ABSTRACT
Extracts from powders of geminated brown rice (Oryza sativa L.), Moringa oleifera, and Cordyceps militaris were combined in various ratios and their health-beneficial properties were investigated. All combined extracts exerted the synergistic effects on antioxidant activity as well as inhibitions against α-amylase and α-glucosidase. Extract formulas T4 (70% germinated brown jasmine rice, 20% M. oleifera dry leaves, and 10% C. militaris) and T2 (80% germinated brown jasmine rice, 10% M. oleifera dry leaves, and 10% C. militaris) elicited the significantly highest antioxidant activity and the significantly highest inhibition against α-glucosidase, respectively. Both formulas exhibited the significantly highest inhibition against α-amylase. As compared to each other, extract formulas T4 and T2 possessed the higher glucose consumption-promoting and antiinflammatory activities, respectively. Both formulas were not toxic to normal RAW-264.7 and SW-480 human colon cancer cells. Bioactive compounds with health-supporting properties were identified using UHPLC-QTOF-MS/MS. These combined extracts can be promising therapeutic food.
1. Introduction
The development of novel traditional drugs having antioxidant, antidiabetic, antiinflammatory, and anticancer properties is warranted due to the lack of effective therapies for some chronic diseases (such as skin diseases, respiratory diseases, hypertension, diabetes, and cancer) and the growing interest in alternative or traditional medicine (Nallathamby et al., Citation2015; Sreelatha & Padma, Citation2009; Thurber & Fahey, Citation2009), Even though some synthetic agents are provided, problems related to their toxicity are concerned. Therefore, the growing attention has been paid to phytochemical-based natural products which are safe and also effective. Additionally, natural products can be easily developed as food supplements with potent marketability.
Oryza sativa L. (rice; Thai jasmine rice) is a species of rice that is consumed by nearly half of the world’s population (Suryanti et al., Citation2020). Rice contains various phenolic compounds which possess antioxidant, antidiabetic, antiinflammatory, and anticancer properties, contributing to its potential to prevent cardiovascular diseases and reduce the risk of diabetes and fatty liver diseases (Aalim & Luo, Citation2021; Chmiel et al., Citation2018; Hu et al., Citation2003; Limtrakul et al., Citation2016; Suryanti et al., Citation2020; Walter & Marchesan, Citation2011). The ethanol extracts from brown rice and rice bran of Thai jasmine rice cultivar Dawk Mali 105 contained total phenolic contents at concentrations of 18.97 ± 1.64 and 23.27 ± 1.88 mg gallic acid equivalent (GAE)/g, respectively, while contained total flavonoid contents at concentrations of 19.73 ± 1.04 and 26.01 ± 1.30 mg rutin hydrate equivalent/g, respectively. The compounds in both groups contributed to the antioxidant activity against 2,2-diphenyl-1- picrylhydrazyl (DPPH) and superoxide (O2−) (Hansakul et al., Citation2011). The ethanol extract from rice bran of Khao Dawk Mali 105 contained total phenolic content and total flavonoid content at concentrations of 15.38 ± 0.94 mg GAE/g and 4.36 ± 0.09 mg catechin equivalent (CE)/g, respectively. The phenolic compounds were identified as caffeic acid, epigallocatechin gallate, o-coumaric acid, quercetin, phytic acid, ferulic acid, chlorogenic acid, kaempferol, hydroxybenzoic acid, rosmarinic acid, catechin, p-coumaric acid, naringin, and naringenin. Among these compounds, phytic acid had the highest concentration (Wisetkomolmat et al., Citation2023).
Brown rice is a rice grain in which bran layers remain intact (Cho & Lim, Citation2016). Brown rice has been reported as a rich source of essential nutrients, vitamins, minerals, dietary fiber, protein, carbohydrates, polyphenols, phytosterols, phytoestrogens, phytic acid, γ-oryzanol, and tricin (Upadhyay & Karn, Citation2018). Germination, a process whereby rice absorbs water and its roots grow outward, improved sensory and nutritional qualities (Ren et al., Citation2020). The extracts from rice, brown rice, and germinated brown rice have exhibited health-beneficial effects such as antioxidant (Kaur et al., Citation2017; Summpunn et al., Citation2022; Suryanti et al., Citation2020; Tyagi et al., Citation2022), α-amylase and α-glucosidase inhibitory (Aalim & Luo, Citation2021; Agustin et al., Citation2021; Quan et al., Citation2019; Ramli & Zin, Citation2018; Sarnthima et al., Citation2020), and antiinflammatory activities (Limtrakul et al., Citation2016; Piazza et al., Citation2022).
Moringa oleifera (moringa) has been used as a traditional indigenous medicine in Asian countries against various ailments including diabetes, inflammatory and infectious diseases, cancer, asthma, infertility, hyperglycemia, dyslipidemia, flu, heartburn, syphilis, malaria, diarrhea, scurvy, and skin diseases (Gopalakrishnan et al., Citation2016; Morimitsu et al., Citation2000; Segwatibe et al., Citation2023; Sreelatha & Padma, Citation2009). The extracts from M. oleifera have been reported for their abilities to scavenge free radicals (Santos et al., Citation2012; Xu, Y. B. et al., Citation2019), inhibit α-amylase and α-glucosidase activities (Karray et al., Citation2022; Magaji et al., Citation2020; Ogundipe et al., Citation2022), decrease blood glucose concentration (Tang et al., Citation2017), ameliorate diabetes (Sissoko et al., Citation2020), reduce blood pressure and cholesterol (Gopalakrishnan et al., Citation2016) as well as prevent neurodegenerative disorders and infections (Mundkar et al., Citation2022; Xiao et al., Citation2020).
Cordyceps mushrooms, especially Cordyceps militaris, have received extensive attention due to their potent pharmacological activities (Lee et al., Citation2015). The extracts from C. militaris have been reported for various health benefits such as antioxidant, antidiabetic, antiinflammatory, anticancer, antitussive, antibacterial, and immune-regulating properties (Awang et al., Citation2021; Nallathamby et al., Citation2015; Qu et al., Citation2022; Quan et al., Citation2022).
Even though the extracts from rice, M. oleifera, and C. militaris have been reported for their antioxidant, antidiabetic, antiinflammatory, and anticancer activities and their bioactive compounds have been identified, there is no demonstration and evaluation of the combined ingredients in terms of synergistic effects on such activities. We therefore undertook the present study to find out the combination ratios that conferred the greatest effects. This is the first study examining the health-promoting properties of the combined extracts from germinated brown rice, M. oleifera dry leaves, and C. militaris dry fruiting bodies.
2. Materials and methods
2.1. Material preparation
Germinated brown jasmine rice (a product of B-Herbs brand, Thailand) was purchased from a convenience store in Nakhon Pathom province of Thailand, and then roasted in an oven at 180°C for 20 min (Aalim & Luo, Citation2021). Thai jasmine rice was chosen because it is the most consumed rice in Thailand and well-known worldwide. Evaluation of health-beneficial properties will add market value to Thai jasmine rice and provide other utilization strategies. M. oleifera leaves and C. militaris fruiting bodies were purchased from Thai traditional drug stores in Lopburi and Nakhon Pathom provinces of Thailand, respectively, and then oven-dried at 50°C for 2 days. These ingredients were ground with mortar and pestle, and then passed through a 60-mesh sieve to obtain fine powders. The powder of each ingredient was separately kept in polyethylene bags and stored in a desiccator to avoid ambient moisture absorption and dust contamination.
2.2. Experimental design and extract preparation
The powders of roasted germinated brown jasmine rice, M. oleifera dry leaves, and C. militaris dry fruiting bodies were combined in various ratios to prepare six test formulas (T1-T6). Three control formulas (C1-C3) were prepared from single ingredients. The proportions of ingredients in nine extract formulas and extraction yields are shown in . The combination ratios of extract formulas were varied by using germinated brown jasmine rice as a main ingredient, ranging from 60 to 85%, and M. oliefera dry leaves and C. militaris dry fruiting bodies as supplementary ingredients. The ingredients of each test formula were thoroughly mixed. The powders of combined and single ingredients (100 g) were extracted with 1 l of 80% (v/v) ethanol, and then incubated at 4°C with shaking at 120 rpm for 10 h. After an incubation period, the suspensions were centrifuged at 10,000 rpm for 30 min to pellet the powders. The obtained supernatants containing the extracted compounds were evaporated under vacuum using a rotary evaporator (IKA Vacstar, IKA® Works, Bangkok, Thailand). The residual solutions were lyophilized using a freeze dryer (Labconco FreeZone6, Labconco Corp., Kansas City, MO, U.S.A.). The saturated extracts were kept at 4°C for subsequent analyses (Nallathamby et al., Citation2015; Siddhuraju & Becker, Citation2003).
Table 1. Proportion of each ingredient (%) in each extract formula and extraction yields.
2.3. Antioxidant activity assessed by DPPH assay
The nine extract formulas were determined for their antioxidant activity. The saturated extracts were prepared to be concentrations of 0.1, 1, 5, and 10 mg/ml. Thirty µl of the saturated extract and 270 µl of DPPH solution in methanol were added into each well of a 96-well microplate and thoroughly mixed. The microplates were allowed to stand at 20°C for 30 min in the dark. The absorbances were read with a microplate reader (Multiskan Skyhigh, Thermo Fisher Scientific Inc., Waltham, MA, U.S.A.) at 517 nm. The absorbance readings were compared with those of the negative control (reactions with methanol instead of the extract) and the positive control (reactions with ascorbic acid, at concentrations of 0.003, 0.03, and 0.3 mg/ml, instead of the extract). The 50% inhibitory concentration (IC50) values were calculated as previously described. Inhibition percentages were plotted against extract concentrations. Two points enclosing an inhibition percentage of 50 were selected to draw the regression line and formulate the regression equation. The IC50 value was the concentration calculated from the regression equation, when an inhibition percentage was substituted with 50 (Shimamura et al., Citation2014).
2.4. Antidiabetic activity assessed by α-amylase inhibition assay
The nine extract formulas were determined for their α-amylase inhibitory activity. The saturated extracts were prepared to be concentrations of 120, 160, 200, and 280 mg/ml. Five hundred μl of the saturated extract was added into a test tube filled with 500 μl of 0.02 M sodium phosphate buffer (pH 6.9 with 0.006 M NaCl) containing 0.5 mg/ml α-amylase and thoroughly mixed. The test tubes were incubated at 25°C for 10 min. After the first incubation, 500 μl of 1% starch solution in 0.02 M sodium phosphate buffer (pH 6.9 with 0.006 M NaCl) was added into each test tube. The reaction mixtures were further incubated at 25°C for 10 min. The reactions were stopped by adding 1.0 ml of dinitrosalicylic acid (DNSA) as a color reagent. The test tubes were then incubated in a boiling water bath for 5 min and cooled to room temperature. The reaction mixtures were diluted by adding 10 ml of distilled water. The negative and positive controls were carried out in which buffer solution and acarbose, respectively, were used instead of the extract. The absorbances were measured at 540 nm. The IC50 values were calculated as previously described (Kusano et al., Citation2011).
2.5. Antidiabetic activity assessed by α-glucosidase inhibition assay
The nine extract formulas were determined for their α-glucosidase inhibitory activity. The saturated extracts were prepared to be concentrations ranging from 1.0 to 3.5 mg/ml (with 0.5 interval). Fifty μl of the saturated extract and 100 μl of 0.1 M phosphate buffer (pH 6.9) containing 1.0 U/ml α-glucosidase were added into each well of a 96-well microplate and thoroughly mixed. The microplates were incubated at 25°C for 10 min. After the first incubation, 50 μl of 5 mM p-nitrophenyl-α-D-glucopyranoside in 0.1 M phosphate buffer (pH 6.9) was added into each well. The reaction mixtures were further incubated at 25°C for 5 min. The negative and positive controls were carried out in which buffer solution and acarbose, respectively, were used instead of the extract. The absorbance readings were recorded at 405 nm before and after incubation by a microplate reader (Multiskan Skyhigh, Thermo Fisher Scientific Inc., Waltham, MA, U.S.A.). The IC50 values were calculated as previously described (Striegel et al., Citation2015). Two extract formulas exerting the highest antioxidant and enzyme-inhibitory activities were selected for all following studies.
2.6. Antidiabetic activity assessed by cellular glucose consumption assay
Each well of a 96-well microplate was seeded with 3T3-L1 preadipocyte cells (ATCC® cL-173™) which were cultured in Dulbecco’s modified Eagle’s medium (DMEM) supplemented with 10% fetal bovine serum (FBS), 2 mM L-glutamine, 100 U/ml penicillin, 100 U/ml streptomycin, and 3 mg/ml glucose. The cell density was 1 × 105 cells/well. The extracts were two-fold diluted to be concentrations ranging from 4.88 to 625 µg/ml. Cells were treated with the extracts and incubated under 5% CO2 at 37°C for 24 h. Then, 10 µl of the supernatants were used in the glucose oxidase-peroxidase (GOD-POD) assay (Zhang et al., Citation2008) in order to measure glucose concentration. The absorbances of the solutions were measured at 510 nm. The percentages of cellular glucose consumption and IC50 values were calculated as previously described (Wan et al., Citation2017; Zhang et al., Citation2008). Metformin at concentrations ranging from 6.25 to 100 μg/ml was used for the positive control.
2.7. Antiinflammatory activity assessed by nitric oxide (NO) inhibition assay
Each well of a 96-well microplate was seeded with normal RAW-264.7 mouse macrophage cells (ATCC® TIB-71™) which were cultured in DMEM supplemented with 10% FBS, 2 mM L-glutamine, 100 U/ml penicillin, and 100 U/ml streptomycin. The cell density was 4 × 104 cells/well. The extracts were two-fold diluted to be concentrations ranging from 4.88 to 625 µg/ml. Cells were treated with 1 µg/ml lipopolysaccharide (LPS) to induce inflammation and then exposed to the extracts for 24 h. The 50-µl supernatants were transferred to a new 96-well microplate and 50 μl of Griess reagent was added into each well. The absorbances were measured at 540 nm. The percentages of inhibition and IC50 values were calculated as previously described (Kang et al., Citation2016; Rujanapun et al., Citation2022). Indomethacin at concentrations ranging from 6.25 to 100 μg/ml was used for the positive control.
2.8. Cytotoxicity assay
Each well of a 96-well microplate was individually seeded with normal RAW-264.7 mouse macrophage cells (ATCC® TIB-71™) and SW-480 human colon cancer cells (ATCC® CCL-228TM) which were cultured in DMEM supplemented with 10% FBS, 2 mM L-glutamine, 100 U/ml penicillin, and 100 U/ml streptomycin. The density was 4 × 104 cells/well. The extracts were two-fold diluted to be concentrations ranging from 4.88 to 5,000 µg/ml. Cells were incubated under 5% CO2 at 37°C for 24 h. After an incubation period, a microplate was centrifuged to pellet cells and the supernatants were removed. Cells were washed with phosphate-buffered saline (PBS). Fresh medium and the extracts were added. Cells were further incubated under the same condition for 24 h. Then 100 μl of 0.5 mg/ml 3-(4,5-dimethylthiazol-2-yl)-2,5-diphenyltetrazolium bromide (MTT) was added and cells were further incubated under the same condition for 24 h. At the end of reaction, 99.5% dimethyl sulfoxide (DMSO) was added to dissolve formazan, a reaction product. The absorbances of the solutions were measured at 570 nm to determine the concentrations of formazan. The percentages of cell viability and IC50 values were calculated as previously described (Fares et al., Citation2015; Nam et al., Citation2017). Doxorubicin at concentrations ranging from 6.25 to 100 μg/ml was used for the positive control.
2.9. Chemical profiles assessed by ultrahigh-performance liquid chromatography-quadrupole time-of-flight mass spectrometry (UHPLC-QTOF-MS/MS) analysis
The Agilent 6500 series LC Q-TOF system with a column (EC-C18; 2.1 × 150 mm, 2.7 µm) (Agilent Technologies, Inc., Santa Clara, CA, U.S.A.) was used. The mobile phase was composed of solvent A (0.1% formic acid in water) and solvent B (0.1% formic acid in acetonitrile) at a flow rate of 200 µl/min. Column elution was started with a linear gradient from 95% solvent A and 5% solvent B to 17% solvent B over 10 min, followed by a linear gradient to 100% solvent B over 20 min. The injection volume was 1.0 μl for measurements in both positive and negative modes. The instrument parameters were as follows: gas temperature of 300°C, gas flow rate of 10 l/min, and nebulizer pressure of 35 psig. The scanning range was set between 50 and 1,100 m/z and the scan rate was 3 spectra/sec. The collision-induced dissociation (CID) energies were fixed at 10, 20, and 40 eV. The reference mass ranges were performed from 121.0508 to 922.0097 m/z and from 112.9855 to 1033.9881 m/z for positive and negative acquisition, respectively. The Mass Hunter Qualitative Analysis software version B.08.00 (Agilent Technologies, Inc., Santa Clara, CA, U.S.A.) was used for the initial processing of the LC-MS/MS data. Bioactive compounds present in the extracts were identified using the Molecular Feature Extraction (MFE) tool in the software by comparison of their pattern spectra with those of the reference compounds in the database.
2.10. Statistical analysis
The data analysis was processed with the SPSS statistical software version 19.0 (IBM Corp., Chicago, IL, U.S.A.). All data analyses were performed with triplicate samples. A randomized complete block design (RCBD) was employed to evaluate the properties of extract formulas including antioxidant activity against DPPH as well as inhibitory activities against α-amylase and α-glucosidase. Significant differences among means were analyzed using analysis of variance (ANOVA) followed by Duncan’s post hoc test. The p-value was considered indicative of significance if it was equal to or less than 0.05.
3. Results
3.1. Antioxidant activity against DPPH
The results of DPPH and enzyme inhibition assays were represented by IC50 values which were calculated from the regression equations. The RCBD analysis indicated the different effects of extract formulas on biological activities (p = .00). The lower IC50 value represents the higher antioxidant and enzyme-inhibitory activities of extract formula. The IC50 values of antioxidant activity of all extract formulas ranged from 2.27 ± 0.10 to 10.06 ± 0.50 mg/ml (). Extract formula T4 exerted the significantly lowest IC50 value, followed by extract formula T2 whereas extract formula C1 exerted the significantly highest IC50 value. All test formulas (T1-T6), which were from the combined ingredients, exerted the IC50 values that were significantly lower than those of all control formulas (C1-C3), which were from the single ingredients.
Table 2. IC50 values against DPPH (for antioxidant activity), α-amylase, and α-glucosidase (for antidiabetic activity) of each extract formula.
3.2. α-Amylase inhibitory activity
The results of α-amylase inhibitory activity of all nine extract formulas are shown in . The IC50 values ranged from 186.25 ± 1.52 to 279.34 ± 3.39 mg/ml. Extract formula T2 exhibited the lowest IC50 value which was not significantly different from that of extract formula T4. Thus, extract formulas T2 and T4 shared the highest rank of α-amylase inhibitory activity. All test formulas (T1-T6), which were from the combined ingredients, exhibited the IC50 values that were obviously significantly lower than those of all control formulas (C1-C3), which were from the single ingredients.
3.3. α-Glucosidase inhibitory activity
As shown in , the IC50 values obtained from all nine extract formulas ranged from 2.47 ± 0.01 to 4.81 ± 0.12 mg/ml. Extract formula T2 exhibited the significantly lowest IC50 value, indicating the significantly highest inhibition against α-glucosidase, followed by extract formula T4. All test formulas (T1-T6), which were from the combined ingredients, exhibited the IC50 values that were significantly lower than those of all control formulas (C1-C3), which were from the single ingredients.
3.4. Enhancement of cellular glucose consumption
According to the results of the prior steps, extract formulas T2 and T4 were the most effective for antioxidant and antidiabetic activities (as based on inhibitions of α-amylase and α-glucosidase), and thus were selected to study their other health-promoting properties, including enhancement of cellular glucose consumption, antiinflammatory activity, and cytotoxicity, as well as to identify bioactive compounds. The results showed that both extract formulas enhanced cellular glucose consumption by 3T3-L1 preadipocyte cells in a dose-dependent manner. As depicted in , at concentrations ranging from 4.88 to 625 µg/ml, extract formula T4 elicited the higher percentages of cellular glucose consumption than extract formula T2. The IC50 value of extract formula T4 (316.58 ± 0.53 μg/ml) was obviously lower than that of extract formula T2 (>625 μg/ml), indicating that extract formula T4 was better in enhancing cellular glucose consumption ().
Figure 1. Effects of extract formulas T2 and T4 on cellular glucose consumption (a) and inhibition of nitric oxide production (b).
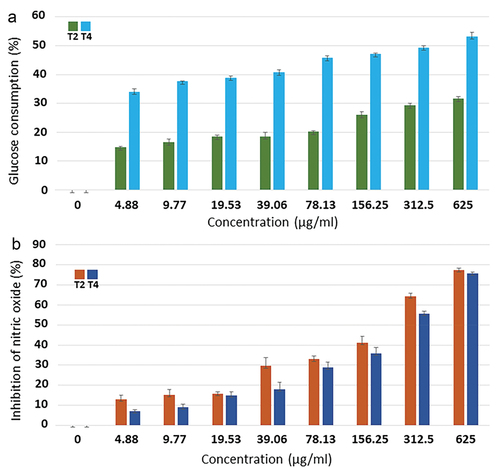
Table 3. IC50 values of extract formulas T2 and T4 for cellular glucose consumption and inhibition of nitric oxide production.
3.5. Antiinflammatory activity
As nitric oxide is one of pro-inflammatory mediators produced during inflammation, antiinflammatory activity was thus assessed from inhibition of nitric oxide production. As depicted in , the results showed that extract formulas T2 and T4 inhibited nitric oxide production in a dose-dependent manner. At concentrations of 312.5 and 625 µg/ml, both formulas inhibited more than 50% of nitric oxide production. At a highest concentration (625 µg/ml), extract formulas T2 and T4 elicited the inhibition percentages that were almost equal (77.48 ± 0.87% and 75.82 ± 0.48%, respectively). Extract formula T2 exhibited the IC50 value of 192.37 ± 0.55 µg/ml, which was lower than that of extract formula T4 (264.71 ± 0.51 µg/ml) (), indicating the more potential of extract formula T2.
3.6. Cytotoxicity to normal and cancer cells
The cytotoxicity of extract formulas T2 and T4 to normal RAW-264.7 mouse macrophage and SW-480 human colon cancer cells was evaluated. The results showed that, at concentrations of 2,500 and 5,000 µg/ml, extract formula T2 gave dramatically higher cell viability of RAW-264.7 cells than extract formula T4. At concentrations ranging from 4.88 to 625 µg/ml, both formulas retained more than 80% of RAW-264.7 cell viability. At higher concentrations ranging from 1,250 to 5,000 µg/ml, both formulas inhibited more than 50% of RAW-264.7 cell viability (). At every concentration, both formulas gave the insignificant percentages of SW-480 cell viability, with more than 70% (). The IC50 values of extract formulas T2 and T4 against RAW-264.7 cells were 1,119.61 ± 1.31 and 1,130.86 ± 1.16 µg/ml, respectively, whereas the IC50 values of both formulas against SW-480 cells were >5,000 µg/ml ().
Figure 2. Effects of extract formulas T2 and T4 on cell viability of RAW-264.7 (a) and SW-480 (b) cells.
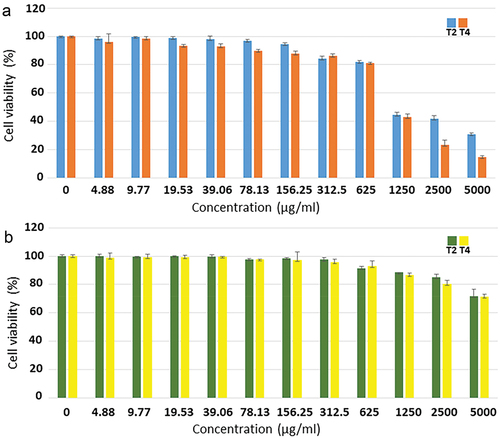
Table 4. IC50 values of extract formulas T2 and T4 against cell viability of RAW-264.7 and SW-480 cells.
3.7. Chemical profiles
The results showed that extract formulas T2 and T4 were composed of 367 and 464 compounds, respectively. The bioactive compounds having health-supporting properties are listed in . The bioactive compounds that were only detected in extract formula T2 included vidarabine, piperine, cordycep peptide A, tephrowatsin C, and etiocholanolone sulfate. The bioactive compounds that were unique to extract formula T4 included betaine, succinic acid, terazosin, sinensin, ampelopsin, and quercetin.
Table 5. Bioactive compounds having health-supporting functions that were detected in extract formulas T2 and T4 and tentatively identified by UHPLC-QTOF-MS/MS analysis.
4. Discussion
In this study, 80% (v/v) ethanol was used as an extractant. The use of ethanol-water solvent system has been recommended for extraction of phytochemicals from plant extracts due to its extraction efficiency and provision of consumer safety (Seneviratne et al., Citation2022). The extraction was carried out at 4°C because it was reported to be appropriate for maintenance of phytochemicals (Kapcum & Uriyapongson, Citation2018). Antioxidants play an important role in inhibiting and scavenging free radicals, providing protection against degenerative diseases and infections (Sreelatha & Padma, Citation2009). Our results demonstrated that extracts from powders of germinated brown jasmine rice, M. oleifera dry leaves, and C. militaris dry fruiting bodies, which were combined in six various ratios (test formulas T1-T6), exerted the significantly higher antioxidant activity than the three single-ingredient extracts (control formulas C1-C3). The results attested the synergistic effects of three ingredients on antioxidant activity. Extract formula T4 conferred the significantly highest antioxidant activity, followed by extract formula T2.
Tyagi et al. (Citation2022) compared antioxidant activity of the extracts from three Korean rice varieties. The black rice extract in 70% ethanol was mostly efficient in scavenging DPPH, followed by the brown rice extract and the white rice extract, respectively. Kaur et al. (Citation2017) reported that germination was effective to increase antioxidant potential. When comparing antioxidant activity of the extracts from ten rice cultivars, the extracts of germinated brown rice displayed dramatically higher scavenging activity (an average of 69.8%) than the extracts of ungerminated brown rice (an average of 35%). However, inconsistent results were observed by Summpunn et al. (Citation2022). When comparing antioxidant activity of the extracts from five Thai rice varieties, the white rice (milled rice grain) extracts had the significantly lowest antioxidant activity against DPPH. As observed in three rice varieties, the extracts from germinated brown rice exerted the significantly higher antioxidant activity than the extracts from ungerminated brown rice. In contrast, the antioxidant activity of the extracts from germinated and ungerminated brown rice in two varieties was not significant. Among M. oliefera plant parts, the highest antioxidant activity against DPPH was derived from the leaf extract, followed by the root extract and the seed extract, respectively (Xu, Y. B. et al., Citation2019). The DPPH inhibition percentage of the aqueous extract of C. militaris was significantly higher than that of the fresh C. militaris (Awang et al., Citation2021).
We also focused on a potent combination of the extracts from powders of germinated brown jasmine rice, M. oleifera dry leaves, and C. militaris dry fruiting bodies as antidiabetic agents. We therefore determined the ability of nine extract formulas to inhibit α-amylase and α-glucosidase activities as well as to enhance cellular glucose consumption. Our results showed that extract formulas T2 and T4 demonstrated the greatest activity against α-amylase. Extract formula T2 performed the best in inhibiting α-glucosidase, followed by extract formula T4. All test formulas (T1-T6), which were composed of all three ingredients, displayed the significantly higher efficiency in inhibitions of both enzymes than all three control formulas (C1-C3), which were from individual ingredients, attesting the synergistic effects of three ingredients.
Aalim and Luo (Citation2021) evaluated α-amylase and α-glucosidase inhibitory activities of the extracts from unpolished rice (brown rice and red rice) which was treated by different cooking methods. The extracts from fried red rice and fried brown rice had the highest inhibitory activities against α-amylase and α-glucosidase, respectively. Magaji et al. (Citation2020) evaluated extraction solvents and M. oleifera plant parts as parameters for inhibiting both enzymes. The methanol and hexane extracts from M. oleifera leaves presented the highest α-amylase inhibitory activity while the hexane extract from M. oleifera roots possessed the maximum effect in inhibiting α-glucosidase.
Extract formulas T2 and T4, which were the best ones for antioxidant as well as α-amylase and α-glucosidase inhibitory properties, were further examined for their ability to enhance cellular glucose consumption. Extract formula T4 was better at enhancing cellular glucose consumption by 3T3-L1 preadipocyte cells.
The ability to enhance cellular glucose consumption has been observed with the extracts from rice, M. oleifera leaves, and C. militaris. The ethanol extracts from both fermented rice germs and M. oleifera leaves reduced blood glucose levels in mice (Hyun et al., Citation2021; Tang et al., Citation2017). Exposure to the C. militaris extract significantly enhanced glucose uptake by hepatocellular carcinoma (HepG2) cells up to 168% over the untreated control (Kim et al., Citation2017).
Inflammation is a protective response of the body to various physical, chemical, or biological stimuli. The deregulated and consistent inflammatory responses may contribute to some diseases such as hepatitis, gastritis, neurodegenerative diseases, and chronic inflammation (Coussens & Werb, Citation2002; Kanwar et al., Citation2009). Nitric oxide is one of pro-inflammatory mediators produced during inflammation (Rujanapun et al., Citation2022). Our results showed that the inflammatory response of LPS-induced normal RAW264.7 mouse macrophage cells triggered nitric oxide production and extract formulas T2 and T4 caused the reduction in nitric oxide production. At concentrations of 312.5 and 625 µg/ml, both extract formulas inhibited more than 50% of nitric oxide production.
The extracts from rice, M. oleifera leaves, and C. militaris have been found with antiinflammatory potential. The polar fractions of red rice extract inhibited nitric oxide production by RAW264.7 cells (Limtrakul et al., Citation2016). The ethanol extract from M. oleifera leaves had better antiinflammatory potential in RAW264.7 cells than the seed extract (Xu, Y. B. et al., Citation2019). Both ethyl acetate and ergosterol-containing fractions of C. militaris extract exerted their antiinflammatory potential with inhibition of nitric oxide production by LPS-induced BV2 microglia cells (Nallathamby et al., Citation2015).
The cytotoxicity of extract formulas T2 and T4 was investigated using normal RAW-264.7 mouse macrophage and SW-480 human colon cancer cells. At concentrations of 2,500 and 5,000 µg/ml, extract formula T2 provided the significantly higher RAW-264.7 cell viability than extract formula T4. At concentrations ranging between 4.88 and 625 µg/ml, both formulas maintained RAW-264.7 cell viability above 80%. At a highest concentration (5,000 µg/ml), extract formulas T2 and T4 reduced RAW-264.7 cell viability to 30.73 ± 1.10% and 14.63 ± 1.14%, respectively. The IC50 values of extract formulas T2 and T4 against RAW-264.7 cell viability were 1,119.61 ± 1.31 and 1,130.86 ± 1.16 µg/ml, respectively. On the other hand, at every concentration (up to 5,000 µg/ml), both formulas maintained SW-480 cell viability above 70%. The IC50 values of both formulas against SW-480 cell viability were >5,000 µg/ml. According to Meyer’s toxicity index, extracts from natural substances are considered toxic if their LC50 values <1,000 µg/ml (Anliza & Rachmawati, Citation2021; Meyer et al., Citation1982). Based on their IC50 values, both extract formulas were considered nontoxic to both normal RAW-264.7 mouse macrophage and SW-480 human colon cancer cells, in which SW-480 was more resistant.
There are reports on cytotoxicity of the extracts from rice, M. oleifara leaves, and C. militaris to normal and cancer cells. The hydrolyzed riceberry rice bran extract mostly inhibited the proliferation of metastatic cancer cells (SW-620), followed by non-metastatic cancer cells (HT-29), and fibroblast normal human cells (PCS-201-010), respectively (Wattayagorn et al., Citation2022). Mumtaz et al. (Citation2021) compared cytotoxicity of the fractions of M. oleifera leaf extract on a human cervical cancer cell line (HeLa). M. oleifera dry leaf powder was extracted with 80% methanol, and then fractionated by solvents with different polarities. At a highest concentration (416 µg/ml), the highest inhibition percentages were obtained from the hexane, chloroform, and butanol fractional extracts. When comparing three leukemia cell lines, KG-1a cell line was more durable to the fractions of C. militaris extract than EoL-1 and U937 cell lines. The greatest cytotoxic effect was obtained from the hexane fractional extract against EoL-1 cell line (Tima et al., Citation2022).
The chemical profiles revealed that extract formulas T2 and T4 contained bioactive compounds with health-supporting activities. Ampelopsin has performed multiple pharmacological roles including antioxidant, antiinflammatory, antihypertensive, antibacterial, antihyperglycemic, anticancer, and hepatoprotective functions (Chen et al., Citation2020; Kou & Chen, Citation2012; Zhou et al., Citation2016). Azelaic acid demonstrated a potent antioxidant effect by decreasing reactive oxygen species (ROS) such as O2, OH−, and H2O2 (Jones, Citation2009). Azelaic acid is also a well-known antiinflammatory and antimicrobial agent used to treat rosacea, a skin disease caused by an inflammatory disorder (Jones, Citation2009; Spaggiari et al., Citation2023). Its potential to reduce blood glucose and improve cholesterol level was reported (Streeper et al., Citation2020). Quercetin and piperine have exerted the same roles as antioxidant, antiinflammatory, and antihypertensive agents (Anand David et al., Citation2016; Tripathi et al., Citation2022; Xu, D. et al., Citation2019). Ethosuximide and pyroglutamic acid have exhibited the same properties as antioxidant, antiinflammatory, and antimicrobial agents (Bilska et al., Citation2018; Gang et al., Citation2018; Gören & Onat, Citation2007; Hakobyan et al., Citation2020; Zaccara & Perucca, Citation2014).
The similar health-beneficial properties have been reported for the extracts of other plant species. The essential oils extracted from leafy stems of Sudanese Ocimum basilicum and Pulicaria undulata exhibited the potent abilities in scavenging free radicals, inhibiting α-amylase, and preventing proliferation of three cancer cell lines including human adenocarcinoma (HT29 and HCT116) and human breast carcinoma (MCF7) cells. The major bioactive compounds present in O. basilicum oil and P. undulata oil were phenylpropene (51.9%) and oxygenated monoterpenes (96.9%), respectively (Mohammed et al., Citation2020). Two types of Italian Capsicum annuum L. (pepper), hot and sweet peppers, were extracted by four techniques including soxhlet extraction, microwave extraction, 60% methanol decoction, and hexane decoction. The extracts of both peppers, which were extracted by soxhlet extraction and 60% methanol decoction, provided the equal highest DPPH scavenging activity. All extracts displayed α-amylase inhibitory activity while α-glucosidase inhibitory activity was obtained from five extracts including the microwave and hexane extracts from hot pepper as well as the soxhlet, microwave, and hexane extracts from sweet pepper. All extracts significantly reduced inflammatory edema as compared to the control (Della Valle et al., Citation2020).
Interestingly, in this report, we demonstrated the synergistic benefits of using the combinations of extracts from powders of germinated brown jasmine rice, M. oleifera dry leaves, and C. militaris dry fruiting bodies for various health-supporting purposes. The extracts from combined ingredients elicited the significantly higher antioxidant as well as α-amylase and α-glucosidase inhibitory activities than the extracts from single ingredients. Due to their extensive nutritional and phytochemical compositions, these combined extracts merit the further development as functional food supplements and alternative natural therapeutic agents.
5. Conclusions
The present study reveals the synergistic advantage of using the combined extracts from powders of germinated brown jasmine rice, M. oleifera dry leaves, and C. militaris dry fruiting bodies as antioxidant agents as well as α-amylase and α-glucosidase inhibitors. The selected combinations (extract formulas T2 with a ratio of 80:10:10 and T4 with a ratio of 70:20:10) also increased cellular glucose consumption by 3T3-L1 preadipocyte cells and exerted antiinflammatory activity in normal RAW-264.7 mouse macrophage cells. Furthermore, both formulas were not toxic to normal and cancer cells in vitro. In conclusion, their perceivable activities would prompt the application of these combined extracts as food supplements for a therapeutic prospect.
Disclosure statement
No potential conflict of interest was reported by the author(s).
Additional information
Funding
References
- Aalim, H., & Luo, Z. (2021). Insight into rice (Oryza sativa L.) cooking: Phenolic composition, inhibition of α-amylase and α-glucosidase, and starch physicochemical and functional properties. Food Bioscience, 40, 100917. https://doi.org/10.1016/j.fbio.2021.100917
- Agustin, A. T., Safitri, A., & Fatchiyah, F. (2021). Java red rice (Oryza sativa L.) nutritional value and anthocyanin profiles and its potential role as antioxidant and anti-diabetic. Indonesian Journal of Chemistry, 21(4), 968–978. https://doi.org/10.22146/ijc.64509
- Anand David, A. V., Arulmoli, R., & Parasuraman, S. (2016). Overviews of biological importance of quercetin: A bioactive flavonoid. Pharmacognosy Reviews, 10(20), 84–89. https://doi.org/10.4103/0973-7847.194044
- Anliza, S., & Rachmawati, N. (2021). Cytotoxic activity of ethanol extract in Namnam leaves (Cynometra cauliflora l.) to Hela cell. Walisongo Journal of Chemistry, 4(2), 107–112. https://doi.org/10.21580/wjc.v4i2.7999
- Awang, M. A., Daud, N. N. N. N. M., Ismail, N. I. M., Cheng, P. G., Ismail, M. F., & Ramaiya, S. D. (2021). Antioxidant and cytotoxicity activity of Cordyceps militaris extracts against human colorectal cancer cell line. Journal of Applied Pharmaceutical Science, 11(7), 105–109. https://doi.org/10.7324/JAPS.2021.110711
- Bilska, K., Stuper-Szablewska, K., Kulik, T., Buśko, M., Załuski, D., & Perkowski, J. (2018). Resistance-related L-pyroglutamic acid affects the biosynthesis of trichothecenes and phenylpropanoids by F. graminearum sensu stricto. Toxins (Basel), 10(12), 492. https://doi.org/10.3390/toxins10120492
- Chen, L., Lin, X., Yao, M., & Teng, H. (2020). Self-nanoemulsions loaded with dihydromyricetin: Insights to their formulation stability. Food Hydrocolloids, 108, 105888. https://doi.org/10.1016/j.foodhyd.2020.105888
- Chmiel, T., Saputro, I. E., Kusznierewicz, B., & Bartoszek, A. (2018). The impact of cooking method on the phenolic composition, total antioxidant activity and starch digestibility of rice (Oryza sativa L.). Journal of Food Processing and Preservation, 42(1), e13383. https://doi.org/10.1111/jfpp.13383
- Cho, D. H., & Lim, S. T. (2016). Germinated brown rice and its bio-functional compounds. Food Chemistry, 196, 259–271. https://doi.org/10.1016/j.foodchem.2015.09.025
- Coussens, L. M., & Werb, Z. (2002). Inflammation and cancer. Nature, 420(6917), 860–867. https://doi.org/10.1038/nature01322
- Della Valle, A., Dimmito, M. P., Zengin, G., Pieretti, S., Mollica, A., Locatelli, M., Cichelli, A., Novellino, E., Ak, G., Yerlikaya, S., Baloglu, M. C., Celik Altunoglu, Y., & Stefanucci, A. (2020). Exploring the nutraceutical potential of dried pepper Capsicum annuum L. on market from Altino in Abruzzo region. Antioxidants (Basel), 9(5), 400. https://doi.org/10.3390/antiox9050400
- Fares, M., Abedi-Valugerdi, M., Hassan, M., & Potácová, Z. (2015). DNA damage, lysosomal degradation and Bcl-xL deamidation in doxycycline- and minocycline-induced cell death in the K562 leukemic cell line. Biochemical and Biophysical Research Communications, 463(3), 268–274. https://doi.org/10.1016/j.bbrc.2015.05.043
- Gang, F. L., Zhu, F., Li, X. T., Wei, J. L., Wu, W. J., & Zhang, J. W. (2018). Synthesis and bioactivities evaluation of L-pyroglutamic acid analogues from natural product lead. Bioorganic and Medicinal Chemistry, 26(16), 4644–4649. https://doi.org/10.1016/j.bmc.2018.07.041
- Gopalakrishnan, L., Doriya, K., & Kumar, D. S. (2016). Moringa oleifera: A review on nutritive importance and its medicinal application. Food Science and Human Wellness, 5(2), 49–56. https://doi.org/10.1016/j.fshw.2016.04.001
- Gören, M. Z., & Onat, F. (2007). Ethosuximide: From bench to bedside. CNS Drug Reviews, 13(2), 224–229. https://doi.org/10.1111/j.1527-3458.2007.00009.x
- Hakobyan, N. Z., Hovasyan, Z. A., Hovakimyan, S. S., Melkonyan, A. G., Pagutyan, N. A., Panosyan, G. A., & Gevorgyan, G. A. (2020). Synthesis and antioxidant activity of N-aminomethyl derivatives of ethosuximide and pufemide anticonvulsants. Russian Journal of General Chemistry, 90(3), 385–389. https://doi.org/10.1134/S1070363220030093
- Hansakul, P., Srisawat, U., Itharat, A., & Lerdvuthisopon, N. (2011). Phenolic and flavonoid contents of Thai rice extracts and their correlation with antioxidant activities using chemical and cell assays. Journal of the Medical Association of Thailand, 94(Suppl 7), S122–S130.
- Hu, C., Zawistowski, J., Ling, W., & Kitts, D. D. (2003). Black rice (Oryza sativa L. indica) pigmented fraction suppresses both reactive oxygen species and nitric oxide in chemical and biological model systems. Journal of Agricultural and Food Chemistry, 51(18), 5271–5277. https://doi.org/10.1021/jf034466n
- Hyun, Y. J., Kim, J. G., Jung, S. K., & Kim, J. Y. (2021). Fermented rice germ extract ameliorates abnormal glucose metabolism via antioxidant activity in type 2 diabetes mellitus mice. Applied Sciences, 11(7), 3091. https://doi.org/10.3390/app11073091
- Jones, D. A. (2009). Rosacea, reactive oxygen species, and azelaic acid. The Journal of Clinical and Aesthetic Dermatology, 2(1), 26–30.
- Kang, D. H., Kang, O. H., Li, Z., Mun, S. H., Seo, Y. S., Kong, R., Tian, Z., Liu, X., & Kwon, D. Y. (2016). Antiinflammatory effects of Ciwujianoside C3, extracted from the leaves of Acanthopanax henryi (Oliv.) Harms, on LPS-stimulated RAW 264.7 cells. Molecular Medicine Reports, 14(4), 3749–3758. https://doi.org/10.3892/mmr.2016.5710
- Kanwar, J. R., Kanwar, R. K., Burrow, H., & Baratchi, S. (2009). Recent advances on the roles of NO in cancer and chronic inflammatory disorders. Current Medicinal Chemistry, 16(19), 2373–2394. https://doi.org/10.2174/092986709788682155
- Kapcum, C., & Uriyapongson, J. (2018). Effects of storage conditions on phytochemical and stability of purple corn cob extract powder. Food Science & Technology, 38(Suppl 1), 301–305. https://doi.org/10.1590/1678-457X.23217
- Karray, A., Alonazi, M., Jallouli, R., Alanazi, H., & Ben Bacha, A. (2022). A proteinaceous alpha-amylase inhibitor from Moringa oleifera leaf extract: Purification, characterization, and insecticide effects against C. maculates insect larvae. Molecules, 27(13), 4222. https://doi.org/10.3390/molecules27134222
- Kaur, M., Asthir, B., & Mahajan, G. (2017). Variation in antioxidants, bioactive compounds and antioxidant capacity in germinated and ungerminated grains of ten rice cultivars. Rice Science, 24(6), 349–359. https://doi.org/10.1016/j.rsci.2017.08.002
- Kim, D. J., Kang, Y. H., Kim, K. K., Kim, T. W., Park, J. B., & Choe, M. (2017). Increased glucose metabolism and alpha-glucosidase inhibition in Cordyceps militaris water extract-treated HepG2 cells. Nutrition Research and Practice, 11(3), 180–189. https://doi.org/10.4162/nrp.2017.11.3.180
- Kou, X., & Chen, N. (2012). Pharmacological potential of ampelopsin in rattan tea. Food Science and Human Wellness, 1(1), 14–18. https://doi.org/10.1016/j.fshw.2012.08.001
- Kusano, R., Ogawa, S., Matsuo, Y., Tanaka, T., Yazaki, Y., & Kouno, I. (2011). α-amylase and lipase inhibitory activity and structural characterization of acacia bark proanthocyanidins. Journal of Natural Products, 74(2), 119–128. https://doi.org/10.1021/np100372t
- Lee, H. H., Lee, S., Lee, K., Shin, Y. S., Kang, H., & Cho, H. (2015). Anti-cancer effect of Cordyceps militaris in human colorectal carcinoma RKO cells via cell cycle arrest and mitochondrial apoptosis. Daru: Journal of Faculty of Pharmacy, Tehran University of Medical Sciences, 23(1), 35. https://doi.org/10.1186/s40199-015-0117-6
- Limtrakul, P., Yodkeeree, S., Pitchakarn, P., & Punfa, W. (2016). Anti-inflammatory effects of proanthocyanidin-rich red rice extract via suppression of MAPK, AP-1 and NF-κB pathways in raw 264.7 macrophages. Nutrition Research and Practice, 10(3), 251–258. https://doi.org/10.4162/nrp.2016.10.3.251
- Magaji, U. F., Sacan, O., & Yanardag, R. (2020). Alpha amylase, alpha glucosidase and glycation inhibitory activity of Moringa oleifera extracts. South African Journal of Botany, 128, 225–230. https://doi.org/10.1016/j.sajb.2019.11.024
- Meyer, B. N., Ferrigni, N. R., Putnam, J. E., Jacobsen, L. B., Nichols, D. E., & McLaughlin, J. L. (1982). Brine shrimp: A convenient general bioassay for active plant constituents. Planta Medica, 45(5), 31–34. https://doi.org/10.1055/s-2007-971236
- Mohammed, A. B. A., Yagi, S., Tzanova, T., Schohn, H., Abdelgadir, H., Stefanucci, A., Mollica, A., Mahomoodally, M. F., Adlan, T. A., & Zengin, G. (2020). Chemical profile, antiproliferative, antioxidant and enzyme inhibition activities of Ocimum basilicum L. and Pulicaria undulata (L.) C.A. Mey. grown in Sudan. South African Journal of Botany, 132, 403–409. https://doi.org/10.1016/j.sajb.2020.06.006
- Morimitsu, Y., Hayashi, K., Nakagawa, Y., Horio, F., Uchida, K., & Osawa, T. (2000). Antiplatelet and anticancer isothiocyanates in Japanese domestic horseradish, wasabi. BioFactors, 13(1–4), 271–276. https://doi.org/10.1002/biof.5520130141
- Mumtaz, M. Z., Kausar, F., Hassan, M., Javaid, S., & Malik, A. (2021). Anticancer activities of phenolic compounds from Moringa oleifera leaves: In vitro and in silico mechanistic study. Beni-Suef University Journal of Basic and Applied Sciences, 10(1), 12. https://doi.org/10.1186/s43088-021-00101-2
- Mundkar, M., Bijalwan, A., Soni, D., & Kumar, P. (2022). Neuroprotective potential of Moringa oleifera mediated by NF-kB/nrf2/HO-1 signaling pathway: A review. Journal of Food Biochemistry, 46(12), e14451. https://doi.org/10.1111/jfbc.14451
- Nallathamby, N., Guan-Serm, L., Vidyadaran, S., Abd Malek, S. N., Raman, J., & Sabaratnam, V. (2015). Ergosterol of Cordyceps militaris attenuates LPS induced inflammation in BV2 microglia cells. Natural Product Communications, 10(6), 885–886. https://doi.org/10.1177/1934578X1501000623
- Nam, T. G., Lim, T. G., Lee, B. H., Lim, S., Kang, H., Eom, S. H., Yoo, M., Jang, H. W., & Kim, D. O. (2017). Comparison of anti-inflammatory effects of flavonoid-rich common and tartary buckwheat sprout extracts in lipopolysaccharide-stimulated RAW 264.7 and peritoneal macrophages. Oxidative Medicine and Cellular Longevity, 2017, 9658030. https://doi.org/10.1155/2017/9658030
- Ogundipe, A., Adetuyi, B., Iheagwam, F., Adefoyeke, K., Olugbuyiro, J., Ogunlana, O., & Ogunlana, O. (2022). In vitro experimental assessment of ethanolic extract of Moringa oleifera leaves as an α-amylase and α-lipase inhibitor. Biochemistry Research International, 2022, 4613109. https://doi.org/10.1155/2022/4613109
- Piazza, S., Colombo, F., Bani, C., Fumagalli, M., Vincentini, O., Sangiovanni, E., Martinelli, G., Biella, S., Silano, M., Restani, P., Dell’agli, M., & DiLorenzo, C. (2022). Evaluation of the potential anti-inflammatory activity of black rice in the framework of celiac disease. Foods, 12(1), 63. https://doi.org/10.3390/foods12010063
- Quan, N. V., Iuchi, Y., Anh, L. H., Hasan, M., & Xuan, T. D. (2022). Simple isolation of cordycepin from Cordyceps militaris by dual-normal phase column chromatography and its potential for making kombucha functional products. Separations, 9(10), 290. https://doi.org/10.3390/separations9100290
- Quan, N. V., Xuan, T. D., Tran, H. D., Ahmad, A., Khanh, T. D., & Dat, T. D. (2019). Contribution of momilactones A and B to diabetes inhibitory potential of rice bran: Evidence from in vitro assays. Saudi Pharmaceutical Journal, 27(5), 643–649. https://doi.org/10.1016/j.jsps.2019.03.006
- Qu, S. L., Li, S. S., Li, D., & Zhao, P. J. (2022). Metabolites and their bioactivities from the genus Cordyceps. Microorganisms, 10(8), 1489. https://doi.org/10.3390/microorganisms10081489
- Ramli, N. S., & Zin, N. H. M. (2018). Alpha-amylase inhibitory activity of inhibitor proteins in different types of commercial rice. Science Heritage Journal (GWS), 2(2), 27–29. https://doi.org/10.26480/gws.02.2018.27.29
- Ren, C., Hong, B., Zheng, X., Wang, L., Zhang, Y., Guan, L., Yao, X., Huang, W., Zhou, Y., & Lu, S. (2020). Improvement of germinated brown rice quality with autoclaving treatment. Food Science and Nutrition, 8(3), 1709–1717. https://doi.org/10.1002/fsn3.1459
- Rujanapun, N., Jaidee, W., Duangyod, T., Phuneerub, P., Paojumroom, N., Maneerat, T., Pringpuangkeo, C., Ramli, S., & Charoensup, R. (2022). Special Thai oolong tea: Chemical profile and in vitro antidiabetic activities. Frontiers in Pharmacology, 13, 797032. https://doi.org/10.3389/fphar.2022.797032
- Santos, A. F., Argolo, A. C., Paiva, P. M., & Coelho, L. C. (2012). Antioxidant activity of Moringa oleifera tissue extracts. Phytotherapy Research, 26(9), 1366–1370. https://doi.org/10.1002/ptr.4591
- Sarnthima, R., Khammuang, S., & Joompang, A. (2020). Glutinous rice (Oryza sativa L.) protein extract with potent α-amylase inhibitory activity. Journal of Food Science and Technology, 57(8), 3157–3163. https://doi.org/10.1007/s13197-020-04560-w
- Segwatibe, M. K., Cosa, S., & Bassey, K. (2023). Antioxidant and antimicrobial evaluations of Moringa oleifera Lam leaves extract and isolated compounds. Molecules, 28(2), 899. https://doi.org/10.3390/molecules28020899
- Seneviratne, K. P., Anjali, N. V. P., Senanayake, C. M., Jayathilaka, N., & Seneviratne, K. N. (2022). Ethanolic extract of rice bran: A thermally stable preservative for edible oils and cake. Food Production, Processing and Nutrition, 4(1), 14. https://doi.org/10.1186/s43014-022-00094-0
- Shimamura, T., Sumikura, Y., Yamazaki, T., Tada, A., Kashiwagi, T., Ishikawa, H., Matsui, T., Sugimoto, N., Akiyama, H., & Ukeda, H. (2014). Applicability of the DPPH assay for evaluating the antioxidant capacity of food additives-inter-laboratory evaluation study. Analytical Sciences, 30(7), 717–721. https://doi.org/10.2116/analsci.30.717
- Siddhuraju, P., & Becker, K. (2003). Antioxidant properties of various solvent extracts of total phenolic constituents from three different agroclimatic origins of drumstick tree (Moringa oleifera Lam.) leaves. Journal of Agricultural and Food Chemistry, 51(8), 2144–2155. https://doi.org/10.1021/jf020444+
- Sissoko, L., Diarra, N., Nientao, I., Stuart, B., Togola, A., Diallo, D., & Willcox, M. (2020). Moringa oleifera leaf powder for type 2 diabetes: A pilot clinical trial. African Journal of Traditional, Complementary and Alternative Medicines, 17(2), 29–36. https://doi.org/10.21010/ajtcam.v17i2.3
- Spaggiari, C., Annunziato, G., Spadini, C., Montanaro, S. L., Iannarelli, M., Cabassi, C. S., & Costantino, G. (2023). Extraction and quantification of azelaic acid from different wheat samples (Triticum durum Desf.) and evaluation of their antimicrobial and antioxidant activities. Molecules, 28(5), 2134. https://doi.org/10.3390/molecules28052134
- Sreelatha, S., & Padma, P. R. (2009). Antioxidant activity and total phenolic content of Moringa oleifera leaves in two stages of maturity. Plant Foods for Human Nutrition, 64(4), 303–311. https://doi.org/10.1007/s11130-009-0141-0
- Streeper, R. T., Louden, C., & Izbicka, E. (2020). Oral azelaic acid ester decreases markers of insulin resistance in overweight human male subjects. In Vivo, 34(3), 1173–1186. https://doi.org/10.21873/invivo.11890
- Striegel, L., Kang, B., Pilkenton, S. J., Rychlik, M., & Apostolidis, E. (2015). Effect of black tea and black tea pomace polyphenols on α-glucosidase and α-amylase inhibition, relevant to type 2 diabetes prevention. Frontiers in Nutrition, 2, 3. https://doi.org/10.3389/fnut.2015.00003
- Summpunn, P., Panpipat, W., Manurakchinakorn, S., Bhoopong, P., Ling-Zhi Cheong, L. Z., & Chaijan, M. (2022). Comparative analysis of antioxidant compounds and antioxidative properties of Thai indigenous rice: Effects of rice variety and processing condition. Molecules, 27(16), 5180. https://doi.org/10.3390/molecules27165180
- Suryanti, V., Riyatun., Suharyana, S., Sutarno, & Saputra, O. A. (2020). Antioxidant activity and compound constituents of gamma-irradiated black rice (Oryza sativa L.) var. Cempo ireng indigenous of Indonesia. Biodiversitas Journal of Biological Diversity, 21(9), 4205–4212. https://doi.org/10.13057/biodiv/d210935
- Tang, Y., Choi, E. J., Han, W. C., Oh, M., Kim, J., Hwang, J. Y., Park, P. J., Moon, S. H., Kim, Y. S., & Kim, E. K. (2017). Moringa oleifera from Cambodia ameliorates oxidative stress, hyperglycemia, and kidney dysfunction in type 2 diabetic mice. Journal of Medicinal Food, 20(5), 502–510. https://doi.org/10.1089/jmf.2016.3792
- Thurber, M. D., & Fahey, J. W. (2009). Adoption of Moringa oleifera to combat under-nutrition viewed through the lens of the “diffusion of innovations” theory. Ecology of Food and Nutrition, 48(3), 212–225. https://doi.org/10.1080/03670240902794598
- Tima, S., Tapingkae, T., To-Anun, C., Noireung, P., Intaparn, P., Chaiyana, W., Sirithunyalug, J., Panyajai, P., Viriyaadhammaa, N., Nirachonkul, W., Rueankham, L., Aung, W. L., Chueahongthong, F., Chiampanichayakul, S., & Anuchapreeda, S. (2022). Antileukaemic cell proliferation and cytotoxic activity of edible golden Cordyceps (Cordyceps militaris) extracts. Evidence-Based Complementary and Alternative Medicine, 2022, 5347718. https://doi.org/10.1155/2022/5347718
- Tripathi, A. K., Ray, A. K., & Mishra, S. K. (2022). Molecular and pharmacological aspects of piperine as a potential molecule for disease prevention and management: Evidence from clinical trials. Beni-Suef University Journal of Basic and Applied Sciences, 11(1), 16. https://doi.org/10.1186/s43088-022-00196-1
- Tyagi, A., Shabbir, U., Chen, X., Chelliah, R., Elahi, F., Ham, H. J., & Oh, D. H. (2022). Phytochemical profiling and cellular antioxidant efficacy of different rice varieties in colorectal adenocarcinoma cells exposed to oxidative stress. In U. Sarker (Ed.), PLoS One, 17, e0269403. https://doi.org/10.1371/journal.pone.0269403
- Upadhyay, A., & Karn, S. K. (2018). Brown rice: Nutritional composition and health benefits. Journal of Food Science and Technology Nepal, 10, 47–52. https://doi.org/10.3126/jfstn.v10i0.19711
- Walter, M., & Marchesan, E. (2011). Phenolic compounds and antioxidant activity of rice. Brazilian Archives of Biology and Technology, 54(2), 371–377. https://doi.org/10.1590/S1516-89132011000200020
- Wan, C. P., Wei, Y. G., Li, X. X., Zhang, L. J., Yang, R., & Bao, Z. R. (2017). Piperine regulates glucose metabolism disorder in HepG2 cells of insulin resistance models via targeting upstream target of AMPK signaling pathway. Zhongguo Zhong Yao Za Zhi, 42(3), 542–547. https://doi.org/10.19540/j.cnki.cjcmm.20161222.059
- Wattayagorn, V., Kongsema, M., Tadakittisarn, S., & Chumnanpuen, P. (2022). Riceberry rice bran protein hydrolyzed fractions induced apoptosis, senescence and G1/S cell cycle arrest in human colon cancer cell lines. Applied Sciences, 12(14), 6917. https://doi.org/10.3390/app12146917
- Wisetkomolmat, J., Arjin, C., Hongsibsong, S., Ruksiriwanich, W., Niwat, C., Tiyayon, P., Jamjod, S., Yamuangmorn, S., Prom-U-Thai, C., & Sringarm, K. (2023). Antioxidant activities and characterization of polyphenols from selected Northern Thai rice husks: Relation with seed attributes. Rice Science, 30(2), 148–159. https://doi.org/10.1016/j.rsci.2023.01.007
- Xiao, X., Wang, J., Meng, C., Liang, W., Wang, T., Zhou, B., Wang, Y., Luo, X., Gao, L., & Zhang, L. (2020). Moringa oleifera Lam and its therapeutic effects in immune disorders. Frontiers in Pharmacology, 11, 566783. https://doi.org/10.3389/fphar.2020.566783
- Xu, Y. B., Chen, G. L., & Guo, M. Q. (2019). Antioxidant and anti-inflammatory activities of the crude extracts of Moringa oleifera from Kenya and their correlations with flavonoids. Antioxidants (Basel), 8(8), 296. https://doi.org/10.3390/antiox8080296
- Xu, D., Hu, M. J., Wang, Y. Q., & Cui, Y. L. (2019). Antioxidant activities of quercetin and its complexes for medicinal application. Molecules, 24(6), 1123. https://doi.org/10.3390/molecules24061123
- Zaccara, G., & Perucca, E. (2014). Interaction between antiepileptic drugs, and between antiepileptic drugs and other drugs. Epileptic Disorders, 16(4), 409–431. https://doi.org/10.1684/epd.2014.0714
- Zhang, L., Hu, J. J., & Du, G. H. (2008). Establishment of a cell-based assay to screen insulin-like hypoglycemic drugs. Drug Discoveries and Therapeutics, 2(4), 229–233.
- Zhou, Y., Wu, Y., Qin, Y., Liu, L., Wan, J., Zou, L., Zhang, Q., Zhu, J., & Mi, M. 2016. Ampelopsin improves insulin resistance by activating PPARγ and subsequently up-regulating FGF21-AMPK signaling pathway. InV. Sánchez-Margalet (Ed.), PLoS One, 11(7), e0159191. https://doi.org/10.1371/journal.pone.0159191