ABSTRACT
The purpose of this study was to investigate the protective effect of xylitol on gut mucosal barrier injury in a type 2 diabetes rat model. Treatment with xylitol not only ameliorated diabetic-induced changes to small intestinal mucosa morphology and decreased diamine oxidase concentration in the serum of diabetic rats, but also modified the inflammatory infiltrates and improved the secretory IgA levels of the small intestinal epithelium. Increased expression of zo-1, occludin, mucin-2 and intestinal trefoil factor also was detected in small intestinal tissue. Intragastric administration of xylitol suppressed diabetes-induced increases of myeloperoxidase, eosinophil peroxidase, IL-6 and TNF-α. These results suggest that xylitol reduces mucosal inflammatory lesions and improves the intestinal barrier in diabetic rats by maintaining the structural integrity of the mucous layer, inhibiting the expression of inflammatory mediators and enhancing the expression of tight junction proteins associated with intestinal permeability.
Introduction
Diabetes mellitus is a chronic metabolic disease characterized by hyperglycemia that can seriously damage human health and lower the quality of life (Ji et al., Citation2015). Long-term hyperglycemia can lead to chronic damage and dysfunction of various tissues, especially eyes, kidneys, heart, blood vessels and nerves. The morbidity of diabetes increases every year. According to the World Health Organization (WHO), the number of people with diabetes rose from 108 million in 1980 to 422 million in 2014. Recently, a survey estimated that there will be more than 642 million people suffering from diabetes, representing nearly all countries, by the year 2040 (Cho, Citation2016). Diabetes mellitus and its complications have become a major public health problem in China. Its incidence in China has increased from 0.67% in the 1980s to 11% in 2013, showing an explosive growth trend (Shen et al., Citation2018). Previous studies have found that most diabetic patients suffer from gastrointestinal complications as diabetes is closely related to intestinal dysfunction and impaired intestinal barrier. Activation of the non-specific immune system is involved in the occurrence and development of insulin resistance and type 2 diabetes mellitus (T2D). Diabetes mellitus can cause chronic mild inflammatory responses mediated by cytokines, which react with the intestinal tract and cause intestinal barrier damage (de Kort et al., Citation2011). Therefore, it is imperative to study the relationship between diabetes mellitus and intestinal function.
Xylitol is an intermediate product of carbon metabolism in mammals and a normal metabolite in the body. It exists in fruits and vegetables, which when extracted from corn cobs, sugar cane (bagasse) and other plant raw materials can be used as a natural sweetener (Ur-Rehman et al., Citation2015). Xylitol has the same sweetness as sucrose, and energy created by complete oxidation decomposition is equivalent to that of glucose (Ghosh & Sudha, Citation2012). However, it can only be absorbed slowly or partially utilized, so it provides 35%-60% fewer calories than other carbohydrates under the same conditions (Van Loveren, Citation2004). Xylitol can not only inhibit oral cariogenic bacteria, reduce body fat accumulation (Ur-Rehman et al., Citation2015) and promote bone health (Sato et al., Citation2011), but also plays a role in alleviating abnormal elevation of blood sugar and alleviating inflammation (Park et al., Citation2014). It can easily cross cell membranes by facilitated diffusion to provide nutrients and energy for cells without causing elevated blood sugar, and regulates inflammatory reactions (Mäkinen, Citation2016).
Damage to the intestinal mucosal barrier is not only the result of diabetes mellitus, but also is an important factor to promote the development of disease (Arrieta et al., Citation2006). Although there are differences in pathological mechanism and clinical manifestations between type I and type II diabetes mellitus, they have a common lesion site – the intestinal mucosal barrier. Studies have confirmed that both forms of diabetes destroy the intestinal epithelial tight junction and increase intestinal permeability. Although it is not clear whether intestinal epithelial barrier dysfunction is a predisposing factor or a concomitant phenomenon of diabetes mellitus, there is no doubt that they are closely related (de Kort et al., Citation2011). Therefore, blocking the interaction between the intestinal epithelial barrier and diabetes mellitus may be of great benefit to alleviate the symptoms of diabetes mellitus and reduce complications. Previous studies have shown that xylitol has a significant hyperglycemic effect (Islam & Indrajit, Citation2012). However, there are few reports about whether xylitol can change glycolipid metabolism by affecting the intestinal mucosal barrier or whether there is a direct relationship between xylitol and intestinal health. This study explores the effect of oral xylitol on intestinal health and intestinal mucosal barrier integrity in diabetic rats.
Material and methods
Reagents and materials
Streptozotocin (STZ) was purchased from Sigma. A 1% STZ solution was prepared with 0.1 mol/L sodium citrate buffer solution (pH 4.5). Xylitol was purchased from Zhejiang Huakang Pharmaceutical Co., Ltd (Kaihua, China). ELISA kits were purchased from Multi Sciences (Lianke) Biotech, Co., Ltd (Hangzhou, China). Immunohistochemical antibodies were purchased from Boster Biological Technology Co. Ltd (Wuhan, China).
Establishment of animal models
Seven-week-old male Wistar rats with a mean body weight of 182.82 ± 1.52 g were procured from the Laboratory animal center located at the Xiasha campus of the Hangzhou Normal University (Hangzhou, China). The animals were housed in a four-in-one medium-sized poly-carbonated cage and maintained in a temperature- and humidity-controlled room with a 12:12 h light-dark cycle under pathogen-free conditions. All animals were fed with a commercial normal (negative control group, NC, n = 12) or high fat (45% fat, diabetes mellitus group, DM, n = 60) pellet diet during the induction of diabetes. After three weeks, the DM group rats were induced by intraperitoneal injection of STZ at 40 mg/kg body weight, whereas the animals in the NC group were injected with a similar volume of vehicle buffer only. Three days after STZ injection, the fasting blood glucose (FBG) levels of all animals was measured in the blood collected from the tail vein using an AlphaTRAK Blood Glucose Monitoring System (Zoetis, Parsippany, New Jersey, US). Animals with a FBG level >16.7 mmol/L in daily tests were considered diabetic. Animals with a FBG level ≤16.7 mmol/L were excluded from the study.
Experimental design
Diabetic rats were divided randomly into four groups (n = 10/group): diabetic rats in the X1, X2, or X3 groups were administered 1.25 g/kg·body weight (bw), 2.5 g/kg·bw, or 5 g/kg·bw xylitol by gavage, respectively (Amo et al., Citation2011). Diabetic rats in the diabetic control (CD) group were administered a similar volume of vehicle buffer. Control (C) group rats obtained from the NC group during the induction of diabetes experiment were processed in the same way as CD group rats. During the experimental period, rats in each group were fed a normal diet and ad lib water. After 5 weeks, all rats were sacrificed under deep anesthesia by cardiac incision following which heart blood, intestinal contents, and organs were collected. The jejunum and ileum were dissected and fixed with paraformaldehyde for histologic sectioning. Another intestinal segment was frozen with liquid nitrogen and stored at −70°C. Whole blood from each animal was collected via cardiac puncture and centrifuged at 3000 rpm for 20 min at 4°C to collect the serum, which was stored at −70°C for further analysis. The caecal contents were collected for DNA extraction of intestinal microflora. Animal studies were approved by the Experiment Animal Ethics Committee of the Zhejiang Gongshang University (Permit Number: ZJGU 20,210,007).
Food intake, body weight and blood glucose monitoring
Body weight changes and food intake of all animals were measured every other day and blood glucose levels were measured weekly during the entire experimental period. To measure the glucose tolerance of each animal, an oral glucose tolerance test (OGTT) was performed in the last week of the intervention period. To perform this test, a single dose of glucose solution (2 g/kg body weight) was orally ingested by each animal and the subsequent levels of blood glucose were measured at 0 (just before glucose ingestion), 30, 60, and 120 min after the dose was given.
Serum insulin level and lipid concentrations
We collected blood samples from the arteria cruralis of rats to measure the levels of insulin, triglyceride (TG), total cholesterol (TC) and high density lipoprotein cholesterol (HDL-C) in serum using an automatic biochemical analyzer.
Measurement of cytokine and inflammatory mediator concentrations
The secretion levels of diamine oxidase (DAO), myeloperoxidase (MPO), eosinophilic peroxidase (EPX), tumor necrosis factor alpha (TNF-α) and IL-6 in serum were measured using ELISA kits (MultiSciences Biotech Co., Ltd., Hangzhou, China). Secretory immunoglobulin A (sIgA) and EPX concentrations in colonic tissue also were determined by a commercial ELISA kit (Cloud-Clone Corp., Wuhan, China).
Histology and immunohistochemistry
For histological analysis, the proximal jejunum and distal ileum of rats in all groups were fixed in 10% buffered formalin overnight and kept in 70% ethanol until processed. Tissues were embedded in paraffin and cut into 5 μm thick sections. Hematoxylin-eosin (HE) staining and alcian blue-periodic acid Schiff (AB-PAS) staining are completed as required. All of them performed using an image analysis system.
For immunohistochemistry, dewaxed sections need antigen retrieval and blocked with 3% BSA at 37°C for 30 min. They were incubated for 2 hours at room temperature in blocking buffer containing CD68 polyclonal antibodies (1:100, Bioss, Beijing, China), and incubated the secondary antibody (1:200, Guge Biology Co., Ltd, Wuhan, China) at 37°C for 1 h. All images were obtained using a Nikon Eclipse Ti-SR microscope plus Nikon DS-U3 camera (Nikon, Osaka, Japan).
Immunofluorescence analysis of tight junction proteins
The sections of jejunum were incubated with blocking buffer containing anti-ZO-1 antibody or anti-occludin antibody (Santa Cruz Biotechnology, Inc., Dallas, TX, U.S.A.), then with a fluorescein isoth – iocyanate (FITC)-conjugated secondary antibody. After washed, the sections were mounted in medium containing 40 mg/mL 6- diamidino-2-phenylindole (DAPI). Finally, the sections were observed and photographed using a fluorescence microscope (ECLIPSE TI-SR, Nikon) and integral optical density of fluorescence were examined by image J software (version 1.53e, Maryland, U.S.A.).
Statistical analysis
SPSS 18.0 (SPSS Inc., Chicago, IL, U.S.A.) was used for data analysis. And the experimental data are presented as the mean ± SEM. Statistical significance was determined by one-way analysis of variance (ANOVA) followed by Duncan’s test as a multiple comparison test for pairwise differences using. Differences were considered significant when p < .05.
Results
Food intake and body weight gain
The data for food intake and body weight gain are presented in . The food intake of the diabetic model group (CD) was significantly greater than that of the control group (C). However, high concentration xylitol (X3 group, 5 g/kg·bw) reduced the food intake of diabetic rats compared with the untreated diabetic group (CD) (). Although food intake of the C group was significantly lower, rats in this groups gained body weight compared to the CD, X1, X2, and X3 groups. Xylitol had no significant effect on the weight of diabetic rats ().
Figure 1. Effects of xylitol on food intake (A) and body weight gain (B) in different animal groups during the whole feeding period. Fasting blood glucose concentrations were measured during the 5-week experimental period (C). Oral glucose tolerance test (OGTT) on the last day of the experimental period (D). Results are given as means ± SEM. Differences between groups were determined by ANOVA followed by Duncan’s test. * p < .05 vs control group; # p < .05 vs diabetic control group. C: control group; CD: diabetic control group; X1: 1.25 g/kg·bw xylitol; X2: 2.5 g/kg·bw xylitol; X3: 5 g/kg·bw xylitol.
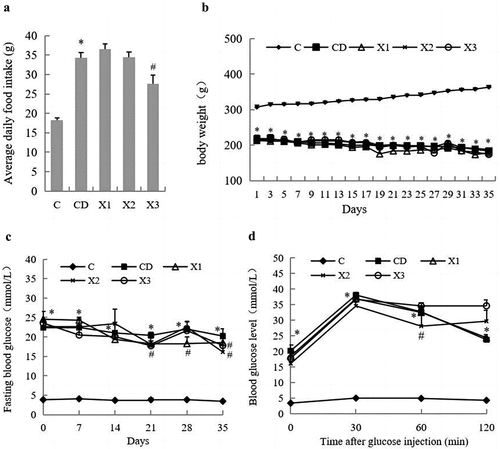
Blood glucose levels and oral glucose tolerance test (OGTT)
Weekly FBG levels are presented in . FBG values (≧16.7 mmol/L) of rats in the CD group always were significantly higher than rats in the C group, which proved the diabetic model’s validity. Although xylitol had no significant effect on FBG in diabetic rats within 14 days, the FBG levels of the X1 group at 21, 28 and 35 days, and the X3 group at 21 and 35 days, were significantly lower than those in the CD group (p < .05) ().
The OGTT was performed in the last week of the 5-week experimental period and the data are shown in . The results showed that the blood sugar levels and area under the curve (AUC) in the CD group were significantly higher than those in C group. Compared with the CD group, the blood glucose values of rats in the X1, X2 or X3 groups decreased to different degrees at 0, 30, 60 and 120 min. The median dose xylitol group (X2) had the most remarkable effect on AUC reduction ().
Changes in insulin, TC, TG and HDL-C concentration in serum
The serum insulin level of diabetic rats (CD group) was significantly lower than that of the control group (C). After xylitol was administered orally for 5 weeks, the insulin concentration levels had not changed significantly compared with the CD group (). The levels of TG and TC in serum of rats in the diabetic control group (CD) were significantly greater than in nondiabetic control rats (p < .05). In comparison with the CD group, the levels of TG and TC in X1 and X2 group rats was significantly lower (p < .05), suggesting that xylitol decreased the TG and TC levels in diabetic rats induced by a high-fat diet and STZ (). HDL-C in diabetic rats was decreased compared with rats in the C group. Xylitol had no effect on the HDL-C levels of diabetic rats ().
Figure 2. Xylitol had no significant effect on insulin concentration in serum but ameliorated lipid disorders in STZ-induced diabetic rats. (A) serum insulin level, (B) TG: triglyceride, (C) TC: total cholesterol, (D) HDL-C: high density lipoprotein cholesterol. Results are given as means ± SEM. Differences between groups were determined by ANOVA followed by Duncan’s test. * p < .05 vs control group; # p < .05 vs diabetic control group. C: control group; CD: diabetic control group; X1: 1.25 g/kg·bw xylitol; X2: 2.5 g/kg·bw xylitol; X3: 5 g/kg·bw xylitol.
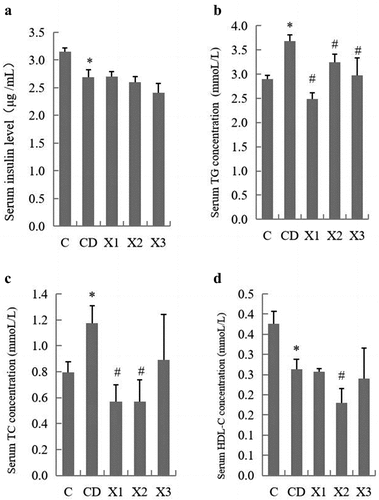
Xylitol ameliorated clinical symptoms in the small intestine of diabetic rats
H&E staining of rat ileum slices was observed under the optical microscope. In group C, the structure of jejunum and ileum tissue was intact, the glands were arranged neatly and tightly, and the villi of the intestinal mucosa were not damaged. Compared to the control (C) group, histological examination of the jejunum () and ileum () in the CD group showed extensive ulceration of the epithelial layer, crypt damage, and destroyed mucosal glands. However, the structure of the jejunum and ileum of the rats in the xylitol groups (X1, X2, X3) was significantly better compared with the CD group. The structure of the mucosa and submucosa was normal, and the layers were clear. A small number of villous epithelial cells were exfoliated, and the crypt structure was clear.
Figure 3. Xylitol ameliorated clinical symptoms in the diabetic rat in small intestine. (A) and (B) are representative H&E stained histological section from the jejunum and ileum (original magnifications, ×100). (C) Xylitol reduced DAO (diamine oxidase) concentration in serum of the diabetic rat. (D) Xylitol increased concentrations of sIgA in intestinal tissues of the diabetic rat. Results are given as means ± SEM. Differences between groups were determined by ANOVA followed by Duncan’s test. * p < .05 vs control group; # p < .05 vs diabetic model group. C: control group; CD: diabetic control group; X1: 1.25 g/kg·bw xylitol; X2: 2.5 g/kg·bw xylitol; X3: 5 g/kg·bw xylitol.
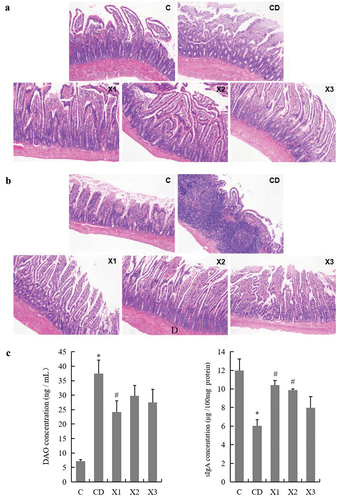
The results of DAO concentration in rat blood by ELISA () showed that DAO concentration in the CD group was significantly higher than that in the C group (p < .05), indicating that the ileal mechanical barrier in diabetic rats was damaged. DAO concentration in the blood of rats in the X1 group decreased significantly compared with the CD group (p < .05), indicating that 1.25 g/kg xylitol could play a role in improving intestinal mechanical barrier function of diabetic rats. Meanwhile, moderate (X2) and high doses (X3) of xylitol non-significantly decreased the content of DAO in the blood. Diabetes mellitus not only destroyed the structure of the small intestine, but also changed the concentration of secretory Ig A (sIgA) in ileal tissue. Compared with group C, the concentrations of sIgA in the CD group rat ileum was significantly lower (p < .05). A greater concentration of sIgA was detected in the rat ileum from the xylitol groups (X1, X2, X3), more similar to the levels closer to the C group ().
Xylitol reduced jejunal and ileal inflammation in diabetic rats
Rats in the CD group had significantly more macroscopic inflammation than those in C group. Compared to rats in the CD group, rats in the xylitol groups had less histologic evidence of diabetes mellitus – induced inflammation (). In this study, the infiltration of CD68+ macrophages into ileal tissue was detected by immunohistochemistry. There was minimal infiltration of macrophages () into the ileum of C group rats. Diabetes mellitus triggered an increased infiltration of CD68+ macrophages into the ileal lesion area in CD group rats compared to C group rats. Treatment with xylitol (X1, X2 and X3 groups) decreased the inflammatory infiltration of macrophages compared with the CD group. To determine the anti- inflammatory effect of xylitol on diabetes mellitus – induced intestinal mucosal barrier injury, secretion of the inflammatory markers TNF-α and IL-6 were evaluated in serum. Diabetes mellitus caused a significant increase in the concentration of TNF-α and IL-6 as compared with that in serum from C group rats. Treatment of rats with xylitol significantly reduced the diabetes mellitus – induced production of TNF-α (in X2 and X3) and IL-6 (in X1 and X2) compared with that in the CD group (p < .05) (). Together, these data indicated that treatment with xylitol ameliorated ileal inflammation caused by diabetes mellitus.
Figure 4. Xylitol ameliorated the small intestinal inflammation during the pathological process in STZ-induced diabetic rats. (A) Representative images of CD68+ cell infiltration (original magnifications, ×200). Formalin – fixed paraffin – embedded 5 μm cross-sections were stained with CD68+ primary antibody and analyzed for macrophage inflammatory infiltration. The concentration of inflammatory markers TNF-α (B) and IL-6 (C) in serum were evaluated by ELISA. The concentration of indicators of inflammatory infiltration myeloperoxidase (MPO) in serum and eosinophil peroxidase (EPX) in ileal tissue were detected using ELISA. Results are given as means ± SEM. Differences between groups were determined by ANOVA followed by Duncan’s test. * p < .05 vs control group; # p < .05 vs diabetic control group. C: control group; CD: diabetic control group; X1: 1.25 g/kg·bw xylitol; X2: 2.5 g/kg·bw xylitol; X3: 5 g/kg·bw xylitol.
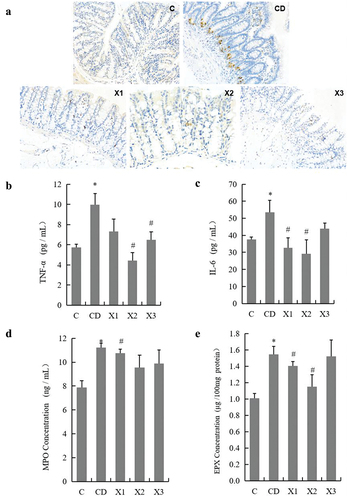
As shown in , there was a marked increase in serum myeloperoxidase (MPO), an indicator of ileal infiltration by polymorphonuclear leukocytes, eosinophil peroxidase (EPX), an indicator of ileal infiltration by eosinophils in ileal tissue from rats in the CD group (11.23 ± 0.36 ng/mL in serum and 1.55 ± 0.10 μg/100 mg tissue protein for MPO and EPX, respectively) compared to control rats (7.88 ± 0.56 ng/mL in serum and 1.01 ± 0.06 μg/100 mg tissue protein for MPO and EPX, respectively) (p < .05). Administration of 1.25 g/kg·bw xylitol to diabetes mellitus rats significantly decreased MPO and EPX (10.75 ± 0.35 ng/mL in serum and 1.41 ± 0.05 μg/100 mg tissue protein for MPO and EPX, respectively) (p < .05).
Xylitol improves the secretion of mucin 2 and the expression and distribution of tight junction proteins
Mucus secreted by goblet cells protects and lubricates mucosa. After AB-PAS staining, the mixed mucus secreted by goblet cells was purple. Mucus secreted by goblet cells in the jejunum and ileum of rats in the CD group was much less than that in the C group, both in the intestinal mucosa and on the surface of the intestinal cavity (). The mucus secreted by goblet cells in the ileum of rats with three different doses of xylitol increased to varying degrees, especially in the intestinal mucosa, compared with that in the CD group.
Figure 5. Xylitol preserves the mucosal barrier by improving the secretion of intestinal mucus. (A) and (B) are representative AB-PAS stained histological sections from the jejunum and ileum (original magnifications, ×100). C: control group; CD: diabetic control group; X1: 1.25 g/kg·bw xylitol; X2: 2.5 g/kg·bw xylitol; X3: 5 g/kg·bw xylitol.
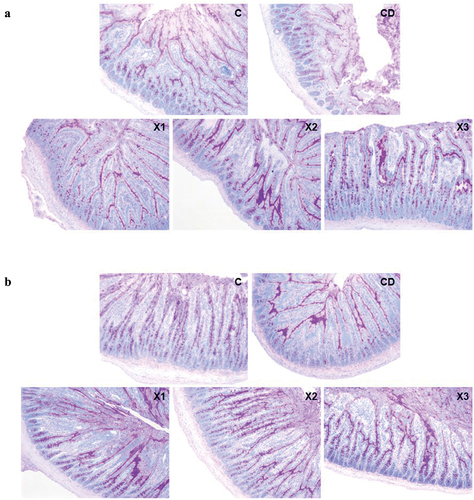
To investigate the effect of xylitol on intestinal mucus secretion in diabetic rats, we detected the expression levels of mucin 2 and intestinal trifoliate factor (ITF) in ileal tissue by immunofluorescence. Diabetes mellitus significantly down-regulated the protein expression levels of mucin 2 () and ITF () compared with those in the C group, whereas xylitol significantly inhibited the diabetes – induced down-regulation of mucin 2 and ITF. Immunofluorescence and confocal imaging analyses were used to determine the expression and location of tight junction-associated ZO-1 and occludin in rats. The results showed that diabetes induced a decrease in the expression levels of ZO-1 () and occludin () in ileal tissue (CD group). By contrast, administration of xylitol at all doses resulted in significantly greater expression of ZO-1 and occludin, with normal protein distribution compared with that in CD group rats.
Figure 6. Xylitol preserves the mucosal barrier by improving the secretion of mucin and ameliorating the expression and distribution of tight junction proteins. Immunostaining of Carnoy-fixed tissue sections for (A) mucin-2 (anti-mucin-2 and FITC-anti-rabbit IgG, green; DAPI, blue) and (B) intestinal trefoil factor (ITF) (anti-intestinal trefoil factor and FITC-anti-rabbit IgG, green; DAPI, blue). (C) Immunostaining of jejunum tissue sections for ZO-1 (anti-ZO-1 and FITC-anti-rabbit IgG, green; DAPI, blue). (D) Immunostaining of jejunum tissue sections for occludin (anti-occludin and FITC-anti-rabbit IgG, green; DAPI, blue). (E) Integral optical density value of immunostaining of jejunum tissue sections for mucin-2, ITF(F), ZO-1(G), and occludin(H). Results are given as means ± SEM. Differences between groups were determined by ANOVA followed by Duncan’s test. * p < .05 vs control group; # p < .05 vs diabetic control group. C: control group; CD: diabetic control group; X1: 1.25 g/kg·bw xylitol; X2: 2.5 g/kg·bw xylitol; X3: 5 g/kg·bw xylitol.
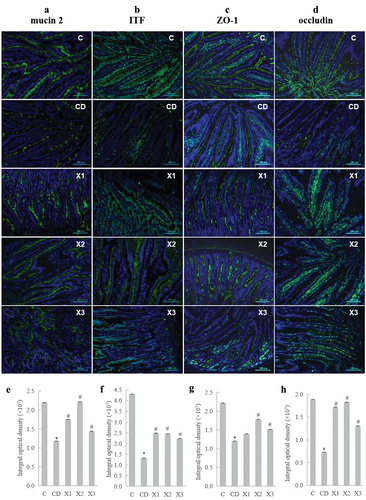
Discussion
Diabetes mellitus is a chronic disease, which can cause many complications in different organs. With the rapidly rising incidence, diabetes mellitus requires lifelong medical attention and dietary restrictions. In this study, we used a combination of high fat diet and low dose of STZ treatment to generate a diabetic rat model. The feeding of high fat diet (HFD) for a period of 3 weeks produced rats with insulin resistance syndrome as was characterized by the mild hyperglycemia, hypertriglyceridemia, hypercholesterolemia and reduced high density lipoprotein cholesterol levels, a condition similar to prediabetic, insulin-resistant state. The development of diabetes occurs in insulin-resistant HFD-fed rats following injected with low dose of STZ. STZ is one of the most prominent diabetogenic chemicals in diabetes research, which is a cytotoxic glucose analogues. STZ induces diabetes mainly by destroying pancreatic β cells, which are the main cells that produce and secrete insulin in the body. When these cells are destroyed, the secretion of insulin will be reduced, resulting in blood sugar not being properly absorbed and utilized. During this process, unutilized blood sugar stimulates the brain’s hunger center, leading to an increase in feed intake. After ingestion, due to insufficient insulin secretion, the anabolism of protein and fat in diabetic rats decreased, and the catabolism accelerated, resulting in weight loss during the experimental period.
Controlling energy intake is one of the major concerns in people who are diabetic. Controlled energy intake is important not only to manage their body weight but also to maintain their status of disease in a physiologically better condition. To successfully achieve this goal, most diabetic individuals use artificial sweeteners such as aspartame, neotame or sucralose. Although all of these chemically originated artificial sweeteners are significantly sweeter than sucrose, the regular or long-term consumption of these sweeteners may be a major concern due to their negative side effects (Islam & Indrajit, Citation2012). In contrast, sugar alcohols have been reported to be better alternative sweeteners with similar sweetness but relatively lower caloric value compared to sucrose, and have many other beneficial effects on health (Mäkinen, Citation2011).
Among the many sugar alcohols, xylitol has been reported as a better sweetener due to reduced or nonexistent side effects, lower caloric value and lower cariogenicity in the normal diet and in the diet of diabetics. The main argument for its use by diabetics is that it does not disturb the diabetic control. It has been reported that intake of xylitol may be beneficial in preventing the development of metabolic abnormalities in rats with diet-induced obesity (Amo et al., Citation2011). It also has been shown that a diet containing 1 or 2 grams of xylitol per 100 kcal of energy did not cause problems with lipogenesis because it suppressed high fat diet induced fat accumulation, and may be useful to control or prevent diabetes and other metabolic disorders (Amo et al., Citation2011). Another study concluded xylitol had significant effects on ameliorating several diabetes-related abnormalities in nondiabetic rats (Islam, Citation2011). Our study revealed that administration of 5 g/kg·bw xylitol could reduce food intake in diabetic rats although change in body weight was not significantly different compared with the untreated diabetic control (CD) group.
Previous research also investigated the anti-diabetic effects of xylitol in a T2D rat model. The results suggested that xylitol has promising effects for ameliorating common T2D-related abnormalities in rats (Islam & Indrajit, Citation2012). However, another study reported that xylitol did not increase serum insulin significantly in either rats or in healthy human subjects after a single 30 g oral dose of xylitol (Salminen et al., Citation1982). In our study, three doses of xylitol had no significant effect on FBG in diabetic rats after 14 days of administration, but significantly lowered FBG levels at the lower dose after 21, 28 and 35 days compared to rats in the CD group. However, xylitol did not significantly increase serum insulin in diabetic rats. Therefore, we hypothesize speculate that xylitol may have other mechanisms to improve the symptoms of diabetes besides affecting insulin secretion.
Some studies investigating the underlying mechanisms involved in disease development in diabetes point to the role of the dysregulation of the intestinal barrier. Recent studies have proved that diabetes mellitus affects gastrointestinal morphology and function. Conversely, several investigations provide evidence that altered bowel function contributes to the pathogenesis of diabetes mellitus. The intestinal barrier is associated with intestinal permeability and intestinal inflammation. There is evidence that xylitol may maintain the barrier function of the skin in sodium lauryl sulphate – induced acute irritation and exhibits anti-inflammatory activities. Evidence in that study suggested that xylitol at 8.26% concentration could exert a beneficial role in improving more severe inflammation (Szél et al., Citation2015). In light of this, our present study investigated the potential of xylitol to alleviate damage to the intestinal mechanical barrier and intestinal inflammation. In our study, we found very serious damage to the villi of the jejunum and ileum in diabetic rats. The ulceration of the epithelial layer, crypt damage, and the destruction of glands in the intestinal mucosa also were found in diabetic rats. Several studies have shown that diabetes mellitus causes morphological alterations in the small intestine (Zhao et al., Citation2006), including increased mucosal surface area, intestinal weight and number of goblet cells per villus (de Kort et al., Citation2011). The change of DAO concentration, which is used to evaluate intestinal injury and intestinal permeability (F. Han et al., Citation2016) proved the destruction of the intestinal mechanical barrier in diabetic rats. Increased intestinal permeability facilitates translocation of harmful substances and pathogens leading to inflammation (Chichlowski et al., Citation2010), and is a major contributing factor in multiple gastrointestinal tract injuries. Our results showed that xylitol significantly ameliorated the structural damage to the villi and crypts in the jejunum and ileum of diabetic rats. This result was supported by the change in DAO concentration. The histological evidence presented here supports the finding that xylitol could exert a protective effect on the intestinal barrier.
Recent studies show that alterations to intestinal permeability compromise intestinal barrier function and enhance the likelihood of inflammatory responses, possibly contributing to the development of insulin resistance. Increased systemic release of pro-inflammatory cytokines (interleukins, TNF-α) that are known to induce peripheral insulin resistance by activating JNK1 and NF-κB (Hotamisligil & Erbay, Citation2008) has been observed in T2D. We found similar results in this study: an increase of TNF-α and IL-6 concentration in diabetic rats accompanied by a decrease in the secretion of sIgA. The major secreted proinflammatory cytokine TNF-α is one marker of inflammatory progression and severity and a principal inducer of effector molecules that cause the breakdown of gastrointestinal tissues. Xylitol (2.5 g/kg·bw) significantly lowered the concentration of TNF-α and IL-6 as compared with that in serum from untreated diabetic rats. In a study of anti-inflammatory effects, porphyromonas gingivalis infection increased the production of representative proinflammatory cytokines, such as TNF-α and interleukin (IL)-1β, whereas xylitol inhibited the release of TNF-α and IL-1β induced by the infection. In vitro, xylitol has been shown to have anti-inflammatory effects that inhibit the production of cytokines induced by porphyromonas gingivalis in Raw 264.7 cells (S.-J. Han et al., Citation2005). Although most of these studies focused on cells, the current study is the first to demonstrate reduction in pro-inflammatory cytokines by xylitol in diabetic rats.
Macrophages are phagocytic cells that play an important role in chronic inflammation and are responsible for the production of proinflammatory cytokines, especially in T2D (Scull et al., Citation2010). The number of macrophages increases in T2D patients. In previous research, the number of macrophages was proven to increase significantly in mouse models of T2D compared to healthy mice. This study showed that VipAlbumin® can reduce macrophage numbers to nearly normal levels by decreasing activation of Treg and suppressing the macrophages. In our study, diabetes mellitus triggered an increased infiltration of CD68+ macrophages into the ileal lesion area in rats, whereas treatment with xylitol at all doses decreased this inflammatory infiltration. This is consistent with the reduced secretion of inflammatory cytokines in diabetic rats. The production of cytokines and chemokines are crucial for the recruitment and activation of immune cells (Oswald, Citation2006). However, at present, whether the inhibitory effect of xylitol on the secretion of inflammatory factors and the inflammatory infiltration of macrophages are closely related to Treg cell activation or to other immune cells remains to be studied further.
Epithelial barrier function is maintained by connections between adjoining epithelial cells consisting of tight junctions (TJs) and adherens junctions. Several reports have reviewed the structure and function of TJs (Anderson & Van Itallie, Citation2009; Sugimoto et al., Citation2019). TJs have a principal role in regulating paracellular transport across the intestinal epithelium, which is responsible for sealing the intercellular spaces between adjacent cells and restricting paracellular movement of compounds across the intestinal mucosa (McCall et al., Citation2009). Increased TJ permeability may provide major sites for both infection and the establishment of inflammation in the gut (Qin et al., Citation2009). Ultrastructural examination of the duodenum from diabetic patients revealed altered TJ structure and an increase in paracellular spaces between epithelial cells compared to healthy control subjects (Secondulfo et al., Citation2004).
In support of the human data, biobreed diabetes-prone rats have been found to have increased intestinal permeability associated with decreased expression of the TJ protein claudin-1 before the onset of insulitis and clinical diabetes. A recent study examined barrier function in diabetic patients at various stages of disease progression. The intestinal permeability was increased in all diabetic groups (de Kort et al., Citation2011). Studies in biobreed rats have shown that the increased permeability detected in prediabetic rats is related to decreased expression of claudin-1 and occludin (Meddings et al., Citation1999; Neu et al., Citation2005). Our data show that xylitol reversed the diabetes-induced expression of TJ proteins (ZO-1 and occludin), thereby reducing the severity of gut inflammation. The results of our study suggest that xylitol is an important therapeutic factor in determining intestinal epithelium integrity in rats with diabetes -induced intestinal mucosal barrier injury.
Conclusion
We report here for the first time that moderate oral administration of xylitol has a protective effect on diabetes -induced intestinal mucosal inflammatory lesions and intestinal permeability changes. Xylitol can alleviate the damage to intestinal structure in diabetic rats by improving the expression of tight junction proteins, and attenuate the intestinal inflammatory response by inhibiting the secretion of pro-inflammatory cytokines. These results suggest that xylitol has the potential to relieve intestinal mucosal barrier injury for diabetic patients when used as a sweetener and may have a positive effect on the health management of patients with diabetes mellitus.
Ethics approval
This study was approved by the Committee on the Ethics of Animal Experiments of Zhejiang Gongshang University (Permit Number: ZJGU 20,210,007).
Acknowledgements
This work was supported by Zhejiang Provincial Natural Science Foundation of China under Grant number LY22C200013; Special Fund for Business Expenses of Colleges and Universities in Zhejiang Province under Grant number XRK21005, and Food Safety Key Laboratory of Zhejiang Province, Ministry of Education, P. R. China, and the enterprise cooperation project with Zhejiang Huakang Pharmaceutical Co., Ltd (Kaihua, China).
Disclosure statement
No potential conflict of interest was reported by the author(s).
Additional information
Funding
References
- Amo, K., Arai, H., Uebanso, T., Fukaya, M., Koganei, M., Sasaki, H., Yamamoto, H., Taketani, Y., & Takeda, E. (2011). Effects of xylitol on metabolic parameters and visceral fat accumulation. Journal of Clinical Biochemistry and Nutrition, 49(1), 1–11. https://doi.org/10.3164/jcbn.10-111
- Anderson, J. M., & Van Itallie, C. M. (2009). Physiology and function of the tight junction. Cold Spring Harbor Perspectives in Biology, 1(2), a002584. https://doi.org/10.1101/cshperspect.a002584
- Arrieta, M. C., Bistritz, L., & Meddings, J. B. (2006). Alterations in intestinal permeability. Gut, 55(10), 1512–1520. https://doi.org/10.1136/gut.2005.085373
- Chichlowski, M., Westwood, G. S., Abraham, S. N., Hale, L. P., & Unutmaz, D. (2010). Role of mast cells in inflammatory bowel disease and inflammation-associated colorectal neoplasia in il-10-deficient mice. PloS One, 5(8), e12220. https://doi.org/10.1371/journal.pone.0012220
- Cho, N. H. (2016). Q&a: Five questions on the 2015 idf diabetes atlas. Diabetes Research and Clinical Practice, 115, 157–159. https://doi.org/10.1016/j.diabres.2016.04.048
- de Kort, S., Keszthelyi, D., & Masclee, A. A. (2011). Leaky gut and diabetes mellitus: What is the link? Obesity Reviews: An Official Journal of the International Association for the Study of Obesity, 12(6), 449–458. https://doi.org/10.1111/j.1467-789X.2010.00845.x
- Ghosh, S., & Sudha, M. L. (2012). A review on polyols: New frontiers for health-based bakery products. International Journal of Food Sciences and Nutrition, 63(3), 372–379. https://doi.org/10.3109/09637486.2011.627846
- Han, F., Lu, Z., Liu, Y., Xia, X., Zhang, H., Wang, X., & Wang, Y. (2016). Cathelicidin-bf ameliorates lipopolysaccharide-induced intestinal epithelial barrier disruption in rat. Life Sciences, 152, 199–209. https://doi.org/10.1016/j.lfs.2016.03.041
- Han, S.-J., Jeong, S.-Y., Nam, Y.-J., Yang, K.-H., Lim, H.-S., & Chung, J. (2005). Xylitol inhibits inflammatory cytokine expression induced by lipopolysaccharide from porphyromonas gingivalis. Clinical and Diagnostic Laboratory Immunology, 12(11), 1285–1291. https://doi.org/10.1128/CDLI.12.11.1285-1291.2005
- Hotamisligil, G. S., & Erbay, E. (2008). Nutrient sensing and inflammation in metabolic diseases. Nature Reviews Immunology, 8(12), 923–934. https://doi.org/10.1038/nri2449
- Islam, M. S. (2011). Effects of xylitol as a sugar substitute on diabetes-related parameters in nondiabetic rats. Journal of Medicinal Food, 14(5), 505–511. https://doi.org/10.1089/jmf.2010.0015
- Islam, M. S., & Indrajit, M. (2012). Effects of xylitol on blood glucose, glucose tolerance, serum insulin and lipid profile in a type 2 diabetes model of rats. Annals of Nutrition & Metabolism, 61(1), 57–64. https://doi.org/10.1159/000338440
- Ji, J., Zhang, C., Luo, X., Wang, L., Zhang, R., Wang, Z., Fan, D., Yang, H., & Deng, J. (2015). Effect of stay-green wheat, a novel variety of wheat in china, on glucose and lipid metabolism in high-fat diet induced type 2 diabetic rats. Nutrients, 7(7), 5143–5155. https://doi.org/10.3390/nu7075143
- Mäkinen, K. K. (2011). Sugar alcohol sweeteners as alternatives to sugar with special consideration of xylitol. Medical Principles and Practice: International Journal of the Kuwait University, Health Science Centre, 20(4), 303–320. https://doi.org/10.1159/000324534
- Mäkinen, K. K. (2016). Gastrointestinal disturbances associated with the consumption of sugar alcohols with special consideration of xylitol: Scientific review and instructions for dentists and other health-care professionals. International Journal of Dentistry, 2016, 5967907. https://doi.org/10.1155/2016/5967907
- McCall, I. C., Betanzos, A., Weber, D. A., Nava, P., Miller, G. W., & Parkos, C. A. (2009). Effects of phenol on barrier function of a human intestinal epithelial cell line correlate with altered tight junction protein localization. Toxicology and Applied Pharmacology, 241(1), 61–70. https://doi.org/10.1016/j.taap.2009.08.002
- Meddings, J. B., Jarand, J., Urbanski, S. J., Hardin, J., & Gall, D. G. (1999). Increased gastrointestinal permeability is an early lesion in the spontaneously diabetic bb rat. The American Journal of Physiology, 276(4), G951–957. https://doi.org/10.1152/ajpgi.1999.276.4.G951
- Neu, J., Reverte, C. M., Mackey, A. D., Liboni, K., Tuhacek-Tenace, L. M., Hatch, M., Li, N., Caicedo, R. A., Schatz, D. A., & Atkinson, M. (2005). Changes in intestinal morphology and permeability in the biobreeding rat before the onset of type 1 diabetes. Journal of Pediatric Gastroenterology and Nutrition, 40(5), 589–595. https://doi.org/10.1097/01.MPG.0000159636.19346.C1
- Oswald, I. P. (2006). Role of intestinal epithelial cells in the innate immune defence of the pig intestine. Veterinary Research, 37(3), 359–368. https://doi.org/10.1051/vetres:2006006
- Park, E., Na, H. S., Kim, S. M., Wallet, S., Cha, S., & Chung, J. (2014). Xylitol, an anticaries agent, exhibits potent inhibition of inflammatory responses in human thp-1-derived macrophages infected with porphyromonas gingivalis. Journal of Periodontology, 85(6), e212–223. https://doi.org/10.1902/jop.2014.130455
- Qin, H., Zhang, Z., Hang, X., & Jiang, Y. (2009). L. Plantarum prevents enteroinvasive Escherichia coli-induced tight junction proteins changes in intestinal epithelial cells. BMC Microbiology, 9(1), 63. https://doi.org/10.1186/1471-2180-9-63
- Salminen, S., Salminen, E., & Marks, V. (1982). The effects of xylitol on the secretion of insulin and gastric inhibitory polypeptide in man and rats. Diabetologia, 22(6), 480–482. https://doi.org/10.1007/BF00282594
- Sato, H., Ide, Y., Nasu, M., & Numabe, Y. (2011). The effects of oral xylitol administration on bone density in rat femur. Odontology, 99(1), 28–33. https://doi.org/10.1007/s10266-010-0143-2
- Scull, C. M., Hays, W. D., & Fischer, T. H. (2010). Macrophage pro-inflammatory cytokine secretion is enhanced following interaction with autologous platelets. Journal of Inflammation (London, England), 7(1), 53. https://doi.org/10.1186/1476-9255-7-53
- Secondulfo, M., Iafusco, D., Carratù, R., de Magistris, L., Sapone, A., Generoso, M., Mezzogiorno, A., Sasso, F. C., Cartenì, M., De Rosa, R., Prisco, F., & Esposito, V. (2004). Ultrastructural mucosal alterations and increased intestinal permeability in non-celiac, type i diabetic patients. Digestive and Liver Disease: Official Journal of the Italian Society of Gastroenterology and the Italian Association for the Study of the Liver, 36(1), 35–45. https://doi.org/10.1016/j.dld.2003.09.016
- Shen, X., Cai, J., Gao, J., Vaidya, A., Liu, X., Li, W., Chen, S., Zhou, Y., Li, Y., Zhang, Y., Zhao, J., Hu, F. B., Wu, S., & Gao, X. (2018). Nonalcoholic fatty liver disease and risk of diabetes: A prospective study in china. Endocrine Practice: Official Journal of the American College of Endocrinology and the American Association of Clinical Endocrinologists, 24(9), 823–832. https://doi.org/10.4158/EP-2018-0098
- Sugimoto, K., Ichikawa-Tomikawa, N., Kashiwagi, K., Endo, C., Tanaka, S., Sawada, N., Watabe, T., Higashi, T., & Chiba, H. (2019). Cell adhesion signals regulate the nuclear receptor activity. Proceedings of the National Academy of Sciences of the United States of America, 116(49), 24600–24609. https://doi.org/10.1073/pnas.1913346116
- Szél, E., Polyánka, H., Szabó, K., Hartmann, P., Degovics, D., Balázs, B., Németh, I. B., Korponyai, C., Csányi, E., Kaszaki, J., Dikstein, S., Nagy, K., Kemény, L., & Erős, G. (2015). Anti-irritant and anti-inflammatory effects of glycerol and xylitol in sodium lauryl sulphate-induced acute irritation. Journal of the European Academy of Dermatology and Venereology, 29(12), 2333–2341. https://doi.org/10.1111/jdv.13225
- Ur-Rehman, S., Mushtaq, Z., Zahoor, T., Jamil, A., & Murtaza, M. A. (2015). Xylitol: A review on bioproduction, application, health benefits, and related safety issues. Critical Reviews in Food Science and Nutrition, 55(11), 1514–1528. https://doi.org/10.1080/10408398.2012.702288
- Van Loveren, C. (2004). Sugar alcohols: What is the evidence for caries-preventive and caries-therapeutic effects? Caries Research, 38(3), 286–293. https://doi.org/10.1159/000077768
- Zhao, J., Frøkjaer, J. B., Drewes, A. M., & Ejskjaer, N. (2006). Upper gastrointestinal sensory-motor dysfunction in diabetes mellitus. World Journal of Gastroenterology, 12(18), 2846–2857. https://doi.org/10.3748/wjg.v12.i18.2846