ABSTRACT
Curcumin is a natural polyphenolic compound exerting antioxidative and anti-inflammatory effects on various tissues, including muscles. Nevertheless, its clinical usage has been limited because of its poor bioavailability. Thus, highly bioavailable curcumin, such as Theracurmin (CR-033P) composed of 36% crystalline curcumin, has been developed. This study investigated the antioxidant activity and muscle-protective effect of Theracurmin Super (TS-P1), a new formulation with 36% amorphous curcumin. TS-P1 and CR-033P demonstrated antioxidant activity in vitro. Compared with pure curcumin, orally administered TS-P1 or CR-033P (16.7 or 50 mg per kg of body weight) at one-third dose inhibited the elevation of plasma lactate dehydrogenase and creatine kinase levels in a mouse downhill running model, indicating superior muscle-protective effect. Particularly, TS-P1 showed better or equivalent effectiveness in restoring the activity of antioxidant enzymes including glutathione peroxidase and catalase, and in suppressing inflammatory responses. Therefore, formulation with amorphous curcumin is a promising strategy for curcumin-based supplements.
1. Introduction
Curcumin is a natural polyphenolic compound, which was first isolated from the rhizome of turmeric, and it has been widely used as a spice and traditional medicine (Pulido-Moran et al., Citation2016). Numerous studies have revealed its versatile effect as an antioxidant, anti-inflammatory, antitumor/anticancer, antimicrobial/antiviral, or antiaging/antisenescence agent (Maheshwari et al., Citation2006; Zia et al., Citation2021). Thus, curcumin has been considered a multitarget drug for chronic diseases induced by complex pathological factors, and clinical trials have been extensively performed (Kunnumakkara et al., Citation2017). According to safety reports of curcumin as food, it is an edible nontoxic compound when ingested up to 12 g/day (Authority, Citation2014; JECFA, Citation2004; Lao et al., Citation2006). Owing to its nontoxic nature, many pharmaceutical companies have attempted to use curcumin as a health supplement and nutraceutical (Kunnumakkara et al., Citation2017). Nevertheless, the use of pure curcumin has been limited because of its chemical properties, including negligible solubility in water, poor absorbability in the intestines, and rapid degradation under alkaline conditions (pH 7–10) (Gornicka et al., Citation2023). Therefore, new formulations are required for the development of highly bioavailable curcumin.
Various highly bioavailable curcumins have been developed according to the main formulation strategies: mixing with fatty or lipidic adjuncts, absorption and dispersal into a matrix, and particle size reduction (Gornicka et al., Citation2023; Hegde et al., Citation2023). Theracurmin was developed using dispersed submicron crystalline curcumin (Sasaki et al., Citation2011). Its human clinical trials as oral drugs or supplements for the management of exercise-induced muscle damage and even treatment of chronic diseases have been extensively performed (Imaizumi, Citation2015; Small et al., Citation2018; Sugimoto et al., Citation2020; Tanabe et al., Citation2015). Recently, it is also available as an encapsulated powder form (CR-033P) containing 36% curcumin (Chung et al., Citation2021). Compared with pure curcumin, orally administered CR-033P showed significantly improved bioabsorption in humans. Theracurmin Super (TS-P1) was also developed by replacing the crystalline curcumin with amorphous curcumin (Hirose et al., Citation2022), which has 17-fold higher water solubility than crystalline curcumin (Pawar et al., Citation2012). Compared with CR-033P, TS-P1 showed an improvement of 1.54-fold on maximum concentration (Cmax) and 1.02-fold on area under the concentration – time curve for 12 h (AUC0–12 h) in human plasma, even at the half-dose level. Thus, the bioavailability of TS-P1 is 85.2-fold higher in humans than that of pure curcumin, implying the great potential of TS-P1. Despite its beneficial effects on common cold symptoms in the human clinical study (Kuwabara et al., Citation2023), no further advanced preclinical or clinical studies of TS-P1 have been performed.
Curcumin ameliorates exercise-induced muscle damage (Nanavati et al., Citation2022). In this study, we investigated the potential of TS-P1 as an oral supplement for exercise-induced muscle damage using an animal model. Downhill running (DHR), a type of eccentric exercise, induces muscle damage through oxidative stress by reactive oxygen species (ROS), leading to the release of muscular proteins including creatine kinase (CK), lactate dehydrogenase (LDH), and myoglobin into the blood (Schwane et al., Citation1983). It also triggers inflammatory responses, which induce the elevation of the levels of proinflammatory cytokines including interleukins, tumor necrosis factor-α (TNF-α), and C-reactive protein, and further activation of macrophages (Fernandez-Lazaro, Mielgo-Ayuso, Seco Calvo, et al., Citation2020; Kawanishi et al., Citation2013; Muller, Citation2001; Wang & Zhou, Citation2022). TS-P1 or CR-033P was orally administered to mice subjected to DHR, and their effect on muscle damage was examined by evaluation of the levels of muscular proteins in the plasma, oxidative stress, and inflammatory markers in skeletal muscles after DHR.
2. Materials and methods
2.1. Animals and ethics statement
Male C57BL/6 mice (age: 8 weeks) were obtained from DBL (Eumseong, Korea), and housed in a controlled environment with a 12-h light/dark cycle and free access to food and water. All animal experiments were performed according to the guidelines and principles for animal care and use set forth by the Institutional Animal Care and Use Committee (IACUC) of DGIST (Daegu, Korea), and approved by the IACUC (#DGIST-IACUC-22122603–0001).
2.2. DHR protocol and compound administration
We adopted a mouse DHR protocol from previous studies with slight modifications (Davis et al., Citation2007; Haramizu et al., Citation2013; Kawanishi et al., Citation2013). Briefly, on experimental days 1 and 2, the mice were trained at a speed of 20 m/min on a flat treadmill (#LE8710MTS and #LE8709TS, Pinlab, Spain) for 20 min once per day. Mice that did not adapt and run well on the treadmill during training were excluded. The well-trained mice were randomly divided into seven experimental groups containing eight mice per group with similar average of their body weight (), and then allowed to rest on day 3. On day 4, the DHR protocol was performed on a − 15%-inclined treadmill at a speed of 22 m/min for 150 min except to resting group.
Table 1. Change of body weight of mice.
Curcumin was purchased from FUJIFILM Wako Pure Chemical Corporation (#038–04921; Osaka, Japan). TS-P1 and CR-033P were freely obtained from Handok R&D Center (Seoul, Korea). CR-033P and TS-P1 contain 36% crystalline curcumin and amorphous curcumin, respectively (Chung et al., Citation2021; Hirose et al., Citation2022). Each solution for administration of curcumin, CR-033P, and TS-P1 was prepared as 6.67 or 20 mg/ml concentrated solution with saline. Following DHR, we orally administered the saline or prepared solution (2.5 ml per body weight kg) into the mice by using an oral zonde needle (#JD-S-124-38-24 G, Jeungdo Bio & Plant Co. Ltd., Seoul, Korea), according to body weight of each mouse. After 24 h of single administration of compound solutions, the mice were anesthetized by intraperitoneal injection of avertin (250 mg/kg) from a 20 mg/ml stock solution containing 2,2,2-tribromoethanol (#T48402-25 G, Merck, Darmstadt, Germany) with 2-methyl-2-butanol (#152463-250 ML, Merck). Finally, their plasma and gastrocnemius muscles were obtained.
2.3. In vitro antioxidant effect assay
Scavenging activity assay against free radical 2,2-Diphenyl-1-picrylhydrazyl (DPPH) (#044150, Alfa Aesar, Ward Hill, MA, U.S.A.) was performed as previously reported (Yong-Hwan et al., Citation2016). Briefly, we incubated 150 μl of 150 μM DPPH with 1.5 μl of serially concentrated solutions (from 1.5625 to 200 μg/ml in saline) of CR-033P or TS-P1 for 30 min, and then measured its absorbance (At) at 520 nm. The following formula was used to calculate the relative antioxidant activity; % of antioxidant activity = [1− (At ÷ control absorbance)] × 100. Ferric reducing antioxidant power (FRAP), and total antioxidant capacity (TAC) assays were used for measurement of conversion of Fe3+ to Fe2+ and Cu2+ to Cu+ by antioxidants, respectively. Fe2+ and Cu+ are colored metal ions having high absorbance at 540 nm and 490 nm, respectively. FRAP and TAC assays were performed using commercial kits, OxiSelect™ Ferric Reducing Antioxidant Power Assay Kit (#STA-859, Cell Biolabs, San Diego, CA, U.S.A.), and OxiSelect™ Total Antioxidant Capacity Assay Kit (#STA-360, Cell Biolabs), according to manufacturer’s protocols. 30 μM ascorbic acid (#L92902, Merk) was used as a positive reference of antioxidant. Quantitative data were analyzed by one-way ANOVA on ranks using Dunnett’s Method.
2.4. Biochemical assays
Crude blood samples were collected into PST™ Tubes with lithium heparin (BD Microtainer®, #365985, BD Biosciences, Bergen County, NJ, U.S.A.). Next, plasma was separated by centrifugation at 2,000 g for 15 min at 4°C. Lactate dehydrogenase (LDH) and creatine kinase CK) activities were measured using commercial kits, LDH assay kit (#ab197000; Abcam, Cambridge, UK), and CK assay kit (#ECPK-100, BioAssay Systems, Hayward, CA, U.S.A.), according to the manufacturer’s protocols. Gastrocnemius muscle was dissected from the hind legs of mice, and homogenized in tissue protein extraction reagent (#78510, Thermo Fisher Scientific, Waltham, MA, U.S.A.) containing a protease inhibitor cocktail (#1861278, Thermo Fisher Scientific) using a pestle homogenizer and an ultrasonic sonicator. Subsequently, we performed biochemical assays using commercial assay kits according to the manufacturer’s instructions: hydrogen peroxide level (#AS-71112, AnaSpec, Fremont, CA, U.S.A.); malondialdehyde level (#ab118970, Abcam); glutathione peroxidase activity (#ab102530, Abcam); and catalase activity (#ab83464, Abcam). Each measured value was calibrated according to the protein amount of GA used in the assay. Quantitative data were analyzed using unpaired Student’s t-test or rank-sum test.
2.5. Real-time reverse transcription-quantitative polymerase chain reaction (PCR)
GA was homogenized into Qiazol lysis buffer (#79306, Qiagen, Germany), and RNA was extracted according to organic extraction methods. Total mRNA was reverse-transcribed to cDNA using Transcriptor First Strand cDNA Synthesis Kit (#04896866001, Roche, Switzerland), according to manufacturer’s instructions. Next, quantitative real-time PCR was performed using a LightCycler® 480 SYBR Green I Master mix (#04887352001, Roche) in a Thermal Cycler (#T100TM, BioRAD, Hercules, CA, U.S.A.). For PCR, DNA primers were designed as previously reported () (Kawanishi et al., Citation2013). The mRNA levels of targets were normalized to those of Gapdh, and quantitative data were analyzed using unpaired Student’s t-test.
Table 2. DNA primers for real-time RT-PCR.
2.6. Statistical analysis
All quantitative analyses and graph plotting were performed by using SigmaPlot 12.5 program (Grafiti LLC, CA, U.S.A.). Detail statistic information is described in the figure legends. Numbers of animal are given in the figure legends as “n”. Error bars represent standard error (SEM). To evaluate statistical significance, we performed two-tailed paired/unpaired t-test, Mann-Whitney rank sum test, or one-way ANOVA on ranks using Dunnett’s Method, and p < .05 is considered as “significant”.
3. Results
3.1. TS-P1 and CR-033P exhibit the antioxidant effect in vitro
First, the antioxidant activities of TS-P1 and CR-033P were examined. TS-P1 and CR-033P significantly reduced the absorbance of DPPH radical at 520 nm in a dose-dependent manner, indicating that they have free radical-scavenging activity ()). Their IC50 values were comparable; 25.48 μg/mL for TS-P1 and 25.0 μg/mL for CR-033P. In addition, ferric reducing ability of plasma (FRAP) and total antioxidant capacity (TAC) assays exhibited that TS-P1 and CR-033P significantly convert Fe3+ and Cu2+ to reduced forms, Fe2+ and Cu+, in a dose-dependent manner ()). The FRAPs of TS-P1 and CR-033P increased up to 1,618% and 1,911% at a concentration of 200 μg/mL, respectively ()). Their TACs increased up to 11,068% and 1,820% at a concentration of 200 μg/ml ()). These data indicate that TS-P1 and CR-033P can function as antioxidants by reducing activity in vitro.
Figure 1. Antioxidant effect of TS-P1 and CR-033P in vitro (a, b) measurement of scavenging activity of TS-P1 (a) and CR-033P (b) against DPPH radical. Ascorbic acid (AA) is used as a positive control. (c, d) measurement of ferric reducing antioxidant power (FRAP) of TS-P1 (c) and CR-033P (d). (e, f) measurement of total antioxidant capacity (TAC) of TS-P1 (e) and CR-033P (f). Data were expressed as mean ± SEM (n = 3, *p < .05, **p < .01, ***p < .001 vs untreated group by one-way ANOVA using Dunnett’s multiple comparison test for TS-P1 and CR-033P; n = 3, ***p < .001 vs untreated group by t-test for AA).
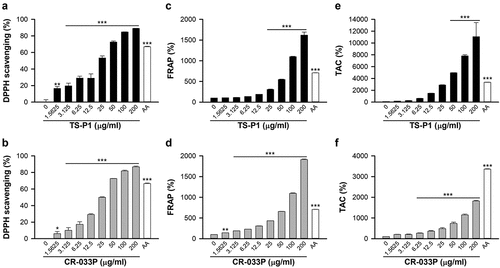
3.2. TS-P1 and CR-033P alleviate muscle tissue damage in the mouse DHR model
To investigate the tissue-protective effects of TS-P1 and CR-033P, DHR of mice was performed, and saline, 16.7 mg TS-P1 (TS-P116.7), 50 mg TS-P1 (TS-P150), 16.7 mg CR-033P (CR-033P16.7) or 50 mg CR-033P (CR-033P50), or 50 mg pure curcumin (curcumin50) per kg of the body weight of mice were orally administered subsequently (). The resting group showed significant increase on body weight (p = .001), but not the DHR group with or without administration of compound (). Expectedly, compared with the resting group, DHR significantly increased the levels of plasma LDH released from damaged tissue (p < .001) (). However, this increase was significantly suppressed by TS-P150, CR-033P16.7, or curcumin50 (TS-P150, p = .021; CR-033P16.7, p < .001; curcumin50, p < .001). In addition, the level of plasma CK, which is released from the damaged muscle as a highly enriched enzyme in muscle cells, was also increased by DHR (p = .001) (). This elevation was also inhibited by TS-P116.7, TS-P150, CR-033P16.7, and curcumin50 (TS-P116.7, p = .02; TS-P150, p = .027; CR-033P16.7, p < .001; curcumin50, p = .002). These data suggest that, similar to curcumin, TS-P1 and CR-033P exert muscle-protective effects upon DHR.
Figure 2. Muscle-protective effect of TS-P1 and CR-033P in mouse DHR model. (a) Experimental scheme of mouse DHR model. For two days, mice were trained to run on flat treadmill for 10 min per day, and then subjected to DHR after 1 day resting. (b, c) measurement of activities of lactate dehydrogenase (LDH) (b) and creatine kinase (CK) (c) in plasma of mice treated with or without saline, TS-P116.7, TS-P150, CR-033P16.7, CR-033P50, and curcumin50. Data were expressed as mean ± SEM (n = 8, *p < .05 for resting and †p < .05 for saline by t-test).
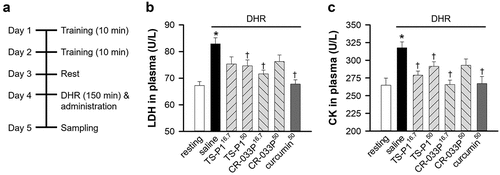
3.3. TS-P1 and CR-033P restore antioxidant enzyme activity in muscle upon DHR
Curcumin functions as an antioxidant against oxidative stress in exercise-induced skeletal muscle damage (Nanavati et al., Citation2022). Thus, whether the TS-P1 and CR-033P mediate antioxidant reaction was examined. DHR markedly increased the levels of hydrogen peroxide (p < .001), a type of ROS, and malondialdehyde (p = .021), a final product of lipid peroxidation, in gastrocnemius muscle (GA) ()). However, TS-P1, CR-033P, and curcumin did not affect the levels of hydrogen peroxide and malondialdehyde upon DHR. In addition, DHR increased the mRNA levels of Nox2 encoding NADPH oxidase 2 (p = .038), which is involved in exercise-evoked oxidative stress as a main ROS-producing enzyme in the muscles (Powers et al., Citation2020) (). Notably, this increase was significantly suppressed by TS-P150 (p = .012), but not by CR-033P and curcumin (). Because curcumin restores the activity of antioxidant enzymes, including glutathione peroxidase (GPx) and catalase, in oxidative stress models (Nanavati et al., Citation2022), whether TS-P1 and CR-033P affect antioxidant enzymes was examined. DHR significantly reduced the activities of GPx and catalase in GA (GPx, p = .001; catalase, p = .026) ()). Interestingly, GPx activity was significantly restored by TS-P116.7 (p < .001), TS-P150 (p = .004), or CR-033P16.7 (p = .029) but not by CR-033P50 and curcumin50 (). Similarly, catalase activity was also restored by TS-P1, CR-033P, and curcumin (TS-P116.7, p = .007; TS-P150, p < .001; CR-033P16.7, p < .001; CR-033P50, p = .004; curcumin50, p < .001) (). These results suggests that TS-P1 and CR-033P restore the activity of antioxidant enzymes upon DHR.
Figure 3. Antioxidant effect of TS-P1 and CR-033P upon DHR-induced oxidative stress (a, b) measurement of levels of hydrogen peroxide (H2O2) (a) and malondialdehyde (MDA) (b) in gastrocnemius muscle (GA) of mice treated with or without saline, TS-P116.7, TS-P150, CR-033P16.7, CR-033P50, and curcumin50. Data were expressed as mean ± SEM (n = 8, *p < .05 for resting by t-test). (c) Relative level of mRNA of Nox2 in GA of mice treated with or without saline, TS-P116.7, TS-P150, CR-033P16.7, CR-033P50, and curcumin50. Data were expressed as mean ± SEM (n = 8, *p < .05 for resting and †p < .05 for saline by t-test). (d, e) measurement of activities of glutathione peroxidase (GPx) (D) and catalase (E) in GA of mice treated with or without saline, TS-P116.7, TS-P150, CR-033P16.7, CR-033P50, and curcumin50. Data were expressed as mean ± SEM (n = 8, *p < .05 for resting and †p < .05 for saline by t-test).
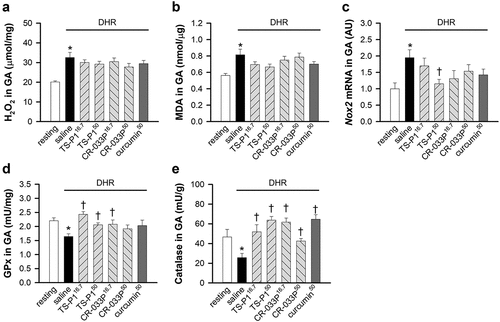
3.4. TS-P1 and CR-033P reduced inflammatory responses in muscle upon DHR
Curcumin inhibits inflammatory response in exercise-induced muscle damage (Kawanishi et al., Citation2013; Ms et al., Citation2020). To investigate whether TS-P1 and CR-033P also suppress inflammatory response upon muscle damage, we examined the expression of inflammatory cytokines induced by muscle damage. The mRNA levels of Il1b, Il6, and Tnfa coding interleukin-1β (IL-1β), interleukin-6 (IL-6), and tumor necrosis factor-α (TNFα) were significantly increased by DHR (Il1b, p < .005; Il6, p = .05; Tnfa, p = .046) ()), as previously reported (Cannon et al., Citation1989; Fielding et al., Citation1993; Hamada et al., Citation2005). Notably, TS-P150 significantly suppressed the upregulation of mRNA levels of Il1b and Il6 (Il1b, p = .039; Il6, p = .05), but not mRNA of Tnfa ()). CR-033P50 slightly inhibited the DHR-induced increase of Il6 mRNA (). In contrast, mRNA level of Il10 coding interleukin-10 (IL-10), an anti-inflammatory cytokine, did not change upon DHR regardless of TS-P1, CR-033P, and curcumin (). In addition, the mRNA level of Ptgs2 coding cyclooxygenase 2 (COX-2), which is involved in muscle regeneration after proinflammatory removal of damaged muscle tissue (Alvarez et al., Citation2020), was increased by DHR in GA (p = .002); this process was not affected by TS-P1, CR-033P, or curcumin (), similar to previous report (Kawanishi et al., Citation2013).
Figure 4. Anti-inflammatory effect of TS-P1 and CR-033P upon DHR. (a-c) measurement of relative levels of mRnas of Il1b (a), Il6 (b), and tnfa (c), coding pro-inflammatory cytokines IL-1β, IL-6 and TNFα respectively, in GA of mice treated with or without saline, TS-P116.7, TS-P150, CR-033P16.7, CR-033P50, and curcumin50. Data were expressed as mean ± SEM (n = 8, *p < .05 for resting and †p < .05 for saline by Mann – Whitney rank sum test). (d) Measurement of relative level of mRNA of Il10, coding IL-10, in GA of mice treated with or without saline, TS-P116.7, TS-P150, CR-033P16.7, CR-033P50, and curcumin50. Data were expressed as mean ± SEM (n = 8, *p < .05 for resting and †p < .05 for saline by Mann – Whitney rank sum test). (e) Measurement of relative levels of mRnas of Ptgs2, coding COX-2, in GA of mice treated with or without saline, TS-P116.7, TS-P150, CR-033P16.7, CR-033P50, and curcumin50. Data were expressed as mean ± SEM (n = 8, *p < .05 for resting and †p < .05 for saline by Mann – Whitney rank sum test).
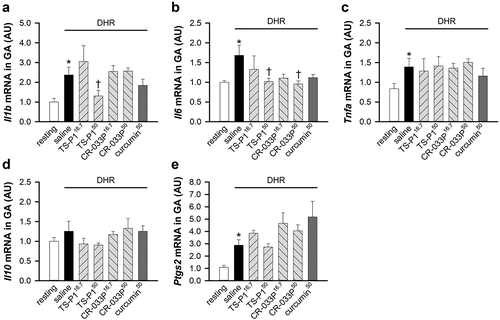
Next, we examined whether TS-P1 and CR-033P affect macrophagic activation. The mRNAs of Mcp1 and Cxcl14, genes of MCP1 and CXCL14 chemotactic factors for macrophagic recruitment into damaged muscle (Kawanishi et al., Citation2013; Muller, Citation2001; Wang & Zhou, Citation2022), were markedly increased by DHR in GA (Mcp1, p < .001; Cxcl14, p < .001) ()). Notably, TS-P1 or CR-033P significantly suppressed the upregulation of mRNAs of Mcp1 (TS-P116.7, p = .038; TS-P150, p < .001; CR-033P16.7, p = .007; curcumin50, p = .05), and Cxcl14 (TS-P150, p < .001; CR-033P50, p = .002), similar to curcumin50 (). Additionally, the mRNA level of Adgre1, coding ADGRE1 as a macrophage marker, was also increased in GA upon DHR (p = .021); it was significantly suppressed by TS-P150 (p < .001), but not CR-033P and curcumin (). These data indicate that DHR-induced macrophage activation is inhibited by TS-P1. Given that TS-P1 exerts similar or better muscle-protective effects at lower doses compared with CR-033P and crude curcumin, enhancing the bioavailability of curcumin using amorphous curcumin is a promising strategy for curcumin-based therapy.
Figure 5. Inhibitory effect of TS-P1 and CR-033P on macrophage activation upon DHR. (a-c) measurement of relative levels of mRnas of Mcp1 (a), Cxcl14 (b), and Adgre1 (c), which are genes involved in macrophagic activation, in GA of mice treated with or without saline, TS-P116.7, TS-P150, CR-033P16.7, CR-033P50, and curcumin50. Data were expressed as mean ± SEM (n = 8, *p < .05 for resting and †p < .05 for saline by Mann – Whitney rank sum test).
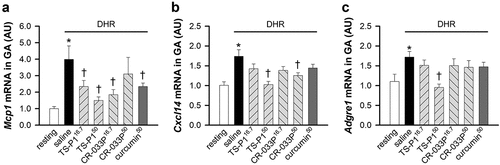
4. Discussion
This study demonstrates the antioxidant and muscle-protective effects of recently developed highly bioavailable curcumins, TS-P1 and CR-033P. TS-P1 and CR-033P dose-dependently inhibited the activity of metal ions, indicating that they can function as direct antioxidants. Their antioxidant activities may result from the high content of curcuminoid, a potent antioxidant characterized by notable reducing capacity (Pulido-Moran et al., Citation2016). In addition, TS-P1 and CR-033P efficiently inhibited muscle damage induced by DHR, similar to pure curcumin. Notably, the administration of 16.7 mg/kg dose of TS-P1 or CR-033P containing only 6 mg of pure curcumin showed similar muscle-protective effects to the 50 mg/kg dose of pure curcumin. In particular, compared with CR-033P and pure curcumin, TS-P1, which is formulated with amorphous curcumin, showed better effects on the restoration of antioxidant enzymes and suppression of inflammatory responses. Taken together, a usage of amorphous curcumin is a good approach for curcumin-based clinical trials.
Curcumin has been suggested as a drug or supplement for health management and treatment of various diseases. Its versatile biological effects are proved by its activation of multiple signaling pathways (Liczbinski et al., Citation2020). For example, curcumin activates the NRF2-mediated antioxidant system, which increases the antioxidant capacity and cellular resistance under physiological and pathological conditions (Ghafouri-Fard et al., Citation2022). In cancer cells, it triggers cell death via calcium release and ER stress, and cell-cycle arrest by inhibiting PI3K/AKT, STAT, and NF-κB signaling pathways (Ramasamy et al., Citation2015). It also suppresses inflammatory responses by inhibiting COX-2 and NF-κB signaling. Therefore, enhanced bioavailability is only a breakthrough in the clinical usage of curcumin.
TS-P1 and CR-033P have better protective effects against muscle damage than pure curcumin because of their high bioabsorption rates. Expectedly, TS-P1 and CR-033P exerted more efficient or similar muscle-protective effects than pure curcumin at even 8.3-times lower dose containing only 12% pure curcumin (Chung et al., Citation2021; Hirose et al., Citation2022). The results re-emphasize the importance of curcumin’s bioavailability for biological effects. A recent review paper summarizes previously developed formulations of curcumin into three generations (Hegde et al., Citation2023). First-generation formulations use some adjuvants including piperine, turmeric fiber, or oil, increasing the absorption rate of curcumin by inhibiting its breakdown. For example, formulation with piperine, which promotes the absorption of drug or nutrient through the epithelial cells of the intestine as a well-known bioenhancer, increases the absorption rate of curcumin by 2.8-fold in rat plasma (Fernandez-Lazaro, Mielgo-Ayuso, Cordova Martinez, et al., Citation2020; Shaikh et al., Citation2009). Some piperine-formulated curcumins, such a curcumin C3 complex bioperine, curcumin forte, and Turmix tablet, have been clinically investigated and exhibited significant anticancer, antipsychotics, or antifibrosis effects on humans without any serious adverse effects (Garcea et al., Citation2005; Miodownik et al., Citation2019; Rai et al., Citation2019; Sharma et al., Citation2004).
Second-generation formulations have been developed for the improvement of the solubility of curcumin using carbohydrate emulsifiers, polysorbate, phospholipid complexes, water-dispersible nanopreparations, nanomicelle lipid complexes, and spray drying. For instance, compared with pure curcumin, Theracurmin, CR-033P, which is developed through the nanoparticle dispersion technique, exhibited approximately 30-fold higher bioabsorption rate in the human plasma (HeeJung et al., Citation2015; Sasaki et al., Citation2011). Until now, many clinical studies have been performed, and Theracurmin exhibited beneficial effects on the neurological phenotype, alcohol intoxication, and exercise without significant adverse effects, as well as some diseases including Crohn’s disease, osteochondral diseases, pancreatic or biliary duct cancer, and diabetes (Funamoto et al.,Citation2016, Citation2019; Kanai et al.,Citation2012, Citation2013; Morimoto et al., Citation2013; Nakagawa et al., Citation2022; Ross, Citation2018; Sasaki et al., Citation2011; Small et al., Citation2018; Sugawara et al., Citation2012; Sugimoto et al., Citation2020; Tanabe et al., Citation2015; Tanabe, Chino, Ohnishi, et al., Citation2019; Tanabe, Chino, Sagayama, et al., Citation2019). Recently, third-generation curcumin formulations, which further increase the bioavailability of bioactive curcuminoids, permeability through the blood – brain barrier, cellular uptake, and tissue distribution without the usage of additional emulsifiers, have emerged. Curcumin galactomannan, CurQfen, Curcuwin Ultra, and Longvida belong to this category (Hegde et al., Citation2023). Recently developed TS-P1 also showed an increase of 2.81-fold on AUC0–1 h, 1.44-fold on AUC0–2 h, and 1.33-fold on AUC0–4 h with a 1.54-fold increase at Cmax in the human plasma, despite its dose was only half compared with CR-033P (Hirose et al., Citation2022). This superior effect of TS-P1 may result from the more rapid bioabsorption due to the use of amorphous curcumin (Pawar et al., Citation2012). In this study, compared with CR-033P, TS-P1 demonstrated better biological effects on the antioxidant system and inflammation in the mouse DHR model. Furthermore, in a previous clinical study, amorphous curcumin formulation showed some improvement in liver function and lipid metabolism in patients with non-alcoholic fatty liver disease (Rahmani et al., Citation2016). Considering that CR-033P is a second-generation formulation, TS-P1 also may be a third-generation formulation.
Curcumin has been widely proposed as a biohealth supplement for exercise-induced muscle damage (Kunnumakkara et al., Citation2017). For example, before or after its administration, curcumin induced an antifatigue effect and better performance with lower inflammatory response and skeletal muscle damage in a mouse DHR model (Davis et al., Citation2007; Kawanishi et al., Citation2013) and an exhaustive mouse swimming model (Chen et al., Citation2022; Huang et al., Citation2015). Human clinical studies involve the use of exercise and highly bioavailable curcumins, including Theracurmin, Meriva, NovaSol, CurQfen, Longvida, and Curcumin C3 complex (Nanavati et al., Citation2022). In human exercise models, the oral administration of these highly bioavailable curcumins improved muscle performance with suppression of muscle soreness and damage by attenuating the inflammatory response and oxidative stress (Nanavati et al., Citation2022). For example, single or repetitive intake of 90–180 mg Theracurmin before and/or after eccentric exercise of the quadriceps or elbow flexors effectively reduced muscle damage (Nakhostin-Roohi et al., Citation2016; Takahashi et al., Citation2014; Tanabe et al., Citation2015; Tanabe, Chino, Ohnishi, et al., Citation2019). Recently, after the administration of CR-033P, muscle-protective effects on eccentric exercises of the elbow flexors were observed (Tanabe, Chino, Sagayama, et al., Citation2019). However, further clinical trials focusing on TS-P1 have not been performed in this field.
Moreover, TS-P1 and CR-033P restored the activity of antioxidant enzymes upon DHR. During exercise, muscular contraction induces the Nox2-dependent production of ROS in skeletal muscle fibers, resulting in hormesis response (Powers et al., Citation2020). This response increases the antioxidant capacity by increasing the levels of antioxidant enzymes, including GPx, catalase, and superoxide dismutase. However, extremely high-intensity, prolonged, or eccentric exercise results in oxidative stress with loss of antioxidant function, leading to muscle damage (Powers et al., Citation2020). Our DHR model also increased the levels of hydrogen peroxide and peroxidized lipid and decreased the activities of GPx and catalase, indicating the induction of oxidative stress. However, TS-P1 strongly suppressed the Nox2 expression, whereas TS-P1 and CR-033P restored the activities of GPx and catalase. These data imply that TS-P1 and CR-033P may facilitate exercise-induced hormesis and reduce muscle damage. The antioxidant effect of curcumin is mediated directly by its antioxidant effects or indirectly by evoking an NRF2-dependent antioxidant response involved in the transcriptional activation of antioxidant enzymes (Trujillo et al., Citation2013). Notably, TS-P1, CR-033P, and curcumin do not directly suppress the increase in ROS levels after 24 h of DHR, although the activities of antioxidant enzymes are significantly restored. This inconsistency results from that the single administration of 16.7 or 50 mg/kg compounds may be not sufficient for scavenging ROS via direct reduction in the early step of muscle damage after 24 h of DHR. Instead, they may evoke “time-required” NRF2-mediated antioxidant response. Particularly, NRF2 signaling transcriptionally activates the expression of antioxidant enzymes and the biogenesis of the mitochondria, contributing to increased antioxidant capacity and enhanced muscle regeneration (Vargas-Mendoza et al., Citation2019). Therefore, we propose that the antioxidant effect of TS-P1 and CR-033P may be mediated by the NRF2-mediated antioxidant response rather than direct reduction. Further investigation is required to test this hypothesis.
Generally, during muscle damage caused by severe exercise or physical injury, necrotic degeneration of myofibers initially appears within 2 days, and it accompanies with elevation of pro-inflammatory cytokines including IL-1β, IL-6 and TNFα from damaged muscle cells and immune cells including macrophages and neutrophils, which reside in muscle tissue or are recruited by damage-associated molecular patterns (Cannon et al., Citation1989; Fielding et al., Citation1993; Hamada et al., Citation2005; Uderhardt et al., Citation2019). This process leads to inflammatory removal of damaged muscle tissue. Notably, we found that TS-P150 inhibits the elevation of IL-1β and IL-6 induced by DHR, but not pure curcumin. These results indicate that TS-P1 has a superior anti-inflammatory effect compared to pure curcumin. After early step of removal of damaged muscle tissue, myogenesis by myogenic precursor cells, muscle remodeling, and functional recovery occurs within 15 days (Forcina et al., Citation2020). IL-10 and COX-2 are involved in anti-inflammatory and regenerative process for muscle regeneration (Alvarez et al., Citation2020; Tidball et al., Citation2014). Our data show that TS-P1, CR-033P and curcumin did not affect expression of IL-10 and COX-2. Based on these data, we think that curcumin compounds may not directly evoke anti-inflammatory and/or regenerative signaling by upregulation of IL-10 and/or COX-2. Alternatively, time-range of our experiment seem to be not suitable for observation of direct anti-inflammatory and/or regenerative effect. Throughout muscle degeneration and repair processes, immune cells, particularly macrophages, play a critical role as scavengers of degenerated muscle cells and activators of satellite cells in the early phase of muscle regeneration (Wang & Zhou, Citation2022). This role of macrophages requires a COX-2-dependent proinflammatory response (Bondesen et al., Citation2004). In the present study, TS-P1, CR-033P, or curcumin did not affect the DHR-induced expression of IL-10 and COX-2 in GA. However, they reduced the expression of chemotactic factors of macrophages, such as MCP1 and CXCL14. Furthermore, TS-P1 significantly suppressed the infiltration of macrophages in the GA. According to previous studies, curcumin regulates NF-κB-mediated macrophage infiltration upon muscle damage (Sahin et al., Citation2016). Thus, we think that the anti-inflammatory effects of TS-P1 and CR-033P may be mediated by the inhibition of NF-κB signaling.
The results of this experimental study involving an animal model demonstrated for the first time the beneficial effect of TS-P1 for the prevention of oxidative stress and inflammation in the presence of skeletal muscle damage. Future studies should investigate the effects and molecular mechanisms of various diseases, including muscle damage. The present findings suggest that TS-P1 is a potentially effective therapeutic agent for skeletal muscle-related disorders.
5. Limitations and another of applications of the study
This study analyzed the protective effects of TS-P1 and CR-033P in early step within 1 day of muscular degeneration after DHR. After muscular degeneration for 2 days, myogenesis, muscle remodeling, and muscle functionalization subsequently appear as regeneration steps for 15 days (Forcina et al., Citation2020). Curcumin promotes muscular regeneration in several animal models of muscle injury induced by traumatic injury, contusion injury, chronic forced exercise, and limb immobilization (Lee et al., Citation2021; Manas-Garcia et al., Citation2020; Thaloor et al., Citation1999; Tsai et al., Citation2020). In particular, curcumin stimulates the proliferation, intercellular fusion, and muscular differentiation of skeletal myoblasts (Thaloor et al., Citation1999). Based on these properties of curcumin, it was proposed as an antisarcopenic compound (Saud Gany et al., Citation2023). Therefore, TS-P1 and CR-033P, the highly bioavailable curcumins, may exhibit more superior muscle-regenerative and antisarcopenic effects. However, this issue has never been illuminated so far. Although we did not investigate this issue, further study should be performed in a later step of the muscular regeneration process upon the longer treatment of TS-P1 and CR-033P. In addition, curcumin exhibits ergogenic effect by enhancing muscle performance. In several rodent models before the administration of curcumin, mouse had significantly increased exhaustion time on running or swimming (Davis et al., Citation2007; Huang et al., Citation2015; Sahin et al., Citation2016; Wafi et al., Citation2019). In human clinical trials, pre-/post-administration of curcumin reduces muscle damage by ameliorating oxidative stress and inflammation, thereby indirectly inducing an ergogenic effect (Hegde et al., Citation2023). Therefore, research about the ergogenic effects of TS-P1 and CR-033P is desired.
Curcumin has received attention as a multitarget therapeutic agent for several chronic diseases (Kunnumakkara et al., Citation2017). Thus, TS-P1 and CR-033P may also be effective in the treatment of chronic diseases; nevertheless, further investigation is warranted to determine their effectiveness. In fact, Theracurmin has already been proposed as therapeutics in cancer, arthritis, neurodegenerative diseases, and cardiovascular disorders (Imaizumi, Citation2015; Small et al., Citation2018; Tanabe et al., Citation2015). A recent study reported that TS-P1 or CR-033P resulted in resistance against common cold symptoms without any adverse effects (Kuwabara et al., Citation2023). However, no other preclinical or clinical evidence supports the role of TS-P1 in chronic diseases. Thus, this study is the first preclinical study of TS-P1 using an animal model. Further extensive study in other models will be conducted.
CRediT authorship contribution statement
Yu Seon Kim: Methodology, Formal analysis, Investigation, Writing – original draft. Bongki Cho: Conceptualization, Methodology, Validation, Formal analysis, Investigation Data curation, Writing – Original Draft, Writing – Review & Editing, Visualization, Supervision, Project administration. Sang-Heon Kim: Methodology, Validation, Formal analysis, Investigation, Resources, Writing – Original Draft. Byoung-Gon Moon: Conceptualization, Resources, Writing – Review & Editing, Project administration, Funding acquisition. Yun-Il Lee: Conceptualization, Validation, Writing – Review & Editing, Supervision, Project administration, Funding acquisition.
Acknowledgments
This work was supported by the Handok Pharmaceuticals in Korea.
Disclosure statement
Sang-Heon Kim and Byoung-Gon Moon are employees of HANDOK Inc. The other authors declare no conflict of interest.
Data availability statement
The raw datasets used and/or analyzed are available from the corresponding author upon reasonable request.
Additional information
Funding
References
- Alvarez, A. M., DeOcesano-Pereira, C., Teixeira, C., & Moreira, V. (2020). IL-1β and TNF-α modulation of proliferated and committed myoblasts: IL-6 and COX-2-Derived prostaglandins as key actors in the mechanisms involved. Cells, 9(9), 2005. https://doi.org/10.3390/cells9092005
- Authority, E. F. S. (2014). Refined exposure assessment for curcumin (E 100). EFSA Journal, 12(10), 3876.
- Bondesen, B. A., Mills, S. T., Kegley, K. M., & Pavlath, G. K. (2004). The COX-2 pathway is essential during early stages of skeletal muscle regeneration. American Journal of Physiology Cell Physiology, 287(2), C475–11. https://doi.org/10.1152/ajpcell.00088.2004
- Cannon, J. G., Fielding, R. A., Fiatarone, M. A., Orencole, S. F., Dinarello, C. A., & Evans, W. J. (1989). Increased interleukin 1 beta in human skeletal muscle after exercise. The American Journal of Physiology, 257(2 Pt 2), R451–455. https://doi.org/10.1152/ajpregu.1989.257.2.R451
- Chen, Y., Wang, J., Jing, Z., Ordovas, J. M., Wang, J., & Shen, L. (2022). Anti-fatigue and anti-oxidant effects of curcumin supplementation in exhaustive swimming mice via NRF2/Keap 1 signal pathway. Current Research in Food Science, 5, 1148–1157. https://doi.org/10.1016/j.crfs.2022.07.006
- Chung, H., Yoon, S. H., Cho, J. Y., Yeo, H. K., Shin, D., & Park, J. Y. (2021). Comparative pharmacokinetics of Theracurmin, a highly bioavailable curcumin, in healthy adult subjects. International Journal of Clinical Pharmacology and Therapeutics, 59(10), 684–690. https://doi.org/10.5414/CP204058
- Davis, J. M., Murphy, E. A., Carmichael, M. D., Zielinski, M. R., Groschwitz, C. M., Brown, A. S., Gangemi, J. D., Ghaffar, A., & Mayer, E. P. (2007). Curcumin effects on inflammation and performance recovery following eccentric exercise-induced muscle damage. American Journal of Physiology Regulatory, Integrative and Comparative Physiology, 292(6), R2168–2173. https://doi.org/10.1152/ajpregu.00858.2006
- Fernandez-Lazaro, D., Mielgo-Ayuso, J., Cordova Martinez, A., & Seco-Calvo, J. (2020). Iron and physical activity: Bioavailability enhancers, properties of Black Pepper (Bioperine®) and potential applications. Nutrients, 12(6), 1886. https://doi.org/10.3390/nu12061886
- Fernandez-Lazaro, D., Mielgo-Ayuso, J., Seco Calvo, J., Cordova Martinez, A., Caballero Garcia, A., & Fernandez-Lazaro, C. I. (2020). Modulation of exercise-induced muscle damage, inflammation, and oxidative markers by curcumin supplementation in a physically active population: A systematic review. Nutrients, 12(2), 501. https://doi.org/10.3390/nu12020501
- Fielding, R. A., Manfredi, T. J., Ding, W., Fiatarone, M. A., Evans, W. J., & Cannon, J. G. (1993). Acute phase response in exercise. III. Neutrophil and IL-1 beta accumulation in skeletal muscle. The American Journal of Physiology, 265(1 Pt 2), R166–172. https://doi.org/10.1152/ajpregu.1993.265.1.R166
- Forcina, L., Cosentino, M., & Musaro, A. (2020). Mechanisms regulating muscle regeneration: Insights into the interrelated and time-dependent phases of tissue healing. Cells, 9(5), 1297. https://doi.org/10.3390/cells9051297
- Funamoto, M., Shimizu, K., Sunagawa, Y., Katanasaka, Y., Miyazaki, Y., Kakeya, H., Yamakage, H., Satoh-Asahara, N., Wada, H., Hasegawa, K., & Morimoto, T. (2019). Effects of highly absorbable curcumin in patients with impaired glucose tolerance and non-insulin-dependent diabetes mellitus. Journal of Diabetes Research, 2019, 8208237. https://doi.org/10.1155/2019/8208237
- Funamoto, M., Sunagawa, Y., Katanasaka, Y., Miyazaki, Y., Imaizumi, A., Kakeya, H., Yamakage, H., Satoh-Asahara, N., Komiyama, M., Wada, H., Hasegawa, K., & Morimoto, T. (2016). Highly absorptive curcumin reduces serum atherosclerotic low-density lipoprotein levels in patients with mild COPD. International Journal of Chronic Obstructive Pulmonary Disease, 11, 2029–2034. https://doi.org/10.2147/COPD.S104490
- Garcea, G., Berry, D. P., Jones, D. J., Singh, R., Dennison, A. R., Farmer, P. B., Sharma, R. A., Steward, W. P., & Gescher, A. J. (2005). Consumption of the putative chemopreventive agent curcumin by cancer patients: Assessment of curcumin levels in the colorectum and their pharmacodynamic consequences. Cancer Epidemiology, Biomarkers & Prevention: A Publication of the American Association for Cancer Research, Cosponsored by the American Society of Preventive Oncology, 14(1), 120–125. https://doi.org/10.1158/1055-9965.120.14.1
- Ghafouri-Fard, S., Shoorei, H., Bahroudi, Z., Hussen, B. M., Talebi, S. F., Taheri, M., & Ayatollahi, S. A. (2022). Nrf2-related therapeutic effects of curcumin in different disorders. Biomolecules, 12(1), 82. https://doi.org/10.3390/biom12010082
- Gornicka, J., Mika, M., Wroblewska, O., Siudem, P., & Paradowska, K. (2023). Methods to improve the solubility of curcumin from turmeric. Life (Basel), 13(1), 207. https://doi.org/10.3390/life13010207
- Hamada, K., Vannier, E., Sacheck, J. M., Witsell, A. L., & Roubenoff, R. (2005). Senescence of human skeletal muscle impairs the local inflammatory cytokine response to acute eccentric exercise. FASEB Journal: Official Publication of the Federation of American Societies for Experimental Biology, 19(2), 264–266. https://doi.org/10.1096/fj.03-1286fje
- Haramizu, S., Ota, N., Hase, T., & Murase, T. (2013). Catechins suppress muscle inflammation and hasten performance recovery after exercise. Medicine and Science in Sports and Exercise, 45(9), 1694–1702. https://doi.org/10.1249/MSS.0b013e31828de99f
- HeeJung, P., Tadashi, H., Atsushi, I., & YoungJoo, A. (2015). Absorption improvement of curcumin powder using Theracurmin® CR-033P [absorption improvement of curcumin powder using Theracurmin® CR-033P]. Food Science and Industry, 48(2), 42–46. http://www.dbpia.co.kr/journal/articleDetail?nodeId=NODE06366316
- Hegde, M., Girisa, S., BharathwajChetty, B., Vishwa, R., & Kunnumakkara, A. B. (2023). Curcumin formulations for better bioavailability: What we learned from clinical trials thus far? American Chemical Society Omega, 8(12), 10713–10746. https://doi.org/10.1021/acsomega.2c07326
- Hirose, A., Kuwabara, Y., Kanai, Y., Kato, C., Makino, Y., Yoshi, F., & Sasaki, K. (2022). Comparative pharmacokinetics of new curcumin preparations and evidence for increased bioavailability in healthy adult participants. International Journal of Clinical Pharmacology and Therapeutics, 60(12), 530–538. https://doi.org/10.5414/CP204257
- Huang, W. C., Chiu, W. C., Chuang, H. L., Tang, D. W., Lee, Z. M., Wei, L., Chen, F. A., & Huang, C. C. (2015). Effect of curcumin supplementation on physiological fatigue and physical performance in mice. Nutrients, 7(2), 905–921. https://doi.org/10.3390/nu7020905
- Imaizumi, A. (2015). Highly bioavailable curcumin (Theracurmin): Its development and clinical application. PharmaNutrition, 3(4), 123–130. https://doi.org/10.1016/j.phanu.2015.08.002
- JECFA. (2004). Curcumin. (prepared by Ivan Stankovic). Chemical and Technical Assessment Compendıum Addendum 11/Fnp 52 Add. 11/29, Monographs 1.
- Kanai, M., Imaizumi, A., Otsuka, Y., Sasaki, H., Hashiguchi, M., Tsujiko, K., Matsumoto, S., Ishiguro, H., & Chiba, T. (2012). Dose-escalation and pharmacokinetic study of nanoparticle curcumin, a potential anticancer agent with improved bioavailability, in healthy human volunteers. Cancer Chemotherapy and Pharmacology, 69(1), 65–70. https://doi.org/10.1007/s00280-011-1673-1
- Kanai, M., Otsuka, Y., Otsuka, K., Sato, M., Nishimura, T., Mori, Y., Kawaguchi, M., Hatano, E., Kodama, Y., Matsumoto, S., Murakami, Y., Imaizumi, A., Chiba, T., Nishihira, J., & Shibata, H. (2013). A phase I study investigating the safety and pharmacokinetics of highly bioavailable curcumin (Theracurmin) in cancer patients. Cancer Chemotherapy and Pharmacology, 71(6), 1521–1530. https://doi.org/10.1007/s00280-013-2151-8
- Kawanishi, N., Kato, K., Takahashi, M., Mizokami, T., Otsuka, Y., Imaizumi, A., Shiva, D., Yano, H., & Suzuki, K. (2013). Curcumin attenuates oxidative stress following downhill running-induced muscle damage. Biochemical and Biophysical Research Communications, 441(3), 573–578. https://doi.org/10.1016/j.bbrc.2013.10.119
- Kunnumakkara, A. B., Bordoloi, D., Padmavathi, G., Monisha, J., Roy, N. K., Prasad, S., & Aggarwal, B. B. (2017). Curcumin, the golden nutraceutical: Multitargeting for multiple chronic diseases. British Journal of Pharmacology, 174(11), 1325–1348. https://doi.org/10.1111/bph.13621
- Kuwabara, Y., Hirose, A., Lee, H., Kakinuma, T., Baba, A., & Takara, T. (2023). Effects of highly bioavailable curcumin supplementation on common cold symptoms and immune and inflammatory functions in healthy Japanese subjects: A randomized controlled study. Journal of Dietary Supplements, 1–28. https://doi.org/10.1080/19390211.2023.2185723
- Lao, C. D., Ruffin, M. T. T., Normolle, D., Heath, D. D., Murray, S. I., Bailey, J. M., Boggs, M. E., Crowell, J., Rock, C. L., & Brenner, D. E. (2006). Dose escalation of a curcuminoid formulation. BMC Complementary and Alternative Medicine, 6(1), 10. https://doi.org/10.1186/1472-6882-6-10
- Lee, D. Y., Chun, Y. S., Kim, J. K., Lee, J. O., Ku, S. K., & Shim, S. M. (2021). Curcumin attenuates sarcopenia in chronic forced exercise executed aged mice by regulating muscle degradation and protein synthesis with antioxidant and anti-inflammatory effects. Journal of Agricultural and Food Chemistry, 69(22), 6214–6228. https://doi.org/10.1021/acs.jafc.1c00699
- Liczbinski, P., Michalowicz, J., & Bukowska, B. (2020). Molecular mechanism of curcumin action in signaling pathways: Review of the latest research. Phytotherapy Research: PTR, 34(8), 1992–2005. https://doi.org/10.1002/ptr.6663
- Maheshwari, R. K., Singh, A. K., Gaddipati, J., & Srimal, R. C. (2006). Multiple biological activities of curcumin: A short review. Life Sciences, 78(18), 2081–2087. https://doi.org/10.1016/j.lfs.2005.12.007
- Manas-Garcia, L., Guitart, M., Duran, X., & Barreiro, E. (2020). Satellite cells and markers of muscle regeneration during unloading and reloading: Effects of treatment with resveratrol and curcumin. Nutrients, 12(6), 1870. https://doi.org/10.3390/nu12061870
- Miodownik, C., Lerner, V., Kudkaeva, N., Lerner, P. P., Pashinian, A., Bersudsky, Y., Eliyahu, R., Kreinin, A., & Bergman, J. (2019). Curcumin as add-on to antipsychotic treatment in patients with chronic schizophrenia: A randomized, double-blind, placebo-controlled study. Clinical Neuropharmacology, 42(4), 117–122. https://doi.org/10.1097/wnf.0000000000000344
- Morimoto, T., Sunagawa, Y., Katanasaka, Y., Hirano, S., Namiki, M., Watanabe, Y., Suzuki, H., Doi, O., Suzuki, K., Yamauchi, M., Yokoji, T., Miyoshi-Morimoto, E., Otsuka, Y., Hamada, T., Imaizumi, A., Nonaka, Y., Fuwa, T., Teramoto, T. … Hasegawa, K. (2013). Drinkable preparation of Theracurmin exhibits high absorption efficiency–a single-dose, double-blind, 4-way crossover study. Biological & Pharmaceutical Bulletin, 36(11), 1708–1714. https://doi.org/10.1248/bpb.b13-00150
- Ms, S. A. B., Waldman Ph, D. H., Krings Ph, D. B., Lamberth Ph, D. J., Smith Ph, D. J., & McAllister Ph, D. M. (2020). Effect of curcumin supplementation on exercise-induced oxidative stress, inflammation, muscle damage, and muscle soreness. Journal of Dietary Supplements, 17(4), 401–414. https://doi.org/10.1080/19390211.2019.1604604
- Muller, W. A. (2001). New mechanisms and pathways for monocyte recruitment. Journal of Experimental Medicine, 194(9), F47–51. https://doi.org/10.1084/jem.194.9.f47
- Nakagawa, Y., Mori, K., Yamada, S., Mukai, S., Hirose, A., & Nakamura, R. (2022). The oral administration of highly-bioavailable curcumin for one year has clinical and chondro-protective effects: A randomized, double-blinded, placebo-controlled prospective study. Arthroscopy, Sports Medicine, and Rehabilitation, 4(2), e393–e402. https://doi.org/10.1016/j.asmr.2021.10.016
- Nakhostin-Roohi, B., Nasirvand Moradlou, A., Mahmoodi Hamidabad, S., & Ghanivand, B. (2016). The effect of curcumin supplementation on selected markers of delayed onset muscle soreness (DOMS). Annals of Applied Sport Science, 4(2), 25–31. https://doi.org/10.18869/acadpub.aassjournal.4.2.25
- Nanavati, K., Rutherfurd-Markwick, K., Lee, S. J., Bishop, N. C., & Ali, A. (2022). Effect of curcumin supplementation on exercise-induced muscle damage: A narrative review. European Journal of Nutrition, 61(8), 3835–3855. https://doi.org/10.1007/s00394-022-02943-7
- Pawar, Y. B., Shete, G., Popat, D., & Bansal, A. K. (2012). Phase behavior and oral bioavailability of amorphous curcumin. European Journal of Pharmaceutical Sciences, 47(1), 56–64. https://doi.org/10.1016/j.ejps.2012.05.003
- Powers, S. K., Deminice, R., Ozdemir, M., Yoshihara, T., Bomkamp, M. P., & Hyatt, H. (2020). Exercise-induced oxidative stress: Friend or foe? Journal of Sport and Health Science, 9(5), 415–425. https://doi.org/10.1016/j.jshs.2020.04.001
- Pulido-Moran, M., Moreno-Fernandez, J., Ramirez-Tortosa, C., & Ramirez-Tortosa, M. (2016). Curcumin and health. Molecules, 21(3), 264. https://doi.org/10.3390/molecules21030264
- Rahmani, S., Asgary, S., Askari, G., Keshvari, M., Hatamipour, M., Feizi, A., & Sahebkar, A. (2016). Treatment of non-alcoholic fatty liver disease with curcumin: A randomized placebo-controlled trial. Phytotherapy Research: PTR, 30(9), 1540–1548. https://doi.org/10.1002/ptr.5659
- Rai, A., Kaur, M., Gombra, V., Hasan, S., & Kumar, N. (2019). Comparative evaluation of curcumin and antioxidants in the management of oral submucous fibrosis. Journal of Investigative and Clinical Dentistry, 10(4), e12464. https://doi.org/10.1111/jicd.12464
- Ramasamy, T. S., Ayob, A. Z., Myint, H. H., Thiagarajah, S., & Amini, F. (2015). Targeting colorectal cancer stem cells using curcumin and curcumin analogues: Insights into the mechanism of the therapeutic efficacy. Cancer Cell International, 15(1), 96. https://doi.org/10.1186/s12935-015-0241-x
- Ross, S. M. (2018). Curcuma longa (Theracumin(r)): A bioavailable form of curcumin and its cognitive benefits. Holistic Nursing Practice, 32(4), 217–220. https://doi.org/10.1097/HNP.0000000000000281
- Sahin, K., Pala, R., Tuzcu, M., Ozdemir, O., Orhan, C., Sahin, N., & Juturu, V. (2016). Curcumin prevents muscle damage by regulating NF-kappaB and Nrf2 pathways and improves performance: An in vivo model. Journal of Inflammation Research, 9, 147–154. https://doi.org/10.2147/JIR.S110873
- Sasaki, H., Sunagawa, Y., Takahashi, K., Imaizumi, A., Fukuda, H., Hashimoto, T., Wada, H., Katanasaka, Y., Kakeya, H., Fujita, M., Hasegawa, K., & Morimoto, T. (2011). Innovative preparation of curcumin for improved oral bioavailability. Biological & Pharmaceutical Bulletin, 34(5), 660–665. https://doi.org/10.1248/bpb.34.660
- Saud Gany, S. L., Chin, K. Y., Tan, J. K., Aminuddin, A., & Makpol, S. (2023). Curcumin as a therapeutic agent for sarcopenia. Nutrients, 15(11), 2526. https://doi.org/10.3390/nu15112526
- Schwane, J. A., Johnson, S. R., Vandenakker, C. B., & Armstrong, R. B. (1983). Delayed-onset muscular soreness and plasma CPK and LDH activities after downhill running. Medicine and Science in Sports and Exercise, 15(1), 51–56. https://doi.org/10.1249/00005768-198315010-00010
- Shaikh, J., Ankola, D. D., Beniwal, V., Singh, D., & Kumar, M. N. (2009). Nanoparticle encapsulation improves oral bioavailability of curcumin by at least 9-fold when compared to curcumin administered with piperine as absorption enhancer. European Journal of Pharmaceutical Sciences, 37(3–4), 223–230. https://doi.org/10.1016/j.ejps.2009.02.019
- Sharma, R. A., Euden, S. A., Platton, S. L., Cooke, D. N., Shafayat, A., Hewitt, H. R., Marczylo, T. H., Morgan, B., Hemingway, D., Plummer, S. M., Pirmohamed, M., Gescher, A. J., & Steward, W. P. (2004). Phase I clinical trial of oral curcumin: Biomarkers of systemic activity and compliance. Clinical Cancer Research, 10(20), 6847–6854. https://doi.org/10.1158/1078-0432.CCR-04-0744
- Small, G. W., Siddarth, P., Li, Z., Miller, K. J., Ercoli, L., Emerson, N. D., Martinez, J., Wong, K. P., Liu, J., Merrill, D. A., Chen, S. T., Henning, S. M., Satyamurthy, N., Huang, S. C., Heber, D., & Barrio, J. R. (2018). Memory and brain amyloid and tau effects of a bioavailable form of curcumin in non-demented adults: A double-blind, placebo-controlled 18-month trial. The American Journal of Geriatric Psychiatry: Official Journal of the American Association for Geriatric Psychiatry, 26(3), 266–277. https://doi.org/10.1016/j.jagp.2017.10.010
- Sugawara, J., Akazawa, N., Miyaki, A., Choi, Y., Tanabe, Y., Imai, T., & Maeda, S. (2012). Effect of endurance exercise training and curcumin intake on central arterial hemodynamics in postmenopausal women: Pilot study. American Journal of Hypertension, 25(6), 651–656. https://doi.org/10.1038/ajh.2012.24
- Sugimoto, K., Ikeya, K., Bamba, S., Andoh, A., Yamasaki, H., Mitsuyama, K., Nasuno, M., Tanaka, H., Matsuura, A., Kato, M., Ishida, N., Tamura, S., Takano, R., Tani, S., Osawa, S., Nishihira, J., & Hanai, H. (2020). Highly bioavailable curcumin derivative ameliorates Crohn’s disease symptoms: A randomized, double-blind, multicenter study. Journal of Crohn’s & Colitis, 14(12), 1693–1701. https://doi.org/10.1093/ecco-jcc/jjaa097
- Takahashi, M., Suzuki, K., Kim, H. K., Otsuka, Y., Imaizumi, A., Miyashita, M., & Sakamoto, S. (2014). Effects of curcumin supplementation on exercise-induced oxidative stress in humans. International Journal of Sports Medicine, 35(6), 469–475. https://doi.org/10.1055/s-0033-1357185
- Tanabe, Y., Chino, K., Ohnishi, T., Ozawa, H., Sagayama, H., Maeda, S., & Takahashi, H. (2019). Effects of oral curcumin ingested before or after eccentric exercise on markers of muscle damage and inflammation. Scandinavian Journal of Medicine & Science in Sports, 29(4), 524–534. https://doi.org/10.1111/sms.13373
- Tanabe, Y., Chino, K., Sagayama, H., Lee, H. J., Ozawa, H., Maeda, S., & Takahashi, H. (2019). Effective timing of curcumin ingestion to attenuate eccentric exercise-induced muscle soreness in men. Journal of Nutritional Science and Vitaminology, 65(1), 82–89. https://doi.org/10.3177/jnsv.65.82
- Tanabe, Y., Maeda, S., Akazawa, N., Zempo-Miyaki, A., Choi, Y., Ra, S. G., Imaizumi, A., Otsuka, Y., & Nosaka, K. (2015). Attenuation of indirect markers of eccentric exercise-induced muscle damage by curcumin. European Journal of Applied Physiology, 115(9), 1949–1957. https://doi.org/10.1007/s00421-015-3170-4
- Thaloor, D., Miller, K. J., Gephart, J., Mitchell, P. O., & Pavlath, G. K. (1999). Systemic administration of the NF-kappaB inhibitor curcumin stimulates muscle regeneration after traumatic injury. The American Journal of Physiology, 277(2), C320–329. https://doi.org/10.1152/ajpcell.1999.277.2.C320
- Tidball, J. G., Dorshkind, K., & Wehling-Henricks, M. (2014). Shared signaling systems in myeloid cell-mediated muscle regeneration. Development, 141(6), 1184–1196. https://doi.org/10.1242/dev.098285
- Trujillo, J., Chirino, Y. I., Molina-Jijon, E., Anderica-Romero, A. C., Tapia, E., & Pedraza-Chaverri, J. (2013). Renoprotective effect of the antioxidant curcumin: Recent findings. Redox Biology, 1(1), 448–456. https://doi.org/10.1016/j.redox.2013.09.003
- Tsai, S.-W., Huang, C.-C., Hsu, Y.-J., Chen, C.-J., Lee, P.-Y., Huang, Y.-H., Lee, M.-C., Chiu, Y.-S., & Tung, Y.-T. (2020). Accelerated muscle recovery after in vivo curcumin supplementation. Accelerated Muscle Recovery After in vivo Curcumin Supplementation Natural Product Communications, 15(1), 1934578X20901898. https://doi.org/10.1177/1934578x20901898
- Uderhardt, S., Martins, A. J., Tsang, J. S., Lammermann, T., & Germain, R. N. (2019). Resident macrophages cloak tissue microlesions to prevent neutrophil-driven inflammatory damage. Cell, 177(3), 541–555 e517. https://doi.org/10.1016/j.cell.2019.02.028
- Vargas-Mendoza, N., Morales-Gonzalez, A., Madrigal-Santillan, E. O., Madrigal-Bujaidar, E., Alvarez-Gonzalez, I., Garcia-Melo, L. F., Anguiano-Robledo, L., Fregoso-Aguilar, T., & Morales-Gonzalez, J. A. (2019). Antioxidant and adaptative response mediated by Nrf2 during physical exercise. Antioxidants (Basel), 8(6), 196. https://doi.org/10.3390/antiox8060196
- Wafi, A. M., Hong, J., Rudebush, T. L., Yu, L., Hackfort, B., Wang, H., Schultz, H. D., Zucker, I. H., & Gao, L. (2019). Curcumin improves exercise performance of mice with coronary artery ligation-induced HFrEF: Nrf2 and antioxidant mechanisms in skeletal muscle. Journal of Applied Physiology: Respiratory, Environmental and Exercise Physiology, 126(2), 477–486. https://doi.org/10.1152/japplphysiol.00654.2018
- Wang, X., & Zhou, L. (2022). The many roles of macrophages in skeletal muscle injury and repair. Frontiers in Cell and Developmental Biology, 10, 952249. https://doi.org/10.3389/fcell.2022.952249
- Yong-Hwan, K., Kyung-Ok, S., & Kyung-Soon, C. (2016). In vitro antioxidant properties of equisetum arvense and its effects on serum lipid levels in mice fed a high-fat diet. The Korean Journal of Food and Nutrition, 29(3), 347–356. https://doi.org/10.9799/ksfan.2016.29.3.347
- Zia, A., Farkhondeh, T., Pourbagher-Shahri, A. M., & Samarghandian, S. (2021). The role of curcumin in aging and senescence: Molecular mechanisms. Biomedicine & Pharmacotherapy, 134, 111119. https://doi.org/10.1016/j.biopha.2020.111119