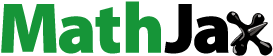
ABSTRACT
This study aimed to devise a novel fermentation unit for yogurt production utilizing phase change materials (PCM) and primarily powered by solar energy. The unit comprises a solar flat plate collector (FPC), PCM cells, and a fermentation cabinet equipped with trays. High-density polyethylene (HDPE) cylindrical cells were employed to contain paraffin wax for thermal energy storage. The performance of the integrated solar system for the fermentation process was assessed across different air velocities (1.2 to 1.8 m/s), with the most rapid temperature rise observed at 1.8 m/s. The PCM-based thermal storage exhibited an impressive capability of releasing heat autonomously for 6 hours without sunlight, eliminating the need for external energy sources. PCM’s capacity to store and release thermal energy ensures fermentation stability, even without solar radiation. This innovative approach offers a compelling environmentally friendly solution with significant implications for sustainable yogurt production, paving the way for industrial integration.
1. Introduction
There has been an urgent quest for alternative energy sources because of the rapid depletion of fossil fuel resources. Amidst various options, solar energy emerges as a highly promising long-term solution to address the escalating energy demand and as per IEA (International Energy Agency) forecast, solar will supply around 45% of global energy demand by 2050 (Brahma et al., Citation2023). Abundant, readily available, and environmentally clean, solar energy offers a native, pollution-free energy source (Kumar et al., Citation2023). The most effective and direct method to harness solar energy involves its conversion into thermal energy, particularly for heating purposes through the utilization of solar collectors, such as solar air heaters (Kumar et al., Citation2021; Pathak et al., Citation2023).
Solar air heaters (SAHs) have gained widespread adoption for conserving energy, particularly in scenarios requiring lower to moderate air temperatures. These heaters present numerous advantages over liquid-based heaters, notably steering clear of issues like freezing, stagnation, leaks, damages, and potential environmental or health risks associated with the heat transfer medium (Goel et al., Citation2021; Qasem et al., Citation2020). Additionally, they contribute to lowering energy consumption expenses for the specific application. SAHs are characterized by its simplicity, comprising a glass enclosure and an absorber plate with insulation, rendering it a cost-effective solution. Remarkably, its design obviates the need for a heat exchanger, as it directly heats the air. Subsequently, the warmed air is channelled into the designated space heating chamber, exemplifying its efficacy for practical application (Benhamza et al., Citation2021; Muthukumaran & Senthil, Citation2022; Olivkar et al., Citation2022).
Several notable strategies have been employed to enhance the performance of solar air heaters (SAHs), encompassing optimization of critical dimensions, implementation of extended surfaces or artificial roughness featuring diverse shapes and sizes, utilization of sensible or latent storage media (thermal energy storage), integration of concentrators to amplify solar radiation availability, and incorporation of photovoltaic elements alongside the heaters (Brahma et al., Citation2023; Pathak et al., Citation2023). Among these, thermal energy storage (TES) serves as a pivotal tool for energy conservation, elevating the overall efficiency of SAH systems. By integrating TES, the reliability of SAHs is bolstered, mitigating the challenges posed by the intermittent nature of solar radiation. Among various TES systems, latent heat storage utilizing phase change materials (PCMs) emerges as particularly promising due to its advantageous attributes such as heightened energy storage density and the ability to retain and release thermal energy at relatively consistent temperatures (Assadeg et al., Citation2021; Sharma et al., Citation2021). Extensive investigations into PCM application have explored a spectrum of operating conditions, ranging from cooling at sub-zero temperatures (as low as −20°C) to heating at elevated temperatures (up to 200°C). The findings of such analyses are invaluable for identifying the most suitable PCM for specific operational requirements (Aftab et al., Citation2021; Nagappan et al., Citation2023).
Paraffin wax is one of the most commonly used PCM in various applications, including building heating, thermal energy storage, and refrigeration. In the context of solar flat plate collectors, paraffin wax has been extensively studied as a PCM due to its attractive properties, such as low-cost, high-energy density, and long-term thermal stability (Babar et al., Citation2020). Paraffin wax is a solid–liquid PCM with a relatively narrow melting range, making it suitable for use in solar flat plate collectors (Javadi et al., Citation2020). When added to the collector, the paraffin wax absorbs excess heat and stores it in the form of latent heat, which can then be released as needed. The amount and type of paraffin wax used in the system can be adjusted to match the specific heating requirements, and the system can be designed to ensure optimal heat transfer and storage (Abu-Hamdeh et al., Citation2022).
People are becoming more concerned about their health, leading to a growing demand for healthy food products. The fermented dairy industry plays a crucial role in meeting this demand with products like yogurt which have various health benefits like including the prevention of osteoporosis, diabetes, and cardiovascular diseases, as well as the promotion of gut health and immune system modulation (Freitas, Citation2017; Gao et al., Citation2021; Hadjimbei et al., Citation2022; Itsaranuwat et al., Citation2003; Rastogi et al., Citation2022). According to the committee on the medical aspects of food policy, one cup of yoghurt (245 g) provides 40% of the reference nutrient intakes (RNI) for calcium, 40% for phosphorus, 10% for potassium, 10% for vitamin A, 30% for vitamin B2, and 60% for vitamin B12 for males and females aged 19–50 (Saleem et al., Citation2024). These products are made by fermenting milk using lactic acid bacteria.
Dairy is regarded as the fifth most energy-consuming industry since the process of manufacturing yogurt from raw milk requires a lot of energy (Husnain et al., Citation2022). Fossil fuels are still used to meet a large portion of the energy demand in the dairy processing industry (Ladha-Sabur et al., Citation2019), which raises operating costs and pollutes the environment (Osman et al., Citation2023). Solving these issues through the use of renewable energy in yogurt production can pave the way for the creation of solar-powered yogurt fermentation equipment.
There is potential to tap solar energy for processes like fermentation. Various studies have been conducted on usage of solar energy in food fermentation for past 10 years (), and therefore a solar-based equipment was developed for yogurt fermentation. This study presents a promising alternative for sustainable yogurt production using a solar-powered fermentation unit with PCM-based thermal energy storage. One of the challenges was to maintain the temperature of air heated using a solar flat plate collector. In the present research, this problem was addressed using process automation and an innovative design combination of temperature controller, air blower, recirculation duct, and PCM cells.
2. Materials and methods
2.1. Design simulation
The assessment of fermentation cabinet stability was conducted by employing stress analysis using ANSYS Static Structural software. Von Mises stress served as a critical measure to determine potential material yielding or failure. The Von Mises yield criterion states that if the Von Mises stress within a material subjected to load surpasses the yield limit of that material under simple tension, it will likely yield or deform. Utilizing Von Mises stress in the model was essential as it signifies the distortion energy present in a material. It asserts that two stress states with equivalent distortion energy will yield the same Von Mises stress value (Lou et al., Citation2017). To analyse the stress levels within the cabinet, a computer simulation was conducted using the ANSYS software. The process began by creating a three-dimensional representation of the cabinet using the ANSYS Design Modeler. This model was then imported into the ANSYS Workbench where the material properties, such as yield stress and Poisson's ratio, were specified. The model was then discretized into a finite element mesh, allowing for the application of boundary conditions such as pressure, temperature, and fixed support. After these steps were completed, the calculation was run to determine the levels of stress within the cabinet.
2.2. Experimental setup
The developed system has a double pass solar flat plate collector (FPC) having provision to load PCM cells, a blower assembly, a fermentation cabinet, and a temperature controller (). Flat plate solar collector was selected for the present study as it operates within the temperature range of 40°C to 60°C. Despite the diverse assortment of solar energy capturing products available in the market, flat plate solar collectors have managed to maintain a significant market share owing to their uncomplicated design and relatively lower cost (Kizildag et al., Citation2022). The FPC has an area of 0.7 m2, and an absorber plate thickness of 0.12 cm with a tilt angle of 39°. The fermentation cabinet was designed using the computational fluid dynamic (CFD) approach having a volume of 0.134 m3 (volumetric capacity: 21 liters). The curd cups (84 cups 250 ml) were placed on four shelves inside the fermentation cabinet. The air was heated using a solar collector and was blown into the fermentation cabinet by an axial fan. Temperature controller (Mode: Selec TC513) was used to control and maintain a constant temperature inside the cabinet. The air blower was automatically shut off once the temperature exceeded the set point temperature. An electric heater was provided to operate the fermentation unit in the absence of sunlight.
2.2.1. PCM cells
Paraffin wax was chosen as PCM because of its required application temperature and thermal characteristics (Babar et al., Citation2020; Nazir et al., Citation2019). Another reason is that the melting and solidification temperature was 10°C to 20°C higher than the optimal yogurt fermentation temperature (37–42°C). The thermal and physical characteristics of paraffin wax used in the study are presented in .
Table 1. Thermophysical properties of PCM.
The computation of energy to be stored in the phase change material Qs (kJ) was calculated using the EquationEquation 1(1)
(1) :
Where, Qs is the energy needed per hour (kJ/h), ts is storage back up time in (h).
The total mass of PCM, Mpcm (kg) required was calculated from the EquationEquation 2.(2)
(2)
Where Lpcm is the latent heat of paraffin wax (383.87 kJ/kg).
[The heat load (Qt) for yogurt incubation for 1 hour is 1176 KJ, and considering 5 hour of incubation period, total heat load required is Qs = 5880 KJ. From EquationEquation 2(2)
(2) , the total mass of PCM required was 15.31 kg].
High-density polyethylene (HDPE) bottle (Diameter = 0.075 m, height = 0.15 m, volume = 0.250 liters) was used as a PCM container. Dimension of cylindrical geometry was selected to fit on the corrugated absorber plate and to aid in heat transfer. The orientation of the container had an impact on the heat transfer between the air and PCM. Therefore, the PCM cells were placed horizontally on the absorber plate for optimal heat transfer (). The cells were painted black to effectively absorb the radiation.
2.3. Performance evaluation
Milk (4.5% fat and 8.5% SNF) was collected from the Experimental Dairy Plant, ICAR-National Dairy Research Institute, Karnal, and standardized to 14% TS (total solids). The flowchart for the manufacturing of yogurt is given in (Aneja et al., Citation2002). The performance evaluation of the unit was carried out by conducting two sets of experiments viz. no load and full-load test. Trials were conducted during sunny hours (9 AM to 4:30 PM) in which PCM cells absorbed stored heat energy for an extended period of time. During the discharging process, PCM cells released heat into the air which helped to maintain the temperature in the fermentation cabinet. The PCM containers were charged from 9:30 AM to 4:30 PM during the sunshine hours, and yogurt was prepared during the discharge hours from 4:30 PM to the set incubation period.
2.3.1. No load testing
A no-load test was conducted to assess the design features of the developed system without yogurt cups inside the fermentation cabinet. The parameters such as air velocity and temperature in various locations inside the fermentation cabinet were monitored. Temperatures were recorded on four different shelves inside the cabinet and at different locations inside the solar FPC using a temperature data logger equipped with multiple pt100 sensors. No load test was conducted at an air velocity of 1.8 m/s.
2.3.2. Full load testing
The solar hybrid system was evaluated at full load with 100 ml cups filled with milk inoculated with yogurt cultures (Lactobacillus bulgaricus and Streptococcus thermophiles). Since the optimal temperature is crucial for the fermentation process and for the proper setting of curd, a PID temperature controller (Model Selec TC-513, India) was used to operate the air blower and maintain the temperature inside the fermentation cabinet. Full-load testing was performed on the solar heating system at three different air velocities: 1.2 m/s, 1.5 m/s, and 1.8 m/s. The tests were conducted for 3–4 hours from 10 AM to 3 PM. Temperature sensors (pt100 type) were placed inside the yogurt cups on each tray inside the cabinet to monitor temperature during the incubation period.
2.4. Comparison of yogurt prepared in the developed solar unit and conventional method
Yogurt was prepared in the solar fermentation system, and its quality attributes were compared with yogurt prepared using the conventional method in an electrically heated incubator. The comparison was done based on several parameters including the compositional analysis of yogurt, such as moisture, protein, fat, lactose, and acidity. Additionally, pH and textural profile parameters were determined, and yogurt was subjected to sensory evaluation.
2.4.1. Compositional analysis
The compositional analysis was done as per the methods of AOAC (AOAC, Citation2005). Moisture content of the samples was evaluated using the gravimetric method. Fat content was determined using the Gerber method. The protein content was determined using the Micro-Kjeldahl method. Lactose content was determined using Lane and Eynon method. The pH was measured as a function of time using a digital pH controller (Model 110-pH, Countron, India). The titration method was used to determine the acidity of yoghurt samples.
2.4.2. Texture Profile Analysis (TPA)
The Texture Profile Analysis (TPA) was conducted utilizing the TA.HD plus Texture Analyser manufactured by Stable Micro Systems Ltd, United Kingdom. The test involved performing compression experiments to observe the force (measured in grams) against time (measured in seconds) of the samples. To measure the texture profile, a 25 mm diameter cylindrical probe was utilized, inserted into a 100 mL cup maintained at a temperature of 10 ± 5°C, with five repetitions. During the initial stage of the test, the samples were compressed by 30% of their original depth, while the probe’s speed was maintained at a constant 0.5 mm/s for both the pre-test compression and the relaxation of the samples. The maximum force registered in TPA was used as an indicator of the sample’s firmness, with a higher value indicating greater firmness. The consistency of the sample was assessed by measuring the area under the curve up to this point; a larger value suggests a thicker consistency. Upon the probe’s return, the weight of the sample was borne on the upper surface of the disc, leading to back extrusion and causing the negative region on the graph. This negative region provides insights into the sample’s resistance to flow off the disc. The cohesiveness of the sample was determined by identifying the highest negative force in the TPA plot; a higher negative force signifies greater cohesiveness. Additionally, the area of the negative zone under the curve represents the work of cohesion, indirectly indicating the sample’s resistance to the probe’s withdrawal. This parameter serves as an indirect measure of cohesiveness and viscosity (Angioloni & Collar, Citation2009).
2.4.3. Sensory evaluation
The sensory evaluation employed the 9-point hedonic scale, involving a panel of 20 members tasked with rating the sample on a scale from 1 to 9, where 1 denoted “dislike extremely,” and 9 represented “like extremely.” Evaluation focused on key sensory attributes including color and appearance, flavor, body and texture, acidity, and overall acceptability. Panellists assessed these attributes by assigning scores on the scale, reflecting their preferences and perceptions.
2.5. Statistical analysis
The experiments were performed in triplicates. The experimental data were analyzed using R software (R Foundation, Indianapolis, Indiana, United States) and results were plotted in Origin Pro Ver 8.0 (OriginLab Corporation, United States) and Excel (Microsoft Corporation, United States). The difference between the mean of groups was analyzed using One-Way Analysis of Variance (ANOVA) with a level of significance at p < .05.
3. Results and discussions
3.1. Design simulation
The evaluation of the design involved an assessment of Von Mises stresses using ANSYS software, incorporating a factor of safety to ensure adequacy for a 3 mm wall thickness. Employing the Von Mises stress analysis technique enabled the simulation of intricate loading scenarios. The outcomes depicted in reveals a peak stress of 136.25 MPa in one area of the unit, whereas the remainder exhibits considerably lower stress levels. Notably, the simulation indicated a Von Mises stress of 135 MPa in the chamber, significantly below the yield stress of AISI 1018 MS (250 MPa) (Sapto, Citation2010). Correspondingly, depicts the deformations resulting from stress analysis.
Further numerical analysis expanded to predict the cabinet’s expected deformation owing to the induced stress. Careful examination, corroborated by the color legend panel, revealed that the region with the highest stress coincided with the area exhibiting maximum deformation (2.5589 × 10−6 m), aligning with prior findings (Shaikh & Nitnaware, Citation2013). Moreover, an additional numerical analysis computed the factor of safety for the unit’s design, depicted in . Inspection of the color legend unveiled a minimum factor of safety of 1.8348 within the highest stress area, surpassing the safety threshold of 1.0 affirming the design’s safety (Kraan et al., Citation2004).
3.2. Performance evaluation under no load testing conditions
The performance of the developed system was evaluated without any product load to study the temperature distribution inside the fermentation cabinet. The maximum solar radiation intensity recorded was 966 W/m2 at 1 PM and the temperature vs time plot suggested that the air temperature range was suitable for yogurt production (). It was observed that the temperature distribution was non-uniform in the absence of the temperature controller. During sunny hours (8 AM to 6 PM), the maximum temperature of the air entering the cabinet was 65.6°C. The peak temperature inside the cabinet was 53.5°C in tray 1, followed by tray 2 (50.9°C), tray 3 (50.5°C), and tray 4 (43°C), while the average ambient air temperature was 38.85°C. Such large temperature variations have also been reported in other studies. The average temperature for collector outlet from indirect-type solar dryer tray 1, tray 2, tray 3, and tray 4 was observed as 61.2°C, 55°C, 52°C, 47°C, 44°C, and 35°C, respectively (Lingayat et al., Citation2017). The study shows that temperature difference exists in a stacked tray design. One of the possible approaches to minimize temperature variations inside the fermentation cabinet was by use of an air blower. No load performance test suggested the need for a temperature controller to maintain the temperature in the narrow range (37°C to 42°C).
3.3. Performance evaluation under full load testing conditions
The performance of the solar fermentation system was tested by loading the 60 cups (200 ml) containing standardized milk (inoculated with cultures) into the fermentation cabinet. The maximum temperature achieved at the collector outlet at different air velocities was 65.6°C (1.8 m/s), 60.1°C (1.5 m/s), and 54°C (1.2 m/s). The relationship of temperature with air velocity is in agreement with the findings of Çakmak and Yıldız (Citation2011). In a similar study, the maximum outlet temperatures reached by the solar air heater with PCM was in the range 79.5–82°C. The outlet temperature without PCM was 10°C lower compared to the collector with PCM (Palacio et al., Citation2022). The present study also demonstrates that PCM has an important role in maintaining temperature for the fermentation process.
The results indicated that an air velocity of 1.8 m/s was most suitable for yogurt production, as it took only 40 minutes to achieve an air temperature of 60°C as compared to 235 minutes at 1.5 m/s. In the case of 1.2 m/s maximum air temperature was 54°C. shows the temperature profile inside the cabinet at three different air velocities for yogurt production at a set temperature of 43°C and incubation time of 4 hours. The cups were loaded at an initial temperature of 37°C and the time required to achieve a set point temperature was 30, 50, and 70 min at air velocities of 1.8, 1.5, and 1.2 m/s, respectively. The temperature rise was rapid at 1.8 m/s due to the better airflow distribution inside the cabinet. The temperature controller was able to maintain uniform temperature distribution for the incubation of yogurt.
Figure 7. Temperature profile inside the cabinet during yogurt production at full load testing conditions (a) Air velocity = 1.8 m/s; (b) Air velocity = 1.5 m/s; (c) Air velocity = 1.2 m/s.
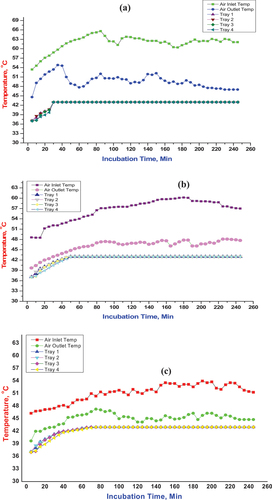
The heat energy gain value for the solar flat plate collector was 485 W with collector efficiency of 71.72%. The corrugated base design could be one possible reason for high collector efficiency. The thermal efficiency of flat-plate collectors has been reported to be in the range of 33.8–62.6% which can be improved up to 94.8% using collector design modification (Mutar & Alaiwi, Citation2023).
3.4. Thermal performance of PCM during yogurt incubation
The trials were performed by loading the samples into the cabinet at 4:30 PM and allowing the cups to incubate for 4 hours. PCM-based thermal storage could effectively provide heat for fermentation for an extended period of time. shows the temperature profile of the PCM containers inside the FPC and a maximum temperature of 80.4°C was recorded in the container located at the center of the collector. Peak temperatures of PCM at other locations were 75.8°C, 69.5°C, 69.4°C, and 63.3°C. The containers effectively absorbed and stored solar energy. During the discharge period and simultaneous yogurt fermentation, average temperature of 58.05°C and 54°C was recorded after 4 and 5 hours, respectively.
These results demonstrate that the design calculation for PCM was adequate and matched the equipment capacity for the production of yogurt. Lower temperatures (<40°C) have been reported to slow down yogurt production, while elevated temperatures (>42°C) result in the highest fermentative rates. Acidification rate and gel formation are highly affected by temperature. Viscous and smoother yogurt is obtained when the temperature process is lowered from 43–45°C to less than 40°C. One major advantage of a higher incubation temperature (43°C) is the reduction in fermentation time required to produce yogurt (Lopes et al., Citation2019).
During the PCM charging, the highest air inlet temperature was 66.7°C. During the discharging period, an air temperature of 53.54°C was recorded after 5 hours. In a similar research work, paraffin wax-based PCM was used as a thermal energy reservoir (TER) in a solar dryer with an onset temperature of 59.2°C and the end of the melting cycle was computed at 66°C (Babar et al., Citation2020). shows the temperature profile inside the fermentation cabinet during yogurt production. PCM thermal storage was able to raise the inlet air temperature to a maximum of 66.7°C during the incubation process. It took around 40 minutes to reach the set temperature (42°C) for yogurt. The study demonstrated that PCM cells can provide a sustained supply of heat required during the yogurt fermentation process and maintain the temperature in a desirable range.
3.5. Comparison of yogurt prepared in the developed solar unit and conventional method
3.5.1. Compositional analysis
The compositional analysis showed a non-significant difference (p > .05) in quality attributes of yogurt prepared using conventional and solar fermentation systems. The moisture, fat, protein, and lactose content values are given in . The proximate composition of the yogurt sample was similar to that reported for plain yogurt in various research studies (Baspinar & Güldaş, Citation2021; Rahman et al., Citation2020). The compositional similarity is because the general composition of yogurt depends on the initial milk composition and starter cultures used for fermentation (Farag et al., Citation2022; Soni et al., Citation2020).
Table 2. Chemical composition of yogurt prepared in conventional and solar fermentation system.
3.5.2. pH profile
The objective of measuring pH continuously during yogurt fermentation was to monitor pH changes during the fermentation process of yogurt. The pH profile was computed as a plot between pH Vs time (min). An initial pH of 6.8 at the start of fermentation was an indicator to detect acidity development due to prolonged storage or any deviation from the production process flow parameters. The final yogurt pH of 4.6 marked the completion of incubation (). pH profile gave an overall view of how well fermentation proceeded in solar and electric-based systems. The drop in yogurt pH was slower in the case of PCM than in the conventional unit but it did not affect the end point pH. There was no significant difference (p > .05) between conventional and solar-fermented yogurt, Bulut et al. (Citation2021) reported a similar pH profile for plain yogurt while studying the effects of yogurt fortification.
3.5.3. Texture profile analysis
The yogurt samples were analyzed for firmness, consistency, cohesion, and work of cohesion, and it was found that the quality of product obtained from the conventional and solar-based systems were similar. The values of TPA parameter are given in . The work of cohesion values was in the range of −118.5 to −119.5 g.sec. The work of cohesion may be referred to as index of viscosity and higher the value, the more difficult it is to remove the sample. It is a parameter that is directly related to cohesion and consistency (Syahariza & Yong, Citation2017). The cohesiveness value is related to the internal forces holding the product together, suggesting that a larger number of linkages within the gel network, which were disrupted during stress application, were reconstructed after the stress was released (Najgebauer-Lejko et al., Citation2020). The higher cohesiveness of solar-prepared yogurt (−15.9 g) compared to conventional yogurt (−16.2 g) suggests better gel strength.
Table 3. Textural profile analysis of yogurt prepared using conventional and solar-based fermentation system.
3.5.4. Sensory evaluation
The sensory qualities of yogurt are critical in determining its acceptability among consumers. The sensory evaluation was conducted using a nine-point hedonic scale, assessing the attributes like colour and appearance, flavour, body and texture, acidity, and overall acceptability of yogurt samples. The sensory evaluation results are shown in . There was no significant (p > .05) difference in the sensory qualities of yogurt prepared in conventional and solar fermentation system. Colour and appearance are the initial sensory parameters that influence the perception of the product quality by the consumer. The colour of yogurt is a parameter dependent on the degree of light reflection and absorption (Kim et al., Citation2020). Flavour perception is a complex phenomenon and is closely related to taste and aroma and its score was in the range of 8.9–8.99. Yoghurt has a distinct flavour and is distinguished by its high concentration of volatile metabolites. These components are by-products of lactic acid fermentation or are formed by other reaction mechanisms. Lactic acid is one of the key compounds produced during yogurt fermentation that contribute significantly to its flavour (Routray & Mishra, Citation2011). The texture is another important aspect of yogurt, especially for set yogurt that is related to its mouthfeel. Yogurt’s texture can range from smooth and creamy to thick and viscous. The protein concentration, fat content, and fermentation period all influence the gel strength of yogurt (Zhao et al., Citation2021). Acidity is a desirable attribute of yogurt, which is related to its pH level. The acidity of yogurt is influenced by the type of starter culture used and the fermentation time. The acidity of sourness scores of 8.93–8.94 shows the level of likeness by the panel members. Overall, acceptability values above 8 indicates a high degree of acceptance by the consumers for both the yogurt samples prepared using conventional and solar fermentation unit.
4. Conclusion
The study successfully tested a batch fermentation unit integrated with PCM cells as thermal energy storage for producing yogurt using solar energy. The fermentation cabinet stability was assessed using ANSYS Static Structural software and found to be stable. The performance evaluation of the system showed that air velocity of 1.8 m/s was most suitable for the cabinet. Paraffin wax PCM cells were effectively absorbed and stored the thermal energy. During PCM charging, a maximum temperature of 80.4°C was recorded in the container located at the centre of the collector, and peak temperatures at other locations were 75.8°C, 69.5°C, 69.4°C, and 63.3°C respectively. The PCM cells were able to provide heat for up to 6 hours in the absence of sunlight. The results showed no significant difference in product quality over conventional manufacturing methods, which makes this system a promising alternative to electricity-based fermentation units. The automation unit used with the solar fermentation system effectively controlled the air temperature and provided optimum conditions for yogurt production.
Author contributions
The authors contributed equally to this work. All authors have read and approved the final version of the manuscript.
Acknowledgment
The first author acknowledges the ICAR fellowship and funding received from ICAR-NDRI to carry out the research work. This project was supported by Researchers Supporting Project number (RSP2024R315) King Saud University, Riyadh, Saudi Arabia.
Disclosure statement
No potential conflict of interest was reported by the author(s).
Data availability statement
We confirm that all the data concerning this manuscript will be available upon request.
Additional information
Funding
References
- Abu-Hamdeh, N. H., Khoshaim, A., Alzahrani, M. A., & Hatamleh, R. I. (2022). Study of the flat plate solar collector’s efficiency for sustainable and renewable energy management in a building by a phase change material: Containing paraffin-wax/Graphene and paraffin-wax/graphene oxide carbon-based fluids. Journal of Building Engineering, 57, 104804. https://doi.org/10.1016/j.jobe.2022.104804
- Aftab, W., Usman, A., Shi, J., Yuan, K., Qin, M., & Zou, R. (2021). Phase change material-integrated latent heat storage systems for sustainable energy solutions. Energy & Environmental Science, 14(8), 4268–12. https://doi.org/10.1039/D1EE00527H
- Aneja, R. P., Mathur, B. N., Chandan, R. C., & Banerjee, A. K. (2002). Technology of Indian milk products: Handbook on process technology modernization for professionals, entrepreneurs and scientists. Dairy India Yearbook.
- Angioloni, A., & Collar, C. (2009). Small and large deformation viscoelastic behaviour of selected fibre blends with gelling properties. Food Hydrocolloids, 23(3), 742–748. https://doi.org/10.1016/j.foodhyd.2008.04.005
- AOAC. (2005). Official methods of analysis (18th ed.). Association of Official Analytical Chemists.
- Assadeg, J., Al-Waeli, A. H., Fudholi, A., & Sopian, K. (2021). Energetic and exergetic analysis of a new double pass solar air collector with fins and phase change material. Solar Energy, 226, 260–271. https://doi.org/10.1016/j.solener.2021.08.056
- Babar, O. A., Tarafdar, A., Malakar, S., Arora, V. K., & Nema, P. K. (2020). Design and performance evaluation of a passive flat plate collector solar dryer for agricultural products. Journal of Food Process Engineering, 43(10), e13484. https://doi.org/10.1111/jfpe.13484
- Baspinar, B., & Güldaş, M. (2021). Traditional plain yogurt: A therapeutic food for metabolic syndrome? Critical Reviews in Food Science and Nutrition, 61(18), 3129–3143. https://doi.org/10.1080/10408398.2020.1799931
- Benhamza, A., Boubekri, A., Atia, A., El Ferouali, H., Hadibi, T., Arıcı, M., & Abdenouri, N. (2021). Multi-objective design optimization of solar air heater for food drying based on energy, exergy and improvement potential. Renewable Energy, 169, 1190–1209. https://doi.org/10.1016/j.renene.2021.01.086
- Brahma, B., Shukla, A. K., & Baruah, D. C. (2023). Design and performance analysis of solar air heater with phase change materials. Journal of Energy Storage, 61, 106809. https://doi.org/10.1016/j.est.2023.106809
- Bulut, M., Tunçtürk, Y., & Alwazeer, D. (2021). Effect of fortification of set‐type yoghurt with different plant extracts on its physicochemical, rheological, textural and sensory properties during storage. International Journal of Dairy Technology, 74(4), 723–736. https://doi.org/10.1111/1471-0307.12803
- Çakmak, G., & Yıldız, C. (2011). The drying kinetics of seeded grape in solar dryer with PCM-based solar integrated collector. Food and Bioproducts Processing, 89(2), 103–108. https://doi.org/10.1016/j.fbp.2010.04.001
- Farag, M. A., Ghazy, A. A., Kamel, S. M., Youssef, F. S., & Mabrok, H. B. (2022). Milk composition and starter cultures used for fermentation. In F. T. Tadros, N. W. Youssef, & M. A. Farag (Eds.), Fermented dairy products and their health benefits (pp. 1–37). Springer.
- Freitas, M. (2017). The benefits of yogurt, cultures, and fermentation. In M. Lyte (Ed.), The microbiota in gastrointestinal pathophysiology (pp. 209–223). Academic Press.
- Gao, J., Li, X., Zhang, G., Sadiq, F. A., Simal‐Gandara, J., Xiao, J., & Sang, Y. (2021). Probiotics in the dairy industry—Advances and opportunities. Comprehensive Reviews in Food Science and Food Safety, 20(4), 3937–3982. https://doi.org/10.1111/1541-4337.12755
- Goel, V., Hans, V. S., Singh, S., Kumar, R., Pathak, S. K., Singla, M., Bhattacharyya, S., Almatrafi, E., Gill, R. S., & Saini, R. P. (2021). A comprehensive study on the progressive development and applications of solar air heaters. Solar Energy, 229, 112–147. https://doi.org/10.1016/j.solener.2021.07.040
- Hadjimbei, E., Botsaris, G., & Chrysostomou, S. (2022). Beneficial effects of yoghurts and probiotic fermented milks and their functional food potential. Foods, 11(17), 2691. https://doi.org/10.3390/foods11172691
- Husnain, S. N., Amjad, W., Munir, A., & Hensel, O. (2022). Development and experimental study of smart solar assisted yogurt processing unit for decentralized dairy value Chain. Sustainability, 14(7), 4285. https://doi.org/10.3390/su14074285
- Itsaranuwat, P., Al‐Haddad, K. S., & Robinson, R. K. (2003). The potential therapeutic benefits of consuming ‘health‐promoting’fermented dairy products: A brief update. International Journal of Dairy Technology, 56(4), 203–210. https://doi.org/10.1046/j.1471-0307.2003.00106.x
- Javadi, F. S., Metselaar, H. S. C., & Ganesan, P. (2020). Performance improvement of solar thermal systems integrated with phase change materials (PCM), a review. Solar Energy, 206, 330–352. https://doi.org/10.1016/j.solener.2020.05.106
- Kim, S. Y., Hyeonbin, O., Lee, P., & Kim, Y. S. (2020). The quality characteristics, antioxidant activity, and sensory evaluation of reduced-fat yogurt and nonfat yogurt supplemented with basil seed gum as a fat substitute. Journal of Dairy Science, 103(2), 1324–1336. https://doi.org/10.3168/jds.2019-17117
- Kizildag, D., Castro, J., Kessentini, H., Schillaci, E., & Rigola, J. (2022). First test field performance of highly efficient flat plate solar collectors with transparent insulation and low-cost overheating protection. Solar Energy, 236, 239–248. https://doi.org/10.1016/j.solener.2022.02.007
- Kraan, M. J., Buskop, J., Doets, M., & Snippe, C. (2004). Structural analysis of the vacuum vessel for the lhcb vertex locator (velo). National Institute for Nuclear Physics and High Energy Physics, Kruislaan, 409(1098), 4–10.
- Kumar, K. R., Chaitanya, N. K., & Kumar, N. S. (2021). Solar thermal energy technologies and its applications for process heating and power generation–A review. Journal of Cleaner Production, 282, 125296. https://doi.org/10.1016/j.jclepro.2020.125296
- Kumar, C. M. S., Singh, S., Gupta, M. K., Nimdeo, Y. M., Raushan, R., Deorankar, A. V., Kumar, T. M. A., Rout, P. K., Chanotiya, C. S., Pakhale, V. D., & Nannaware, A. D. (2023). Solar energy: A promising renewable source for meeting energy demand in Indian agriculture applications. Sustainable Energy Technologies and Assessments, 55, 102905. https://doi.org/10.1016/j.seta.2022.102905
- Ladha-Sabur, A., Bakalis, S., Fryer, P. J., & Lopez-Quiroga, E. (2019). Mapping energy consumption in food manufacturing. Trends in Food Science & Technology, 86, 270–280. https://doi.org/10.1016/j.tifs.2019.02.034
- Lingayat, A., Chandramohan, V. P., & Raju, V. R. K. (2017). Design, development and performance of indirect type solar dryer for banana drying. Energy Procedia, 109, 409–416. https://doi.org/10.1016/j.egypro.2017.03.041
- Lopes, R. P., Mota, M. J., Sousa, S., Gomes, A. M., Delgadillo, I., & Saraiva, J. A. (2019). Combined effect of pressure and temperature for yogurt production. Food Research International, 122, 222–229. https://doi.org/10.1016/j.foodres.2019.04.010
- Lou, Y., Chen, L., Clausmeyer, T., Tekkaya, A. E., & Yoon, J. W. (2017). Modeling of ductile fracture from shear to balanced biaxial tension for sheet metals. International Journal of Solids and Structures, 112, 169–184. https://doi.org/10.1016/j.ijsolstr.2016.11.034
- Mutar, W. M., & Alaiwi, Y. (2023). Experimental investigation of thermal performance of single pass solar collector using high porosity metal foams. Case Studies in Thermal Engineering, 45, 102879. https://doi.org/10.1016/j.csite.2023.102879
- Muthukumaran, J., & Senthil, R. (2022). Experimental performance of a solar air heater using straight and spiral absorber tubes with thermal energy storage. Journal of Energy Storage, 45, 103796. https://doi.org/10.1016/j.est.2021.103796
- Nagappan, B., Devarajan, Y., Kariappan, E., Raja, T., & Thulasiram, R. (2023). A novel attempt to enhance the heat transfer rate of thermal energy storage using multitemperature phase change materials through experimental and numerical modelling. Applied Thermal Engineering, 227, 120457. https://doi.org/10.1016/j.applthermaleng.2023.120457
- Najgebauer-Lejko, D., Witek, M., Żmudziński, D., & Ptaszek, A. (2020). Changes in the viscosity, textural properties, and water status in yogurt gel upon supplementation with green and Pu-erh teas. Journal of Dairy Science, 103(12), 11039–11049. https://doi.org/10.3168/jds.2020-19032
- Nazir, H., Batool, M., Osorio, F. J. B., Isaza-Ruiz, M., Xu, X., Vignarooban, K., Phelan, P.I., & Kannan, A. M. (2019). Recent developments in phase change materials for energy storage applications: A review. International Journal of Heat and Mass Transfer, 129, 491–523. https://doi.org/10.1016/j.ijheatmasstransfer.2018.09.126
- Olivkar, P. R., Katekar, V. P., Deshmukh, S. S., & Palatkar, S. V. (2022). Effect of sensible heat storage materials on the thermal performance of solar air heaters: State-of-the-art review. Renewable and Sustainable Energy Reviews, 157, 112085. https://doi.org/10.1016/j.rser.2022.112085
- Osman, A. I., Chen, L., Yang, M., Msigwa, G., Farghali, M., Fawzy, S., Rooney, D. W., & Yap, P. S. (2023). Cost, environmental impact, and resilience of renewable energy under a changing climate: A review. Environmental Chemistry Letters, 21(2), 741–764. https://doi.org/10.1007/s10311-022-01532-8
- Palacio, M., Ramírez, C., Carmona, M., & Cortés, C. (2022). Effect of phase-change materials in the performance of a solar air heater. Solar Energy, 247, 385–396. https://doi.org/10.1016/j.solener.2022.10.046
- Pathak, S. K., Tyagi, V. V., Chopra, K., Kalidasan, B., Pandey, A. K., Goel, V., Saxena, A., & Ma, Z. (2023). Energy, exergy, economic and environmental analyses of solar air heating systems with and without thermal energy storage for sustainable development: A systematic review. Journal of Energy Storage, 59, 106521. https://doi.org/10.1016/j.est.2022.106521
- Qasem, N. A., Arnous, M. N., & Zubair, S. M. (2020). A comprehensive thermal-hydraulic assessment of solar flat-plate air heaters. Energy Conversion and Management, 215, 112922. https://doi.org/10.1016/j.enconman.2020.112922
- Rahman, M. T., Zubair, M. A., Shima, K., & Chakma, M. P. (2020). Development of quality parameters for yogurt with strawberry juice. Food and Nutrition Sciences, 11(12), 1070. https://doi.org/10.4236/fns.2020.1112075
- Rastogi, Y. R., Thakur, R., Thakur, P., Mittal, A., Chakrabarti, S., Siwal, S. S., Thakur, V. K., Saini, R. V., & Saini, A. K. (2022). Food fermentation–Significance to public health and sustainability challenges of modern diet and food systems. International Journal of Food Microbiology, 371, 109666. https://doi.org/10.1016/j.ijfoodmicro.2022.109666
- Routray, W., & Mishra, H. N. (2011). Scientific and technical aspects of yogurt aroma and taste: A review. Comprehensive Reviews in Food Science and Food Safety, 10(4), 208–220. https://doi.org/10.1111/j.1541-4337.2011.00151.x
- Saleem, G. N., Gu, R., Qu, H., Bahar Khaskheli, G., Rashid Rajput, I., Qasim, M., & Chen, X. (2024). Therapeutic potential of popular fermented dairy products and its benefits on human health. Frontiers in Nutrition, 11, 1328620. https://doi.org/10.3389/fnut.2024.1328620
- Sapto, A. (2010). Frying vacuum machine design and vacuum tube thermal analysis using catia P3 V5r14. Universitas Gunadarma Jakarta.
- Shaikh, A. S. A., & Nitnaware, P. T. (2013). Finite element analysis & thickness optimization of vacuum chamber for electron microscopy applications. International Journal of Modern Engineering Research (IJMER), 3(3), 1666–1671.
- Sharma, A., Chauhan, R., Kallioğlu, M. A., Chinnasamy, V., & Singh, T. (2021). A review of phase change materials (PCMs) for thermal storage in solar air heating systems. Materials Today: Proceedings (Vol. 44, pp. 4357–4363). https://doi.org/10.1016/j.matpr.2020.10.560
- Soni, R., Batish, V. K., Grover, S., & Mohanty, A. K. (2020). Milk composition and starter cultures used for fermentation. In A. H. Eissa, & R. A. Awad (Eds.), Fermented milk and dairy products (pp. 1–28). Academic Press.
- Syahariza, Z. A., & Yong, H. Y. (2017). Evaluation of rheological and textural properties of texture-modified rice porridge using tapioca and sago starch as thickener. Journal of Food Measurement and Characterization, 11(4), 1586–1591. https://doi.org/10.1007/s11694-017-9538-x
- Zhao, J., Bhandari, B., Gaiani, C., & Prakash, S. (2021). Physicochemical and microstructural properties of fermentation-induced almond emulsion-filled gels with varying concentrations of protein, fat and sugar contents. Current Research in Food Science, 4, 577–587. https://doi.org/10.1016/j.crfs.2021.08.007