ABSTRACT
It aims to offer an alternative product to the food industry for the increasing demands of healthy nutrition with production of yogurt containing bee pollen. Probiotic yogurt prepared with different levels of bee pollen (0.5%, 1.5%, 3% and 6%) were evaluated for antimicrobial properties, viability of S. thermophilus, L. bulgaricus, L. acidophilus, and Bifidobacterium spp. during 14 days of refrigerated storage, and resistance to in vitro gastrointestinal conditions. Yogurt samples showed antimicrobial activity against L. monocytogenes, S. Typhimurium, E. coli, E. coli O157:H7, and S. aureus at high level of cell concentration (108 CFU/mL). The viability of S. thermophilus, L. bulgaricus, L. acidophilus, and Bifidobacterium spp. decreased in vitro gastrointestinal digestion model depending on bee pollen content and bacterial species as the application time increased. The simulated gastric and intestinal juice tolerance of bacteria was strain dependent and pH-dependent. The addition bee pollen enhanced the survival of L. acidophilus in both simulated juices.
1. Introduction
Functional foods are defined as food or food components that not only meet the body’s basic nutritional needs but also provide additional benefits for human physiology and metabolic functions, thus offering various advantages for disease prevention and maintaining a healthier lifestyle (Hacıoğlu & Kurt, Citation2012; Yılmaz, Citation2006). In the Turkish Food Law, functional foods are defined as “foods that have scientifically and clinically proven effects in addition to their nutritional effects and that can protect, improve health or reduce the risk of diseases, depending on one or more active components” (Soylu & Bayram, Citation2020).
Functional foods include various supplements such as probiotics, prebiotics, phytochemicals and omega-3 fatty acids, in addition to essential nutrients like vitamins, minerals, carbohydrates, proteins and fibres (Güneş et al., Citation2018). Two basic approaches are generally defined for the production of functional foods. The first one is functional products are obtained by the more or less natural presence of a functionally important component in the food. The second approach involves the production of functional products by externally adding components with functional properties to the food (Şimşekli & Doğan, Citation2015). Examples of functional foods today include dairy products, cereal products, meat products, beverages, confectionery, snacks, spreads and baby foods (Güneş et al., Citation2018). The functional products widely preferred are dairy products (Karaman Mutlu, Citation2019). The scientific support for the positive health effects of functional dairy products has increased consumer and producer interest in these products, leading to accelerated research and development activities and production in the food industry (Seçkin & Baladura, Citation2011; Sezen & Koçak, Citation2006).
According to the functional food market analysis, the size of the functional food market was evaluated to be $177,770 million in 2019. It is projected that the functional food market will reach $267,924.4 million by 2027, with a compound annual growth rate (CAGR) of 6.7% from 2021 to 2027. This was found to be $48,831 million for dairy products in 2019, and it is expected to reach $73,030 million by 2027, with an annual growth rate of 6.6%. Probiotics, on the other hand, accounted for approximately one-third of the global functional food market share in 2019 and is expected to maintain this share until 2027 (Anonymous, Citation2023a). According to another functional foods market report, whose forecast period is from 2022 to 2030, the market size value in 2022 is $304.2 billion and is expected to reach $586.1 billion in 2030 with an annual growth rate of 8.5%. The increased demand for functional foods due to their nutritional benefits has a link with consumer awareness of healthy diets globally. The segment of dairy products is estimated to grow at a CAGR of nearly 7.9% between 2022 and 2030. The dairy product had 38% of the market share with the highest value in 2022. Yogurt as a functional dairy product is one of the trending functional foods and is fortified with nutritional additives or probiotics by manufacturers. Yogurt is popular and probiotic yogurt has high consumption all over the world (Anonymous, Citation2023b; Ghaderi‐Ghahfarokhi et al., Citation2021; Molaee Parvarei et al., Citation2021). Probiotic food and beverage had over 60% market share in 2022. It is mentioned in the probiotics market report, whose forecast period is from 2023 to 2030, the market size value in 2022 is $77.12 billion and is expected to reach $220.14 billion in 2030 with an annual growth rate of 14% (Anonymous, Citation2023c).
In recent years, various functional foods supplemented with probiotic bacteria and/or bioactive compounds have been offered to consumers. Due to their beneficial effects on health, the increasing interest in functional yogurt products has led to the development of new products. Probiotics, one of the main components of functional foods, defined as “live microorganisms that, when administered in adequate amounts, confer a health benefit on the host” (Hill et al., Citation2014). Among fermented milk products, yogurt is the most popular one that supports the growth and vitality of probiotic organisms (Yilmaz-Ersan & Kurdal, Citation2014). The demand for probiotic products is increasing because of their antimicrobial properties, effects on bowel movements, reducing the risk of bacterial colon diseases, control of various infections, alleviating lactose intolerance, protecting and healing against various diseases, strengthening the immune system, reducing serum cholesterol levels, anticarcinogenic and antiallergic effects (Adolfsson et al., Citation2004; Fernandez et al., Citation2017; Gahruie et al., Citation2015; Kok & Hutkins, Citation2018; Makino et al., Citation2006; Michels et al., Citation2020).
Bee pollen, collected by bees from flowers and contains the saliva of worker bees and a small amount of plant nectar or honey, has the potential to be a good alternative for functional foods (Matuszewska et al., Citation2021). Bee pollen is an important place in human nutrition depending on its rich content of carbohydrates, proteins, enzymes, fatty acids, carotenoids, phenolic compounds, flavonoids, sterols, terpenes, minerals, vitamins, and free amino acid (Estevinho et al., Citation2012; Feás et al., Citation2012; Hani et al., Citation2012). Bee pollen is a popular apitherapeutic product due to its positive effects on health (Aylanc et al., Citation2022). The health benefits of bee pollen are antioxidative (associated with the activity of antioxidant enzymes as well as the content of phenolic substances, carotenoids, vitamins C and E) (Denisow & Denisow‐Pietrzyk, Citation2016), immunomodulatory (increasing the activity of humoral immune cells and phagocytes, increasing the number of red blood cells, accelerating the formation of antibodies, and delaying the destruction of antibodies) (Algethami et al., Citation2022), anti-inflammatory (inhibition of enzymes responsible for the development of the inflammatory process in tissues by flavonoids, phenolic acids, fatty acids, and phytosterols) (Komosinska-Vassev et al., Citation2015), cardioprotective (linked to the presence of essential unsaturated fatty acids, vitamin E, phytosterols, phospholipids, and flavonoids) (Rzepecka-Stojko et al., Citation2015), hepatoprotective (preventing lipid peroxidation and reducing body weight, AST and ALT enzyme levels, histological damage, and apoptosis) (Farag & El-Rayes, Citation2016; Yıldız et al., Citation2013), skin treatment (connection with its antioxidant capacity) (Sun et al., Citation2017), and diabetes (reduces blood sugar thanks to the phenols, flavonoids, and enzymes that hydrolyze carbohydrates such as amylase and glucosidase (Algethami et al., Citation2022).
Bee pollen has been used in different products such as pineapple juice-based beverage matrix (Zuluaga et al., Citation2016), kombucha (Uțoiu et al., Citation2018), cheese (Abbas et al., Citation2023; Abd Elhamid & Elbayoumi, Citation2017), acidophilus milk (Glušac et al., Citation2015), sausages (de Florio Almeida et al., Citation2017), bread (Conte et al., Citation2018), pudding (Anjos et al., Citation2019) and yogurt (Atallah, Citation2016; Karabagias et al., Citation2018; Özcan et al., Citation2020) for improving their functional properties. It has functional effects such as antimicrobial, antioxidant, anti-inflammatory, and anti-carcinogenic due to its bioactive component. Bee pollen has antibacterial, antifungal, and antiviral activities. Its antimicrobial properties are attributed to content of phenolic compounds, glucose oxidase, flavonoid, fatty acids, and seconder metabolites from plants (De Melo & de Almeida-Muradian, Citation2017; Eşerler et al., Citation2023; Thakur & Nanda, Citation2020). The current study aimed to provide the food industry with an alternative product to meet the increasing consumer demand for healthy nutrition by producing yogurt containing bee pollen, assessing its antimicrobial properties and revealing its functional properties such as viability in the gastrointestinal system. With these objectives in mind, by revealing the possibilities of using bee pollen in fermented dairy product yogurt, it was aimed to create an alternative in functional food production through the incorporation of bee pollen into probiotic yogurt before fermentation, thus to provide economic benefits to beekeeping industry and contribute to human health. In this context, the objectives of present study are i) the production of probiotic yogurts containing bee pollen, ii) the antimicrobial properties of the probiotic yogurts enriched with bee pollen and iii) determination of the viability of microorganisms in an in vitro gastrointestinal digestion model.
2. Materials and method
2.1. Food samples
In this study, commercially available 3% fat high temperature pasteurized milk (İçim, Ak Gıda, Sakarya, Turkey) was used for yogurt production. Bee pollen produced in the region was obtained from a local beekeeper who produces in Muğla province (Turkey). The utilized fresh bee pollen is a natural and completely unprocessed foodstuff. The bee pollen was harvested by the beekeeper and froze directly. The bee pollen, purchased in 3 kg for a whole analysis, was transported to the laboratory in an icebox while still in its original packaging. The bee pollen was kept at −18°C until analysis. The bee pollen, used in this study, is originating from a mixture of flowers likely willow, mustard, sweetgum, laurel-leaf cistus, common poppy, and pink rock rose according to information obtained from the beekeeper.
2.2. Commercial starter cultures
The probiotic yogurt starter culture, which obtained by drying the probiotic yogurt, was obtained from a local company (Yayla Maya, Maysa Gıda, Istanbul, Turkey) and was used for producing yogurt samples. The company has mentioned the diversity of microorganisms in the culture as Streptococcus thermophilus, Lactobacillus delbrueckii subsp. bulgaricus, Lactobacillus acidophilus and Bifidobacterium animalis spp. lactis (Anonymous, Citation2023d). The probiotic yogurt starter culture contains the yogurt bacteria of S. thermophilus and L. bulgaricus and the probiotic bacteria of L. acidophilus and Bifidobacterium animalis spp. lactis. The counts of microorganisms in the starter culture were determined. S. thermophilus, L. bulgaricus, L. acidophilus, and Bifidobacterium spp. were obtained as 9.04 ± .04, 8.91 ± .13, 8.42 ± .14, and 7.54 ± .18 log CFU/g in the starter culture, respectively.
2.3. Test cultures
As test bacteria, Listeria monocytogenes ATCC 35,152, Salmonella Typhimurium ATCC 14,028, Escherichia coli ATCC 25,922, E. coli O157:H7 ATCC 35,150 and Staphylococcus aureus ATCC 6538 were obtained from Mugla Sıtkı Koçman University (Scientific Research Laboratory), Niğde Ömer Halisdemir University (Engineering Faculty, Food Engineering Department) and Tokat Food Control Laboratory. All the cultures were grown in Brain Heart Infusion (BHI, Biolife 4,012,302, Italy) broth at 35 ± 2°C. Stock cultures were kept at −20°C in BHI broth containing 20% glycerol (Glycerin, Tekkim, TK.070190.02501, Turkey).
2.4. Production of probiotic yogurt containing bee pollen
Bee pollen to be used in production were treated with ultraviolet (UV) for 60 minutes and then was added at determined rates (0.5%, 1.5%, 3% and 6%) into the pasteurized milk. Heat treatment (5 minutes at 70°C) was applied to the milk samples with bee pollen for inhibition of the initial microbial load. After the heat treatment, the milk samples with or without bee pollen were cooled to 42°C and inoculated with 0.1% (1 g/1 L milk) of starter culture (Karabagias et al., Citation2018; Lomova et al., Citation2014; Özcan et al., Citation2020). The samples were portioned into sterile containers under aseptic conditions and incubated at 42°C (Daihan scientific, Thermo Stable IG-105, Korea). The pH was monitored throughout the incubation and when the pH reached 4.6, the incubation process was terminated and the samples were brought to 4°C for storage. Analyses were carried out by taking samples on the 1st, 7th and 14th days of the storage (Sert et al., Citation2011) ().
2.4.1. pH determination
Measurement of the pH value of the samples was performed using a pH meter (Mettler Toledo, Five easy plus FP20, China) calibrated with appropriate buffer solutions (pH 4.01, Mettler Toledo, 51 302 069, Switzerland; pH 7, Mettler Toledo 51,302,047, Switzerland). For the pH value measurement of the samples, the electrode of the device was immersed in the sample and the pH values were determined (AOAC, Citation1995).
2.5. The antimicrobial properties of probiotic yogurt produced with bee pollen
In order to determine the antimicrobial activity of probiotic yogurts containing bee pollen, analyses were carried out using the disk diffusion method on the 1st, 7th and 14th days of storage (CLSI M02, Citation2015; CLSI M45, Citation2016). Paper disks were placed into the yogurt samples and were kept there for 30 minutes. Then, the yogurt-soaked 6 mm diameter disks were placed onto the agar surfaces inoculated with indicator bacteria (approximately 108 CFU/mL) and incubated for 24 hours (CLSI M07-A8, Citation2009). The incubation temperature is 35 ± 2°C, which is the optimum growth temperature of the tested indicator bacteria. The zone of inhibition was measured. The pasteurized milk and bee pollen were used as negative control, separately. The yogurt sample without bee pollen was evaluated as positive control.
2.6. Viability of microorganisms in an in vitro gastrointestinal digestion model
In order to determine the probiotic properties of probiotic yogurts produced with bee pollen, an in vitro static model was used to simulate the gastrointestinal digestive system. This analysis was conducted on probiotic yogurt samples produced with bee pollen on the 1st, 7th and 14th days of storage at 4°C. To this end, simulated gastric and small intestinal juices were prepared fresh every day. Counting of L. bulgaricus, S. thermophilus, L. acidophilus and Bifidobacterium spp. was performed. Bee pollen and pasteurized milk samples were used as negative control, separately. The yogurt sample without bee pollen was taken as positive control.
2.6.1. Preparation of simulated gastric juice and small intestinal juices
For simulated gastric juice, pepsin (1:10,000 ICN) (P7000, Sigma Aldrich, Switzerland) was added to a 0.5% saline solution (NaCl, Tekkim, TK.17054001002, Turkey) with a final concentration of 3 g/L. The pH values of the solution prepared in this way were adjusted to pH 2, pH 3 and pH 4 with concentrated 1 mol/L and 0.1 mol/L hydrochloric acid (HCl 37%, Tekkim, TK.911011.01001, Turkey) or sterile 0.1 and 1 mol/L sodium hydroxide (NaOH, Tekkim, TK.170511.01002, Turkey) (Huang & Adams, Citation2004).
For simulated small intestinal juice, pancreatin USP (P1625, Sigma Aldrich, U.S.A.) was added to a 0.5% saline solution with a final concentration of 1 g/L. The pH values of the solution prepared in this way were adjusted to pH 8 with sterile 0.1, 0.05 and 0.01 mol/L NaOH (Charteris et al., Citation1998). This solution was also prepared to contain additional 0.3% bile salts (Ox-Bile, Fluka 70,168, Italy) (Ranadheera et al., Citation2012).
2.6.2. Determination of viability of probiotic yogurt starter cultures in gastric juice
A sample of 1 g yogurt was taken and put into 9 mL of simulated gastric juice. This process was repeated for each gastric juice adjusted to pH 2, pH 3 and pH 4. Mixing was applied at the highest speed for 10 seconds and incubated at 37°C. Dilutions were prepared by taking samples at the 1st, 30th, 60th and 180th minutes of the incubation. The enumeration of L. bulgaricus, S. thermophilus, L. acidophilus, and Bifidobacterium spp. from dilutions was performed (Ranadheera et al., Citation2012).
2.6.3. Determination of viability of probiotic yogurt starter cultures in small intestinal juice
A sample of 1 g yogurt was taken and placed in 9 mL of simulated small intestinal juice. Mixing was applied at the highest speed for 10 seconds and incubated at 37°C. Dilutions were prepared by taking samples at the 1st, 120th and 240th minutes of the incubation. The enumeration of L. bulgaricus, S. thermophilus, L. acidophilus, and Bifidobacterium spp. from dilutions was performed (Ranadheera et al., Citation2012).
2.6.4. Preparation of samples for microbiological analysis
Under aseptic conditions, 25 g of yogurt sample was transferred to a stomacher bag and homogenized for 1 minute in a stomacher device (Isolab, Laborgerate GmbH, Germany) with 225 mL of 0.1% peptone water (Oxoid, LP0037, UK). Decimal dilutions were prepared using 0.1% peptone water from the prepared homogenate (ISO 6887–1, Citation2017). These prepared dilutions were used for microbiological analyses.
2.6.5. The count of L. bulgaricus
Dilutions prepared for counting L. bulgaricus from yogurt bacteria were inoculated onto de Man, Rogosa and Sharpe (MRS, Biokar, BK089HA, France) agar with the spread plate method (0.1 mL). Petri dishes were incubated at 37°C for 72 hours in anaerobic conditions produced by combustion (Daihan scientific, Thermo Stable IG-105, Korea) (TS ISO 7889, Citation2004).
2.6.6. The count of S. thermophilus
M-17 (M-17, Biokar, BK088HA, France) agar was used for counting S. thermophilus in yogurt samples. The inoculations were performed using the spread plate method (0.1 mL), followed by incubation at 37°C for 48 hours (TS ISO 7889, Citation2004).
2.6.7. The count of L. acidophilus
Bile-MRS agar was used for counting L. acidophilus in yogurt samples. Bile-MRS agar was prepared by adding 1.5 g/1 L bile (Ox-Bile, Fluka 70,168, Italy) to MRS agar medium. After inoculation using the spread plate method (0.1 mL), the petri dishes were incubated at 37°C for 72 hours (Vinderola & Reinheimer, Citation1999).
2.6.8. The count of Bifidobacterium spp.
LP-MRS agar medium was used for counting Bifidobacterium spp. in yogurt samples. LP-MRS agar was prepared by adding lithium chloride (LiCl) (Sigma 413,271,000, UK) and sodium propionate (C3H5NaO2) (Sigma 149,010,250, Netherlands) to the MRS agar. 2 g of LiCl and 3 g of C3H5NaO2 were added to 1 litre of MRS agar. After inoculations were performed using the spread plate method (0.1 mL), they were incubated at 37°C under anaerobic conditions using the anaerobic Gas Pack for 72 hours (Lapierre et al., Citation1992; Vinderola & Reinheimer, Citation1999).
2.7. Statistical analysis
In the study, each analysis was conducted in a 2 × 2 design, with two replicates and two parallels for each. In the current study, microbiological analysis results were presented as log CFU/g, and the detection limit was chosen as 10 CFU/g (<1.00 log CFU/g). Duncan test was used to compare the means and data analyses were performed using SPSS version 17 (17..3, 2010, SPSS Inc., Chicago, U.S.A.) statistical package program with 95% confidence interval.
3. Results
3.1. Antimicrobial properties of probiotic yogurt made with bee pollen
Antimicrobial activities of yogurt samples containing different proportions of bee pollen were tested against the L. monocytogenes ATCC 35,152, S. Typhimurium ATCC 14,028, E. coli ATCC 25,922, E. coli O157:H7 ATCC 35,150 and S. aureus ATCC 6538 pathogens by using the disc diffusion method on the 1st, 7th and 14th days of storage (). The statistical data are given in supplementary materials (Table S1). The pasteurized milk as a negative control did not have any inhibitory effect against target microorganisms. However, the pasteurized milk with bee pollen at the rate of 6% was only effective on E. coli (7 mm), S. aureus (8 mm), and S. Typhimurium (7 mm).
Figure 2. Antimicrobial activity of yogurt samples (inhibition zones mm). n = 4, ┬ ┴ standard deviations. Control: probiotic yogurt without bee pollen, 0.5%: probiotic yogurt containing 0.5% bee pollen, 1.5%: probiotic yogurt containing 1.5% bee pollen, 3%: probiotic yogurt containing 3% bee pollen, 6%: probiotic yogurt containing 6% bee pollen.
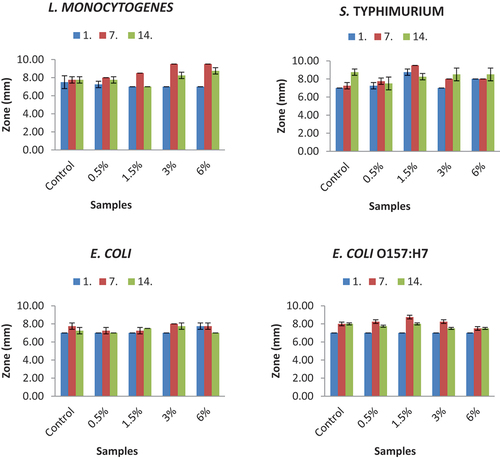
The inoculation level of the indicator bacteria L. monocytogenes is between 8.69 and 8.83 log CFU/mL. The maximum disc diameter was determined on the 7th day for all the samples. On the 7th day, the disc area, which was 7.75 mm in the control sample, increased as the amount of bee pollen increased and it was measured as 9.50 mm in the sample containing 3% and 6% bee pollen.
The inoculation level of S. Typhimurium indicator bacteria is between 8.55 and 8.88 log CFU/mL. In the control sample, the disc diameter increased with storage. The largest disc diameters were determined as 7.75 mm and 9.50 mm on the 7th day in the samples containing 0.5% and 1.5% bee pollen and 8.50 mm on the 14th day in the samples containing 3% and 6% bee pollen.
The inoculation level of E. coli indicator bacteria is between 8.82 and 8.87 log CFU/mL. The smallest disc diameter was found to be 7.00 mm and the largest was found to be 8.00 mm. In general, it is seen that the disc diameters of the samples decreased during storage.
The inoculation level of E. coli O157:H7 indicator bacteria is between 8.53 and 8.94 log CFU/mL. Disc diameters vary between 7.00 and 8.75 mm. The greatest values were found on the 7th day of storage.
The inoculation level of S. aureus indicator bacteria is between 8.43 and 8.78 log CFU/mL. The largest disc diameters were calculated as 9.00 mm in the sample containing 6% bee pollen on the 1st and 7th days.
3.2. Viability of microorganisms in an in vitro gastrointestinal digestion model
Yogurt samples containing different proportions of bee pollen were analyzed in an in vitro static model using simulated gastric and small intestinal juices on certain days of storage (1st, 7th and 14th days). The count of bee pollen and pasteurized milk samples individually as negative controls were found below the detectable values for all storage days and all microorganisms at all simulated juices.
3.2.1. Viability of probiotic yogurt starter cultures in gastric juice
The numbers of viable bacteria in simulated gastric juice adjusted to pH 2 are presented in . The statistical data are given in supplementary materials (Table S2). L. bulgaricus values decreased with increasing time in all the samples. The lowest viable bacteria counts were obtained at 180 minutes on storage days. S. thermophilus values showed a decrease with application time and bee pollen amount. While it was between 8.11 and 8.26 log CFU/g in the 1st minute at the beginning of the storage, it was determined to be between 7.74 and 8.10 log CFU/g at the end of the storage period. It was observed that the L. acidophilus values were directly proportional to the bee pollen ratio and inversely proportional to the application time. L. acidophilus was found to be below the detectable limit in the control and 0.5% bee pollen-containing samples on the last day of storage. Bifidobacterium spp. values were found to be below the detectable values in all the samples on the 7th and 14th days at all the application periods. At the beginning of the storage, a count was made between 3.62 and 5.72 log CFU/g in the 1st minute, and <1.00 log CFU/g was determined in the 180th minute.
Figure 3. The viability of yogurt starter culture in simulated gastric juice adjusted to pH 2 (log CFU/g). n = 4, ┬ ┴ standard deviations. Control: probiotic yogurt without bee pollen, 0.5%: probiotic yogurt containing 0.5% bee pollen, 1.5%: probiotic yogurt containing 1.5% bee pollen, 3%: probiotic yogurt containing 3% bee pollen, 6%: probiotic yogurt containing 6% bee pollen.
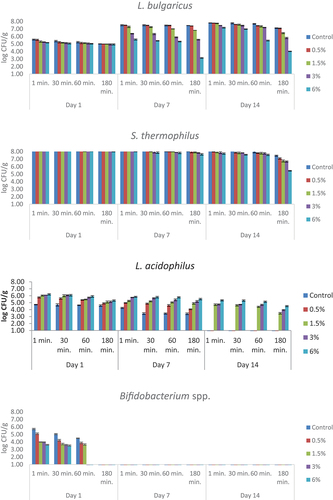
The numbers of viable bacteria in simulated gastric juice adjusted to pH 3 are presented in . The statistical data are given in supplementary materials (Table S3). L. bulgaricus values decreased inversely with bee pollen amount and application time. On the first day of storage, the highest value was determined as 8.17 log CFU/g in the control sample and the lowest value as 7.57 log CFU/g in the yogurt sample containing 6% bee pollen. The lowest count results in S. thermophilus values were found in yogurt samples containing 6% bee pollen on the 1st, 7th and 14th days of the analysis. However, the lowest count was found to be 6.93 log CFU/g in the 180th minute on the 14th day. The highest count result of L. acidophilus was found in the sample containing 6% bee pollen in the 1st minute application at the beginning of storage (6.39 log CFU/g). As the residence time in gastric juice increased, the number of viable bacteria decreased. Bifidobacterium spp. counts decreased as the amount of bee pollen increased and the application time increased.
Figure 4. The viability of yogurt starter culture in simulated gastric juice adjusted to pH 3 (log CFU/g). n = 4, ┬ ┴ standard deviations. Control: probiotic yogurt without bee pollen, 0.5%: probiotic yogurt containing 0.5% bee pollen, 1.5%: probiotic yogurt containing 1.5% bee pollen, 3%: probiotic yogurt containing 3% bee pollen, 6%: probiotic yogurt containing 6% bee pollen.
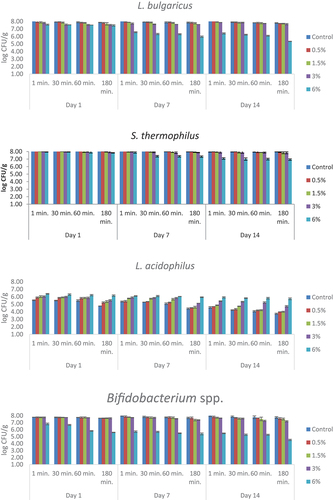
The numbers of viable bacteria in simulated gastric juice adjusted to pH 4 are presented in . The statistical data are given in supplementary materials (Table S4). L. bulgaricus values were found to be between 6.79 and 8.10 log CFU/g at the end of the 180 minutes application period at the beginning of the storage, and between 5.49 and 7.89 log CFU/g in the same application period at the end of the storage. S. thermophilus values in the control sample were found to be 8.24 log CFU/g in the 1st minute and 8.17 log CFU/g in the 180th minute on the 1st day and in the sample having the highest amount of pollen, it was found to be 7.80 log CFU/g in the 1st minute, 7.60 log in the 180th minute of the same day. While the number of L. acidophilus was below the detectable level in the control sample in the 60th and 180th minute of the application on the 14th day of storage, it was 5.32 log CFU/g and 4.83 log CFU/g, respectively, in the sample containing 6% bee pollen. Bifidobacterium spp. counts were found to be the lowest at the end of the 180-minute application at the end of the storage as 4.49 log CFU/g in the sample containing the most bee pollen.
Figure 5. The viability of yogurt starter culture in simulated gastric juice adjusted to pH 4 (log CFU/g). n = 4, ┬ ┴ standard deviations. Control: probiotic yogurt without bee pollen, 0.5%: probiotic yogurt containing 0.5% bee pollen, 1.5%: probiotic yogurt containing 1.5% bee pollen, 3%: probiotic yogurt containing 3% bee pollen, 6%: probiotic yogurt containing 6% bee pollen.
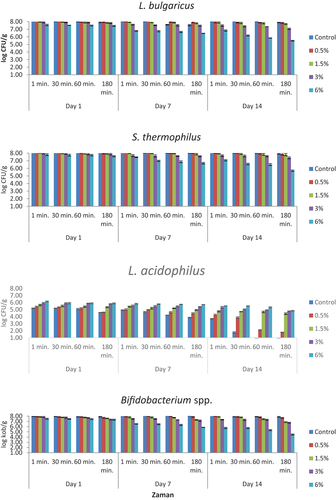
As the pH value decreased in simulated gastric juices, the number of viable bacteria decreased (L. bulgaricus, S. thermophilus, L. acidophilus and Bifidobacterium spp.). As the bee pollen ratio increased at the same pH value, L. bulgaricus, S. thermophilus, and Bifidobacterium spp. counts decreased, while the number of L. acidophilus increased. At the same pH value, the viability count results of all the tested bacteria decreased as the application time increased in the same sample.
3.3. Viability of probiotic yogurt starter cultures in small intestinal juice
The numbers of viable bacteria in simulated small intestinal juices that contained bile salts and whose pH value was adjusted to 8 are presented in . The statistical data are given in supplementary materials (Table S5). L. bulgaricus values were found to be <1.00 log CFU/g on the 7th day of storage after the 240th minute in the control and 0.5% bee pollen samples, after the 120th minute in the sample containing 1.5% bee pollen and after the 1st minute in the samples containing 3% and 6% bee pollen, and in the control and samples containing 0.5% and 1.5% bee pollen on the 14th day of storage except for the 1st minute. The number of S. thermophilus in the control sample at the beginning of storage in the 1st minute was found as 7.94 log CFU/g, and 4.29 log CFU/g in the 180th minute. In the yogurt samples containing bee pollen, the number of viable bacteria on the same day ranged from 3.58 log CFU/g to 7.10 log CFU/g, while in the samples containing 3% and 6% bee pollen on the 7th day, in the 120th and 240th minutes, in the 240th minute at the end of storage, it was found to be <1.00 log CFU/g. While the number of L. acidophilus was between <1.00 log CFU/g and 5.95 log CFU/g in the yogurt samples including the control on the 1st day, it was below the detectable limit at the end of the 14th day, excluding the 1st minute of yogurts containing 1.5%, 3% and 6% bee pollen. Bifidobacterium spp. cells were inhibited in 240 minutes on the 1st day, 120th and 240th minutes on the 7th day and all the applications on the 14th day of storage.
Figure 6. The viability of yogurt starter culture in simulated small intestinal juices that contained bile salts (log CFU/g). n = 4, ┬ ┴ standard deviations. Control: probiotic yogurt without bee pollen, 0.5%: probiotic yogurt containing 0.5% bee pollen, 1.5%: probiotic yogurt containing 1.5% bee pollen, 3%: probiotic yogurt containing 3% bee pollen, 6%: probiotic yogurt containing 6% bee pollen.
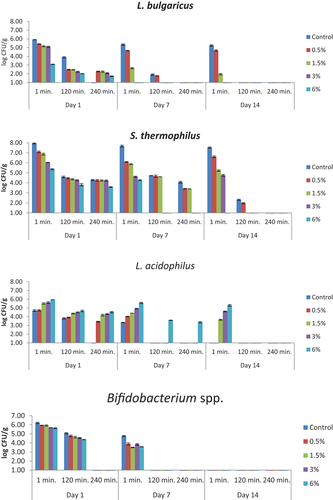
The numbers of viable bacteria in simulated small intestinal juices whose pH values was adjusted to 8 and which were free of bile salts are presented in . The statistical data are given in supplementary materials (Table S6). L. bulgaricus values were determined below detectable levels in samples containing 6% bee pollen on the 7th and 14th days of storage. The number of S. thermophilus decreased with the amount of bee pollen added and the application time. The number of L. acidophilus increased with the increasing bee pollen ratio. At the end of storage, 3.48 log CFU/g was counted after 120 minutes of application in the sample containing 6% bee pollen. Bifidobacterium spp. cells were found to be 4.82–6.46 log CFU/g in yogurt samples containing bee pollen at the beginning, and <1.00 log CFU/g after 240 minutes of application.
Figure 7. The viability of yogurt starter culture in simulated small intestinal juices which were free of bile salts (log CFU/g). n = 4, ┬ ┴ standard deviations. Control: probiotic yogurt without bee pollen, 0.5%: probiotic yogurt containing 0.5% bee pollen, 3%: probiotic yogurt containing 3% bee pollen, 6%: probiotic yogurt containing 6% bee pollen.
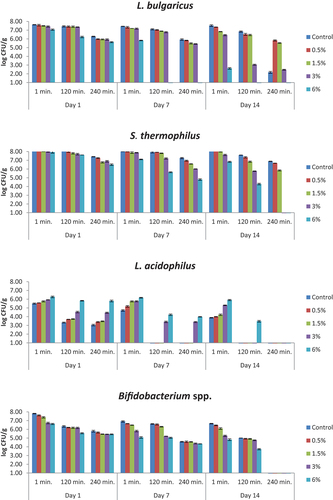
The number of viable bacteria decreased in simulated small intestinal juices containing bile salts (L. bulgaricus, S. thermophilus, L. acidophilus and Bifidobacterium spp.). As the amount of bee pollen increased in yogurts, the numbers of L. bulgaricus, S. thermophilus and Bifidobacterium spp. decreased, and the number of L. acidophilus increased in simulated intestinal juices that did not contain bile salt and that contained bile salt. In both simulated small intestinal juices, the viable count results of all the tested bacteria decreased as the application time increased in the same sample.
4. Discussion
4.1. Antimicrobial properties of probiotic yogurt made with bee pollen
Yogurt samples containing bee pollen, including the control, showed antimicrobial activity against L. monocytogenes, S. Typhimurium, E. coli, E. coli O157:H7 and S. aureus as test bacteria. The largest disc area for E. coli was measured on the 7th day in the yogurt containing 3% bee pollen, and there was no significant difference in disc sizes between yogurts (p > 0.05). For E. coli O157:H7, yogurt samples showed the greatest antimicrobial effect on the 7th day of storage as 8.75 mm. This value is similar to other results obtained on the same day (except 6% bee pollen yogurt) (p > 0.05). As with E. coli and E. coli O157:H7, the highest inhibitory effect was 9.50 mm, 9.00 mm and 9.50 mm for L. monocytogenes, S. aureus and S. Typhimurium on the 7th day of storage, respectively. Addition of pollen on day 7 for E. coli, day 1 for E. coli O157:H7 and L. monocytogenes, day 14 for S. aureus and S. Typhimurium did not show any effect on test pathogens (p > 0.05).
The antimicrobial activity of the yogurt samples was tested on a total of five microorganisms. Generally, bee pollen addition had a limited effect on E. coli, E. coli O157:H7, and L. monocytogenes, while addition of bee pollen at a dose of 6% had inhibitory effect on S. aureus and S. Typhimurium when compared with control. Therefore, antimicrobial activity may be improved by increasing the concentration of bee pollen. Yogurt samples with bee pollen at the rate of 3% and %6 had slightly more inhibitory effect than the others. The higher inhibitory effect of these samples is thought to be due to the fact that include higher amount of bee pollen. The antibacterial activity of pollen could be attributed mainly to the content of flavonoids, protein, glucose oxidase, and non volatile compounds (De Melo & de Almeida-Muradian, Citation2017; Mohdaly et al., Citation2015). On the other way, the presence of yogurt starter and probiotics in yogurt samples may influence the antimicrobial activity against tested pathogens. The microbial populations of yogurt are capable of producing a wide range of antimicrobial compounds, including organic acids, carbon dioxide, hydrogen peroxide, ethanol, acetaldehyde, diacetyl, fatty acids, enzymes, and peptides (bacteriocins) (Markowiak & Śliżewska, Citation2017; Mirzaei et al., Citation2018; Peng et al., Citation2021). These compounds interact with each other to enhance their antimicrobial effects. Both the microbial content of the yogurt and the composition of the pollen have enriched the functionality of the yogurt produced in this study despite the high concentration of the test pathogens (108 CFU/mL).
The antimicrobial activities of fermented milk drinks containing 2.5–20 mg/mL bee pollen were tested on 9 different microorganisms. While there was no inhibitory effect against Clostridium jejuni and Bacillus cereus, a zone between 9 and 14 mm was measured for S. Typhimurium and E. coli (Yerlikaya, Citation2014). As it is known, antimicrobial activity can also express with different methods. Assessment of the antibacterial activity of yogurt made by cow, camel, ewe, and goat milk on S. aureus, E. coli, S. Enterica, and L. monocytogenes was measured using an agar diffusion technique. The inhibitory zones were 17.9–37.7 mm for S. aureus, 12.4–28.2 mm for E. coli, 10.3–39.00 mm for S. Enterica, and 15.0–28.9 mm for L. monocytogenes (Azizkhani et al., Citation2021). Mohsin et al. (Citation2022) were conducted to elucidate the antimicrobial effect of yogurt samples prepared using buffalo milk on some bacteria, including S. aureus, E. coli O157, and Salmonella. These results showed a similar antimicrobial affect which in turn 7.000 mm, 8.667 mm, and 11.667 mm, if compared with the results of this study. Another research was performed to determine the inhibitory effect of non-industrial yogurts (30 samples) against Shigella sonnei, S. flexneri, S. boydii, and S. Enteritidis. 20% of the yogurt samples did not have any antimicrobial effects for target strains (Namaei et al., Citation2015). Similar results were reported by other researchers. These studies were done with several microorganisms comprising B. cereus, Campylobacter jejuni, Candida albicans, B. subtilis, Enterobacter aerogenes, E. coli, L. monocytogenes, S. Typhimurium, Pseudomonas aeruginosa, and S. aureus. Yerlikaya et al. (Citation2021) reported the antimicrobial activity of L. bulgaricus and S. thermophilus strains against Gram-positive and Gram-negative bacteria.
The reasons for the different results obtained in antimicrobial activity studies with yogurt are thought to be due to the use of starter culture or previously made yogurt in its production, the differences in the microbial composition of starter culture, constituents of bee pollen, and the different target species or strains of target bacteria used in the antimicrobial activity test. The type of milk, source of bee pollen, method of analysis, and concentration of test pathogen also affect the antibacterial activity of yogurt samples (Azizkhani et al., Citation2021; Mohdaly et al., Citation2015; Yerlikaya, Citation2014).
Microorganisms affect many aspects of human health and physiology. Yogurt samples produced with bee pollen in this study contain a high number of live microorganisms including probiotics. These microorganisms adhere to endothelial cells, to prevent the access of pathogens to the intestinal epithelium, generating antimicrobial compounds, and releasing proteolytic enzymes (Gahruie et al., Citation2015; Michels et al., Citation2020). Bee pollen has demonstrated numerous health-promoting actions including antimicrobial properties (Karabagias et al., Citation2018). Bee pollen contains compounds that inhibit the growth of Gram-positive and Gram-negative bacteria by deactivating enzymes, promoting alterations in the permeability of membranes, and loss of cellular material, by deactivating or destroying genetic material of bacterial cells (De Melo & de Almeida-Muradian, Citation2017). So, probiotic yogurt supplemented with various amounts of bee pollen inhibits pathogenic microorganisms and its consumption might alter the gut microbiota. As a result, beneficial microorganisms increase with numerous health benefits such as improving the intestinal microbiota balance through antimicrobial effects, reduction and prevention of diarrhea, decreasing lactose intolerance symptoms and food allergy, improving immune potency, and antitumorigenic activities (Azizkhani & Tooryan, Citation2016).
4.2. Viability of microorganisms in an in vitro gastrointestinal digestion model
In this section, where the effects of different pH and different conditions of the gastrointestinal tract, such as bile salt, on the viability of bacteria in yogurt enriched with bee pollen were tested, in general, as the pH value decreased in the simulating gastric juices, bacterial viability was adversely affected in the presence of bile in the small intestinal juice. In both environments, as the application time increased, bacterial viability decreased, although it differed depending on bee pollen content and bacterial species. S. thermophilus, L. bulgaricus and L. acidophilus cell viability in fermented beverages obtained from cow’s milk were analyzed in three phases: stomach, small intestine and large intestine. Bacterial viability, which was initially 8–9 log CFU/mL, was greatly reduced in the gastric phase (Da Silva et al., Citation2020). S. thermophilus bacteria are with the highest number of viable organisms in simulated gastric juice at three different pH conditions (P< 0.05). In simulated small intestinal juice containing bile, bile caused a reduction in viable cell counts of all the bacteria in all the samples. Bile disrupts cellular homeostasis by affecting the phospholipids and proteins of bacterial cell membranes (Bedani et al., Citation2013). S. thermophilus has more viable cells than other bacteria in the presence of bile, which is in compliance with the study by Ziar et al. (Citation2014).
Apple, banana and grape flours were added to 1% fermented milk beverage and stored for 28 days. The viability of probiotic bacteria in simulated gastric (pH 2.0–2.2) and intestinal juice (pH 4.3–5.2 and 7.0–7.3) was analyzed. It was found that all probiotic bacteria decreased in the in vitro gastrointestinal tract. On the other hand, it was observed that the added flours increased the resistance of L. acidophilus bacteria to gastrointestinal conditions (Casarotti & Penna, Citation2015). A similar relationship was also found in the current study. Addition of bee pollen increased the tolerance of L. acidophilus probiotic bacteria.
Ice cream, plain and fruit yogurt were produced from goat milk and the viability of probiotic bacteria (L. acidophilus LA-5, B. lactis BB-12 and Propionibacterium jensenii 702) were tested. From the simulated gastric juices, pH 2 provided a significant decrease on the viability of probiotic bacteria compared to pH 3 and pH 4 (Ranadheera et al., Citation2012). This situation, which is similar to the results of the current study, may also be due to the internal properties of probiotic bacteria, as well as the fact that the optimum activity of pepsin enzyme in simulated gastric juice is between pH 1.5 and 2.0, affecting the viability of probiotic cells (Vinderola et al., Citation2011). While the number of Bifidobacterium lactis in plain yogurt and fruit yogurt (containing 10% fruit juice) was 7.58 log CFU/g and 7.78 log CFU/g in the 1st minute, respectively, at pH 2, it fell below the detectable limit in plain yogurt in the 30th minute of the application and fruity yogurt in the 60th minute of the application (Ranadheera et al., Citation2012). In the current study, at the same pH value, Bifidobacterium spp. count was found to be <1.00 log CFU/g in the 180th minute of application in the control yogurt and the yogurt containing 0.5% and 1.5% bee pollen, and at 60th minute in the yogurts containing 3% and 6% bee pollen.
While there was no bile salt in the simulated intestinal juice, L. acidophilus was found as 7.67 log CFU/g and 7.58 log CFU/g in plain and fruity yogurt in the 1st minute of the application and as 6.80 log CFU/g and 7.34 log CFU/g in the 240th minute, in the presence of bile salt, it was found to be 6.28 log CFU/g and 7.14 log CFU/g in plain and fruit yogurt in the 1st minute, and 3.80 log CFU/g and 3.07 log CFU/g in the 240th minute (Ranadheera et al., Citation2012). Bile salts are natural detergents that facilitate digestion and absorption of the hydrophobic components of the diet. Their antimicrobial effects arise from these properties. With their amphiphilic structure, they damage the cell membranes of bacteria and prevent their survival in the gastrointestinal tract (Madureira et al., Citation2011).
The viability of 13 species of propionic acid bacteria was tested in simulated gastric juice with different pH values in the gastrointestinal tract and small intestinal juice with and without bile salt. pH 2 conditions showed a stronger inhibitory effect on each bacterial species analyzed than pH 3 and pH 4 conditions. As the pH value increased and the acidity decreased, the number of viable cells of the bacteria increased. The bacterial strain tested in simulated small intestinal juice free of bile salts remained viable in similar numbers after 240 minutes of administration. No decrease was observed in the simulated fluid containing bile salt, except for two species (Huang & Adams, Citation2004).
Chickpea flour was added (0–5%) to probiotic yogurts (L. acidophilus LA5 and Bifidobacterium BB12) and stored at 4°C for 5 weeks. The number of viable cells of both probiotic bacteria in simulated gastric juice at pH 2 decreased as the application time increased. L. acidophilus LA5 was reported to tolerate simulated gastric juice better than Bifidobacterium BB12 in all the yogurt samples. The control sample without chickpea flour showed protection for probiotic bacteria, as in the results of the current study. L. acidophilus in simulated small intestinal juice had higher viable cell counts in all the yogurts, similar to the results of the current study. This can be explained by the fact that L. acidophilus better tolerates acidic conditions, which is strain specific (Kaur Sidhu et al., Citation2020).
L. acidophilus DSM 20.079 and L. acidophilus NCFM bacteria were tested against stress conditions in a dynamic gastrointestinal model in ice cream, yogurt, white brine cheese and acidophilus milk samples. The highest number of viable cells in the gastrointestinal tract was found in the yogurt sample. Viable L. acidophilus counts in all the samples decreased with increasing time, which is consistent with the results of the current study. Mouth, stomach and small intestine were modelled in the tract. In the yogurt sample, while passing through the system L. acidophilus NCFM was found to be 8.66 log CFU/g in the mouth, 8.60 log CFU/g in the stomach in 1 hour and 7.17 log CFU/g at the end of 2 hours. It was found to be 6.61 log CFU/g in 1 hour and 6.44 log CFU/g at the end of 2 hours in the small intestine. Similarly, L. acidophilus DSM 20.079 was found to be 8.64 log log CFU/g, 8.59 log CFU/g, 7.26 log CFU/g, 6.71 log CFU/g and 6.53 log CFU/g. Bacteria type and strain are effective on the survival of probiotics, and the type of food they are in is also important. Yogurt has a nature that negatively affects the viability of probiotic bacteria due to the acidity formed during storage and the low pH values caused by it (Gocer et al., Citation2021).
The bee pollen addition generally did not have much effect on the viability of L. bulgaricus and S. thermophilus which could be related to better consumption of lactose as a carbon source by yogurt starter (Glušac et al., Citation2015). However, the viability of Bifidobacterium spp. was affected negatively probably due to the organic acid and phenolic constitution of bee pollen (Karabagias et al., Citation2018). The level of dissolved oxygen in yogurt and acidity can reduce the viability of Bifidobacterium spp (Atallah, Citation2016; Kailasapathy & Rybka, Citation1997). Adversely, the viability of L. acidophilus has increased with increasing bee pollen addition. L. acidophilus can survive better in acidic conditions because of its ability to resist cytoplasmic pH due to its high cytoplasmic buffering capacity and membrane H+ conductance (Kailasapathy & Rybka, Citation1997). The bee pollen has 10–40% protein (Ares et al., Citation2018). The denaturation and release of the peptides from milk and bee pollen during fermentation help the growth and survival of L. acidophilus, due to its lack of a good proteolytic system for hydrolyzing proteins (Glušac et al., Citation2015).
Pepsin is a digestive enzyme that breaks down proteins into smaller peptides. The optimum pH value of pepsin activity is between 1.5 and 2.5 (Del Rio et al., Citation2021). So, as the pH value decreased in simulated gastric juices, the number of viable bacteria decreased. The low pH value of gastric juice and the presence of pepsin break the cell membrane and the cell wall of the bacteria; influencing the membrane pathway, leading to undesirable metabolic processes, energy depletion, and finally cell death (Da Silva et al., Citation2020). Microorganisms survive in these conditions by some mechanisms such as producing alkaline compounds, ingestion of protons inside cells, etc (Ayyash et al., Citation2021).
Microorganisms encounter bile salts and pancreatin in simulated intestine juice. Pancreatin is a combination of digestive enzymes (e.g. amylase, lipase, and protease), and is crucial for digesting fats, proteins, and sugars. Bile salts may have affected bacterial phospholipids and membrane proteins, impairing cell homeostasis (Megur et al., Citation2023). Ziar et al. (Citation2014) investigated whether the bile survival of the different strains (B. lactis L. rhamnosus L. bulgaricus, and S. thermophilus) could be influenced by the addition of carbohydrates as the sole carbon source. The different levels of bile tolerance were found to be influenced by the type of carbon source used. The sugar component of the bee pollen used in this study may impress the sensitivity of the starter culture or probiotic microorganism to bile salts.
There was a decrease in the population of the tested bacteria over storage time, cause at the end of the cold storage bacterial cells were damaged and stressed compared to the beginning. All of the bee pollen concentration had a positive effect on the survival of L. acidophilus compared with that of the control treatment. The microenvironments produced by the food matrix or ingredient in the intestine may protect L. acidophilus from the harsh conditions present in the simulated gastrointestinal digestion model. Moreover, components of bee pollen could bind to bile acids, reducing their toxic effect on cells (Casarotti & Penna, Citation2015).
5. Conclusion
As a result, a functional product enriched with bee pollen obtained from the region was produced with a local starter culture. Probiotic yogurt cultures were developed in milk containing bee pollen at different rates throughout the incubation period, and set type yogurt was produced. It was determined that bee pollen did not prevent the growth and reproduction of bacteria in the culture. Yogurts containing bee pollen, including the control sample, had inhibitory activity against the tested pathogenic bacteria including two Gram positive and three Gram negative. The bacteria in yogurt samples were tested against different enzymes (pepsin and pancreatin), acidic environments, and bile salts in vitro static gastrointestinal model. The viability of bacteria was affected by the increase in acidity and the presence of bile. Bee pollen rate, application time and storage time were other factors that affected the number of viable cells. Bee pollen addition has positive effect on survival of L. acidophilus in gastrointestinal digestion model. When examined in general, it is seen that the sample containing 0.5% bee pollen statistically has the closest values to the control sample. The yogurts produced within the scope of this project are in compliance with the Turkish Food Codex Communiqué on Fermented Dairy Products. The developed functional product has the potential to contribute to the development and improvement of health and the prevention of disease risks. In this context, the investigation of organoleptic properties may be the subject of future studies. Particularly, sensory research of products is required to evaluate the perception of consumers. Furthermore, consumer attitudes should be carefully assessed for commercialization in the food industry. It is also important to investigate the physicochemical properties of bee pollen and probiotic yogurt with bee pollen to understand the effect of food ingredients on the survival of tested bacteria in further studies. In the case of the development of a novel formulation for functional food enriched with honeybee pollen, it should be considered that the use of bee pollen must be mentioned on the label to prevent allergic risks.
Supplemental Material
Download MS Word (63.4 KB)Disclosure statement
No potential conflict of interest was reported by the author(s).
Supplementary material
Supplemental data for this article can be accessed online at https://doi.org/10.1080/19476337.2024.2319834.
Additional information
Funding
References
- Abbas, K. A., Othman, F. A., Deghedie, M. A., & Abd Elmontaleb, H. S. (2023). The impact of bee pollen addition on the quality characteristics of probiotic UF-soft cheese. Egyptian Journal of Food Science, 51(1), 33–16. https://doi.org/10.21608/EJFS.2023.174240.1146
- Abd Elhamid, A. M., & Elbayoumi, M. M. (2017). Influence of bee pollen on the bioactive behavior, sensory and physicochemical properties of white cheese made from camel and cow milk mixture. Journal of Food and Dairy Sciences, 8(11), 419–424. https://doi.org/10.21608/jfds.2017.38933
- Adolfsson, O., Meydani, S. N., & Russell, R. M. (2004). Yogurt and gut function. The American Journal of Clinical Nutrition, 80(2), 245–256. https://doi.org/10.1093/ajcn/80.2.245
- Algethami, J. S., El-Wahed, A. A. A., Elashal, M. H., Ahmed, H. R., Elshafiey, E. H., Omar, E. M., Naggar, Y. A., Algethami, A. F., Shou, Q., Alsharif, S. M., Xu, B., Shehata, A. A., Guo, Z., Khalifa, S. A. M., Wang, K., & El-Seedi, H. R. (2022). Bee pollen: Clinical trials and patent applications. Nutrients, 14(14), 2858: 1–26. https://doi.org/10.3390/nu14142858
- Anjos, O., Fernandes, R., Cardoso, S. M., Delgado, T., Farinha, N., Paula, V., Estevinho, L. M., & Carpes, S. T. (2019). Bee pollen as a natural antioxidant source to prevent lipid oxidation in black pudding. LWT - Food Science and Technology, 111, 869–875. https://doi.org/10.1016/j.lwt.2019.05.105
- Anonymous. (2023a). Functional Food Market by Ingredient (Probiotics, Minerals, Proteins & Amino Acids, Prebiotics, & Dietary Fibers, Vitamins and Others), Product (Bakery & Cereals, Dairy Products, Meat, Fish & Eggs, Soy Products, Fats & Oils and Others), Application (Sports Nutrition, Weight Management Clinical Nutrition, Cardio Health, and Others): Global Opportunity Analysis and Industry Forecast 2021–2027. Retrieved May 11, 2023, from https://www.alliedmarketresearch.com/functional-food-market
- Anonymous. (2023b). Functional foods market size, share & trends analysis report by ingredient (carotenoids, prebiotics & probiotics, fatty acids, dietary fibers), by product, by application, by region, and segment forecasts, 2022 - 2030. Retrieved December 31, 2023, from https://www.grandviewresearch.com/industry-analysis/functional-food-market?utm_source=prnewswire&utm_medium=referral&utm_campaign=CMFE_15-Dec-22&utm_term=functional_food_market&utm_content=rd
- Anonymous. (2023c). Probiotics market size, share & trends analysis report by product (food & beverages, dietary supplements), by ingredient (bacteria, yeast), by distribution channel, by end-use, by region, and segment forecasts, 2023 - 2030. Retrieved December 31, 2023, from https://www.grandviewresearch.com/industry-analysis/probiotics-market
- Anonymous. (2023d). Probiyotik Yoğurt Mayası. Retrieved January 01, 2024, from https://doi.org/10.1000/yaylamaya
- AOAC. (1995). pH of acidified foods method, method no. 981.12. In P. Cunniff (Ed.), Official methods of AOAC international analyses (16th ed., pp. 780). Association of Official Analytical Chemists.
- Ares, A. M., Valverde, S., Bernal, J. L., Nozal, M. J., & Bernal, J. (2018). Extraction and determination of bioactive compounds from bee pollen. Journal of Pharmaceutical & Biomedical Analysis, 147, 110–124. https://doi.org/10.1016/j.jpba.2017.08.009
- Atallah, A. A. (2016). The production of bio-yoghurt with probiotic bacteria, royal jelly and bee pollen grains. Journal of Nutrition & Food Sciences, 6(3), 510.
- Aylanc, V., Ertosun, S., Russo‐Almeida, P., Falcão, S. I., & Vilas‐Boas, M. (2022). Performance of green and conventional techniques for the optimal extraction of bioactive compounds in bee pollen. International Journal of Food Science & Technology, 57(6), 3490–3502. https://doi.org/10.1111/ijfs.15672
- Ayyash, M. M., Abdalla, A. K., AlKalbani, N. S., Baig, M. A., Turner, M. S., Liu, S. Q., & Shah, N. P. (2021). Invited review: Characterization of new probiotics from dairy and nondairy products—insights into acid tolerance, bile metabolism and tolerance, and adhesion capability. Journal of Dairy Science, 104(8), 8363–8379. https://doi.org/10.3168/jds.2021-20398
- Azizkhani, M., Saris, P. E. J., & Baniasadi, M. (2021). An in-vitro assessment of antifungal and antibacterial activity of cow, camel, ewe, and goat milk kefir and probiotic yogurt. Journal of Food Measurement and Characterization, 15(1), 406–415. https://doi.org/10.1007/s11694-020-00645-4
- Azizkhani, M., & Tooryan, F. (2016). Antimicrobial activities of probiotic yogurts flavored with peppermint, Basil, and Zataria against Escherichia coli and Listeria monocytogenes. Journal of Food Quality & Hazards Control, 3(3), 79–86.
- Bedani, R., Rossi, E. A., & Saad, S. M. I. (2013). Impact of inulin and okara on Lactobacillus acidophilus La-5 and Bifidobacterium animalis Bb-12 viability in a fermented soy product and probiotic survival under in vitro simulated gastrointestinal conditions. Food Microbiology, 34(2), 382–389. https://doi.org/10.1016/j.fm.2013.01.012
- Casarotti, S. N., & Penna, A. L. B. (2015). Acidification profile, probiotic in vitro gastrointestinal tolerance and viability in fermented milk with fruit flours. International Dairy Journal, 41, 1–6. https://doi.org/10.1016/j.idairyj.2014.08.021
- Charteris, W. P., Kelly, P. M., Morelli, L., & Collins, J. K. (1998). Development and application of an in vitro methodology to determine the transit tolerance of potentially probiotic Lactobacillus and Bifidobacterium species in the upper human gastrointestinal tract. Journal of Applied Microbiology, 84(5), 759–768. https://doi.org/10.1046/j.1365-2672.1998.00407.x
- CLSI M02, M02-A12. (2015). Performance standards for antimicrobial disk susceptibility tests. (Chap. 1, 12th ed., Vol. 35). Clinical and Laboratory Standards Institute.
- CLSI M07-A8. (2009). Methods for dilution antimicrobial susceptibility tests for bacteria that grow aerobically. (Chap. 2, 8th ed., Vol. 26). Clinical and Laboratory Standards Institute.
- CLSI M45. (2016). Methods for antimicrobial dilution and disk susceptibility testing of ınfrequently ısolated or fastidious bacteria (Chap. 17, 3rd ed., Vol.35). Clinical and Laboratory Standards Institute.
- Conte, P., Del Caro, A., Balestra, F., Piga, A., & Fadda, C. (2018). Bee pollen as a functional ingredient in gluten-free bread: A physical-chemical, technological and sensory approach. LWT - Food Science and Technology, 90, 1–7. https://doi.org/10.1016/j.lwt.2017.12.002
- Da Silva, T. M. S., Piazentin, A. C. M., Mendonça, C. M. N., Converti, A., Bogsan, C. S. B., Mora, D., & de Souza Oliveira, R. P. (2020). Buffalo milk increases viability and resistance of probiotic bacteria in dairy beverages under in vitro simulated gastrointestinal conditions. Journal of Dairy Science, 103(9), 7890–7897. https://doi.org/10.3168/jds.2019-18078
- de Florio Almeida, J., dos Reis, A. S., Heldt, L. F. S., Pereira, D., Bianchin, M., de Moura, C., Plata-Oviedo, M. V., Haminiuk, C. W. I., Ribeiro, I. S., da Luz, C. F. P., & Carpes, S. T. (2017). Lyophilized bee pollen extract: A natural antioxidant source to prevent lipid oxidation in refrigerated sausages. LWT-Food Science and Technology, 76, 299–305. https://doi.org/10.1016/j.lwt.2016.06.017
- Del Rio, A. R., Keppler, J. K., Boom, R. M., & Janssen, A. E. (2021). Protein acidification and hydrolysis by pepsin ensure efficient trypsin-catalyzed hydrolysis. Food & Function, 12(10), 4570–4581. https://doi.org/10.1039/D1FO00413A
- De Melo, A. A. M., & de Almeida-Muradian, L. B. (2017). Health benefits and uses in medicine of bee pollen. In J. M. Alvarez-Suarez (Ed.), Bee Products-Chemical and Biological Properties, 261–276. Springer International Publishing AG.
- Denisow, B., & Denisow‐Pietrzyk, M. (2016). Biological and therapeutic properties of bee pollen: A review. Journal of the Science of Food and Agriculture, 96(13), 4303–4309. https://doi.org/10.1002/jsfa.7729
- Eşerler, S., Vardarlı, S., Savaş, G., & Mutlu, C. (2023). Arı poleninin bazı fiziksel, fonksiyonel ve kimyasal özellikleri ve biyolojik etkileri (Some physical, functional and chemical properties and biological effects of bee pollen). Uludağ Arıcılık Dergisi, 23(2), 280–295. https://doi.org/10.31467/uluaricilik.1319365
- Estevinho, L. M., Rodrigues, S., Pereira, A. P., & Feás, X. (2012). Portuguese bee pollen: palynological study, nutritional and microbiological evaluation. International Journal of Food Science & Technology, 47(2), 429–435. https://doi.org/10.1111/j.1365-2621.2011.02859.x
- Farag, S. A., & El-Rayes, T. (2016). Research article effect of bee-pollen supplementation on performance, carcass traits and blood parameters of broiler chickens. Asian Journal of Animal and Veterinary Advances, 11(3), 168–77. https://doi.org/10.3923/ajava.2016.168.177
- Feás, X., Vázquez-Tato, M. P., Estevinho, L., Seijas, J. A., & Iglesias, A. (2012). Organic bee pollen: botanical origin, nutritional value, bioactive compounds, antioxidant activity and microbiological quality. Molecules, 17(7), 8359–8377. https://doi.org/10.3390/molecules17078359
- Fernandez, M. A., Panahi, S., Daniel, N., Tremblay, A., & Marette, A. (2017). Yogurt and cardiometabolic diseases: A critical review of potential mechanisms. Advances in Nutrition, 8(6), 812–829. https://doi.org/10.3945/an.116.013946
- Gahruie, H. H., Eskandari, M. H., Mesbahi, G., & Hanifpour, M. A. (2015). Scientific and technical aspects of yogurt fortification: A review. Food Science and Human Wellness, 4(1), 1–8. https://doi.org/10.1016/j.fshw.2015.03.002
- Ghaderi‐Ghahfarokhi, M., Yousefvand, A., Ahmadi Gavlighi, H., & Zarei, M. (2021). The effect of hydrolysed tragacanth gum and inulin on the probiotic viability and quality characteristics of low‐fat yoghurt. International Journal of Dairy Technology, 74(1), 161–169. https://doi.org/10.1111/1471-0307.12742
- Glušac, J. R., Stijepić, M. J., Milanović, S. D., & Đurđević-Milošević, D. M. (2015). Physicochemical properties of honeybee pollen enriched acidophilus milk and probiotic yoghurt. Acta Periodica Technologica, 46(46), 45–54. https://doi.org/10.2298/APT1546045G
- Gocer, E. M. C., Ergin, F., Kücükcetin, I. O., & Kücükcetin, A. (2021). In vitro gastrointestinal resistance of Lactobacillus acidophilus in some dairy products. Brazilian Journal of Microbiology, 52(4), 2319–2334. https://doi.org/10.1007/s42770-021-00590-4
- Güneş, R., Palabıyık, İ., & Kurultay, Ş. (2018). Şekerleme teknolojisinde fonksiyonel ürün üretimi. Gıda, 43(6), 984–1001. https://doi.org/10.15237/gida.GD18088
- Hacıoğlu, G., & Kurt, G. (2012). Tüketicilerin fonksiyonel gıdalara yönelik farkındalığı, kabulü ve tutumları: İzmir ili örneği. Business and Economics Research Journal, 3(1), 161–171.
- Hani, B., Dalila, B., Saliha, D., Daoud, H., Mouloud, G., & Seddik, K. (2012). Microbiological sanitary aspects of pollen. Advances in Environmental Biology, 6(4), 1415–1420.
- Hill, C., Guarner, F., Reid, G., Gibson, G. R., Merenstein, D. J., & Pot, B., Morelli, L., Canani, R. B., Flint, H. J., Salminen, S., Calder, P. C. & Sanders, M. E. (2014). Expert consensus document: The international scientific association for probiotics and prebiotics consensus statement on the scope and appropriate use of the term probiotic. Nature Reviews Gastroenterology & Hepatology, 11, 506–514. https://doi.org/10.1038/nrgastro.2014.66
- Huang, Y., & Adams, M. C. (2004). In vitro assessment of the upper gastrointestinal tolerance of potential probiotic dairy propionibacteria. International Journal of Food Microbiology, 91(3), 253–260. https://doi.org/10.1016/j.ijfoodmicro.2003.07.001
- ISO 6887-1. (2017). International organization for standardization, microbiology of the food chain — preparation of test samples, initial suspension and decimal dilutions for microbiological examination — part 1: General rules for the preparation of the initial suspension and decimal dilutions, ISO 6887-1. Swiss.
- Kailasapathy, K., & Rybka, S. (1997). L. Acidophilus and bifidobacterium spp. - their therapeutic potential and survival in yogurt. Australian Journal of Dairy Technology, 52(1), 28–35.
- Karabagias, I. K., Karabagias, V. K., Gatzias, I., & Riganakos, K. A. (2018). Bio-functional properties of bee pollen: The case of “bee pollen yoghurt”. Coatings, 8(12), 423. https://doi.org/10.3390/coatings8120423
- Karaman Mutlu, S. (2019). Investigation of the effect of acacia gum and pectin addition on the functional and technological properties of black carrot added probiotic yogurt [ Master thesis, Uludağ University].
- Kaur Sidhu, M., Lyu, F., Sharkie, T. P., Ajlouni, S., & Ranadheera, C. S. (2020). Probiotic yogurt fortified with chickpea flour: Physico-chemical properties and probiotic survival during storage and simulated gastrointestinal transit. Foods, 9(9), 1144. https://doi.org/10.3390/foods9091144
- Kok, C. R., & Hutkins, R. (2018). Yogurt and other fermented foods as sources of health-promoting bacteria. Nutrition Reviews, 76(Supplement_1), 4–15. https://doi.org/10.1093/nutrit/nuy056
- Komosinska-Vassev, K., Olczyk, P., Kaźmierczak, J., Mencner, L., & Olczyk, K. (2015). Bee pollen: Chemical composition and therapeutic application. Evidence-Based Complementary and Alternative Medicine, 2015, 1–6. https://doi.org/10.1155/2015/297425
- Lapierre, L., Undeland, P., & Cox, L. J. (1992). Lithium chloride-sodium propionate agar for the enumeration of bifidobacteria in fermented dairy products. Journal of Dairy Science, 75(5), 1192–1196. https://doi.org/10.3168/jds.S0022-0302(92)77866-7
- Lomova, N., Snizhko, O., & Narizhniy, S. (2014). Yoghurt enrichment with natural bee farming products. Ukrainian Food Journal, 3(3), 405–411. http://193.138.93.8/bitstream/BNAU/905/1/Yoghurt_enrichm.pdf
- Madureira, A. R., Amorim, M., Gomes, A. M., Pintado, M. E., & Malcata, F. X. (2011). Protective effect of whey cheese matrix on probiotic strains exposed to simulated gastrointestinal conditions. Food Research International, 44(1), 465–470. https://doi.org/10.1016/j.foodres.2010.09.010
- Makino, S., Ikegami, S., Kano, H., Sashihara, T., Sugano, H., Horiuchi, H., Saito, T., & Oda, M. (2006). Immunomodulatory effects of polysaccharides produced by Lactobacillus delbrueckii ssp. bulgaricus OLL1073R-1. Journal of Dairy Science, 89(8), 2873–2881. https://doi.org/10.3168/jds.S0022-0302(06)72560-7
- Markowiak, P., & Śliżewska, K. (2017). Effects of probiotics, prebiotics, and synbiotics on human health. Nutrients, 9(9), 1021. https://doi.org/10.3390/nu9091021
- Matuszewska, E., Klupczynska, A., Maciołek, K., Kokot, Z. J., & Matysiak, J. (2021). Multielemental analysis of bee pollen, propolis, and royal jelly collected in west-central Poland. Molecules, 26(9), 2415. https://doi.org/10.3390/molecules26092415
- Megur, A., Daliri, E. B. M., Balnionytė, T., Stankevičiūtė, J., Lastauskienė, E., & Burokas, A. (2023). In vitro screening and characterization of lactic acid bacteria from Lithuanian fermented food with potential probiotic properties. Frontiers in Microbiology, 14, 14. https://doi.org/10.3389/fmicb.2023.1213370
- Michels, K. B., Willett, W. C., Vaidya, R., Zhang, X., & Giovannucci, E. (2020). Yogurt consumption and colorectal cancer incidence and mortality in the Nurses’ Health Study and the health professionals follow-up study. The American Journal of Clinical Nutrition, 112(6), 1566–1575. https://doi.org/10.1093/ajcn/nqaa244
- Mirzaei, E. Z., Lashani, E., & Davoodabadi, A. (2018). Antimicrobial properties of lactic acid bacteria isolated from traditional yogurt and milk against Shigella strains. GMS Hygiene and Infection Control 13(Doc01),1–5.
- Mohdaly, A. A., Mahmoud, A. A., Roby, M. H., Smetanska, I., & Ramadan, M. F. (2015). Phenolic extract from propolis and bee pollen: Composition, antioxidant and antibacterial activities. Journal of Food Biochemistry, 39(5), 538–547. https://doi.org/10.1111/jfbc.12160
- Mohsin, A. Z., Marzlan, A. A., Muhialdin, B. J., Wai, L. K., Mohammed, N. K., & Hussin, A. S. M. (2022). Physicochemical characteristics, GABA content, antimicrobial and antioxidant capacities of yogurt from Murrah buffalo milk with different fat contents. Food Bioscience, 49, 101949. https://doi.org/10.1016/j.fbio.2022.101949
- Molaee Parvarei, M., Fazeli, M. R., Mortazavian, A. M., Sarem Nezhad, S., & Mortazavi, S. A. (2021). Comparative effect of probiotic and paraprobiotic addition on rheological and sensory properties of yoghurt. International Journal of Dairy Technology, 74(1), 95–106. https://doi.org/10.1111/1471-0307.12727
- Namaei, M. H., Ghannadkafi, M., & Ziaee, M. (2015). Antibacterial effect of non-industrial yogurt on salmonella and shigella. Modern Care Journal, 12(3), 109–113.
- Özcan, M., Fındık, S., Uylaşer, V., & Çoban, D. İ. (2020). Investigation of the physical and chemical properties of traditional homemade yogurt with different rates of pollen additions. The European Journal of Science and Technology, 20, 516–521. https://doi.org/10.31590/ejosat.736476
- Peng, S., Song, J., Zeng, W., Wang, H., Zhang, Y., Xin, J., & Suo, H. (2021). A broad-spectrum novel bacteriocin produced by lactobacillus plantarum SHY 21–2 from yak yogurt: Purification, antimicrobial characteristics and antibacterial mechanism. LWT - Food Science and Technology, 142, 110955. https://doi.org/10.1016/j.lwt.2021.110955
- Ranadheera, C. S., Evans, C. A., Adams, M. C., & Baines, S. K. (2012). In vitro analysis of gastrointestinal tolerance and intestinal cell adhesion of probiotics in goat’s milk ice cream and yogurt. Food Research International, 49(2), 619–625. https://doi.org/10.1016/j.foodres.2012.09.007
- Rzepecka-Stojko, A., Stojko, J., Kurek-Górecka, A., Górecki, M., Kabała-Dzik, A., Kubina, R., Moździerz, A., & Buszman, E. (2015). Polyphenols from bee pollen: Structure, absorption, metabolism and biological activity. Molecules, 20(12), 21732–21749. https://doi.org/10.3390/molecules201219800
- Seçkin, A. K., & Baladura, E. (2011). Süt ve süt ürünlerinin fonksiyonel özellikleri. Celal Bayar University Journal of Science, 7(1), 27–38.
- Sert, D., Akın, N., & Dertli, E. (2011). Effects of sunflower honey on the physicochemical, microbiological and sensory characteristics in set type yoghurt during refrigerated storage. International Journal of Dairy Technology, 64(1), 99–107. https://doi.org/10.1111/j.1471-0307.2010.00635.x
- Sezen, F., & Koçak, C. (2006). Fonksiyonel süt ürünleri teknolojisindeki gelişmeler. In 9. Gıda Kongresi, Bolu, Turkey, 24-26 May 2006 (pp. 89–92). Gıda Teknolojisi Derneği Yayın.
- Şimşekli, N., & Doğan, İ. S. (2015). Geleneksel ve fonksiyonel ürün olarak Maraş tarhanası. Iğdır University Journal of the Institute of Science and Technology, 5(4), 33–40.
- Soylu, P., & Bayram, B. (2020). Bal, propolis, arı sütü, çivanperçemi (Achillea millefolium) ve ekinezya (Echinacea paradoxa) karışımından fonksiyonel gıda üretimi, ürünün fizikokimyasal ve biyokimyasal özelliklerinin incelenmesi. Journal of Bahri Dagdas Animal Research, 9(1), 25–38.
- Sun, L., Guo, Y., Zhang, Y., & Zhuang, Y. (2017). Antioxidant and anti-tyrosinase activities of phenolic extracts from rape bee pollen and inhibitory melanogenesis by cAMP/MITF/TYR pathway in B16 mouse melanoma cells. Frontiers in Pharmacology, 8(104), 1–9. https://doi.org/10.3389/fphar.2017.00104
- Thakur, M., & Nanda, V. (2020). Composition and functionality of bee pollen: A review. Trends in Food Science & Technology, 98, 82–106. https://doi.org/10.1016/j.tifs.2020.02.001
- TS ISO 7889. (2004). Yoğurt. Karakteristik mikroorganizmaların sayımı. 37°C‘de koloni sayım tekniği. Türk Standartlar Enstitüsü.
- Uțoiu, E., Matei, F., Toma, A., Diguță, C. F., Ștefan, L. M., Mănoiu, S., Vrăjmașu, V. V., Moraru, I., Oancea, A., & Israel-Roming, F. (2018). Bee Collected Pollen with enhanced health benefits, produced by fermentation with a Kombucha Consortium. Nutrients, 10(10), 1365. https://doi.org/10.3390/nu10101365
- Vinderola, G., Cespedes, M., Mateolli, D., Cardenas, P., Lescano, M., Aimaretti, N., & Reinheimer, J. (2011). Changes in gastric resistance of Lactobacillus casei in flavoured commercial fermented milks during refrigerated storage. International Journal of Dairy Technology, 64(2), 269–275. https://doi.org/10.1111/j.1471-0307.2010.00659.x
- Vinderola, C. G., & Reinheimer, J. A. (1999). Culture media for the enumeration of bifidobacterium bifidum and Lactobacillus acidophilus in the presence of yoghurt bacteria. International Dairy Journal, 9(8), 497–505. https://doi.org/10.1016/S0958-6946(99)00120-X
- Yerlikaya, O. (2014). Effect of bee pollen supplement on antimicrobial, chemical, rheological, sensorial properties and probiotic viability of fermented milk beverages. Mljekarstvo, 64(4), 268–279. https://doi.org/10.15567/mljekarstvo.2014.0406
- Yerlikaya, O., Saygili, D., & Akpinar, A. (2021). Evaluation of antimicrobial activity and antibiotic susceptibility profiles of Lactobacillus delbrueckii subsp. bulgaricus and streptococcus thermophilus strains isolated from commercial yoghurt starter cultures. Food Science and Technology, 41(2), 418–425. https://doi.org/10.1590/fst.03920
- Yıldız, O., Can, Z., Saral, O., Yuluğ, E., Oztürk, F., Aliyazıcıoğlu, R., Canpolat, S., & Kolaylı, S. (2013). Hepatoprotective potential of chestnut bee pollen on carbon tetrachloride‐induced hepatic damages inrats. Evidence‐Based Complementary and Alternative Medicine, 2013, 1–9. https://doi.org/10.1155/2013/461478
- Yılmaz, L. (2006). The use of different probiotic culture combinations in production of yoghurt-like fermented dairy products [ Doctoral thesis, Uludağ University].
- Yilmaz-Ersan, L., & Kurdal, E. (2014). The production of set-type-bio-yoghurt with commercial probiotic culture. International Journal of Chemical Engineering and Applications, 5(5), 402. https://doi.org/10.7763/IJCEA.2014.V5.418
- Ziar, H., Gérard, P., & Riazi, A. (2014). Effect of prebiotic carbohydrates on growth, bile survival and cholesterol uptake abilities of dairy‐related bacteria. Journal of the Science of Food and Agriculture, 94(6), 1184–1190. https://doi.org/10.1002/jsfa.6395
- Zuluaga, C., Martínez, A., Fernández, J., López-Baldó, J., Quiles, A., & Rodrigo, D. (2016). Effect of high pressure processing on carotenoid and phenolic compounds, antioxidant capacity, and microbial counts of bee-pollen paste and bee-pollen-based beverage. Innovative Food Science & Emerging Technologies, 37, 10–17. https://doi.org/10.1016/j.ifset.2016.07.023