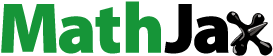
ABSTRACT
Polygonatum cyrtonema Hua polysaccharides (PCP) have beneficial effects on lipid metabolism and oxidative stress. Here, PCP extraction was optimized using response surface methodology (RSM), and the antioxidant and lipid-lowering effects of its main component, PCP1, were evaluated in vitro. The maximum PCP yield was 11.05% at an extraction temperature was 72°C, liquid-to-solid ratio of 21, extraction time of 96 min, and two extraction rounds. PCP1 was purified by column chromatography and showed significant scavenging activity against 1,1-diphenyl-2-picrylhydrazyl (DPPH), hydroxyl, and 2, 2'-azino-bis 3-ethylbenzthiazo-line-6-sulfonic acid (ABTS) radicals at concentration of 0.2–1.0 mg/mL. At a concentration of 200 g/mL, PCP1 significantly improved lipid disorder and damage induced by palmitic acid in HepG2 cells. These results show that PCP has potent antioxidant and lipid-lowering effects in vitro; moreover, the PCP extraction rate is significantly increased using RSM. Therefore, PCP is an effective antioxidant and lipid-lowering product with potential medicinal applications.
1. Introduction
Polygonatum cyrtonema Hua (PCH), a perennial herb in the family Liliaceae, is often used as a tonic for kidney disorders, a yin nourishing food, and a tissue moistener (J. Li et al., Citation2022). PCH contains natural substances, such as polysaccharides, saponins, flavonoids, and amino acids, and has various biological activities (L. Li et al., Citation2018; Tang et al., Citation2019). As the main natural active substance in PCH, PCH polysaccharide (PCP) has hypoglycemic, anti-inflammatory, anti-oxidation, anti-fatigue, and anti-depression effects (Cui et al., Citation2018; J. Li et al., Citation2022; S. Liu et al., Citation2022; F. Shen et al., Citation2022; W. D. Shen et al., Citation2021). Polysaccharides extracted from PCH cultivated on Mount Jiuhua are considered high quality. At present, PCH from Mount Jiuhua is not only an important source of income for residents, but has also been developed into tea, medicinal wine, and other products. Therefore, the enormous medicinal and economic value of PCH requires further research (J. Hu et al., Citation2021; Y. Hu et al., Citation2022).
The dysregulation of glucose and lipid metabolism can result in various diseases and poses a significant threat to human health (Ding, Wang, et al., Citation2021; Sun et al., Citation2023). Oxidative stress, insulin resistance, and inflammatory damage are considered as important mechanisms for the occurrence and development of glycolipid metabolic disorder (Ding, Tang, et al., Citation2021; Zhou et al., Citation2023). In a preliminary experiment, we found that a neutral polysaccharide, PCP1, is the main component of PCP. Chemical analysis showed that the molecular weight of PCP1 is 4,650 g/mol, and that it is mainly composed of fructose, glucose, and galactose. Pharmacological studies showed that PCP1 has the effect of improving lipid metabolism disorders, oxidative stress, and structural damage to the intestinal microflora in non-alcoholic fatty liver disease (S. Liu et al., Citation2022). However, we also found that PCP has a low yield and is difficult to separate in the extraction process, which may be related to the solution viscosity and other physical properties of the high-molecular-weight polysaccharide. Response surface methodology (RSM) is often used to identify optimal extraction process parameters and can systematically provide people with effective production processes (Dai et al., Citation2020; Tang et al., Citation2023). Thus, RSM was used to optimize the conditions for PCP extraction.
In this study, hot water extraction and ethanol precipitation were used to extract PCP to ensure the integrity of the polysaccharides. DEAE-52 cellulose and Sephadex G-75 dextran gels were used for the separation and purification of PCP, respectively. Then, the homogeneous component PCP1, as determined by liquid chromatography, was evaluated for in vitro antioxidant and lipid-lowering activities. The objective of this study was to explore the optimal extraction process, and antioxidant and lipid-lowering effects of PCP. The results of this study provide a theoretical reference for the development and utilization of PCH in the Mount Jiuhua area, and for the development of safe and effective drugs for non-alcoholic fatty liver disease prevention and treatment.
2. Materials and methods
2.1 Materials and chemicals
The rhizome of PCH was collected from Mount Jiuhua (30.5° N, 117.8° E) and authenticated by Professor Chunyan Liu (Wannan Medical College, Wuhu, China). The DEAE-52 cellulose and Sephadex G-75 dextran gels were supplied by Solarbio (Beijing, China). Kits for measuring the levels of 1,1-diphenyl-2-picrylhydrazyl (DPPH) radical, hydroxyl radical, 2, 2'-azino-bis 3-ethylbenzthiazo-line-6-sulfonic acid (ABTS) radical, triglycerides (TG), total cholesterol (TC), alanine transaminase (ALT), and aspartate transaminase (AST) were procured from the Nanjing Jiancheng Institute of Biotechnology (Nanjing, China).
2.2 Optimization of PCP extraction
The extraction procedure followed the method described by W. Liu et al. (Citation2022). The PCH rhizome was washed, cut into 6–8 mm slices, baked at 60°C for 72 h, dried, and crushed into a powder with a ~250 μm particle size. Then, 4 g of powder was added to deionized water for extraction according to the experimental design requirements. The cooled extract was centrifuged at 8,000 rpm for 15 min to concentrate the supernatant to 1/10 of the original volume under reduced pressure. Then, we slowly added 3 × the volume of a concentrated solution of anhydrous ethanol, precipitated it in a 4°C freezer for 12 h, and freeze-dried the material to obtain a PCP dry powder. Finally, the PCP yield (%) was calculated using Equation.1:
where W is the weight (g) of PCP and Wm is the weight (g) of the dried PCH powdered sample.
To investigate the effects of extraction temperature (X1: 50, 60, 70, 80, 90°C), liquid-to-solid ratio (X2: 10, 15, 20, 25, 30 mL/g), extraction time (X3: 30, 60, 90, 120, 150 min), and number of extractions (one, two, or three) on PCP yield, we first performed single-factor experiments for which the parameter settings are shown in .
Table 1. Single-factor experimental design.
After determining the range of preliminary extraction parameters using a single-factor experiment, a three-level, three-variable Box – Behnken design (BBD) was applied to statistically optimize the yield of PCP. Three independent variables were tested, including extraction temperature (X1: 60–80°C), liquid-to-solid ratio (X2: 15–25 mL/g), and extraction time (X3: 60–120 min); the response variable was the yield of PCP. The Design-expert 12.0.3 software was employed for statistical analysis of experimental data and 17-run extraction experimental designs were conducted.
2.3 PCP1 isolation and purification
Briefly, 100 mg of PCP powder, from which pigments and protein were previously removed by macroporous resin and Sevag reagent, respectively, was dissolved in 10 mL of deionized water, loaded with a DEAE-52 cellulose column (2.5 × 40 cm), eluted with deionized water at a flow rate of 0.6 mL/min, and collected in a test tube. The anthrone-sulfuric acid reagent was used to track the degree of elution of the polysaccharides. The eluate was collected according to absorbance and subsequently concentrated, dialyzed, and freeze-dried to obtain the PCP1 polysaccharide components.
A Sephadex-75 dextran gel-chromatography column (1.6 × 60 cm) was then used to purify PCP1. Briefly, 30 mg of PCP1 was loaded onto the Sephadex-75 chromatography column and eluted with deionized water at a flow rate of 0.4 mL/min. Lastly, the main peak of the elution curve was collected to obtain the purified PCP1 component.
The Lc-l0Avp high performance gel permeation chromatography (HPGPC) system (Shimadzu, Japan) equipped with a RID-10A refractive index detector and TSKgel G3000PWXL column (7.8 × 300 mm, Tosoh Corp., Japan) was used to determine the homogeneity of PCP1. Parameter settings were as follows: PCP1 concentration, 5 mg/mL; mobile phase, deionized water; flow rate, 0.7 mL/min; injection amount, 20 μL; column temperature, 40°C.
2.4 PCP1 antioxidant activity
2.4.1 PCP1 DPPH radical-scavenging capacity
The DPPH radical-scavenging capacity assay was based on a previously reported method with slight modifications (Souza et al., Citation2012). Briefly, PCP1 was dissolved in deionized water to prepare solutions with concentrations of 0.2, 0.4, 0.6, 0.8, and 1.0 mg/mL. After reacting a mixture of 0.6 mL DPPH reagent and 0.4 mL PCP1 solution under dark conditions at 25°C for 30 min, the absorbance of the mixture was measured at 517 nm and Vc was used as a positive control. The scavenging ability was calculated using Equation. 2:
where Ab is the absorbance measured using ethanol instead of the sample, As is the absorbance of the sample reacting with the DPPH reagent, and Ac is the absorbance of the DPPH reagent replaced by deionized water.
2.4.2 PCP1 hydroxyl radical-scavenging capacity
The Fenton-type reaction (Pan & Wu, Citation2014) was used for the determination of PCP1 hydroxyl radical-scavenging capacity. Briefly, 0.1 mL of different concentrations (0.2, 0.4, 0.6, 0.8, and 1.0 mg/mL) of PCP1 solution were mixed with 1 mL of 9 mM salicylic acid and 1 mL of 9 mM ferrous sulfate solution, and deionized water was added to 10 mL. Then, 10 mL of 9 mM hydrogen peroxide solution was added and the absorbance of the mixed solution was measured at 510 nm after 1 h at 37°C. Vc was used as a positive control and the scavenging capacity was calculated using Equation. 3:
where Ab is the absorbance measured by replacing the sample with deionized water, As is the absorbance of the test sample reaction, and Ac is the absorbance of the salicylic acid – ethanol solution replaced with deionized water.
2.4.3 PCP1 ABTS radical-scavenging capacity
The reaction mixture solution underwent oxidation by oxidizing enzymes to produce ABTS free radicals (Zhu et al., Citation2016). Then, 10 μL of PCP1 solution at different concentrations was mixed with 20 mL of peroxidase solution and 170 μL of ABTS radical solution at 25°C for 6 min and the absorbance was measured at 405 nm; Vc was used as a control group and the scavenging ability of ABTS radicals was calculated using Equation. 4:
where Ab is the absorbance measured by replacing the sample with deionized water, As is the absorbance of the sample reacting with ABTS radical solution, and Ac is the absorbance of ABTS radical solution replaced with deionized water.
2.5 In vitro PCP1 lipid-lowering activity
2.5.1 Cell culture
Human hepatocellular carcinoma cell line HepG2 was purchased from the Cell Bank of the Chinese Academy of Sciences and cultured at 37°C in a humidified thermostatic incubator in the presence of 5% CO2. The culture was performed in Dulbecco’s modified Eagle’s medium (DMEM; Gibco, CA, U.S.A.) containing 10% fetal bovine serum and 1% penicillin – streptomycin. Palmitic acid (PA, 0.2 mM) incubation for 48 h was used to induce cellular lipid accumulation, and different concentrations (100 and 200 μg/mL) of PCP1 solution were added for the last 24 h of the incubation period. PCP1 showed high solubility in the above culture medium and was readily dissolved by slight shaking at 37°C.
2.5.2 CCK-8 assay
HepG2 cells were cultured in 96-well plates, incubated with different concentrations of PA and PCP1 solution, and then detected using CCK-8 reagents.
2.5.3 Oil red O staining
Lipid droplets in HepG2 cells were observed using Oil Red O staining. After the cells cultured in 6-well plates were incubated in PA and PCP1 solution, 4% paraformaldehyde was fixed for 10 min. Then, cells were stained with Oil Red O staining solution for 10 min followed by staining with hematoxylin solution for 3 min, before finally being washed with phosphate buffer saline (PBS) and observed under a light microscope (OLYMPUS IX51, OLYMPUS, U.S.A.).
2.5.4 Biochemical index detection
Cells were cultured in a 6-well cell plate, incubated with PA and PCP1 solutions, and the levels of TG, TC, ALT, and AST were measured using the corresponding commercial kits.
2.6 Statistical analysis
All experimental data were expressed as the mean ± standard deviation (SD). The GraphdPad 8.0.1 software was used for data analysis. Design Expert (Version 12.0.3.0; Stat-Ease Inc., Minneapolis, MN, U.S.A.) was used for the design and data analysis of RSM. Treatment effects were compared using analysis of variance (ANOVA), and the significance of treatment differences was evaluated using the least significant difference (LSD) multi-scope test at a p < .05 significance level.
3. Results and discussion
3.1 Single-factor experiments to determine optimum conditions for PCP extraction yield
To maximize the PCP yield, single-factor experiments were performed using the parameter settings listed in . The extraction process was repeated three times for each set of parameters. The PCP yield increased slightly with increases in the extraction temperature from 40 to 70°C (), the liquid-to-solid ratio from 10 to 20 mL/g (), time from 30 to 90 min (), and extraction rounds from one to two (). Therefore, 70°C, 20 mL/g, 90 min, and two rounds were selected for subsequent analyses.
3.2 Statistical analysis and model fitting
A BBD was applied using the PCP yield as the response variable, and 17 runs were conducted to optimize individual parameters (). In a multiple regression analysis, a quadratic polynomial regression model was fitted and the relationship between PCP yield (Y) and test variables (X1, X2, X3) was defined by Equation. 5, as follows:
Table 2. Box – Behnken design and response value of polygonatum cyrtonema Hua polysaccharide (PCP) extraction.
where X1, X2, and X3 are the extraction temperature, liquid-to-solid ratio, and extraction time, respectively.
ANOVA results are shown in . The F-statistic (13.58) and p-value (0.0012) indicated a good model fit. A lack-of-fit F-statistic of 0.3009 and high p-value (0.8243) indicated that a lack of fitting was not significant compared with the error; therefore, the model was suitable for accurately predicting variation. In addition, the coefficient of determination (R2 = 0.8762) and adjusted coefficient of determination (R2Adj = 0.7714) indicated a high correlation between the independent and response variables (Tian et al., Citation2021; Wang et al., Citation2020).
Table 3. Analysis of variance (ANOVA) of a fitted response-surface quadratic polynomial model.
3.3 Response surface analysis
The Design Expert software was used to describe the interaction between the extraction process and PCP yield, and to estimate the yield over ranges of values for independent variables. The three dimensional (3D) response-surface plots and two dimensional (2D) contour maps are shown in . The PCP yield increased as the liquid-to-solid ratio (X2) increased from 15 to 20 mL/g and the temperature (X1) increased from 60 to 70°C. When X1 or X2 exceeded this range, the PCP yield decreased gradually (). The PCP yield increased as the temperature (X1) increased from 60 to 70°C and time (X3) increased from 60 to 90 min, but decreased gradually when X1 or X3 exceeded these levels (). Finally, the PCP yield increased as X2 increased from 15 to 20 mL/g and X3 increased from 60 to 90 min but decreased as X2 and X3 exceeded these values ().
3.4 Model verification
Using the Box – Behnken design, the theoretical optimal combination of variables in the extraction process was as follows: extraction temperature of 71.547°C, liquid-to-solid ratio of 20.821 mL/g, extraction time of 95.961 min, and two extraction rounds. The theoretical PCP yield was 11.09%. To ensure the operability of the extraction process, the parameters were adjusted as follows: extraction temperature, 72°C; liquid-to-solid ratio, 21 mL/g; extraction time, 96 min, and rounds of extraction, two. An actual yield of 11.05% ± 0.13% was obtained, which was in good agreement with the predicted value (p > .05).
3.5 Isolation and purification of PCP1
As shown in , the main polysaccharide component of PCP, PCP1, was obtained after the separation of PCP using a DEAE-52 cellulose column and purification using a Sephadex-75 chromatography column. HPGPC () revealed that PCP1 is a polysaccharide with a narrow molecular weight distribution.
3.6 PCP1 antioxidant activity
As shown in , in the concentration range of 0.2 to 1.0 mg/mL, the scavenging ability of PCP1 against DPPH, hydroxyl, and ABTS radicals increased gradually with increasing concentrations. Furthermore, PCP1 was more sensitive to DPPH radicals than to hydroxyl or ABTS radicals. At a concentration of 1.0 mg/mL, the maximum scavenging rate (94.7% ± 2.9%) was close to that of Vitamin C (Vc). Reducing excessive free radicals can delay aging and prevent cancer, cardiovascular, and cerebrovascular diseases (Shi et al., Citation2023; Venkatesan et al., Citation2019). These data demonstrate that PCP1 has a high antioxidant capacity and the potential to be used for disease prevention.
Figure 4. In vitro antioxidant activity analysis of polygonatum cyrtonema Hua polysaccharide 1 (PCP1). (a) 1,1-diphenyl-2-picrylhydrazyl (DPPH) radical-scavenging activity. (b) Hydroxyl radical-scavenging activity. (c) 2, 2'-azino-bis 3-ethylbenzthiazo-line-6-sulfonic acid (ABTS) radical-scavenging activity. Data are shown as mean ± standard deviation (S.D.).
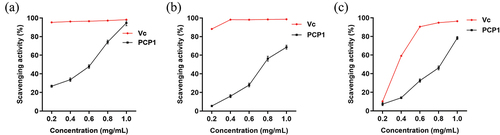
3.7 PCP1 in vitro lipid-lowering activity
Long-term overload with fatty acids and/or sugars can lead to an increase in lipid accumulation in cells, leading to cytotoxicity (Gan et al., Citation2022; Park et al., Citation2014). As shown in , incubation with 0.2 mmol/L PA for 48 h significantly reduced the activity of HepG2 cells and was used to construct the model (Yao et al., Citation2011). As shown in , PCP1 significantly increased cell activity in the PA-treated group. However, at 400 μg/mL, cell activity decreased significantly, presumably owing to the inhibitory effect of PCP1 on cell activity. Therefore, PCP1 concentrations of 100 and 200 μg/mL were selected for subsequent testing.
Figure 5. Lipid-lowering effect of polygonatum cyrtonema Hua polysaccharide 1 (PCP1) in vitro. (a) Effect of palmitic acid (PA) concentration on the activity of HepG2 cells. (b) Effect of PCP1 on the activity of HepG2 cells incubated with PA. (c) Intracellular triglycerides (TG) level. (d) Intracellular total cholesterol (TC) level. (e) Intracellular alanine transaminase (ALT) level. (f) Intracellular aspartate transaminase (AST) level. (g) Oil red O staining diagram. Data are shown as mean ± standard deviation (S.D.) **p < .01 versus normal group, ##p < .01 versus PA incubation group.
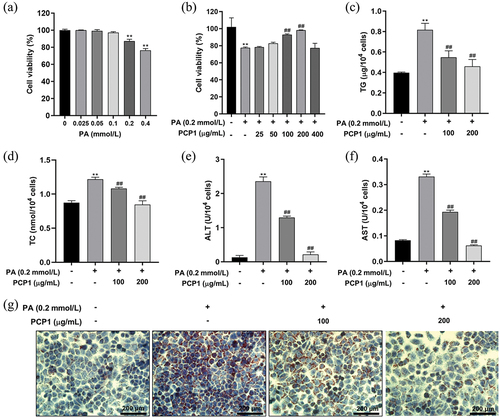
The effects of PCP1 on liver cell damage and fat accumulation are summarized in . Incubation with PCP1 can significantly reduce PA-related increases in cellular TG, TC, ALT, and AST levels, particularly at a PCP1 concentration of 200 µg/mL (). In addition, Oil Red O staining () showed that PCP1 could reduce the significant increases in intracellular lipid droplets and aggregation caused by PA stimulation. These findings indicate that PCP1 can significantly reduce PA-induced cellular fat accumulation in vitro.
4. Conclusions
In this study, PCP was extracted from PCH using hot water extraction and ethanol precipitation, and its yield was optimized using BBD. The optimum extraction process parameters for PCP were extraction temperature of 72°C, liquid-to-solid ratio of 21 mL/g, extraction time of 96 min, and two rounds of extraction. Under these conditions, the extraction yield of PCP was 11.05%, which was consistent with the predicted results. Furthermore, PCP1, a homogeneous polysaccharide component that was isolated and purified from PCP, exhibited a strong ability to scavenge DPPH, hydroxyl, and ABTS radicals in vitro at a concentration of 0.2 to 1.0 mg/mL, and was more responsive to DPPH radicals with a rate of 94.7%. Moreover, PCP1 showed a good alleviating effect on HepG2 cell damage and lipid metabolism disorder induced by palmitic acid. These findings support the development and use of PCP as a natural antioxidant, and provide a theoretical reference for further research on its lipid-lowering and liver protective mechanism.
Disclosure statement
No potential conflict of interest was reported by the author(s).
Additional information
Funding
References
- Cui, X., Wang, S., Cao, H., Guo, H., Li, Y., Xu, F., Zheng, M., Xi, X., & Han, C. (2018). A Review: The Bioactivities and Pharmacological Applications of Polygonatum sibiricum polysaccharides. Molecules, 23(5), 1170. https://doi.org/10.3390/molecules23051170
- Dai, J., Ding, M., Chen, J., Qi, J., Zhu, Y., Li, Z., Zhu, L., & Wang, G. (2020). Optimization of gel mixture formulation based on weighted value using response surface methodology. CyTA - Journal of Food, 18(1), 500–8. https://doi.org/10.1080/19476337.2020.1789746
- Ding, M., Tang, Z., Liu, W., Shao, T., Yuan, P., Chen, K., Zhou, Y., Han, J., Zhang, J., & Wang, G. (2021). Burdock fructooligosaccharide attenuates high glucose-induced apoptosis and oxidative stress injury in renal tubular epithelial cells. Frontiers in Pharmacology, 12, 784187. https://doi.org/10.3389/fphar.2021.784187
- Ding, M., Wang, G., Yuan, P., He, S., Shao, T., Liu, C., & Kong, X. (2021). Research progress in the role and mechanism of polysaccharides in regulating glucose and lipid metabolism. Nan Fang Yi Ke da Xue Xue Bao = Journal of Southern Medical University, 41(3), 471–475. https://doi.org/10.12122/j.issn.1673-4254.2021.03.23
- Gan, Q., Wang, X., Cao, M., Zheng, S., Ma, Y., & Huang, Q. (2022). NF-kappaB and AMPK-Nrf2 pathways support the protective effect of polysaccharides from polygonatum cyrtonema Hua in lipopolysaccharide-induced acute lung injury. Journal of Ethnopharmacology, 291, 115153. https://doi.org/10.1016/j.jep.2022.115153
- Hu, J., Cheng, H., Xu, J., Liu, J., Xing, L., Shi, S., Wang, R., Wu, Z., Yu, N., & Peng, D. (2021). Determination and analysis of monosaccharides in polygonatum cyrtonema Hua polysaccharides from different areas by ultra-high-performance liquid chromatography quadrupole trap tandem mass spectrometry. Journal of Separation Science, 44(18), 3506–3515. https://doi.org/10.1002/jssc.202100263
- Hu, Y., Yin, M., Bai, Y., Chu, S., Zhang, L., Yang, M., Zheng, X., Yang, Z., Liu, J., Li, L., Huang, L., & Peng, H. (2022). An evaluation of traits, nutritional, and medicinal component quality of Polygonatum cyrtonema Hua and P. sibiricum red. Frontiers in Plant Science, 13, 891775. https://doi.org/10.3389/fpls.2022.891775
- Li, L., Liao, B. Y., Thakur, K., Zhang, J. G., & Wei, Z. J. (2018). The rheological behavior of polysaccharides sequential extracted from polygonatum cyrtonema Hua. International Journal of Biological Macromolecules, 109, 761–771. https://doi.org/10.1016/j.ijbiomac.2017.11.063
- Liu, S., Jia, Q. J., Peng, Y. Q., Feng, T. H., Hu, S. T., Dong, J. E., & Liang, Z. S. (2022). Advances in mechanism research on polygonatum in prevention and treatment of diabetes. Frontiers in Pharmacology, 13, 758501. https://doi.org/10.3389/fphar.2022.758501
- Liu, W., Shao, T., Tian, L., Ren, Z., Gao, L., Tang, Z., Fang, Z., Yuan, P., Liu, C., Li, J., Wang, G., & Han, J. (2022). Structural elucidation and anti-nonalcoholic fatty liver disease activity of polygonatum cyrtonema Hua polysaccharide. Food & Function, 13(24), 12883–12895. https://doi.org/10.1039/d2fo03384d
- Li, J., Wang, X., Zhou, R., Cheng, F., Tang, X., Lao, J., Xu, L., He, W., Wan, D., Zeng, H., & Zhang, S. (2022). Polygonatum cyrtonema Hua Polysaccharides Protect BV2 Microglia Relief Oxidative Stress and Ferroptosis by Regulating NRF2/HO-1 Pathway. Molecules, 27(20), 7088. https://doi.org/10.3390/molecules27207088
- Pan, S., & Wu, S. (2014). Cellulase-assisted extraction and antioxidant activity of the polysaccharides from garlic. Carbohydrate Polymers, 111, 606–609. https://doi.org/10.1016/j.carbpol.2014.05.022
- Park, E. J., Lee, A. Y., Park, S., Kim, J. H., & Cho, M. H. (2014). Multiple pathways are involved in palmitic acid-induced toxicity. Food & Chemical Toxicology, 67, 26–34. https://doi.org/10.1016/j.fct.2014.01.027
- Shen, W. D., Li, X. Y., Deng, Y. Y., Zha, X. Q., Pan, L. H., Li, Q. M., & Luo, J. P. (2021). Polygonatum cyrtonema Hua polysaccharide exhibits anti-fatigue activity via regulating osteocalcin signaling. International Journal of Biological Macromolecules, 175, 235–241. https://doi.org/10.1016/j.ijbiomac.2021.01.200
- Shen, F., Xie, P., Li, C., Bian, Z., Wang, X., Peng, D., Zhu, G., & Huang, Z. (2022). Polysaccharides from polygonatum cyrtonema hua reduce depression-like behavior in mice by inhibiting oxidative stress-calpain-1-NLRP3 signaling axis. Oxidative Medicine and Cellular Longevity, 2022, 1–17. https://doi.org/10.1155/2022/2566917
- Shi, L., Jiang, C., Xu, H., Wu, J., Lu, J., He, Y., Yin, X., Chen, Z., Cao, D., Shen, X., Hou, X., & Han, J. (2023). Hyperoside ameliorates cerebral ischaemic–reperfusion injury by opening the TRPV4 channel in vivo through the IP 3 -PKC signalling pathway. Le Pharmacien biologiste, 61(1), 1000–1012. https://doi.org/10.1080/13880209.2023.2228379
- Souza, B. W. S., Cerqueira, M. A., Bourbon, A. I., Pinheiro, A. C., Martins, J. T., Teixeira, J. A., Coimbra, M. A., & Vicente, A. A. (2012). Chemical characterization and antioxidant activity of sulfated polysaccharide from the red seaweed gracilaria birdiae. Food Hydrocolloids, 27(2), 287–292. https://doi.org/10.1016/j.foodhyd.2011.10.005
- Sun, Y., Guo, L. Q., Wang, D. G., Xing, Y. J., Bai, Y. P., Zhang, T., Wang, W., Zhou, S. M., Yao, X. M., Cheng, J. H., Chang, W. W., Lv, K., Li, C. X. & Kong, X. (2023). Metformin alleviates glucolipotoxicity-induced pancreatic beta cell ferroptosis through regulation of the GPX4/ACSL4 axis. European Journal of Pharmacology, 956, 175967. https://doi.org/10.1016/j.ejphar.2023.175967
- Tang, C., Yu, Y. M., Qi, Q. L., Wu, X. D., Wang, J., & Tang, S. A. (2019). Steroidal saponins from the rhizome of Polygonatum sibiricum. Journal of Asian Natural Products Research, 21(3), 197–206. https://doi.org/10.1080/10286020.2018.1478815
- Tang, Z., Zhang, M., Gao, L., Bao, Y., Li, P., Wang, M., Shao, T., Wang, G., & Liu, C. (2023). Optimal extraction of polysaccharides from Stevia rebaudiana roots for protection against hydrogen peroxide-induced oxidative damage in RAW264.7 cells. Natural Product Research, 1–5. https://doi.org/10.1080/14786419.2023.22639052
- Tian, H., Liu, H., Song, W., Zhu, L., & Yin, X. (2021). Polysaccharide from Caulerpa lentillifera: Extraction optimization with response surface methodology, structure and antioxidant activities. Natural Product Research, 35(20), 3417–3425. https://doi.org/10.1080/14786419.2019.1700507
- Venkatesan, M., Arumugam, V., Pugalendi, R., Ramachandran, K., Sengodan, K., Vijayan, S. R., Sundaresan, U., Ramachandran, S., & Pugazhendhi, A. (2019). Antioxidant, anticoagulant and mosquitocidal properties of water soluble polysaccharides (WSPs) from Indian seaweeds. Process Biochemistry, 84, 196–204. https://doi.org/10.1016/j.procbio.2019.05.029
- Wang, Y., Li, Y., Li, T., Wei, Y., & Li, X. (2020). Optimization of impregnation conditions for furfurylated bamboo on response surface methodology. Journal of Biobased Materials and Bioenergy, 14(1), 50–57. https://doi.org/10.1166/jbmb.2020.1940
- Yao, H. R., Liu, J., Plumeri, D., Cao, Y. B., He, T., Lin, L., Li, Y., Jiang, Y. Y., Li, J., & Shang, J. (2011). Lipotoxicity in HepG2 cells triggered by free fatty acids. American Journal of Translational Research, 3(3), 284–291. https://www.ncbi.nlm.nih.gov/pubmed/21654881
- Zhou, Y., Liu, L., Xiang, R., Bu, X., Qin, G., Dai, J., Zhao, Z., Fang, X., Yang, S., Han, J., & Wang, G. (2023). Arctigenin mitigates insulin resistance by modulating the IRS2/GLUT4 pathway via TLR4 in type 2 diabetes mellitus mice. International Immunopharmacology, 114, 109529. https://doi.org/10.1016/j.intimp.2022.109529
- Zhu, Z.-Y., Liu, F., Gao, H., Sun, H., Meng, M., & Zhang, Y.-M. (2016). Synthesis, characterization and antioxidant activity of selenium polysaccharide from cordyceps militaris. International Journal of Biological Macromolecules, 93, 1090–1099. https://doi.org/10.1016/j.ijbiomac.2016.09.076