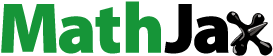
ABSTRACT
This study delves into the biological characteristics of Clinopodium nepeta subsp. ascendens (CNEO) essential oil, an indigenous subspecies of eastern Morocco. Distinguished by its purple or pale pinkish-white flowers, CNEO was subjected to thorough phytochemical analysis via gas chromatography-mass spectrometry (GC-MS), revealing 21 terpene compounds, notably Dihydrocarvone (22.70%), Neoisomenthol (43.59%), and Pulegone (20.55%). These compounds, recognized for their biological and pharmacological attributes, were further investigated for their potential applications. Notably, CNEO exhibited dose-dependent anti-inflammatory effects by attenuating nitric oxide (NO) and prostaglandin E2 (PGE2) production. Moreover, it demonstrated promising anticancer activity against MDA-MB-468, HepG2, and HCT-15 cancer cell lines, rivaling doxorubicin’s effectiveness. These findings underscore CNEO’s nutraceutical potential, particularly in food science and industry, suggesting its use as a functional food additive with both nutritional and health benefits
1. Introduction
The use of medicinal plants as a source of natural remedies is an ancient and universal practice rooted in the biomedical benefits and cultural beliefs of diverse populations (Leonti & Casu, Citation2013). In Morocco, traditional plant-based medicine possesses a rich historical and cultural heritage, offering effective solutions for the treatment of various ailments, although the therapeutic and chemical properties of the utilized plants have not been sufficiently explored from a scientific perspective (Bourhia et al., Citation2019; El-Mernissi et al., Citation2023). Among the traditional medicinal plants in Morocco, some stand out for their remarkable biological activities, potentially enhancing the health and well-being of local communities (Ait-Sidi-Brahim et al., Citation2019).
The use of plants for agri-food purposes is also an area of growing interest. Their aromatic properties and potential health benefits make them interesting additives for a variety of food products. Essential oils, extracted from aromatic and medicinal plants, are volatile compounds renowned for their chemical complexity and diverse biological properties (Dhifi et al., Citation2016). Their composition varies according to the plant species of origin, but they generally contain a range of active compounds such as terpenes, phenols and ketones (Noriega, Citation2020). They are often used as natural flavoring agents in the food industry, offering an alternative to artificial flavors and adding a distinctive flavor to finished products (Bakkali et al., Citation2008).
The exploration of natural products for their therapeutic and dietary potential, as well as their health benefits, has been a cornerstone in the development of new eco-friendly treatments for various diseases. Essential oils, in particular, have attracted attention for their diverse pharmacological activities, including anti-inflammatory and anti-cancer properties (Taibi, Elbouzidi, Ouahhoud, et al., Citation2023). Their use as food additives in the food industry offers a natural alternative to synthetic chemicals, while adding distinctive flavours and health benefits to finished products (Grigoriadou et al., Citation2023; Taban et al., Citation2021).
Preservatives and chemical additives are associated with adverse health effects, and their use may contribute to the incidence of cancer (Javanmardi et al., Citation2019; Mirza et al., Citation2017). Studies have highlighted the link between exposure to these substances and the development of various types of cancer. The underlying mechanisms often involve carcinogenesis processes linked to toxicity and disruption of normal biological processes (Dybing et al., Citation2002; Xu et al., Citation2021).
Cancer remains one of the leading causes of mortality worldwide, and the development of new anti-cancer agents is of paramount importance (Burrell & Swanton, Citation2014). In Morocco, the prevalence of cancer is on the rise, particularly for breast, liver, and colon cancers, which are among the most frequent and deadly (Khalis et al., Citation2021). Inflammation is a complex biological response to harmful stimuli, such as pathogens, damaged cells, or irritants (Nathan, Citation2002). Chronic inflammation is known to play a significant role in the pathogenesis of various diseases, including cancer, arthritis, and cardiovascular diseases (Medzhitov, Citation2010). The search for effective anti-inflammatory agents is crucial in managing these conditions. Given this scenario, the exploration of novel plant-derived anticancer and anti-inflammatory agents emerges as a promising avenue.
In this context, the present study focuses on the essential oil composition of a Clinopodium nepeta subspecies in the Eastern region of Morocco. The studied subspecies, C. nepeta subsp. ascendens, is distinguished by its purple or pale pinkish-white flowers, measuring 8–10 mm in length. The calyx slightly swells at the base when mature (Quézel & Santa, Citation1962). This plant is employed in traditional Moroccan medicine for treating various ailments, such as digestive disorders, respiratory infections, rheumatism, and wounds. C. nepeta exhibits remarkable chemical diversity and significant within-species variation. However, scientific data on the biological activities of this plant are limited and fragmented. Therefore, the objective of this study is to assess the anti-inflammatory effects of the essential oil of C. nepeta subsp. ascendens (CNEO), particularly in the context of (LPS)-induced inflammation, a commonly used experimental model for studying inflammatory processes (Gao et al., Citation2021). In addition, this study evaluates the anti-cancer activity of the essential oil against three representative cell lines, namely breast cancer (MDA-MB-468), hepatocellular carcinoma (HepG2) and colon cancer (HCT-15). The development of natural food additives is primarily aimed at avoiding the use of chemical additives with adverse health effects, while capitalizing on the potential benefits of essential oil to promote health and dietary well-being. This approach is based on a rigorous methodology, including exhaustive phytochemical analyses of CNEO to characterize its active compounds. These analyses seek to identify the main constituents responsible for this medicinal plant’s potential anti-inflammatory and anti-cancer properties. The results of this study could not only enrich Morocco’s medicinal and industrial heritage, but also open up new prospects for the development of innovative natural therapies and dietary bio-additives in the fight against inflammation and cancer.
2. Materials and methods
2.1. Plant material
The investigation was centered on Clinopodium nepeta subsp. ascendens, a species indigenous to the Ahfir province situated in the northeastern region of Morocco. The aerial components of the plant, including leaves and flowers, were systematically procured from a local market during the spring of 2022 and subsequently dispatched to the Faculty of Sciences at Université Mohammed 1er d’Oujda. The primary objective of this botanical study was to subject the specimens to rigorous taxonomic identification, a critical step aimed at ensure precise categorization of the various plant species under consideration. It’s important to note that the collection intentionally encompassed both vegetative and reproductive structures of the plant. This comprehensive approach was designed to facilitate a thorough taxonomic identification, considering not only the vegetative morphology but also the floral characteristics of Clinopodium nepeta subsp. ascendens.
2.2. Essential oil extraction
Essential oil extraction was carried out from the Clinopodium nepeta sp. ascendens aerial part using the hydrodistillation method with a modified Clevenger configuration. This technique was chosen to preserve the volatile components of the essential oil, in line with the methodology described by (Taibi, Elbouzidi, Haddou, Loukili, et al., Citation2024; Taibi, Elbouzidi, Ou-Yahia, et al., Citation2023; Taibi, Elbouzidi, Ouahhoud, et al., Citation2023).
2.3. Phytochemical composition by GC-MS
A Shimadzu GC system, coupled with an MS QP2010 (Kyoto, Japan) was used to analyze the chemical composition of Clinopodium nepeta subsp. ascendens essential oil. The setup included a RTX-5 capillary column phase (30 m × 0.25 mm inner diameter × 0.25 µm film thickness) with pure helium gas (99.99%) as the carrier. Injection, ion source, and interface temperatures were maintained at 250°C. Sample components were ionized in the EI mode (70 ev), and data were collected in the mass range of 40–300 m/z. Compound identification relied on comparing retention times and fragmentation patterns with authenticated standards and databases such as NIST 147. Data collection and processing were performed using Laboratory Solutions software (v2.5) (Haddou et al., Citation2023; Loukili et al., Citation2023). This comprehensive analytical approach facilitates a comprehensive exploration of the phytochemical composition inherent in this essential oil. By establishing a detailed understanding of the chemical constituents present, this analysis significantly contributes to unraveling the nuanced properties and potential applications of CNEO within the academic realm of botanical and pharmaceutical research.
2.4. Anti-inflammatory activity
2.4.1. Cell culture and cell viability assay
RAW 264.7 murine macrophage cells utilized in this investigation were sourced from the American Type Culture Collection (ATCC, Manassas, VA, U.S.A.). Adhering to ATCC’s recommended guidelines, the cells were cultured at 37°C in Dulbecco’s Modified Eagle Medium (DMEM), supplemented with 10% fetal bovine serum (FBS), 4.5 g/L glucose, 4.5 mM glutamine, 100 units/mL penicillin, and 100 μg/mL streptomycin. Cell viability was assessed using an MTT (3-(4,5-dimethylthiazol-2-yl)-2,5-diphenyltetrazolium bromide) colorimetric assay. Initially, RAW 264.7 cells were seeded in 96-well culture plates at a density of 1 × 104 cells per well per well (Lin et al., Citation2023). Subsequently, the cells underwent treatment with varying concentrations of CNEO (25–200 µg/mL) in the presence or absence of lipopolysaccharide (LPS) at a concentration of 1 μg/mL for a duration of 24 hours. After the treatment period, The culture medium was removed by aspiration, and the cells were subsequently incubated with MTT solution (10 µg/mL) in 200 µL of fresh DMEM for 1 hour at 37°C. Following incubation, the resulting violet formazan crystals produced through MTT metabolism were dissolved in 200 μL of dimethyl sulfoxide (DMSO). Subsequently, absorbance was measured at 570 nm (A570), with a background control reading taken at 630 nm (A630), using an ELISA microplate reader. This standardized methodology facilitated the quantitative determination of cell viability, offering crucial insights into the potential impact of CNEO on the survival of RAW 264.7 macrophage cells under inflammatory conditions induced by LPS. Cell viability was calculated as follows:
2.4.2. Determination of NO and PGE2
To assess nitric oxide (NO) levels in the culture media, we employed the Griess reaction assay, a well-established method described in previous protocols (Kumar et al., Citation2015; Taibi, Elbouzidi, Haddou, Baraich, et al., Citation2024). RAW 264.7 cells were seeded at a density of 1 × 104 cells per well per well in a 96-well culture plate. Subsequently, the cells were treated with lipopolysaccharide (LPS), either in the presence or absence of varying concentrations of CNEO (25–100 μg/mL), for a duration of 24 hours. After the treatment period, equivalent volumes of culture supernatants were combined with Griess reagent and incubated at room temperature for 30 minutes. The resulting intracellular nitrate levels, a principal stable product of NO, were quantified using an ELISA microplate reader set at 540 nm (microQuant, Bio-Tek Instruments, Winooski, VA, U.S.A.). This quantitative analysis provided valuable insights into how RAW 264.7 macrophage cells modulate NO production in response to CNEO under inflammatory conditions induced by LPS. Concurrently, the intracellular levels of prostaglandin E2 (PGE2) were assessed utilizing an enzyme immunoassay (EIA) kit obtained from R&D Systems (Minneapolis, MN, U.S.A.), following the manufacturer’s protocols. This comprehensive approach facilitated the assessment of both NO and PGE2, which serve as pivotal mediators in the inflammatory response. The outcomes offer a thorough understanding of CNEO’s impact on these crucial signaling molecules, contributing to the broader comprehension of the oil’s potential anti-inflammatory properties.
2.5. Anticancer activity
2.5.1. Cell viability by MTT assay
In accordance with the methodological framework delineated in references (Chaudhary et al., Citation2015; Elbouzidi et al., Citation2022), the MTT (3-(4,5-dimethylthiazol-2-yl)-2,5-diphenyltetrazolium bromide) assay was implemented to ascertain the potential impact of CNEO on impeding the proliferation of cancerous cells. The experimental procedure unfolded in a systematic manner: Initially, exponentially proliferating cell lines comprising HepG2, MDA-MB-468, and HCT-15 were meticulously seeded into 96-well plates, each accommodating 10,000 cells suspended in 100 µL of growth medium. A crucial incubation period of 24 hours was observed to facilitate cellular adhesion and establish a conducive microenvironment. Subsequently, meticulous preparations of CNEO solutions were conducted. The CNEO samples, solubilized in 0.1% DMSO (Dimethyl sulfoxide), underwent serial dilution with growth medium to generate a diverse range of concentrations, thus enabling a comprehensive dose-dependent analysis. Following the preparation of CNEO solutions, the respective cell cultures were subjected to varying dosages of CNEO and subsequently incubated for a prescribed duration of 72 hours. This temporal window allowed for the manifestation and discernment of any inhibitory effects exerted by CNEO on cell proliferation. A meticulously established negative control group, receiving treatment solely with growth medium supplemented with 0.1% DMSO, served as a baseline reference to discern any cellular responses solely attributed to CNEO treatment. Upon the completion of the designated incubation period, the MTT assay was employed to evaluate cellular viability and metabolic activity. This entailed replacing the culture medium with 200 µL of fresh medium, followed by the addition of 20 µL of MTT reagent (comprising 5 mg/mL MTT in PBS) to each well. The subsequent 4-hour incubation period at 37°C facilitated the conversion of MTT to formazan crystals, indicative of viable cell populations. Post-incubation, the medium was aspirated, and 100 µL of DMSO was added to solubilize the formazan crystals. Employing a microplate reader (Synergy HT Multi-Detection microplate reader, Bio-Tek, Winooski, VT, U.S.A.), the absorbance of the resultant solution was measured at 540 nm, enabling the quantification of cell viability. Cell viability percentages were computed utilizing the formula (Mosmann, Citation1983).
where At is the absorbance of cells treated with CNEO at various doses, and Ao is the absorbance of cells treated with 0.1% DMSO media. To ensure the robustness and reliability of the experimental findings, triplicate trials were conducted for each treatment condition, with doxorubicin serving as the designated positive control for comparative analysis. The determination of half-maximal inhibitory concentration (IC50) values was executed through the construction of dose-response inhibition curves, facilitated by GraphPad Prism 8.01 software.
Additionally, to assess the potential cytotoxic effects of CNEO on peripheral blood mononuclear cells (PBMCs), these cells were isolated from human blood samples via Ficoll-Hypaque density centrifugation, adhering strictly to the manufacturer’s stipulated protocols (Capricorn Scientific).
3. Results and discussion
3.1. Phytochemical composition
The constituents of the essential oil are listed in the order of their elution on the RTX-5 capillary column ( and ). Analysis of the chemical composition of the essential oil reveals the presence of 21 compounds, some of which show significant percentages. The three main compounds are neoisomenthol, representing a preponderant share of 43.59%, followed by dihydrocarvone with 22.70%, and finally pulegone with 20.55%. This distribution highlights the chemical richness of the essential oil, with each compound contributing its own distinctive characteristics. It also contains smaller quantities of 2,3-Pinanediol (3.70%) and menthone (2.98%). Within this complexity, the oxygenated monoterpenes category stands out for its abundance, representing 95.27% of the total compounds identified. Furthermore, the constituents listed in have been classified based on their chemical structure and propreties. Each compound’s molecular weight and type have been included, allowing a more comprehensive understanding of the chemical composition.The abundance of terpene compounds in CNEO suggests a potential synergy of health benefits, including relaxation, anti-inflammation and anti-cancer (Beddiar et al., Citation2021).These bioactive molecules may play an essential role in the various biological activities of this aromatic and medicinal plant. The complex interaction of these compounds could be behind the plant’s pharmacological effects, which include antimicrobial, anti-inflammatory and even anti-cancer properties (Rodenak-Kladniew et al., Citation2023; Tomko et al., Citation2020).
Table 1. Phytochemical constituents of CNEO.
Several studies have been carried out on essential oil extracted from C. nepeta (Beddiar et al., Citation2021; Benkhaira et al., Citation2023; Debbabi et al., Citation2020; Elbe et al., Citation2020; Öztürk et al., Citation2021). To the best of our knowledge, the present study marks the first investigation into essential oil extracted from the Moroccan subspecies C. nepeta subsp. ascendens. The results obtained differ from those reported by Öztürk et al. (Citation2021) for oil extracted from C. nepeta subsp. glandulosum, who identified piperitone oxide (47.8%), limonene (18.6%) and piperitone oxide II (13.6%) as the major components (Öztürk et al., Citation2021). These variations in chemical composition can be attributed to a number of factors, including subspecies, environmental factors, the vegetative cycle, plant age, and even genetic factors (El Guerrouj et al., Citation2023; Massad et al., Citation2011).
The identification of phytochemical composition paves the way for further exploration of the biological properties, as well as the nutraceutical implications of these specific compounds. This detailed analysis provides a better understanding of the mechanisms of action of the various constituents present in the essential oil, which could lead to the discovery of new therapeutic and nutritional applications. By closely examining the chemical structure of compounds, it is possible to determine their potential pharmacological activity and assess their efficacy in the treatment of various ailments. This approach opens up the possibility of fully exploiting the benefits of the natural compounds present in essential oil to improve human health and well-being, whether as a food dietary supplement.
3.2. Anti-inflammatory activity
First and foremost, evaluating cell viability in lipopolysaccharide (LPS)-activated RAW 264.7 cells is a pivotal step in comprehending the influence of CNEO on the inflammatory response. The data illustrated in compellingly indicate that escalating concentrations of CNEO preserved cell viability and suggested a propensity for cell protection compared to the group treated solely with LPS. These findings underscore the capacity of CNEO to modulate cellular responses within an inflammatory milieu. This study seeks to appraise the anti-inflammatory potential of CNEO by influencing the production of inflammatory mediators, notably nitric oxide (NO) and prostaglandin E2 (PGE2). It is crucial to highlight the significance of NO and PGE2 in the anti-inflammatory process, where their inhibition assumes a pivotal role in regulating inflammatory responses. NO, an essential regulator of inflammation-related cellular cascades, and PGE2, closely associated with mediating inflammatory responses and sensitizing nociceptors, emphasize the importance of targeting these mediators. The ability of CNEO to dose-dependently inhibit the production of these inflammatory mediators suggests promising prospects in the realm of anti-inflammation, laying the foundation for innovative therapeutic strategies.
Figure 2. Impact of CNEO on the cell viability of RAW 264.7 cells under stimulation with LPS (1 μg/mL) and incubation in the presence or absence of escalating concentrations (12.5–200 µg/mL. Of CNEO for a duration of 24 hours. Cell Viability was assessed utilizing the MTT colorimetric assay. A significance level of p < .05 signifies a statistically significant difference between the control and LPS-Only treated groups.
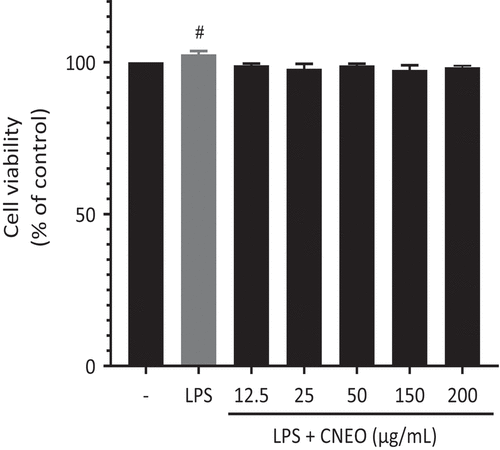
The results in expound upon the concentrations measured under specific experimental conditions. delineates that the control group manifests a basal NO production of 4,400 ± 0.43 µM while introducing lipopolysaccharide (LPS) induces a substantial increase to 49.04 ± 2.22 µM. The simultaneous addition of CNEO, spanning concentrations from 25 to 200 µg/mL with LPS (1 µg/mL), demonstrates a dose-dependent reduction in NO production, ranging between 37.56 ± 0.87 and 14.80 ± 1.80 µM. Analogously, in , the control group exhibits a PGE2 production of 54.20 ± 2.77 pg/mL, while LPS alone significantly amplifies this production to 657.1 ± 18.60 pg/mL. The introduction of CNEO at varying concentrations induces a dose-dependent decline in PGE2 production, ranging from 504.2 ± 5.37 to 93.47 ± 10.74 pg/mL.
Figure 3. Impact of CNEO on the production of nitric oxide (NO) and prostaglandin E2 (PGE2) induced by LPS. (a) RAW 264.7 cells were stimulated with LPS (1 μg/mL) and cultured with escalating concentrations (25–200 µg/mL) of CNEO for a duration of 24 hours. The Griess reagent assay was employed to assess the nitrite concentration in the culture media. (b) PGE2 levels in the culture media were quantified using a commercially available assay kit, following the outlined methods in the materials. The data presented represent the mean ± SD of three independent experiments. The symbol # (p < .001) denotes a significant difference between the control and LPS-only treated groups. Additionally, ** (p < .01) and *** (p < .001) indicate noteworthy distinctions between the LPS-alone and CNEO treatment groups.
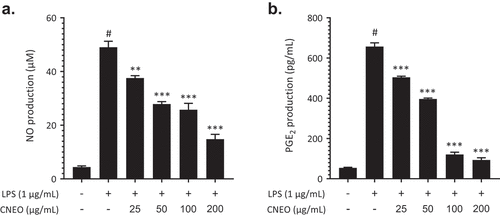
These outcomes posit that CNEO harbors anti-inflammatory activity, exemplifying a dose-dependent efficacy in attenuating LPS-induced NO and PGE2 production. These findings underscore the advantageous potential of CNEO in modulating inflammatory processes. The assays reveal a dose-dependent decrease in NO and PGE2 production in the presence of CNEO, thereby illustrating its capacity to modulate inflammatory responses. Notably, the 200 µg/mL CNEO concentration significantly diminishes NO and PGE2 production compared to the group stimulated solely by LPS.
This remarkable activity is probably attributed to bioactive compounds, with pulegone and neoisomenthol appearing as the predominant constituents of this essential oil. These components play a central role in the anti-inflammatory activity demonstrated by the essential oils containing them, providing further evidence in support of the hypothesis that species producing these compounds have potential as effective anti-inflammatory agents (Roy et al., Citation2018; Zhao et al., Citation2022). Our study stands out for observing a remarkable effect of this activity, emphasizing the uniqueness of the chemical composition of our essential oil compared to other nepeta extrac (Benkhaira et al., Citation2023; Öztürk et al., Citation2021; Rodenak-Kladniew et al., Citation2023). Despite the absence of previous studies reporting significant effects of this essential oil as an anti-inflammatory agent, our results highlight a unique and promising prospect that deserves further exploration.
It should be noted that essential oils in general are recognized for their broad antioxidant and anti-inflammatory properties (Miguel, Citation2010; Pérez et al., Citation2011). These results suggest that essential oils may represent a natural alternative in the agri-food and pharmaceutical sectors, offering interesting possibilities for the development of food products/supplements with health benefits.
3.3. Anticancer activity
The assessment of Clinopodium nepeta subsp. ascendens essential oil’s (CNEO) anticancer efficacy on three distinct cell lines – MDA-MB-468 (representing triple-negative breast cancer), HepG2 (a hepatocellular carcinoma model), and HCT-15 (a representative of colorectal cancer) – along with mononuclear blood cells (PBMC) has yielded promising outcomes. (Selected cell lines are well-established models, each emblematic of frequently encountered cancers). MDA-MB-468’s triple-negative status, marked by the absence of estrogen, progesterone, and human epidermal growth factor 2 (HER2) receptors, underscores its heightened aggressiveness. HepG2, derived from hepatocellular carcinoma, typifies a form of liver cancer often associated with an unfavorable prognosis. HCT-15 serves as a representative model for colorectal cancer, a prevalent malignancy affecting the colon or rectum. The obtained results indicate a significant anticancer activity of CNEO against these specific cancer cell lines, as evidenced by IC50 values of 25.31 ± 1.32, 35.95 ± 1.33, and 38.70 ± 2.41 µg/mL, respectively (). Particularly noteworthy is the observed selectivity towards cancer cells compared to healthy PBMC (IC50 = 735.3 ± 8.10 µg/mL). In comparison, the positive control treatment with doxorubicin, a widely used anticancer agent, demonstrated lower IC50 values for MDA-MB-468, HepG2, and HCT-15, signifying substantial efficacy of the reference drug. Intriguingly, CNEO exhibited selectivity indices (29.08 ± 1.34, 20.45 ± 1.15, and 20.00 ± 2.42 for MDA-MB-468, HepG2, and HCT-15, respectively) comparable to or even higher than those of doxorubicin (11.17 ± 3.30, 18.21 ± 7.11, and 13.70 ± 4.37, respectively) (). These selectivity indices underscore CNEO’s ability to selectively target cancer cells while minimizing impact on healthy cells. These findings suggest that CNEO possesses significant anticancer activity, rivaling or potentially surpassing that of the positive doxorubicin treatment, with a notable degree of selectivity towards cancer cells.
Figure 4. Cell viability of MDA-MB-468, HepG2, HCT-15, and PBMC cells after 72 h of treatment with C. nepeta subsp. ascendens essential oil (CNEO) (a), and doxorubicin (positive control, (b)) using MTT test. IC50 values of CNEO (c), and doxorubicin (d) against the studied cell lines. IC50 are obtained from three independent experiments and expressed as means ± SD. a, b, c letters indicate a significant difference at p < .05.
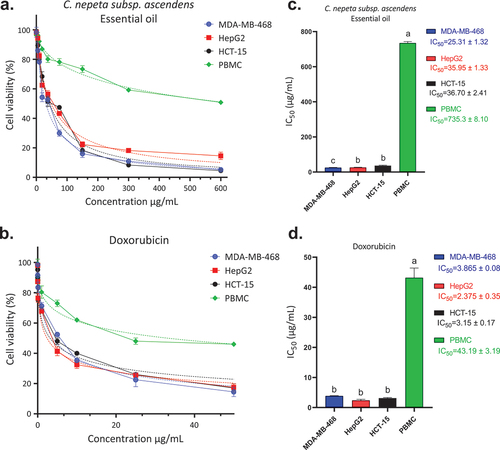
Table 2. Selectivity indexes of C. nepeta subsp. ascendens essential oil (CNEO) on cancer cell lines (MDA-MB-468, HepG2, and HCT-15).
The remarkable activity of CNEO can be attributed to its main components, Neoisomenthol and Pulegone, which have demonstrated considerable cytotoxic potential against various cancer cell lines (Fatima et al., Citation2021Boni et al., Citation2016). These outcomes validate the conclusions of Rodenak-Kladniew et al. (Citation2023), underscoring the efficacy of this Argentine essential oil against diverse cancer cell lines. This convergence enhances the credibility of the findings concerning CNEO’s anticancer activity, presenting promising prospects for its potential application as a therapeutic agent against various cancer types (Rodenak-Kladniew et al., Citation2023).
The inclusion of various cell lines representative of common human cancers reinforces the applicability of these results, underlining the potential of CNEO as a promising anticancer agent. This suggests that CNEO could be used for pharmaceutical purposes and in the food industry as a food additive, offering a dual advantage. On the one hand, it could be used for its aromatic properties, and on the other, it could be incorporated for its health benefits, or even as a dietary supplement.
4. Conclusions
This comprehensive investigation delved into the analysis of the essential oil derived from the Moroccan subspecies C. nepeta subsp. ascendens, elucidating a composition abundant in terpene compounds, notably Neoisomenthol and Pulegone. The dose-dependent modulation of inflammatory responses accentuated CNEO’s potential as an anti-inflammatory agent. Examination of its anticancer activity disclosed substantial efficacy and selectivity against cancer cells, positioning CNEO as a potential and discerningly selective anticancer agent. These auspicious findings not only contribute to elucidating the potential applications of CNEO, but also advocate for sustained research efforts to harness its therapeutic potential across diverse realms of health. The investigated biological properties of this essential oil could be of a great interest in the agri-food sector particularly in food preservation and processing. Nevertheless, it is essential to proceed with caution and conduct thorough toxicological and clinical investigations before widespread adoption in the agri-food sector.
Credit authorship contribution statement
Mohamed Taibi: Conceptualization, original draft writing, reviewing, and editing. Amine Elbouzidi: Conceptualization, original draft writing, reviewing, and editing. Mounir Haddou: Conceptualization, original draft writing, reviewing, and editing. Yousra Belbachir: Conceptualization, original draft writing, reviewing, and editing. Abdellah Baraich: Conceptualization, original draft writing, reviewing, and editing. Zineb Khalil: Original draft writing, reviewing, and editing. Reda Bellaouchi: Formal analysis, investigations, funding acquisition, reviewing, and editing. Abdeslam Asehraou: Formal analysis, investigations, funding acquisition, reviewing, and editing. Mohamed Addi: Formal analysis, investigations, funding acquisition, reviewing, and editing. Ammar AL-Farga: Resources, data validation, and data curation. Saleh M. Al-Maaqar: Resources, data validation, an data curation. Bouchra El Guerrouj: Resources, data validation, data curation, and supervision. Khalid Chaabane: Resources, data validation, data curation, and supervision.
Acknowledgments
The authors express their gratitude to the Moroccan Ministry of Higher Education, Scientific Research and Innovation, and the European Union.
Disclosure statement
No potential conflict of interest was reported by the author(s).
Data availability statement
Data will be available upon request from the corresponding author.
References
- Ait-Sidi-Brahim, M., Markouk, M., & Larhsini, M. (2019). Moroccan medicinal plants as antiinfective and antioxidant agents. In M. S. A. Khan, I. Ahmad & D. Chattopadhyay (Eds.), New look to phytomedicine (pp. 91–9). Elsevier.
- Bakkali, F., Averbeck, S., Averbeck, D., & Idaomar, M. (2008). Biological effects of essential oils–a review. Food and Chemical Toxicology, 46(2), 446–475. https://doi.org/10.1016/j.fct.2007.09.106
- Beddiar, H., Boudiba, S., Benahmed, M., Tamfu, A. N., Ceylan, Ö., Hanini, K., Kucukaydin, S., Elomri, A., Bensouici, C., & Laouer, H. (2021). Chemical composition, anti-quorum sensing, enzyme inhibitory, and antioxidant properties of phenolic extracts of Clinopodium nepeta L. Kuntze. Plants, 10(9), 1955. https://doi.org/10.3390/plants10091955
- Benkhaira, N., El Hachlafi, N., Abdnim, M., Jeddi, R., Koraichi, M., Bnouham, S. I., & Fikri-Benbrahim, K. (2023). Unveiling the phytochemical profile, in vitro bioactivities evaluation, in silico molecular docking and ADMET study of essential oil from Clinopodium nepeta grown in Middle Atlas of Morocco. Biocatalysis and Agricultural Biotechnology, 54, 102923. https://doi.org/10.1016/j.bcab.2023.102923
- Boni, G. C., de Feiria, S. N. B., de Laet Santana, P., Anibal, P. C., Boriollo, M. F. G., Buso-Ramos, M. M., Barbosa, J. P., de Oliveira, T. R., & Hofling, J. F. (2016). Antifungal and cytotoxic activity of purified biocomponents as carvone, menthone, menthofuran and pulegone from Mentha spp. African Journal of Plant Science, 10(10), 203–210. https://doi.org/10.5897/AJPS2016.1454
- Bourhia, M., Abdelaziz Shahat, A., Mohammed Almarfadi, O., Ali Naser, F., Mostafa Abdelmageed, W., Ait Haj Said, A., El Gueddari, F., Naamane, A., Benbacer, L., & Khlil, N. (2019). Ethnopharmacological survey of herbal remedies used for the treatment of cancer in the greater Casablanca-Morocco. Evidence-Based Complementary and Alternative Medicine, 2019, 1–9. https://doi.org/10.1155/2019/1613457
- Burrell, R. A., & Swanton, C. (2014). Tumour heterogeneity and the evolution of polyclonal drug resistance. Molecular Oncology, 8(6), 1095–1111. https://doi.org/10.1016/j.molonc.2014.06.005
- Chaudhary, S., Chandrashekar, K. S., Pai, K. S. R., Setty, M. M., Devkar, R. A., Reddy, N. D., & Shoja, M. H. (2015). Evaluation of antioxidant and anticancer activity of extract and fractions of nardostachys jatamansi DC in breast carcinoma. BMC Complementary and Alternative Medicine, 15(1), 1–13. https://doi.org/10.1186/s12906-015-0563-1
- Debbabi, H., El Mokni, R., Chaieb, I., Nardoni, S., Maggi, F., Caprioli, G., & Hammami, S. (2020). Chemical composition, antifungal and insecticidal activities of the essential oils from Tunisian Clinopodium nepeta subsp. nepeta and Clinopodium nepeta subsp. glandulosum. Molecules, 25(9), 2137. https://doi.org/10.3390/molecules25092137
- Dhifi, W., Bellili, S., Jazi, S., Bahloul, N., & Mnif, W. (2016). Essential oils’ chemical characterization and investigation of some biological activities: A critical review. Medicines, 3(4), 25. https://doi.org/10.3390/medicines3040025
- Dybing, E., Doe, J., Groten, J., Kleiner, J., O’brien, J., Renwick, A. G., Schlatter, J., Steinberg, P., Tritscher, A., Walker, R., & Younes, M. (2002). Hazard characterisation of chemicals in food and diet: Dose response, mechanisms and extrapolation issues. Food and Chemical Toxicology, 40(2–3), 237–282. https://doi.org/10.1016/S0278-6915(01)00115-6
- Elbe, H., Yigitturk, G., Cavusoglu, T., Uyanikgil, Y., & Ozturk, F. (2020). Apoptotic effects of thymol, a novel monoterpene phenol, on different types of cancer. Bratislava Medical Journal/Bratislavske Lekarske Listy, 121(2), 122–128. https://doi.org/10.4149/BLL_2020_016
- Elbouzidi, A., Ouassou, H., Aherkou, M., Kharchoufa, L., Meskali, N., Baraich, A., Mechchate, H., Bouhrim, M., Idir, A., Hano, C., Zrouri, H., & Addi, M. (2022). LC–MS/MS phytochemical profiling, antioxidant activity, and cytotoxicity of the ethanolic extract of atriplex halimus L. against breast cancer cell lines: Computational studies and experimental validation. Pharmaceuticals, 15(9), 1156. https://doi.org/10.3390/ph15091156
- El Guerrouj, B., Taibi, M., Elbouzidi, A., Bouhassoun, S., Loukili, E. H., Moubchir, T., Haddou, M., Hammouti, Y., Khoulati, A., & Addi, M. (2023). The effect of altitude on the chemical composition, antioxidant and antimicrobial activities of eucalyptus globulus Labill. Essential oils. Tropical Journal of Natural Product Research, 7(11), 5279–5285.
- El-Mernissi, Y., Zouhri, A., Labhar, A., El Menyiy, N., Salhi, S., El Barkany, A., Hajji, A., Bouyahya, L., & Amhamdi, H. (2023). Indigenous knowledge of the traditional use of aromatic and medicinal plants in Rif Mountains Ketama District. Evidence-Based Complementary and Alternative Medicine, 2023, 1–16. https://doi.org/10.1155/2023/3977622
- Fatima, K., Masood, N., Wani, Z. A., Meena, A., & Luqman, S. (2021). Neomenthol prevents the proliferation of skin cancer cells by restraining tubulin polymerization and hyaluronidase activity. Journal of Advanced Research, 34, 93–107. https://doi.org/10.1016/j.jare.2021.06.003
- Gao, H., Yang, T., Chen, X., & Song, Y. (2021). Changes of lipopolysaccharide-induced acute kidney and liver injuries in rats based on metabolomics analysis. Journal of Inflammation Research, 14, 1807. https://doi.org/10.2147/JIR.S306789
- Grigoriadou, K., Cheilari, A., Dina, E., Alexandri, S., & Aligiannis, N. (2023). Medicinal and aromatic plants as a source of potential feed and food additives. In G. Arsenos & I. Giannenas Eds.), Sustainable use of feed additives in livestock: Novel ways for animal production (pp. 117–135). Springer.
- Haddou, M., Taibi, M., Elbouzidi, A., Loukili, E. H., Yahyaoui, M. I., Ou-Yahia, D., Mehane, L., Addi, M., Asehraou, A., Chaabane, K., Bellaouchi, R., & El Guerrouj, B. (2023). Investigating the impact of irrigation water quality on secondary metabolites and chemical profile of mentha piperita essential oil: Analytical profiling, characterization, and potential pharmacological applications. International Journal of Plant Biology, 14(3), 638–657. https://doi.org/10.3390/ijpb14030049
- Javanmardi, F., Rahmani, J., Ghiasi, F., Hashemi Gahruie, H., & Mousavi Khaneghah, A. (2019). The association between the preservative agents in foods and the risk of breast cancer. Nutrition and Cancer, 71(8), 1229–1240. https://doi.org/10.1080/01635581.2019.1608266
- Khalis, M., Diaby, M., Toure, A. B., Charaka, H., Abousselham, L., Belakhel, L., Bendahhou, K., Al Hassani, W., Soliman, A. S., & Nejjari, C. (2021). The past, present, and future of professional cancer education in Morocco. Journal of Cancer Education, 36(Suppl 1), 95–100. https://doi.org/10.1007/s13187-021-02039-6
- Kumar, K. J. S., Li, J., Vani, M. G., Hsieh, Y.-H., Kuo, Y.-H., & Wang, S.-Y. (2015). Bornyl cinnamate inhibits inflammation-associated gene expression in macrophage cells through suppression of nuclear factor-κB signaling pathway. Planta Medica, 81(1), 39–45. https://doi.org/10.1055/s-0034-1383361
- Leonti, M., & Casu, L. (2013). Traditional medicines and globalization: Current and future perspectives in ethnopharmacology. Frontiers in Pharmacology, 4, 92. https://doi.org/10.3389/fphar.2013.00092
- Lin, W.-T., He, Y.-H., Lo, Y.-H., Chiang, Y.-T., Wang, S.-Y., Bezirganoglu, I., & Kumar, K. J. S. (2023). Essential oil from glossogyne tenuifolia inhibits lipopolysaccharide-induced inflammation-associated genes in macro-phage cells via suppression of NF-κB signaling pathway. Plants, 12(6), 1241. https://doi.org/10.3390/plants12061241
- Loukili, E. H., Ouahabi, S., Elbouzidi, A., Taibi, M., Yahyaoui, M. I., Asehraou, A., Azougay, A., Saleh, A., Al Kamaly, O., Parvez, M. K., El Guerrouj, B., Touzani, R., & Ramdani, M. (2023). Phytochemical composition and pharmacological activities of three essential oils collected from Eastern Morocco (origanum compactum, Salvia officinalis, and syzygium aromaticum): A comparative study. Plants, 12(19), 3376. https://doi.org/10.3390/plants12193376
- Massad, T. J., Fincher, R. M., Smilanich, A. M., & Dyer, L. (2011). A quantitative evaluation of major plant defense hypotheses, nature versus nurture, and chemistry versus ants. Arthropod-Plant Interactions, 5(2), 125–139. https://doi.org/10.1007/s11829-011-9121-z
- Medzhitov, R. (2010). Inflammation 2010: New adventures of an old flame. Cell, 140(6), 771–776. https://doi.org/10.1016/j.cell.2010.03.006
- Miguel, M. G. (2010). Antioxidant and anti-inflammatory activities of essential oils: A short review. Molecules, 15(12), 9252–9287. https://doi.org/10.3390/molecules15129252
- Mirza, S. K., Asema, U. K., & Kasim, S. S. (2017). To study the harmful effects of food preservatives on human health. Journal of Medical Chemistry on Drug Discovery, 2(2), 610–616.
- Mosmann, T. (1983). Rapid colorimetric assay for cellular growth and survival: Application to proliferation and cytotoxicity assays. Journal of Immunological Methods, 65(1–2), 55–63. https://doi.org/10.1016/0022-1759(83)90303-4
- Nathan, C. (2002). Points of control in inflammation. Nature, 420(6917), 846–852. https://doi.org/10.1038/nature01320
- Noriega, P. (2020). Terpenes in essential oils: Bioactivity and applications. In S. Perveen & A. M. Al-Taweel (Eds.), Terpenes and terpenoids—Recent advances (pp. 142). IntechOpen.
- Öztürk, G., YILMAZ, G., Gülnur, E., & DEMİRCİ, B. (2021). Chemical composition and antibacterial activity of Clinopodium nepeta subsp. glandulosum (req.) govaerts essential oil. Natural Volatiles & Essential Oils, 8(3), 75–80. https://doi.org/10.37929/nveo.949959
- Pérez, G., Zavala, S., S, M., Arias, G. L., & Ramos, L. M. (2011). Anti-inflammatory activity of some essential oils. Journal of Essential Oil Research, 23(5), 38–44. https://doi.org/10.1080/10412905.2011.9700480
- Quézel, P., & Santa, S. (1962). Nouvelle flore de l’algerie et des regions desertiques meridionales: avec la collab. technique de Mme O. Schotter. Pref. du pr. L. Emberger. Editions du CNRS.
- Rodenak-Kladniew, B., Castro, M. A., Gambaro, R. C., Girotti, J., Cisneros, J. S., Viña, S., Padula, G., Crespo, R., Castro, G. R., & Gehring, S. (2023). Cytotoxic screening and enhanced anticancer activity of lippia alba and clinopodium nepeta essential oils-loaded biocompatible lipid nanoparticles against lung and colon cancer cells. Pharmaceutics, 15(8), 2045. https://doi.org/10.3390/pharmaceutics15082045
- Roy, A., Park, H.-J., Abdul, Q. A., Jung, H. A., & Choi, J. S. (2018). Pulegone exhibits anti-inflammatory activities through the regulation of NF-κB and nrf-2 signaling pathways in LPS-stimulated RAW 264.7 cells. Natural Product Sciences, 24(1), 28–35. https://doi.org/10.20307/nps.2018.24.1.28
- Taban, B. M., Stavropoulou, E., Winkelströter, L. K., & Bezirtzoglou, E. (2021). Value-added effects of using aromatic plants in foods and human therapy. Food Science and Technology, 42, e43121. https://doi.org/10.1590/fst.43121
- Taibi, M., Elbouzidi, A., Haddou, M., Baraich, A., Loukili, E. H., Moubchir, T., Allali, A., Asehraou, R., Bellaouchi, A., & Addi, M. (2024). Phytochemical characterization and multifaceted bioactivity assessment of essential oil from ptychotis verticillata Duby: Anti-diabetic, anti-tyrosinase, and anti-inflammatory activity. Heliyon, 10(8), e29459. https://doi.org/10.1016/j.heliyon.2024.e29459
- Taibi, M., Elbouzidi, A., Haddou, M., Loukili, E. H., Bellaouchi, R., Asehraou, A., Douzi, Y., Addi, M., Salamatullah, A. M., & Nafidi, H.-A. (2024). Chemical profiling, antibacterial efficacy, and synergistic actions of ptychotis verticillata duby essential oil in combination with conventional antibiotics. Natural Product Communications, 19(1), 1934578X231222785. https://doi.org/10.1177/1934578X231222785
- Taibi, M., Elbouzidi, A., Ouahhoud, S., Loukili, E. H., Ou-Yahya, D., Ouahabi, S., Alqahtani, A. S., Noman, O. M., Addi, M., Bellaouchi, R., Asehraou, A., Saalaoui, E., El Guerrouj, B., & Chaabane, K. (2023). Evaluation of antioxidant activity, cytotoxicity, and genotoxicity of ptychotis verticillata essential oil: Towards novel breast cancer therapeutics. Life, 13(7), 1586. https://doi.org/10.3390/life13071586
- Taibi, M., Elbouzidi, A., Ou-Yahia, D., Dalli, M., Bellaouchi, R., Tikent, A., Roubi, M., Gseyra, N., Asehraou, A., Hano, C., Addi, M., El Guerrouj, B., & Chaabane, K. (2023). Assessment of the antioxidant and antimicrobial potential of ptychotis verticillata duby essential oil from Eastern Morocco: An in vitro and in silico analysis. Antibiotics, 12(4), 655. https://doi.org/10.3390/antibiotics12040655
- Tomko, A. M., Whynot, E. G., Ellis, L. D., & Dupré, D. J. (2020). Anti-cancer potential of cannabinoids, terpenes, and flavonoids present in cannabis. Cancers, 12(7), 1985. https://doi.org/10.3390/cancers12071985
- Xu, X., Liu, A., Hu, S., Ares, I., Martínez-Larrañaga, M.-R., Wang, X., Martínez, M., Anadón, A., & Martínez, M.-A. (2021). Synthetic phenolic antioxidants: Metabolism, hazards and mechanism of action. Food Chemistry, 353, 129488. https://doi.org/10.1016/j.foodchem.2021.129488
- Zhao, H., Ren, S., Yang, H., Tang, S., Guo, C., Liu, M., Tao, Q., Ming, T., & Xu, H. (2022). Peppermint essential oil: Its phytochemistry, biological activity, pharmacological effect and application. Biomedicine & Pharmacotherapy, 154, 113559. https://doi.org/10.1016/j.biopha.2022.113559