry, 368, 130814. https://doi.org/10.1016/j.foodchem.2021.130814
- Maduko, Y., & Akoh, C. C. (2006). Effect of EPA and DHA fortification on the oxidation stability of caprine milk infant formula analogs ADSA/ASAS Joint Meeting.
- Mehta, B. M., & Deeth, H. C. (2016). Blocked lysine in dairy products: Formation, occurrence, analysis, and nutritional implications. Comprehensive Reviews in Food Science and Food Safety, 15(1), 206–218. https://doi.org/10.1111/1541-4337.12178
- Nasirpour, A., Scher, J., & Desobry, S. (2006). Baby foods: Formulations and interactions (a review). Critical Reviews in Food Science and Nutrition, 46(8), 665–681. https://doi.org/10.1080/10408390500511896
- Nunes, L., Martins, E., Tuler Perrone, Í., & Fernandes de Carvalho, A. (2019). The Maillard reaction in powdered infant formula. Journal of Food and Nutrition Research, 7(1), 33–40. https://doi.org/10.12691/jfnr-7-1-5
- Perez-Locas, C., & Yaylayan, V. A. (2010). The Maillard reaction and food quality deterioration. In L. H. Skibsted, J. Risbo, & M. L. Andersen (Eds.), Chemical deterioration and physical instability of food and beverages (pp. 70–94). https://doi.org/10.1533/9781845699260.1.70
- Phosanam, A., Chandrapala, J., Huppertz, T., Adhikari, B., & Zisu, B. (2020). Changes in physicochemical and surface characteristics in model infant milk formula powder (imf) during storage. Drying Technology, 39(15), 2119–2129. https://doi.org/10.1080/07373937.2020.1755978
- Ramirez-Jimenez, A., Garcia-Villanova, B., & Guerra-Hernandez, E. J. (2004). Nonenzymatic browning during storage of infant cereals. Cereal Chemistry, 81(3), 399–403. https://doi.org/10.1094/CCHEM.2004.81.3.399
- Rückold, S., Grobecker, K. H., & Isengard, H. D. (2000). Determination of the contents of water and moisture in milk powder. Fresenius’ Journal of Analytical Chemistry, 368(5), 522–527. https://doi.org/10.1007/s002160000511
- Tham, T. W. Y., Xu, X., Yeoh, A. T. H., & Zhou, W. (2017). Investigation of caking by fat bridging in aged infant formula. Food Chemistry, 218, 30–39. https://doi.org/10.1016/j.foodchem.2016.09.043
- Tham, T. W. Y., Yeoh, A. T. H., & Zhou, W. (2017). Characterisation of aged infant formulas and physicochemical changes. Food Chemistry, 219, 117–125. https://doi.org/10.1016/j.foodchem.2016.09.107
- Walshe, E. J., O’Regan, J., & O’Mahony, J. A. (2021). Influence of protein content and profile on the processing characteristics and physical properties of model infant formula powders. International Journal of Dairy Technology, 74(3), 592–599. https://doi.org/10.1111/1471-0307.12788
- Wu, F. Y., Chen, F., Pu, Y. Z., Qian, F., Leng, Y. B., Mu, G. Q., & Zhu, X. M. (2022). Effects of soy lecithin concentration on the physicochemical properties of whey protein isolate, casein-stabilised simulated infant formula emulsion and their corresponding microcapsules. International Journal of Dairy Technology, 75(3), 513–526. https://doi.org/10.1111/1471-0307.12861
- Xing, Q., Fu, X., Liu, Z., Cao, Q., & You, C. (2021). Contents and evolution of potential furfural compounds in milk-based formula, ultra-high temperature milk and pasteurised yoghurt. International Dairy Journal, 120, 105086. https://doi.org/10.1016/j.idairyj.2021.105086
- Yuhas, R., Pramuk, K., & Lien, E. L. (2006). Human milk fatty acid composition from nine countries varies most in DHA. Lipids, 41(9), 851–858. https://doi.org/10.1007/s11745-006-5040-7
- Zhu, R. G., Cheng, H., Li, L., Erichsen, H. R., Petersen, M. A., Soerensen, J., & Skibsted, L. H. (2017). Temperature effect on formation of advanced glycation end products in infant formula milk powder. International Dairy Journal, 77, 1–9. https://doi.org/10.1016/j.idairyj.2017.09.005
- Zou, L., Pande, G., & Akoh, C. C. (2016). Infant formula fat analogs and human milk fat: New focus on infant developmental needs. Annual Review of Food Science and Technology, 7(1), 139–165. https://doi.org/10.1146/annurev-food-041715-033120