ABSTRACT
The ability of Grewia tenax fruit dried-ground (GTF-DG) as a source of antioxidants, and the influence of varying concentrations (0, 2, 4, and 6%) of GTF-DG on beef burger quality after 21 days of refrigerated storage, were explored. GTF-DG contained high phenolics and flavonoid contents and, therefore, displayed strong DPPH, radical ABTS, and ferric-reducing activity. The GTF-DG effectively stopped the development of bacterial strains at 100 g/disc. The burgers’ pH did not change significantly after the addition of GTF-DG. The burger’s antioxidant content and activity increased when GTF-DG was added at 4 and 6%, resulting in a significant decrease in microbial spoilage and lipid oxidation throughout the storage. GTF-DG caused a significant (p ≤ .05) improvement in burger cooking parameters. Burgers with 4% and 6% GTF-DG had higher sensory acceptance (p ≤ .05) than other treatments. In conclusion, burgers’ shelf life can be improved by the bioactive components in GTF-DG.
1. Introduction
The production and consumption of meat products expanded significantly in recent decades due to increased consumer requests, improved quick processing, and great nutritional and health values of these products (Hadidi et al., Citation2022). The quality attributes of beef products are diminished by lipid oxidation during preparation and preservation and the oxidation of meat-based food is the main factor that determines the acceptance, and quality, as well as the loss of their flavor and taste (Das et al., Citation2021). Meat is vulnerable to oxidative deterioration because muscle tissues contain significant concentrations of unsaturated fats, heme-containing pigments, metallic catalysts, and oxidizing substances (Das et al., Citation2021). Discoloration, off-flavor development, hazardous chemical generation, poor storability, as well as nutritional and osmatic losses, are all indications of oxidative deterioration in beef and other meat products (Amoli et al., Citation2021).
According to Hadidi et al. (Citation2022), processed beef oxidation can be reduced by using artificial antioxidants namely butylated-hydroxyl-toluene and butylated-hydroxyl-anisole. Questions about the use and safety of the aforementioned synthetic antioxidants have grown among both consumers and health professionals (Hadidi et al., Citation2022). Therefore, natural alternatives, such as plant-derived antioxidants, must be investigated for their inexpensiveness and health advantages (Moosavy et al., Citation2017). Spices and herbs are two examples of plant materials that are abundant in phenolics and flavonoids, which are bioactive compounds with antioxidant and antibacterial characteristics (Moosavy et al., Citation2017). Many bioactive compounds derived from plants, such as baobab fruit (Al-Juhaimi et al., Citation2020), Acacia nilotica (Al-Juhaimi et al., Citation2020; Babiker et al., Citation2019), and clove powder (Ahmed et al., Citation2022) provided exceptional quality attributes and improved meat products shelf-life during cold storage.
Grewia tenax fruit (GTF) is a deciduous tropical shrub or tree that grows wildly in various semi-arid and sub-humid areas of Africa and Asia. Because of the high nutritional and health value of GTF, it is regarded as a viable candidate for domestication and commercialization as a new crop (Sharma & Patni, Citation2013). Further, they reported that GTF contains high quantities of potassium, calcium, zinc, magnesium, iron, and vitamins, which provide amounts of 20–30 times the daily requirement of these nutrients. The GTF is high in essential and non-essential amino acids as well as bioactive chemicals like sterols, polyphenols, and flavonoids (Hamid & Arabi, Citation2021). The extract of GTF possessed antioxidant, hematological, histopathological, hepato-protective, and lipid-lowering effects in carbon tetrachloride-induced hepatotoxicity in mice (Hamid & Arabi, Citation2021). The fruits are used for the production of nutritious juice, jam, and syrup (Suliman et al., Citation2018). Despite the high nutritional quality and bioactive properties of GTF, its exploitation as a stabilizing agent in food products is scarce. Thus, the present study aimed to assess the antioxidant capacity and antibacterial activity of GTF dried-ground (GTF-DG) as well as the impact of GTF-DG on a beef burger’s physicochemical, microbiological, and sensory aspects during cold storage for 3 weeks.
2. Materials and methods
2.1. Materials
Twenty-four hours postmortem, boneless beef rounds of young Holstein Friesians (Bos taurus) were acquired from a meat slaughtering house in Riyadh, Saudi Arabia. Other ingredients and dried GTF were bought from a Saudi bazaar. The GTF was ground to pass through a sieve (1-mm), and the dried-ground (GTF-DG) was autoclaved and sealed in plastic containers and kept at 4°C for analysis and beef burger production. The chemicals and media utilized in this study were all of the analytical variety.
2.2. Methods
2.2.1. Preparation of burger- GTF-DG formulates
According to F. Y. Al-Juhaimi et al. (Citation2020), beef burgers with and without GTF-DG were prepared. Briefly, minced beef, minced fat, spices, and salt were uniformly mixed followed by adding different levels (0%, 2%, 4%, and 6%) of GTF-DG and calculated amount of water and start were added and the mixture was homogenized for 5 min using a Stephan UM 12 (Stephan U. Sohner GmbH & Co., Germany) mixer. After that, portions of the blend (100 g each) were formed into a burger using a burger-forming device (Expro. Co., Shanghai, China). The prepared burger samples were then put in sealed plastic containers with small holes kept at 4°C for three weeks and tested every seven days. A comparable manufacturing process was used to manufacture 480 beef burgers in total: 10 burgers per treatment x 4 treatments (0%, 2%, 4%, and 6% GTF-DG), x 4 periods of storage (0, 7, 14, and 21 days), and x 3 separate replications.
2.2.2. Cooking of burger- GTF-DG formulates
Burger samples were subjected to cooking in a conventional oven (Hobart Corp., Troy, Ohio, U.S.A.) at 180°C. The burgers were rotated and flipped every 5 minutes to achieve equal cooking (Al‐Juhaimi et al., Citation2020).
2.2.3. Cooking properties of burger- GTF-DG formulates
At zero time and every 7 days from storage, cooking characteristics (cooking yield, fat retention, moisture retention, and dimensional shrinkage) of burger- GTF-DG formulate were assessed using the method of Murphy et al. (Citation1975).
2.2.4. pH of beef burger- GTF-DG formulates
The raw beef burger sample (10 g) was homogenized in 100 mL distilled water using a PolyTron® PT-2500E homogenizer (Luzern, Switzerland), and the pH of the homogenate was estimated using a pH meter (Corning Scientific Products, New York, U.S.A.). Three-point standardization (pH 4, pH 7, and pH 10) is utilized for high-accuracy pH readings.
2.2.5. Preparation of GTF-DG and beef burger extracts
Raw and cooked beef burgers were freeze-dried and ground to a fine powder. The preparation of burger and GTF-DG extracts was carried out by suspending the sample (1.0 g) in distilled water (10 mL) followed by continuous stirring (Fisher, 14–511-1A, U.S.A.) overnight at 4°C. After 30 minutes of centrifugation at 5000 × g, the supernatants were collected for further use.
2.2.6. Antimicrobial activity assessment of GTF-DG
The GTF-DG extract inhibition ability was assessed on different Gram-negative and Gram-positive strains (Salmonella typhimurium ATCC 14028, Escherichia coli ATCC 10536, Klebsiella pneumoniae ATCC 10031, Listeria monocytogenes ATCC 19114, Yersinia enterocolitica ATCC 27729, Bacillus cereus ATCC 14579, Clostridium perfringens ATCC 13124, Micrococcus luteus ATCC 10240, and Staphylococcus aureus ATCC 29737) using a disc diffusion method (Efstratiou et al., Citation2012).
2.2.7. Microbial load determination
The raw beef burger total plate count was measured using Oxoid plate count agar (Oxoid, Basingstoke, UK). After incubating the plates for 48 hours at 37°C, the colonies were calculated and the data was stated as log 10 cfu/g.
2.2.8. Total phenolic content determination
As designated in Singleton and Rossi’s (Citation1965) method, the assessment of the phenolics of GTF-DG and burger extracts was done using a Folin-Ciocalteu reagent by measuring the absorbance at 765 nm using a PD-303UV visible spectrophotometer (Apel, Saitama, Japan).
2.2.9. Total flavonoid content determination
As described in Kim et al. (Citation2003) method, the total flavonoid as mg/100 g CE equivalents of GTF-DG and burger extracts was determined spectrophotometrically at 510 nm using a PD-303UV visible spectrophotometer (Apel, Saitama, Japan).
2.2.10. Antioxidant determination
2.2.10.1. DPPH antiradical activity
The method of Lee et al. (Citation1998) was employed to ascertain the DPPH radial scavenging activity of the GTF-DG and burger extracts at 517 nm using a PD-303UV visible spectrophotometer (Apel, Saitama, Japan).
2.2.10.2. ABTS assay
The ABTS assay of the GTF-DG and burger extracts was done as designated by Re et al. (Citation1999). The ABTS was evaluated in micromoles of Trolox equivalents per gram of material (g TE/g).
2.2.10.3. Determination of FRAP activity
The FRAP was calculated by Oyaizu’s (Citation1986) method. Micromoles of Trolox equivalents/gram of GTF-DG and/or burger samples (g TE/g) were used to express the results.
2.2.11. Determination of TBARS
The TBARS levels in burger samples were determined following the Rosmini et al. (Citation1996) technique. Tetraethoxy-1,1,3,3-propane was utilized to create a standard curve for TBARS values, and the results were expressed as mg malonaldehyde/kg sample.
2.2.12. Color measurement
According to the instruction manual, a Hunter Lab colorimeter (Miniscan® XE plus 4500L, Reston, VA, U.S.A.) equipped with a D65 illuminant, 25 mm aperture, and a 2° observer angle was used to evaluate the characteristics of the color of the raw burger. Five records were taken at random positions across the burger surface for each sample, and the results were averaged.
2.2.13. Sensory evaluation
A 9-point hedonic scale was employed by a group of twenty semi-trained male panelists (students and staff from King Saud University’s College of Food and Agricultural Sciences) to assess the sensory attributes (color, taste, flavor, texture, juiciness, and overall acceptance) of cooked beef burgers. The panel participants attended three training sessions to refresh their memory on the qualities that would be assessed before the sensory analysis. Written informed consent was collected from participants before the test. The evaluation was done in the sensory room and the samples were presented on white plates numbered three times at random. During the sampling period, three sensory sessions were conducted, with each panelist receiving four samples during each session. Tests were run three times at each storage period, with scores ranging from “like extremely” = 9 to “dislike extremely” = 1. The mean scores obtained were computed for every sample and session.
2.2.14. Multivariate analysis
Multivariate analysis of the data was achieved by analyzing principal component (PCA) and hierarchical cluster analysis (HCA) using MULTBIPLOT software (Vicente-Villardón, Citation2010) according to the instructions manual.
2.2.15. Statistical analysis
The antibacterial activity (inhibition zone) and bioactive characteristics (TP, TF, DPPH, ABTS, and FRAP) of GTF-DG data were statistically examined using ANOVA, and the Duncan multiple range test (DMRT) was applied for mean comparison at p ≤ .05. For the burger experiments, an entirely randomized block design was applied. The study contained 4 treatments (control, 2% GTF-DG, 4% GTF-DG, and 6% GTF-DG), and measurements were done across 4 storage intervals (0, 7, 14, and 21 days). On four distinct days, the complete block was repeated three times (n = 3). The effect of treatments (T) and storage time (S), besides the interaction (T × S), on the physicochemical, microbiological, storage stability, and sensory aspects of burger-GTF-DG formulations, was examined using two-way ANOVA and DMRT (SAS 8.0 software, SAS Institute, Inc., Cary, NC, U.S.A.) with T, S, and T × S as fixed terms and all quality attributes and replication as random terms. The results were provided as mean values with standard deviation (SD), with p ≤ .05 considered significant.
3. Results and discussion
3.1. Total phenolic (TP), flavonoid (TF), antioxidant, and antibacterial activity of GTF-DG
The TP, TF, antioxidant, and antibacterial properties of GTF-DG are listed in . The results exhibited that the GTF-DG had a TP of 28.34 mg GAE/g and a TF of 21.25 mg CE/g. The GTF-DG antioxidant activity as DPPH was found to be 83.85%, as ABTS was 7.08 mg TE/g, and as FRAP was 7.59 mg TE/g. The TP, TF, and antioxidant activity obtained were high compared to those reported by Suliman et al. (Citation2018). Moreover, Isra (Citation2020) reported low TP from GTF-DG using four different solvents. Differences in bioactive compounds between studies may be due to variations in Grewia tenax postharvest treatments, genotypes, stages of maturity, locations, growing seasons, and methods of extraction. Because of its high concentration of bioactive molecules and antioxidant activity, GTF-DG might be employed as a functional component to prolong the shelf-life by minimizing lipid oxidation. Moreover, Grewia species are thought to be one of the most nutritious foods since they are high in fiber, vitamins, carbs, protein, and minerals, all of which are necessary for a healthy diet. Grewia is high in supplements, which could be important support for boosting the nutrient content of rural and urban populations in many African and Asian countries (Qamar et al., Citation2021).
Table 1. Grewia tenax fruit dried-ground (GTF-DG) oxidative characteristics and antimicrobial activity.
3.2. Antimicrobial activity of GTF-DG extracts (GTF-DGE)
compares the influences of the extract of GTF-DG (100 μg/disc) on nine Gram-positive and Gram-negative bacterial strains to penicillin as a conventional antibiotic. In terms of inhibition zone, the GTF-DGE for Klebsiella pneumonia (ATCC 10031) was comparable to that of penicillin. However, for Micrococcus luteus (ATCC 10240) and Bacillus cereus (ATCC 14579), GTF-DGE had significant (p ≤ .05) high inhibition zones compared to penicillin. The inhibition-zone values for Salmonella typhimurium (ATCC 14028), Escherichia coli (ATCC 10536), Yersinia enterocolitica (ATCC 27729), Clostridium perfringens (ATCC 13124), Listeria monocytogenes (ATCC 19114), and Staphylococcus aureus (ATCC 29737) were significantly (p ≤ .05) lower than that of penicillin. Elrofaei et al. (Citation2018) observed that when Grewia tenax fruit extract was tested against Escherichia coli, the extract demonstrated strong antibacterial action, however, extract in petroleum ether and chloroform did not affect the pathogen. The presence of alkaloids may be responsible for the plant’s high activity. The current results are analogous to a study on clove extracts (100 μg/mL) which displayed a strong antibacterial action against Gram-negative and Gram-positive bacteria, according to Ahmed et al. (Citation2022). Moreover, the antimicrobial activity of Grewia tenax was found to be comparable to the previous plant extract study on baobab fruit (Al-Juhaimi et al., Citation2020), which was found to be efficient in inhibiting microbial growth.
In the current study, the high levels of phenols and flavonoids from GTF-DG are most likely responsible for the powder’s antibacterial activity. According to Ahmed et al. (Citation2022), the outer layer of Gram-positive bacteria’s cell membrane, which is made up of dense lipopolysaccharide molecules is more sensitive to antibacterial substances than that of Gram-negative bacteria, which is made up of perivascular enzymes. These results showed that Gram-positive strain cells are more sensitive to GTF-DG extract than Gram-negative strain cells. Because of this, some Gram-positive bacteria’s cell walls and cytoplasmic membranes are easily disintegrated by antimicrobial substances, allowing the release of the cytoplasm’s contents and the eventual death of the bacteria. Thus, the GTF-DG is a natural antibacterial agent that may help food last longer by limiting the growth of microbes.
3.3. Burger- GTF-DG formulates cooking properties during cold storage
The formulation of the burgers with varied concentrations of GTF-DG had major (p ≤ .05) impacts on the characteristics of cooked burgers, as indicated in . At 2%, the formulation of burgers with GTF-DG considerably (p ≤ .05) augmented the yield of cooking, holding of fat, and holding of moisture to 78.67, 82.25, and 74.38%, respectively when compared to the control at the start of storage. These effects increased concomitantly (p ≤ .05) as the GTF-DG concentration was increased to 4% and 6%. The preserved burgers at 7, 14, and 21 days, had high retention of fat values, which was detected in the burgers containing 6%, as well as the yield of cooking and retention of moisture. Dimensional shrinkages of the control beef burger were considerably (p ≤ .05) greater than those of the burgers with GTF-DG. Increases in GTF-DG concentration considerably (p ≤ .05) reduced the beef burgers’ dimensional shrinkages during cooking at all storage durations. Several variables contribute to burger shrinkage during cooking, such as the loss of liquefied fat and liquids, denaturation of muscle protein, and evaporation of water, all of which are likely to affect the cooked burger’s texture. The study’s results agree with those of burgers formulated with Argel leaf powder (Al-Juhaimi et al., Citation2018). The reason for the high cooking yield, moisture retention, and fat retention and decreased dimensional shrinkage in burgers formulated with GTF-DG is probably due to the potential for GTF-DG to change the beef burgers’ matrix structure and hence enhance the product’s capacity to retain fat and moisture. The designed product’s cooking yield may increase as a result of the potential of GTF-DG to keep more fat and water within the burger matrix (Al-Juhaimi et al., Citation2018). Additionally, the binding properties of GTF-DG may stabilize the burger-matrix structure, minimizing moisture and juiciness losses while maintaining the size and shape of the burger. Furthermore, maintaining more fat and moisture inside the matrix of the burger may enhance the sensory and the burger’s physical properties (Al-Juhaimi et al., Citation2018). The GTF-DG, which comprises fibers, proteins, and starches in addition to phenolic chemicals, was employed in this investigation. These ingredients may change the structure of the burger matrix and enhance its cooking qualities through their interactions with it during preparation and cooking.
Table 2. Changes in cooking characteristics of beef burgers mixed with different levels of Grewia tenax fruit dried-ground (GTF-DG) during refrigerated storage (4°C).
3.4. Raw burger- GTF-DG formulates pH, microbiological analysis, and antioxidant activity during cold storage
As indicated in , the GTF-DG addition had no appreciable influence on the burger’s pH during storage (21 days) when compared to the control samples. During cold storage, the pH of untreated burgers decreased significantly (p ≤ .05) from 5.66 on day 0 to 4.57 on day 7 of storage. The decrease of unformulated burgers’ pH level during cold storage was likely to be due to acid-producing bacteria which generate acidic substances (Wang et al., Citation2013). Whereas, the pH of beef burgers formulated with different levels (2%, 4%, and 6%) of GTF-DG was not altered during storage suggesting the pH-maintaining potentials of GTF-DG. The results are in line with earlier data on beef burgers made with different amounts of clove extract and kept refrigerated for 21 days, during this time the pH values of the burgers did not significantly alter (Ahmed et al., Citation2022). Furthermore, after 7 days (Zahid et al., Citation2018) and 10 days (Zahid et al., Citation2020) in the refrigerator at 4°C, no significant pH changes in beef burgers containing clove extract were observed.
Table 3. Raw beef burgers with varying levels of Grewia tenax fruit dried-ground (GTF-DG) pH, plate count, and oxidative properties during refrigerated storage (4°C).
The plate count of the treated and untreated burger samples at 2% (3.08) and 4% GTF-DG (3.01) was significantly (p ≤ .05) higher at day zero of storage than the burger samples that contained 6% GTF-DG (2.54). It was noted that the plate counts significantly (p ≤ .05) dropped as the GTF-DG concentrations increased. Both the treated and control beef burgers’ plate counts increased as the storage period lengthened, despite the fact that the increment in untreated burgers was considerably (p ≤ .05) greater than that of the GTF-DG-formulated. On days 14 and 21, the control samples’ plate count (log CFU/g) exceeded 7.0, and on day 21, for 2% GTF-DG, making these burger samples unsafe for human consumption. As a result, no additional storage or analysis was carried out on these burger samples. The burger treated with 6% GTF-DG had the fewest plates count throughout the storage time. As a result, 6% GTF-DG can be thought of as a green processing preservative that improves burger storage stability for a maximum of 21 days at 4°C. The considerable decrease in microbial growth in beef burgers with GTF-DG could be related to the GTF-DG antimicrobial activity (). During the storage period, GTF-DG and other TP-rich plant materials can help preserve meat and meat products while also improving product stability (Munekata et al., Citation2020). This was validated in the current investigation, which looked at the total phenolic (TP) content of designed beef burgers. The mechanisms by which plant phenolic compounds suppress microbial growth in meat products are not fully understood, however, it has been hypothesized that phenolic compounds could destabilize the microbial cell and membranes leading to the destruction of cell wall, inactivation of intercellular enzymes, and coagulation of cell contents (Kalogianni et al., Citation2020). In addition, the phenolic ring in the phenolic compounds acts as a cation transporter leading to the efflux of K+ and influx of H+ and destruction of ATP synthesis pathways, whereas, hydrophobicity of phenolic compounds aids in binding with lipid bilayer in microbial cells leading to loss of cell integrity, pore formation, leaching out the cellular components, and dysregulation of protein and DNA synthesis (Kalogianni et al., Citation2020).
As GTF-DG increased, TP levels in GTF-DG-treated burgers significantly (p ≤ .05) increased () with a concomitant increase in antioxidant activity. Because of hydrolysis and the use of phenolic compounds to prevent burger peroxidation during storage, the TP of GTF-DG-formulated burgers is decreased. Therefore, GTF-DG-containing burgers concurrently lost antioxidant activity during storage. The amount and type of each polyphenol have a major influence on the biological characteristics of phenol-rich plants and their extracts, including their antibacterial and antiviral, as well as antioxidant activities (Takó et al., Citation2020). The results obtained agree with a previous investigation, which demonstrated that beef burgers’ TP and antioxidant activity were significantly increased when plant extracts were added (Babiker et al., Citation2019). We deemed the control samples unfit for human consumption because the TP and antioxidant-activity assessments on 14 and 21 days, as well as on 21 days for 2% GTF-DG of cold storage, could not be determined as a result of high microbial load.
As shown in , the GTF-DG-formulates had considerably (p ≤ .05) lowered values of TBARS when compared to untreated burger samples. When the amount of GTF-DG was increased in the burger, the amount of TBARS dropped dramatically (p ≤ .05), with the lowest decrease observed in 6% GTF-DG-formulated burgers. In all four treatments, the TBARS grew as storage time increased, showing that the stored compounds’ aldehyde synthesis persisted. In comparison to the other treatments at the same storage duration, the burgers treated with 6% GTF-DG on day 14 had the lowest TBARS amount (0.53 mg malonaldehyde/kg sample). GTF-DG’s phenolics prevented lipid oxidation. The lipid oxidation process is initiated by the interaction of activated oxygen with unsaturated fatty acids promoted by the presence of reactive oxygen species (ROS), reactive nitrogen species (RNS), and transition metals thereby leading to the formation of hydrogen peroxides and hydroxyl and superoxide anions radicals (Domínguez et al., Citation2019). Plant phenolic compounds prevent lipid oxidation by controlling the formation of ROS and free radicals, scavenging free radicals, and chelating transition metals (Hadidi et al., Citation2022), the mechanisms by which GTF-DG’s phenolic compounds prevented lipid oxidation of beef burgers. The results of studies on meat burgers including clove extract (Zahid et al., Citation2020), plants by-products (Hadidi et al., Citation2022), and baobab fruit extract (Al-Juhaimi et al., Citation2020) are consistent with this finding. Antioxidants should prevent oxidation in beef burgers during refrigerated storage due to their presence in treated burgers. Reduced TP and antioxidant activity in the GTF-DG-treated burgers suggest that these processes were employed to stave off oxidation during storage.
In chicken patties with Acacia nilotica fruit extract (Babiker et al., Citation2019), and meat burgers treated with clove powder (Ahmed et al., Citation2022), a similar observation was obtained during cold storage. As evidenced by the great total polyphenols and antioxidant activity in the GTF-DG-formulating burgers during storage, GTF-DG addition was successful in preventing lipid oxidation and any unfavorable reaction that could have harmed the product, enhancing the health benefits, and prolonging the shelf life of the burger.
3.5. Raw beef burger-GTF-DG color properties during storage
displays the color features of the raw beef burgers kept for 21 days at 4°C. The addition of GTF-DG to beef burgers increased the color quality (L*, a*, b*, and c*) when compared to the control at the start of storage (p ≤ .05), with 6% treated burgers having high values of the color attributes. Compared to the untreated burgers, the L* values of the GTF-DG-formulated burgers were considerably (p ≤ .05) greater. The GTF-DG’s yellow-to-brown color may be the cause of the beef burgers’ elevated a*, b*, and c* following its addition. The concentration of the burger color by the GTF-DG most likely caused the beef burger’s L* value to increase following the addition of the GTF-DG. Because many plant materials and extracts contain naturally occurring pigments, including polyphenols, such plant materials can affect color even in cases when antioxidant activity is low. As a result, the plant material pigments utilization may be responsible for the variation in color characteristics, which may have a big effect on the burgers’ appearance.
Table 4. Raw beef burgers with different levels of Grewia tenax fruit dried-ground (GTF-DG) color properties during refrigerated storage (4°C).
The study results are inconsistent with previous reports on the baobab fruit powder (Al-Juhaimi et al., Citation2020), but they are similar to earlier reports showing an upsurge in the L*, a*, and b* levels of meat burgers made with Moringa fruit powder (Al-Juhaimi et al., Citation2016). All burgers containing GTF-DG showed a decrease in their a* values during storage (p ≤ .05), and they experienced another decline as the storage time increased. However, the L* values decreased concurrently (p ≤ .05) in the GTF-DG-formulated burgers. Throughout storage, the GTF-DG-formulated burgers’ b* and c* values likewise decreased. The decrease in color features of the beef patties containing GTF-DG at the end of storage might be explained by the greater liberation of colorants during storage (Ferreira et al., Citation2022). Additionally, because GTF-DG has a great concentration of bioactive antioxidants and strong antioxidant activity, it may have stabilized the color of the beef burger.
3.6. Cooked beef burger-GTF-DG formulates sensory attributes
The sensory attributes of a cooked beef burger prepared with variable levels of GTF-DG and kept at 4°C are displayed in . Compared to untreated and other GTF-DG-formulated samples, the color acceptability of beef burgers with the highest dosage of GTF-DG (6%) declined significantly (p ≤ .05) over time. This might be because the panelists didn’t like the GTF-DG because of its slightly yellow/brown color. Similar findings, including a modest change in the color of beef burgers containing clove powder, were observed by Ahmed et al. (Citation2022). Compared to the control samples, the burgers’ flavor, taste, texture, and juiciness were considerably (p ≤ .05) improved by the addition of GTF-DG after cooking. Even while the burgers’ organoleptic properties deteriorated with storage, the formulated ones were still superior to the unformulated ones. This supports the research of Hawashin et al. (Citation2016), who found that cold storage slightly decreased the sensory qualities of a beef burger made with a destoned olive cake. According to the current research, adding 4 to 6% GTF-DG could lengthen the burger’s shelf life without compromising its organoleptic qualities.
Table 5. Sensory attributes of cooked beef burger made with different levels of Grewia tenax fruit dried-ground (GTF-DG) during refrigerated storage (4°C).
3.7. PCA analysis of GTF-DG burger
The GTF-DG-formulated burgers result in principal component analysis (PCA) and hierarchical clustering analysis (HCA) were conducted () to profoundly evaluate the combined influence of different concentrations of GTF-DG and storage intervals on the physicochemical, microbial, and sensory quality traits of formulated products. As the control (0% GTF-DG) containing burgers spoilage ≥14 days of storage and that of 2% GTF-DG containing burgers at day 21 of storage, control burgers at 14 and 21 days, and 2% GTF-DG containing burgers were excluded from the PCA and HCA. The PCA results () demonstrated high variability (81.51%) of plotted components with the highest contribution of the observed variability being seen for the first principal component (PC1, 68.53%). The correlations among the traits are shown in the biplot by the cosine of the angle between the vectors of the traits, with acute, obtuse, and right angles indicating positive, negative, and no correlations (Osman et al., Citation2022). In this regard, there were positive correlations between texture, color, cooking, and bioactive properties, as well as sensory attributes, and these were negatively correlated with total plate count, TBARS, and dimensional shrinkage, while the latter showed positive correlations between them. Clustering analysis displayed three divergent clusters based on the differences in formulated burgers as affected by the level of added GTF-DG and storage duration. The first group (black circle symbol) is composed of the control (0% GTF-DG-containing) burgers at 7 days of storage which is characterized by high microbial load, TBARS, and increased dimensional shrinkage suggesting less storage stability and rapid spoilage of control burgers. The second group (red diamond symbol) is composed of the burgers formulated with 0% GTF-DG at 0 day of storage, 2% GTF-DG at day 7 and 14 of storage, 4% GTF-DG at day 14 and 21 of storage, and 6% GTF-DG at day 21 of storage, which was characterized by high microbial load, TBARS, and dimensional shrinkage. Interestingly, increasing the incorporation level of GTF-DG in the burgers greatly delayed the spoilage as burgers with 0–2% GTF-DG showed high levels of microbial load, TBARS, and dimensional shrinkage at ≤14 days of storage, whereas those with 4–6% GTF-DG reached such high levels of these attributes at day 21 of storage. The third group (blue triangle symbol) contains the burgers articulated using 2% GTF-DG at day 0 of storage, 4% GTF-DG at days 0 and 7 of storage, and 6% GTF-DG at days 0, 7, and 14 of storage. Within this group, high sensory attributes were seen in cooked burgers formulated with 2% GTF-DG, high color attributes were found in raw burgers formulated with 4% GTF-DG at day 0 and 7 of storage, and improved texture, cooking, while bioactive properties were observed in burgers containing 6% GTF-DG at day 0–14% of storage. HCA was also made to extremely confirm the impacts of GTF-DG addition and subsequent storage conditions on the physicochemical traits of beef burgers and the results are shown in . In the HCA heatmap, the red color demonstrates high values and the green color indicates low values of the quality characteristics of beef burgers as influenced by the treatment and storage period. The phenotypic similarity of the physicochemical and sensory quality characteristics of the burgers as influenced by the treatment and storage is shown by the vertical branches of the treatments and the horizontal branches of the attributes. The upper branches of the control burgers (stored for 7 days) and GTF-DG-containing burgers stored for ≥14 days are characterized by low values of color, texture, sensory, and bioactive properties of the burgers, whereas they showed high values of dimensional shrinkage (DS), plate count, and TBARS. The highest values of DS, plate count, and TBARS were seen in control burgers stored for ≥7 days followed by that formulated with 4% GTF-DG at 21 days, 2% GTF-DG at 14 days, and 6% GTF-DG at 21 days suggesting that long storage negatively affected the stability of the burgers and this effect could be greatly diminished by increasing the concentration of GTF-DG in the patty formulation. The lower branches were composed of burgers formulated with different levels of GTF-DG and stowed for ≤14 days and these samples were considered by high levels of most physicochemical and sensory quality characteristics and low values of DS, plate count, and TBARS. Amongst these samples, the maximum levels of almost all attributes were seen in fresh burgers containing 6% GTF-DG, followed by fresh burgers containing 4% GTF-DG and 6% GTF-DG containing burgers stored for 7 days indicating that increasing the GTF-DG in the burgers formulation could improve the quality characteristics and storage stability of the product. Interestingly, it could be concluded that the incorporation of GTF-DG at (4–6%) in the burger formulations critically enhanced the physical, cooking, and bioactive quality attributes of the burgers and sustained these quality attributes during cold storage for up to 14 days.
Figure 1. (a) Principle component analysis (PCA) HJ-biplot and (b) heatmap of hierarchical clustering analysis (HCA) of the physicochemical and sensory attributes of beef burgers with different levels (0, 2, 4, and 6%) of Grewia tenax fruit dried-ground (GTF-DG) during refrigerated storage (4°C) for different periods (0, 7, 14, and 21 days). In the heatmap, the red color indicates high interrelation whereas the green color specifies low interrelations.
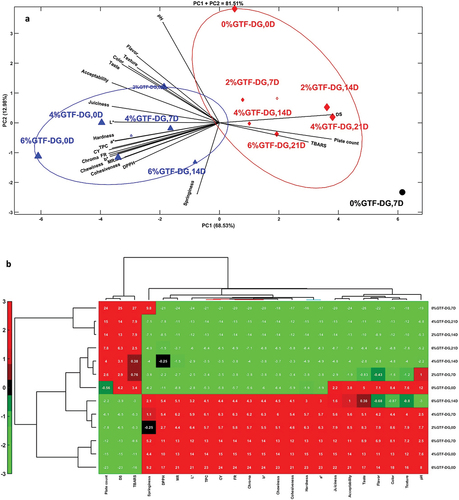
4. Conclusions
According to the findings, GTF-DG was rich in TP and high antioxidant activity. Incorporating 4 or 6% GTF-DG improved antioxidant activity, microbial stability, fat oxidation, and textural properties of the burgers. As a result, beef burgers with a GTF-DG content of 4% to 6% can work as an ingredient, prolonging storage time and improving nutritional quality and consumer acceptability of the product for up to 14 days without major effect on the sensory quality characteristics. Future studies could look into the nutritional characteristics of the burger and its impact on consumer health using animal experiments.
Disclosure statement
No potential conflict of interest was reported by the author(s).
Additional information
Funding
References
- Ahmed, I. A. M., Babiker, E. E., Al-Juhaimi, F. Y., & Bekhit, A. E. D. A. (2022). Clove polyphenolic compounds improve the microbiological status, lipid stability, and sensory attributes of beef burgers during cold storage. Antioxidants, 11(7), 1354. https://doi.org/10.3390/antiox11071354
- Al‐Juhaimi, F. Y., Almusallam, I. A., Mohamed Ahmed, I. A., Ghafoor, K., & Babiker, E. E. (2020). Potential of Acacia nilotica fruit flesh extract as an anti‐oxidative and anti‐microbial agent in beef burger. Journal of Food Processing and Preservation, 44(7), e14504. https://doi.org/10.1111/jfpp.14504
- Al-Juhaimi, F. Y., Babtain, I. A., Ahmed, I. A. M., Alsawmahi, O. N., Ghafoor, K., Adiamo, O. Q., & Babiker, E. E. (2020). Assessment of oxidative stability and physicochemical, microbiological, and sensory properties of beef patties formulated with baobab seed (adansonia digitata) extract. Meat Science, 162, 108044. https://doi.org/10.1016/j.meatsci.2019.108044
- Al-Juhaimi, F., Ghafoor, K., Hawashin, M. D., Alsawmahi, O. N., & Babiker, E. E. (2016). Effects of different levels of Moringa (Moringa oleifera) seed flour on quality attributes of beef burgers. CyTA-Journal of Food, 14(1), 1–10. https://doi.org/10.1080/19476337.2015.1034784
- Al-Juhaimi, F. Y., Shahzad, S. A., Ahmed, A. S., Adiamo, O. Q., Mohamed Ahmed, I. A., Alsawmahi, O. N., Ghafoor, K., & Babiker, E. E. (2018). Effect of Argel (Solenostemma argel) leaf extract on quality attributes of chicken meatballs during cold storage. Journal of Food Science and Technology, 55(5), 1797–1805. https://doi.org/10.1007/s13197-018-3094-1
- Amoli, P. I., Hadidi, M., Hasiri, Z., Rouhafza, A., Jelyani, A. Z., Hadian, Z., Khaneghah, A. M., & Lorenzo, J. M. (2021). Incorporation of low molecular weight chitosan in a low-fat beef burger: Assessment of technological quality and oxidative stability. Foods, 10(8), 1959. https://doi.org/10.3390/FOODS10081959
- Babiker, E. E., Al-Juhaimi, F. Y., Alqah, H. A., Adisa, A. R., Adiamo, O. Q., Mohamed Ahmed, I. A., Alsawmahi, O. N., Ghafoor, K., & Ozcan, M. M. (2019). The effect of Acacia nilotica seed extract on the physicochemical, microbiological and oxidative stability of chicken patties. Journal of Food Science and Technology, 56(8), 3910–3920. https://doi.org/10.1007/s13197-019-03862-y
- Das, A. K., Nanda, P. K., Chowdhury, N. R., Dandapat, P., Gagaoua, M., Chauhan, P., Pateiro, M., & Lorenzo, J. M. (2021). Application of pomegranate by-products in muscle foods: Oxidative indices, colour stability, shelf life and health benefits. Molecules, 26(2), 467. https://doi.org/10.3390/molecules26020467
- Domínguez, R., Pateiro, M., Gagaoua, M., Barba, F. J., Zhang, W., & Lorenzo, J. M. (2019). A comprehensive review on lipid oxidation in meat and meat products. Antioxidants, 8(10), 1–31. https://doi.org/10.3390/antiox8100429
- Efstratiou, E., Hussain, A. I., Nigam, P. S., Moore, J. E., Ayub, M. A., & Rao, J. R. (2012). Antimicrobial activity of Calendula officinalis petal extracts against fungi, as well as Gram-negative and Gram-positive clinical pathogens. Complementary Therapies in Clinical Practice, 18(3), 173–176. https://doi.org/10.1016/j.ctcp.2012.02.003
- Elrofaei, N. A., Elsharif, K. H., Elshikh, A. A., Bashir, M. E., Ahmed, I. F., Garbi, M. I., Kabbashi, A. S., & Saleh, M. S. (2018). Studies on antibacterial activity of some medicinal plants against selected bacterial strain. Journal of Antimicrobiological Agents, 4(2). https://doi.org/10.4172/2472-1212.1000170
- Ferreira, C. S. R., Saqueti, B. H. F., dos Santos, P. D. S., da Silva, J. M., Matiucci, M. A., Feihrmann, A. C., Graton Mikcha, J. M., & Santos, O. O. (2022). Effect of Salvia (Salvia officinalis) on the oxidative stability of salmon hamburgers. LWT-Food Science and Technology, 154, 112867. https://doi.org/10.1016/j.lwt.2021.112867
- Hadidi, M., Orellana-Palacios, J. C., Aghababaei, F., Gonzalez-Serrano, D. J., Moreno, A., & Lorenzo, J. M. (2022). Plant by-product antioxidants: Control of protein-lipid oxidation in meat and meat products. LWT-Food Science and Technology, 169, 114003. https://doi.org/10.1016/j.lwt.2022.114003
- Hamid, H. B. B., & Arabi, A. M. (2021). Impact of Grewia tenax (Guddaim) fruit supplementation on Serum Albumin and Serum Calcium among Khalwa pupils, Omdurman city, Sudan. Ahfad Journal, 38(1), 46–48.
- Hawashin, M. D., Al-Juhaimi, F., Ahmed, I. A. M., Ghafoor, K., & Babiker, E. E. (2016). Physicochemical, microbiological and sensory evaluation of beef patties incorporated with destoned olive cake powder. Meat Science, 122, 32–39. https://doi.org/10.1016/j.meatsci.2016.07.017
- Isra, A. M. A. (2020). Phenolic and flavonoid contents of Grewia tenax polar extracts. Journal Sebha University, 2020.
- Kalogianni, A. I., Lazou, T., Bossis, I., & Gelasakis, A. I. (2020). Natural phenolic compounds for the control of oxidation, bacterial spoilage, and foodborne pathogens in meat. Foods, 9(6), 794. https://doi.org/10.3390/foods9060794
- Kim, D. O., Jeong, S. W., & Lee, C. Y. (2003). Antioxidant capacity of phenolic phytochemicals from various cultivars of plums. Food Chemistry, 81(3), 321–326. https://doi.org/10.1016/S0308-8146(02)00423-5
- Lee, S. K., Mbwambo, Z. H., Chung, H., Luyengi, L., Gamez, E. J., Mehta, R. G., Kinghorn, A. D., & Pezzuto, J. M. (1998). Evaluation of the antioxidant potential of natural products. Combinatorial Chemistry & High Throughput Screening, 1(1), 35–46. https://doi.org/10.2174/138620730101220118151526
- Moosavy, M. H., Hassanzadeh, P., Mohammadzadeh, E., Mahmoudi, R., Khatibi, S. A., & Mardani, K. (2017). Antioxidant and antimicrobial activities of essential oil of Lemon (Citrus limon) peel in vitro and in a food model. Journal of Food Quality and Hazards Control, 4(2), 42–48.
- Munekata, P. E. S., Rocchetti, G., Pateiro, M., Lucini, L., Domínguez, R., & Lorenzo, J. M. (2020). Addition of plant extracts to meat and meat products to extend shelf-life and health-promoting attributes: An overview. Current Opinion in Food Science, 31, 81–87.
- Murphy, E. W., Criner, P. E., & Gray, B. C. (1975). Comparisons of methods for calculating retentions of nutrients in cooked foods. Journal of Agricultural and Food Chemistry, 23(6), 1153–1157. https://doi.org/10.1021/jf60202a021
- Osman, M. F. E., Mohamed, A. A., Ahmed, I. A. M., Alamri, M. S., Al Juhaimi, F. Y., Hussain, S., Ibraheem, M. A., & Qasem, A. A. (2022). Acetylated corn starch as a fat replacer: Effect on physiochemical, textural, and sensory attributes of beef patties during frozen storage. Food Chemistry, 388, 132988. https://doi.org/10.1016/j.foodchem.2022.132988
- Oyaizu, M. (1986). Studies on products of browning reaction antioxidative activities of products of browning reaction prepared from glucosamine. The Japanese Journal of Nutrition and Dietetics, 44(6), 307–315. https://doi.org/10.5264/eiyogakuzashi.44.307
- Qamar, M., Akhtar, S., Ismail, T., Wahid, M., Barnard, R. T., Esatbeyoglu, T., & Ziora, Z. M. (2021). The chemical composition and health-promoting effects of the Grewia species—A systematic review and meta-analysis. Nutrients, 13(12), 4565. https://doi.org/10.3390/nu13124565
- Re, R., Pellegrini, N., Proteggente, A., Pannala, A., Yang, M., & Rice-Evans, C. (1999). Antioxidant activity applying an improved ABTS radical cation decolorization assay. Free Radical Biology and Medicine, 26(9–10), 1231–1237. https://doi.org/10.1016/S0891-5849(98)00315-3
- Rosmini, M. R., Perlo, F., Pérez-Alvarez, J. A., Pagán-Moreno, M. J., Gago-Gago, A., López-Santoveña, F., & Aranda-Catalá, V. (1996). TBA test by an extractive method applied to ‘paté’. Meat Science, 42(1), 103–110. https://doi.org/10.1016/0309-1740(95)00010-0
- Sharma, N., & Patni, V. (2013). In vivo and in vitro qualitative phytochemical screening of Grewia species. International Journal of Biology and Pharmaceutical Research, 4(9), 634–639.
- Singleton, V. L., & Rossi, J. A. (1965). Colorimetry of total phenolics with phosphomolybdic-phosphotungstic acid reagents. American Journal of Enology and Viticulture, 16(3), 144–158. https://doi.org/10.5344/ajev.1965.16.3.144
- Suliman, Z. E., Zidan, N. S., & Foudah, S. H. (2018). Chemical compositions, antioxidant, and nutritional properties of the food products of Guddaim (Grewia tenax). International Journal of Pharmaceutical Research & Allied Sciences, 7(3), 172–182.
- Takó, M., Kerekes, E. B., Zambrano, C., Kotogán, A., Papp, T., Krisch, J., & Vágvölgyi, C. (2020). Plant phenolics and phenolic-enriched extracts as antimicrobial agents against food-contaminating microorganisms. Antioxidants, 9(2), 165. https://doi.org/10.3390/antiox9020165
- Vicente-Villardón, J. L. (2010). A package for multivariante analysis using biplots. Department of Statistics, University of Salamanca, University of Salamanca.
- Wang, X. H., Ren, H. Y., Liu, D. Y., Zhu, W. Y., & Wang, W. (2013). Effects of inoculating lactobacillus sakei starter cultures on the microbiological quality and nitrite depletion of Chinese fermented sausages. Food Control, 32(2), 591–596. https://doi.org/10.1016/j.foodcont.2013.01.050
- Zahid, M. A., Choi, J. Y., Seo, J. K., Parvin, R., Ko, J., & Yang, H. S. (2020). Effects of clove extract on oxidative stability and sensory attributes in cooked beef patties at refrigerated storage. Meat Science, 161, 107972. https://doi.org/10.1016/j.meatsci.2019.107972
- Zahid, M. A., Seo, J. K., Park, J. Y., Jeong, J. Y., Jin, S. K., Park, T. S., & Yang, H. S. (2018). The effects of natural antioxidants on protein oxidation, lipid oxidation, color, and sensory attributes of beef patties during cold storage at 4°C. Korean Journal for Food Science of Animal Resources, 38(5), 1029. https://doi.org/10.5851/kosfa.2018.e36