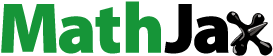
ABSTRACT
The individual and combined effects of electron beam (EB) irradiation and thermal treatments were investigated on Chinese braised beef samples inoculated with Bacillus cereus spores. Log reductions of 1.19, 2.10, and 3.62 log CFU/g were recorded for B. cereus spores after 6, 9, and 12 kGy lone EB doses, respectively. An 85°C thermal treatment at 20 min (H) reduced the spores population by 1.03 log CFU/g. The combination of the thermal and EB irradiation treatments (HEB) of HEB-6 and HEB-9 achieved 1.69 and 2.89 log CFU/g reductions, respectively. The B. cereus spores population was depleted to below the detection limit following HEB-12 treatment. No significant pH change was observed in the treatments except for HEB-12. The hurdle treatment of HEB-12 caused marked changes in color, aroma, and overall acceptability. Although HEB irradiation improved microbial safety, careful selection of approved EB doses can effectively enhance food safety and maintain reasonable quality standards.
1. Introduction
The demand for ready-to-eat (RTE) food products has risen in the last decade due to a preference for fast, convenient, and easy-to-prepare meals. This is due to the increasing number of women busy with white color jobs, lack of time and cooking skills, and an increased number of small and single-households (Kanzler et al., Citation2015; Oh et al., Citation2017). Chinese braised beef, potatoes, and rice are important RTE cuisines popularly consumed in China and are highly nutritious and accessible among the Chinese people.
Foodborne pathogens can grow on meat and meat products because meat is an excellent growing medium for pathogens, resulting in foodborne diseases (Dave & Ghaly, Citation2011). From 1998 to 2008, contaminated meat and meat products such as poultry, beef, pork, and games accounted for 22.2% of foodborne illnesses and 28.8% of deaths in the United States (Painter et al., Citation2013). From 2007 to 2014, the European Union Food Safety Authority (EFSA) reported B. cereus as the causative pathogen of foodborne outbreaks in the European Union (EU). A total of 6,657 human cases and 352 hospitalizations with no death cases were recorded (EFSA BIOHAZ Panel, Citation2016). Bacillus cereus is a spore-forming pathogen reported in pulses, soils, raw and cooked meats, milk, rice, egg, and vegetables (Guérin et al., Citation2017; Lv et al., Citation2019; Muhammad, Lv, et al., Citation2019). Spore formation by vegetative cells of B. cereus is a response strategy to unfavorable external stresses. These spores can stay for many years in dormant form until a suitable condition for germination arises (Soni et al., Citation2016; Wells-Bennik et al., Citation2016).
To prevent meat-related outbreaks from pathogens and minimize contamination, meat must be efficiently decontaminated with minimum sensory quality changes. One way to achieve this is by utilizing ionizing radiation such as photon-based X-rays, gamma rays, and electron beam radiations. Electron beam irradiation (EB) is used to decontaminate poultry meat, meat, and meat products due to its established microbial inactivation (Ahn et al., Citation2013). A minimum dose of 1–10 kGy for food irradiation was recommended by the International Atomic Energy Authority (IAEA) based on microorganism reduction level and shelf life extension of different food products, including strawberries, refrigerated meats, and fish, and ready-to-eat meals (IAEA, Citation2015). Likewise, the United States Food and Drug Administration (FDA) recommended food products prepared explicitly for the space program, hospitals, emergency rations, and food for astronauts be irradiated with doses above 10 kGy to reduce the microorganism to the point of sterility (FDA, Citation2019; IAEA, Citation2015). Ionizing radiations such as EB is an emerging non-thermal technique employed in the food sector for microbial decontamination. The technology is chemical-free and noninvasive, and it utilizes a stream of high-energy electrons generated from electricity by an electron accelerator to inactivate microorganisms. The ionized electrons contribute to two effects. One is direct damage to the target molecules by the energy transfer of electrons. The other one is an indirect effect that occurs due to the interaction of the energized electrons and water molecules in the biomaterial, which results in the generation of free radicals, such as hydroxyl radicals as a powerful oxidizing agent (Lung et al., Citation2015; Tahergorabi et al., Citation2012; Zhang et al., Citation2018). These free radicals are linked to damage of cellular components, including DNA, fatty acids, pigments, and membrane lipids (Hertwig et al., Citation2018; Zhang et al., Citation2018).
In the last decade, numerous researchers have investigated EB irradiation on various food products. Decontamination of E. coli in ground beef, boneless skinless chicken breast, and trout fillets was reported at different temperatures after exposure to different EB doses. The D-values (dose required to achieve 1 log or 90% reduction of the microbial population) obtained ranged from 0.22 to 0.35 kGy in the treated samples at 4 °C and −20 °C, respectively (Black & Jaczynski, Citation2006). Beef samples exposed to 5 kGy EB irradiation resulted in a decrease in the population of Listeria monocytogenes and E. coli from an initial count of 8.83 and 8.05 log CFU/g, respectively, to below the detection limit of 1 log CFU/g (Yim et al., Citation2016). Similarly, pork jerky inoculated with L. monocytogenes and E. coli at initial concentrations of 8.02 and 7.88 log CFU/g, respectively, were reduced to undetectable level following EB irradiation at 2 kGy (Kim et al., Citation2014). Lee et al. (Citation2018) studied the influence of EB irradiation on dried laver products. They recorded log reductions of 2.5 and 2.0 log CFU/g for coliform and total aerobic bacteria after 4 and 7 kGy irradiation doses, respectively. Meanwhile, the D-values for E. coli K12 on clover, mung bean, and fenugreek were 1.21, 1.11, and 1.40 kGy, respectively, after 4–12 kGy irradiation doses. The researchers further affirmed that EB irradiation at 12 kGy dose did not significantly influence the seeds’ germination and growth (Fan et al., Citation2017). EB irradiation has a low penetration ability that depends on the electron accelerator and the areal density of the target food product. EB can be used to treat a thin layer of food material with uniform dose distribution.
The occurrence of B. cereus in meat, meat products, and ready-to-eat food products during and after processing has encouraged cross-contamination and, thus, necessitated the exploration of novel decontamination strategies with less quality deterioration (Milojevic et al., Citation2019; Rosenquist et al., Citation2005). Moreover, the application of high temperatures to meat and meat products has caused detrimental effects on the quality attributes, including the formation of carcinogenic substances such as polycyclic aromatic hydrocarbons and heterocyclic amines (John et al., Citation2011). To the best of our knowledge, no study has explored the sequential application of mild heat and EB irradiation for the inactivation of B. cereus in braised meat. Therefore, this study employed a low-temperature thermal treatment and EB irradiation as a combined strategy for the inactivation of B. cereus spores inoculated in Chinese braised meat cuisine, and analyzed the changes in quality attributes of the product. The reason for the hurdle approach was EB radiation limits of 10 kGy recommended by FAO/WHO are not sufficient to reduce spore population by more than 3–4 log cycles, hence not adequate to ensure food sterilization and safety (De Lara et al., Citation2002). Nowadays, non-thermal technologies and thermal treatments are employed as hurdle techniques to improve inactivation efficacy in food pathogens control (Muhammad, Chen, et al., Citation2019; Singh & Shalini, Citation2016). Low-temperature thermal treatments (40–90
) are selected because they produce meat with improved tenderness and better appearance than when cooked at higher temperatures (Dominguez-Hernandez et al., Citation2018).
2. Material and methods
2.1. Chinese braised beef preparation
Chinese braised beef (thigh part) sample was purchased from Sanquan Food Co. Ltd. (Zhengzhou, China). The Chinese braised beef cuisine is comprised of beef and potato slices immersed in soup at a ratio of 1:1:1 and has a pH of 6. Both the beef and potato slices were approximately 5 5
5 mm in size. The nutritional profile of the sample is 5.5 g/100 g protein, 7.5 g/100 g fat, 8.5 g/100 g carbohydrate, 593 mg/100 g sodium, and 516 kJ energy. This cuisine is often served with cooked rice as a ready-to-eat meal.
2.2. Preparation of Bacillus cereus spores
Spore preparation followed the method described by Jiao et al. (Citation2019) with some modifications. Bacillus cereus ATCC 14,579, purchased from Hope Bio-Technology Co., Ltd., Qingdao, Shandong, China, was reactivated in nutrient broth (NB, Hope Bio-Technology Co., Ltd., Qingdao, Shandong, China) at 37°C under continuous shaking at 150 rpm overnight. The overnight culture was plated on a nutrient medium (Hope Bio-Technology Co., Ltd., Qingdao, Shandong, China) supplemented with MnSO4 (50 mg/L, Sigma) and incubated at 37°C for 10–12 days. The spores were observed for 95% sporulation using a microscope (UPH 203i Phase Microscope, Aopu Photoelectric Technology Co., Ltd., Chongqing, China) and collected by scraping with sterile slides and suspended in sterile deionized water. The remaining vegetative cells were inactivated by heating at 60°C for 10 min (Evelyn & Silva, Citation2015). The spore suspension was washed three times by centrifugation (TGL-20 M centrifuge; Kaida Scientific Instruments Co., Ltd., Changsha, Hunan, China) and resuspended in sterile water. The initial spore’s population was about 108 CFU/mL, as determined by plate count assay.
2.3. Sample inoculation and packaging
About 400 g of the Chinese braised beef cuisine was aseptically inoculated with 4 mL of the already prepared B. cereus spores suspension in section 2.2 to give a final concentration of 6.74 log CFU/g as confirmed by plating on tryptic soy agar (TSA, Hope Bio-Technology Co., Ltd., Qingdao, Shandong, China) supplemented with 0.1% soluble starch. The TSA plates were inverted and incubated at 37°C for 12–24 h. About 50 g of the inoculated sample was packaged aseptically in low-density polyethylene (LDPE) bags. The packages were sealed with a sealing machine to give an approximate dimension of 130 mm × 100 mm × 10 mm in length, width, and thickness, respectively. The sealed inoculated samples are ready for heat and irradiation treatments. The samples for quality analysis were not inoculated with the spores.
2.4. Electron beam irradiation and heat treatment of samples
For each single EB irradiation, packaged samples were arranged on a conveyor placed 0.84 m away from the radiation source and exposed to DZ-10/20 EB irradiation equipment (China Institute of Atomic Energy, China). The equipment was operated at an energy level of 10 MeV at room temperature (24 oC). displays the EB irradiation facility used for the study. The Chinese braised beef samples were irradiated at doses of 6, 9, and 12 kGy, and these are denoted as EB-6, EB-9, and EB-12, respectively. The heat treatment was carried out according to Ren et al. (Citation2018) with some modifications. A thermostat-controlled water bath (SHJ-6AB, Changzhou Jintan Instrument Co. Ltd., China) already set at 85
oC for 20 min was used. This temperature was selected based on the report of Zhang et al. (Citation2012) and the series of trials conducted. The packaged samples were immersed in hot water already at 85
oC and allowed to stay for 20 min. After that, samples were immediately cooled in ice water to quench the heating effect. This process was represented as H. Some of the thermally treated samples were further subjected to subsequent EB treatment at doses 6, 9, and 12 kGy and were denoted as HEB-6, HEB-9, and HEB-12, respectively. The untreated sample (EB-0) was not submitted to any EB irradiation or heat treatments.
2.5. Dosimetry
The dosimetry used was B3000 WINdose Dosimeter films (Gex Corporation, U.S.A.). For each sample treatment, one dosimeter film was carefully placed on the surface of the sample package using masking tape. The samples were then submitted to the EB irradiation at the preset doses. The films were removed from the sample surfaces and incubated at 58.5°C for 15 min to stabilize the dosimeter response. Each film was placed into the dosimeter holder and inserted into the spectrophotometer (GENESYS TM 30, ThermoFisher Scientific, U.S.A.) compartment for measurement. Before the dosimeter measure, a calibration was carried out to ensure the reported doses from the EB irradiation have measurement traceability of the national standards (Zhang et al., Citation2018). The overall calibration uncertainty was at k = 2 or 95% confidence based on the so-called GEX “top-down method. The absorbance was measured at 550 nm wavelength.
2.6. Spores enumeration
For the enumeration of the survival spores, the LDPE bags were pommeled with an Interlab stomacher blender (iMix, Interlab, Mourjou, France) for 2 min at 230 rpm. The homogenates ( 2
0.2 g) were added to a test tube containing 2 mL of 0.85% sterile saline solution. Serial dilution was performed, and appropriate dilutions were plated on tryptic soy agar (TSA, Hope Bio-Technology Co., Ltd., Qingdao, Shandong, China) supplemented with 0.1% soluble starch. These dilutions were included in the calculation of spore survival. The TSA plates were inverted for incubation at 37°C for 12–24 h (Lv et al., Citation2019).
2.7. Determination of quality characteristics
2.7.1. pH measurement
The pH was determined according to Kim et al. (Citation2015), with some modifications. About 2 g of each sample was added to 18 mL of water. The mixture was homogenized at 12,000 rpm for 2 min using a homogenizer (Yang Digital High-speed homogenizer, China), and the pH was measured with a pH meter (Mettler Toledo, Hangzhou Lohand Biological Science and Technology Co.).
2.7.2. Color analysis
The instrumental color of the samples was determined using a Konica Minolta CM-600d spectrophotometer (Konica Minolta Holdings, Inc., Tokyo, Japan). Samples were pommeled with an Interlab stomacher blender (iMix, Interlab, Mourjou, France) for 2 min at 230 rpm to obtain homogenized samples. About 20 g of each treated sample was placed into the glass cuvette of the equipment and analyzed based on the color coordinate system (L*, a*, and b*) as reported elsewhere (Muhammad, Chen, et al., Citation2019). Before data collection, the equipment was calibrated with standard white and black tiles. The color indices recorded were L* which represented whiteness/darkness, a* showed redness/greenness, b* which referred to yellowness/blueness, C depicts Chroma and describes the dullness or vividness of the color in the samples, which refers to how close the color is to either gray or pure hue. C is calculated using EquationEquation 1.(1)
(1) The total color difference (
) is computed according to EquationEquation 2.
(2)
(2)
2.7.3. Measurement of lipid oxidation
Lipid oxidation was assayed following the method described by Muhammad et al. (Citation2018) with modifications. About 5 g of pommeled meat sample was added to 17 mL 7.5% trichloroacetic acid (TCA) solution in water and 5 mL of 0.02 M thiobarbituric acid (TBA) and centrifuged for 15 min at 6000 rpm. Two milliliters of the supernatant was transferred to a test tube containing 3 mL of water. The test tube was vortexed and incubated at 90°C in a water bath (SHJ-6AB, Changzhou Jintan Instrument Co. Ltd., China) for 40 min to develop color. Samples were immediately cooled on ice water to room temperature, and the absorbance of each sample was measured at 532 nm against a blank using a microtiter plate reader (Multiskan Go, Thermo Scientific). A standard curve of tetraethoxypropane (TEP) was prepared as the malondialdehyde (MDA) standard. The TBA values were expressed as milligram MDA per kilogram of samples.
2.7.4. Sensory evaluation
A 9-point hedonic test was performed to analyze the treated samples, as described in Liu et al. (Citation2019). The samples were evaluated for color, appearance, and aroma by a panel of 10 highly trained individuals (four males and six females between 26 and 38 years old) from the Department of Food Science and Nutrition, Zhejiang University Hangzhou, China. Panelists were placed in separate booths with illumination, and each booth contained samples randomly coded in falcon paper plates. Sensory evaluation was conducted at 24 ± 2°C, and the mean scores of all sensorial attributes were used to determine the overall acceptability of the treated samples.
2.8. Statistical analysis
All statistical analyses were performed using SPSS version 25 (IBM Incorporation, U.S.A.). The results were presented as mean and standard deviation (SD) of three independent measurements unless stated otherwise. Color values were analyzed using multivariate analysis of variance (MANOVA), while the rest of the data were analyzed using ANOVA. The marginal means were compared using the Duncan multiple range test at p < .05.
3. Results and discussion
3.1. Spore inactivation by individual and sequential treatments
The survival of B. cereus spores in Chinese braised beef samples after EB irradiation, thermal treatment, and their combinations are presented in . The initial spore population in the untreated sample was 6.470.02 log CFU/g. Following EB irradiation at 6, 9, and 12 kGy, the spore population was significantly reduced by 1.19, 2.01, and 3.62 log CFU/g, respectively. The log reductions in the lone EB irradiated samples differ significantly when compared to the untreated sample. The thermal treatment at 85°C for 20 min resulted in 1.03 log CFU/g reduction, which differs significantly from all the treated samples except for log reduction recorded with EB-6 samples. In addition, it can be observed that the EB irradiation of 9 and 12 kGy doses have significant inactivation efficacy compared to the single thermal treatment. This could be attributed to the antimicrobial properties of hydroxyl radicals produced by EB irradiation. The combined treatments of thermal and EB irradiation demonstrated greater antimicrobial efficacy than the lone treatments. Chinese braised beef samples subjected to thermal treatment at 85°C for 20 min, followed by EB irradiation doses of 6 and 9 kGy resulted in 1.69 and 2.89 log CFU/g reductions, respectively. These reductions differ significantly when compared with other treated and untreated samples. In contrast, the sequential application of the thermal treatment and 12 kGy EB irradiation treatments led to the spores’ inactivation to an undetectable limit (detection limit is 1 log CFU/g). These findings indicated that the thermal treatment significantly improved the inactivation efficacy of the EB irradiation. Numerous studies have reported that single EB irradiation can effectively inactivate different microorganisms on various food products. These include the reduction of E. coli population on beef carcasses (Arthur et al., Citation2005), mung bean, clover, fenugreek (Fan et al., Citation2017), ground beef, boneless chicken breast, and trout fillets (Black & Jaczynski, Citation2006). A reduction of 6–7 log CFU/g of L. monocytogenes and E. coli was reported in beef loin after an EB irradiation dose of 4 kGy. A further increase in EB dose to 5 kGy reduced the bacterial counts to undetectable limits (Yim et al., Citation2016). Likewise, B. subtilis, B. megaterium, and B. pumilus spores were completely inactivated after an EB irradiation dose of 30 kGy (Pillai et al., Citation2006).
Figure 2. Survival of B. cereus spores in braised meat as affected by EB, thermal, and combinations of thermal and EB treatments. Different letters on error bars indicate significant difference (p < .05). The surviving population of B. cereus spores from the untreated sample (EB-0) was 6.74 ± 0.02 log CFU/g. Error bars represent standard deviation of means from three replicates. ND refers to population below the detection limit (<1 log CFU/g).
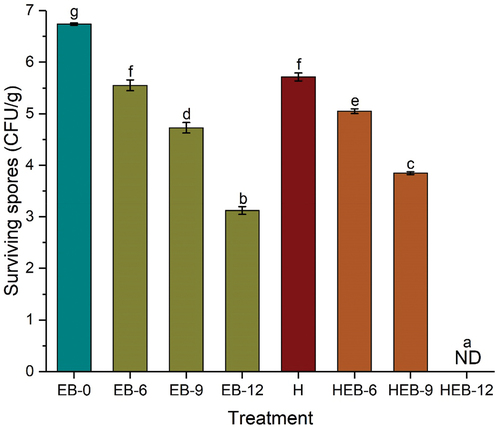
Sporulation conditions, bacterial species, and EB doses influence the inactivation of spores (Y. Zhang et al., Citation2018). As such, this could be the reason for the significant inactivation recorded in our study with higher EB doses. Besides, Ahn et al. (Citation2013) and Muhammad, Chen, et al. (Citation2019) have reported that combining two or more physical and chemical interventions enhanced the inactivation efficacy of hurdle processing strategies. In our results, the EB irradiation and thermal treatment hurdle have significantly improved the inactivation by more than two-fold for HEB-12 treatment as against the lone treatments. The oxidizing effect of hydroxyl radicals coupled with the thermal effect resulted in significant log reductions of the B. cereus spores populations. Y. Zhang et al. (Citation2018) pointed out that DNA is one of the major targets of EB irradiation during the inactivation of spores. In addition, structural damage of the inner membrane and coat, and fragmentation of the DNA, were also reported by Fiester et al. (Citation2012). Liao et al. (Citation2018) and Hertwig et al. (Citation2018) have demonstrated the effects of energized electrons and hydroxyl radicals in the degradation of cellular components such as DNA and oxidation of lipids and proteins. The breakage of the DNA strand extends to the disruption of cell multiplication. Also, Lv et al. (Citation2019) established that thermal treatment decreased the electron density in the nucleoid of B. cereus spores. Therefore, among the single and combined treatments in our study, it can be concluded that HEB-12 has the most antimicrobial efficacy compared to HEB-6 and HEB-9. Shelf life study is paramount in a microbial study of spores and forms part of our future research to reveal whether the injured cells can recover after irradiation and storage.
3.2. pH
pH is an important parameter that is considered when determining the quality of cooked meat and meat products. This quality parameter reduced the intense redness of raw meat due to myoglobin denaturation, which directly impacts the redness (a* value) of meat (Moon et al., Citation2016). The changes in pH of the Chinese braised beef samples are shown in . A slight decrease in pH value was noticed in the lone EB irradiation group compared to the untreated sample (6.02). Similar changes in pH values were recorded in the combined irradiated samples (HEB-6 and HEB-9). Meanwhile, the HEB-12 sample exhibited a significant pH reduction compared to the untreated sample. This significant pH reduction in HEB-12 could be due to the high EB dose of 12 kGy. The changes in pH might be attributed to hydroxyl radicals’ oxidation effect from the radiolysis of water molecules, which produced hydronium ions responsible for the acidity. The pH of the lone thermal treated sample was slightly reduced as compared to the untreated. It has been reported that thermal treatment at 85°C did not significantly alter the pH values (Gironés-Vilaplana et al., Citation2016). A drop in pH value from 10.0 to 7.59 was reported after the EB irradiation dose of 100 kGy (Selambakkannu et al., Citation2011). Ocloo et al. (Citation2014) reported a significant pH decrease after an irradiation dose of 10 kGy.
3.3. Instrumental color
Meat color is a critical quality attribute considered during quality and consumer acceptance evaluation. EB irradiation and thermal treatments influenced the color of meat and meat products, depending on the applied dose and temperature (Ahn et al., Citation2013; Moon et al., Citation2016). The color changes of the EB irradiation, thermal, and their combinations are presented in . The L* values, which denote lightness, increased significantly after Chinese braised beef samples were submitted to lone EB irradiation of 9 and 12 k Gy doses compared with the untreated sample. Besides, a significant decline in L* values was observed in the thermal-treated sample. In the lone treatments of EB-9 and EB-12 and the combined treatments of HEB-6 and HEB-9, a non-significant change was observed among these treatments, but when compared with the untreated sample, the L* values increased significantly. Likewise, HEB-12 showed a significant increase as against all other treatment groups. Meanwhile, the a* values (redness) of the lone irradiated samples significantly differ from the untreated sample. The a* values for the lone thermal treatment and all the combined treatment groups significantly decline when compared with the untreated sample. However, slight differences were observed in the a* values of combined thermal and EB irradiated samples. The thermal-treated sample differs significantly from all other treatment groups, including the untreated sample. The b* values (yellowness) of all the treatment groups do not differ significantly except for untreated and EB-12 samples. The EB-12 irradiated sample significantly decreased compared to the untreated sample. The redness in cooked beef is affected by pH and myoglobin denaturation during cooking. The pH recorded in our study ranged from 6.02 to 5.72. At this pH level, the myoglobin could not withstand the thermal treatment, and thus, a decreased redness was evident in lone thermal and combined treatment groups. It has been documented that cooked beef at high temperatures has a mild red color at pH ranges of 5.3 to 5.7 (Moon et al., Citation2016). The chroma values for EB-6, EB-9, H, HEB-9, and HEB-12 do not differ significantly but showed a significant decrease when compared with the untreated sample. A similar decline was also observed in EB-12. Meanwhile, HEB-6 treated samples showed a significant increase in chroma value when compared to the treated and untreated samples. The color changes in all the treated Chinese braised beef samples did differ significantly except for HEB-12, which showed a significant increase when compared to all the treated and untreated samples. The color changes in EB-6, H, and HEB-6 samples were statistically similar. Also, slight changes of non-significance importance were noticed among EB-9, EB-12, and HEB-9 samples. An intense color variation was observed in the HEB-12 treated sample. Loss of redness and undesired brownish or greenish color in meat patties have been associated with myoglobin, which is oxidized to metmyoglobin or sulfmyoglobin during irradiation or thermal processing (Giroux et al., Citation2001). In our study, the significant loss of redness and color changes observed in our experimental samples might be due to the cooking process. This was confirmed in the sensory analysis discussed in the sensory analysis section. Reduction in L* and a* values of meatballs was reported after exposure to irradiation doses of 1.5 to 3 kGy (Gunes et al., Citation2011). Giroux et al. (Citation2001) reported some detrimental effects on L*, a*, and b* values after irradiation of meat samples.
Table 1. Color changes of braised meat samples after treatments.
3.4. Lipid oxidation
Lipid oxidation is a secondary oxidation product that results in undesired odor in food products due to hydroxyl radicals’ oxidizing effect. Meat contains more than 75% water, and hydroxyl radicals have been established as lipid oxidation initiators in EB-irradiated meat and meat products (Ahn et al., Citation2013). Depending on the food product, the off-odor could be in the form of a fishy, rancid, or oxidized odor that could lead to low sensory scores (Kochhar, Citation1996). The 2-thiobarbituric acid reactive substance (TBARS) is usually used to quantify the level of lipid oxidation in food products. As can be observed from , the TBARS for the untreated Chinese braised beef sample was 1.10 mg MDA/kg. Following EB irradiation treatments at 6, 9, and 12 kGy, the TBARS increased significantly compared to the untreated sample. A similar increase was observed with the thermally treated sample. The combined treatment group had an increase of about 1.15 mg MDA/kg against the untreated. No significant differences were observed among the lone EB treatment and among the combined groups except for EB-6 treated samples. Heightened lipid oxidation is expected in EB-irradiated foods because of the high-energy electrons produced by the ionizing radiation, which reacts with the water molecules to produce hydroxyl radicals (radiolytic products) that can induce lipid oxidation. The observed changes in lipid oxidation can be reduced by minimizing exposure of cooked meat to oxygen during irradiation due to heightened hydroxyl radicals formation via the Fenton reaction. The presence of oxygen has been reported to significantly affect lipid oxidation and odor production in meat (Ahn et al., Citation2013). Previous studies have reported the accumulation of TBARS in EB-irradiated poultry meat after exposure to 1.0 and 1.8 kGy doses (Cuppett et al., Citation2000). A significant increase in TBARS was observed in beef loin after 2 kGy EB irradiation doses (Yim et al., Citation2016). Ahn et al. (Citation2000) reported that the TBARS level could be reduced in irradiated cooked meat by minimizing meat exposure to oxygen. Given the reports mentioned earlier of heightened lipid peroxidation, extensive studies need to be conducted, especially in meat and meat products vacuum packaged, to ascertain the extent of the oxidation, such as detecting triacylglycerols, an intermediate product of lipid peroxidation. Analytical tools, including gas chromatography-mass spectrometry (GC-MS) and liquid chromatography-mass spectrometry (LC-MS), could be used to analyze 2-alkylcyclobutanones generated from irradiated fatty acids (Lung et al., Citation2015).
3.5. Sensory analysis
The sensory attributes of lone EB irradiation, thermal, and combined thermal and EB irradiated Chinese braised beef samples are presented in . The samples were analyzed for color, appearance, aroma, and overall acceptability. The sensory analysis revealed slight changes in the color attribute for samples treated with lone thermal and 6 kGy EB irradiation. Meanwhile, samples exposed to 9 and 12 kGy doses resulted in a significant change in color attribute compared to the untreated. Similarly, slight changes at lone treatments (thermal, 6, and 9 kGy) were evident in sensory appearance traits. However, the lone EB-12 and combined treatments of HEB-9 and HEB-12 resulted in a significant change in appearance. The aroma of the Chinese braised beef samples treated with lone thermal, EB-6, and EB-9 and combined HEB-6 and HEB-9 did not differ significantly from the untreated. The aroma of the HEB-12 recorded the lowest sensory score and differed significantly from the other treated or untreated samples. Off-aroma generated in EB irradiated and thermal-treated meat samples were correlated with sulfur compounds. Intense off-odor has been reported in samples irradiated with a higher EB dose of 10 kGy (Ahn et al., Citation2000). In our result, the overall acceptability of thermal, EB-6, EB-9, HEB-6, and HEB-9 treated samples did not differ significantly from untreated samples, whereas the overall acceptability of samples treated with EB dose of EB-12, HEB-9, and HEB-12 significantly deteriorated.
Figure 5. Sensory scores of braised meat as affected by lone EB irradiations, thermal, and combined thermal and EB irradiations.
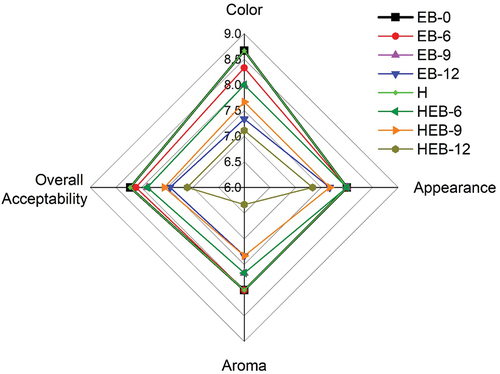
The thermal-treated Chinese braised beef sample had the highest sensory score in terms of color, appearance, aroma, and overall acceptability among the treatment groups and was comparable to the untreated sample. At the same time, the HEB-12 sample exhibited the lowest sensory score and received the maximum treatments. Overall, EB irradiation could negatively affect irradiated Chinese braised beef samples’ sensory characteristics, especially at a high dose of 12 kGy. Numerous sensory results have linked EB irradiation to the off-odor formation. Further studies should explore the mechanism of this off-odor formation in irradiated food products during storage. Giroux et al. (Citation2001) observed no detrimental effect on irradiated beef patties for irradiation doses of less than 4 kGy. The overall acceptability of poultry meat deteriorated following EB irradiation at 1.8 kGy and increased days of storage of 28 days (Cuppett et al., Citation2000).
4. Conclusion
This study applied a combined low-temperature thermal treatment and EB irradiation for the inactivation of B. cereus spores inoculated in Chinese braised meat cuisine and analyzed the ensuing quality changes from the product. The application of combined thermal and EB irradiation treatments demonstrated higher sporicidal efficacy than the lone thermal and EB irradiation treatments. B. cereus spores were inactivated below the detection limit in the Chinese braised beef sample after a hurdle of thermal (85°C at 20 min) and 12 kGy EB irradiation (HEB-12). This hurdle intervention achieved more than the 5-slog reduction benchmark set by United States Food and Drug Administration (FDA) for the inactivation of food pathogens by novel emerging technologies. However, in the treatment condition of HEB-12, significant changes in pH, total color changes, lipid oxidation, and some sensory attributes were noticed. The lone thermal, EB irradiation, and HEB-6 treatments did not cause severe changes in pH, some color parameters, and sensory qualities. The results of this study demonstrated that the hurdle approach could be a new strategy for improving the microbial safety of cooked meat and meat products under a careful selection of treatment conditions. This could be a strategy to improve the safety and shelf life of ready-to-eat meals, hospital meals, military rations, and food for astronauts. The heightened lipid oxidation recorded in EB irradiated-package foods could be minimized via vacuum packaging. Such a strategy should be utilized to package food products intended for EB irradiation treatment. It is strongly suggested that the maximum absorbed dose applied to food products should be less than that, which could adversely affect the physical, nutritional, or sensory attributes. A more in-depth study is needed to unravel the impact of EB irradiation of meat products under different storage and packaging conditions. Furthermore, analytical tools such as GC-MS and LC-MS should be employed to analyze the secondary metabolites generated during lipid oxidation of irradiated meat products. Optimization of the process conditions within the regulatory limit is recommended to achieve efficient microbial decontamination while ensuring minimum quality changes.
Acknowledgment
This study is financially supported by the Natural Science Foundation of Zhejiang Province, China (LR21C200002). The authors are very grateful to Dr Liangyan Wang for assisting in equipment operation.
Disclosure statement
No potential conflict of interest was reported by the author(s).
Additional information
Funding
References
- Ahn, D. U., Jo, C., & Olson, D. G. (2000). Analysis of volatile components and the sensory characteristics of irradiated raw pork. Meat Science, 54(3), 209–10. https://doi.org/10.1016/S0309-1740(99)00081-9
- Ahn, D. U., Jo, C., Olson, D. G., & Nam, K. C. (2000). Quality characteristics of pork patties irradiated and stored in different packaging and storage conditions. Meat Science, 56(2), 203–209. https://doi.org/10.1016/S0309-1740(00)00044-9
- Ahn, D. U., Kim, I. S., & Lee, E. J. (2013). Irradiation and additive combinations on the pathogen reduction and quality of poultry meat. Poultry Science, 92(2), 534–545. https://doi.org/10.3382/ps.2012-02722
- Arthur, T. M., Wheeler, T. L., Shackelford, S. D., Bosilevac, J. M., Nou, X., & Koohmaraie, M. (2005). Effects of low-dose, low-penetration electron beam irradiation of chilled beef carcass surface cuts on Escherichia coli O157: H7 and meat quality. Journal of Food Protection, 68(4), 666–672. https://doi.org/10.4315/0362-028X-68.4.666
- Black, J. L., & Jaczynski, J. (2006). Temperature effect on inactivation kinetics of Escherichia coli O157: H7 by electron beam in ground beef, chicken breast meat, and trout fillets. Journal of Food Science, 71(6), 221–227. https://doi.org/10.1111/j.1750-3841.2006.00105.x
- Cuppett, S. L., Mckee, S. R., Lewis, S. J., & Vela, A. (2000). Effect of electron beam irradiation on poultry meat safety and quality. Poultry Science, 81(6), 896–903. https://doi.org/10.1093/ps/81.6.896
- Dave, D., & Ghaly, A. E. (2011). Meat spoilage mechanisms and preservation techniques: A critical review. American Journal of Agricultural and Biological Science, 6(4), 486–510. https://doi.org/10.3844/ajabssp.2011.486.510
- De Lara, J., Fernández, P. S., Periago, P. M., & Palop, A. (2002). Irradiation of spores of Bacillus cereus and Bacillus subtilis with electron beams. Innovative Food Science and Emerging Technologies, 3(4), 379–384. https://doi.org/10.1016/S1466-8564(02)00053-X
- Dominguez-Hernandez, E., Salaseviciene, A., & Ertbjerg, P. (2018). Low-temperature long-time cooking of meat: Eating quality and underlying mechanisms. Meat Science, 143, 104–113. https://doi.org/10.1016/j.meatsci.2018.04.032
- EFSA BIOHAZ Panel. (2016). Scientific opinion on the risks for public health related to the presence of Bacillus cereus and other Bacillus spp. including Bacillus thuringiensis in foodstuffs. The EFSA Journal, 14(7), 93. 4524. https://doi.org/10.2903/j.efsa.2016.4524
- Evelyn, E., & Silva, F. V. M. (2015). Thermosonication versus thermal processing of skim milk and beef slurry: Modeling the inactivation kinetics of psychrotrophic Bacillus cereus spores. Food Research International, 67, 67–74. https://doi.org/10.1016/j.foodres.2014.10.028
- Fan, X., Sokorai, K., Weidauer, A., Gotzmann, G., Rögner, F. H., & Koch, E. (2017). Comparison of gamma and electron beam irradiation in reducing populations of E. coli artificially inoculated on mung bean, clover and fenugreek seeds, and affecting germination and growth of seeds. Radiation Physics and Chemistry, 130, 306–315. https://doi.org/10.1016/j.radphyschem.2016.09.015
- FDA. (2019). Irradiation in the production, processing, and handling of food. Retrieved December 7, 2019, from CFR - Code of Federal Regulations Title 21 website. https://www.accessdata.fda.gov/scripts/cdrh/cfdocs/cfcfr/CFRSearch.cfm?fr=179.26
- Fiester, S. E., Helfinstine, S. L., Redfearn, J. C., Uribe, R. M., & Woolverton, C. J. (2012). Electron beam irradiation dose dependently damages the Bacillus spore coat and spore membrane. International Journal of Food Microbiology, 9(1), 579593. Finten. https://doi.org/10.1155/2012/579593
- Gironés-Vilaplana, A., Huertas, J. P., Moreno, D. A., Periago, P. M., & García-Viguera, C. (2016). Quality and microbial safety evaluation of new isotonic beverages upon thermal treatments. Food Chemistry, 194, 455–462. https://doi.org/10.1016/j.foodchem.2015.08.011
- Giroux, M., Ouattara, B., Yefsah, R., Smoragiewicz, W., Saucier, L., & Lacroix, M. (2001). Combined effect of ascorbic acid and gamma irradiation on microbial and sensorial characteristics of beef patties during refrigerated storage. Journal of Agricultural and Food Chemistry, 49(2), 919–925. https://doi.org/10.1021/jf000544k
- Guérin, A., Dargaignaratz, C., Clavel, T., Broussolle, V., & Nguyen-The, C. (2017). Heat-resistance of psychrotolerant Bacillus cereus vegetative cells. Food Microbiology, 64, 195–201. https://doi.org/10.1016/j.fm.2017.01.009
- Gunes, G., Ozturk, A., Yilmaz, N., & Ozcelik, B. (2011). Maintenance of safety and quality of refrigerated ready-to-cook seasoned ground beef product (meatball) by combining gamma irradiation with modified atmosphere packaging. Journal of Food Science, 76(6). https://doi.org/10.1111/j.1750-3841.2011.02244.x
- Hertwig, C., Meneses, N., & Mathys, A. (2018). Cold atmospheric pressure plasma and low energy electron beam as alternative non-thermal decontamination technologies for dry food surfaces: A review. Trends in Food Science & Technology, 77(October 2017), 131–142. https://doi.org/10.1016/j.tifs.2018.05.011
- IAEA. (2015). Manual of good practice in food irradiation: Sanitary, phytosanitary and other applications. International Atomic Energy Agency. http://www-pub.iaea.org/MTCD/Publications/PDF/trs481web-98290059.pdf
- Jiao, S., Zhang, H., Hu, S., & Zhao, Y. (2019). Radio frequency inactivation kinetics of Bacillus cereus spores in red pepper powder with different initial water activity. Food Control, 105(June), 174–179. https://doi.org/10.1016/j.foodcont.2019.05.038
- John, E. M., Stern, M. C., Sinha, R., & Koo, J. (2011). Meat consumption, cooking practices, meat mutagens, and risk of prostate cancer. Nutrition and Cancer, 63(4), 525–537. https://doi.org/10.1080/01635581.2011.539311
- Kanzler, S., Manschein, M., Lammer, G., & Wagner, K. H. (2015). The nutrient composition of European ready meals: Protein, fat, total carbohydrates and energy. Food Chemistry, 172, 190–196. https://doi.org/10.1016/j.foodchem.2014.09.075
- Kim, H.-J., Jung, S., Yong, H. I., Bae, Y. S., Kang, S. N., Kim, I. S., & Jo, C. (2014). Improvement of microbiological safety and sensorial quality of pork jerky by electron beam irradiation and by addition of onion peel extract and barbecue flavor. Radiation Physics and Chemistry, 98, 22–28. https://doi.org/10.1016/j.radphyschem.2014.01.003
- Kim, K. I., Lee, S. Y., Hwang, I. G., Yoo, S. M., Min, S. G., & Choi, M. J. (2015). Quality characteristics of beef by different cooking methods for frozen home meal replacements. Korean Journal for Food Science of Animal Resources, 35(4), 441–448. https://doi.org/10.5851/kosfa.2015.35.4.441
- Kochhar, S. P. (1996). Oxidative pathways to the formation of off-flavours. In M. J. Saxby (Ed.), Food taints and off-flavours (pp. 168–225). https://doi.org/10.1007/978-1-4615-2151-8_6
- Lee, E. J., Ameer, K., Kim, G. R., Chung, M. S., & Kwon, J. H. (2018). Effects of approved dose of e-beam irradiation on microbiological and physicochemical qualities of dried laver products and detection of their irradiation status. Food Science and Biotechnology, 27(1), 233–240. https://doi.org/10.1007/s10068-017-0194-z
- Liao, X., Muhammad, A. I., Chen, S., Hu, Y., Ye, X., Liu, D., & Ding, T. (2018). Bacterial spore inactivation induced by cold plasma. Critical Reviews in Food Science and Nutrition, 59(16), 1–11. https://doi.org/10.1080/10408398.2018.1460797
- Liu, Y., Chen, S., Pu, Y., Muhammad, A. I., Hang, M., Liu, D., & Ye, T. (2019). Ultrasound-assisted thawing of mango pulp: Effect on thawing rate, sensory, and nutritional properties. Food Chemistry, 286, 576–583. https://doi.org/10.1016/j.foodchem.2019.02.059
- Lung, H. M., Cheng, Y. C., Chang, Y. H., Huang, H. W., Yang, B. B., & Wang, C. Y. (2015). Microbial decontamination of food by electron beam irradiation. Trends in Food Science and Technology, 44(1), 66–78. https://doi.org/10.1016/j.tifs.2015.03.005
- Lv, R., Zou, M., Chantapakul, T., Chen, W., Muhammad, A. I., Zhou, J., Ding, T., Ye, X., & Liu, D. (2019). Effect of ultrasonication and thermal and pressure treatments, individually and combined, on inactivation of Bacillus cereus spores. Applied Microbiology and Biotechnology, 103(5), 2329–2338. https://doi.org/10.1007/s00253-018-9559-3
- Milojevic, L., Velebit, B., Djordjevic, V., Jankovic, V., Lakicevic, B., Bajcic, A., & Betic, N. (2019). Screening of Bacillus cereus presence in minced meat and meat products originating from Serbian retail facilities. IOP Conference Series: Earth and Environmental Science, 333(1), 012079. https://doi.org/10.1088/1755-1315/333/1/012079
- Moon, C. T., Yancey, J. W. S., Apple, J. K., Hollenbeck, J. J., Johnson, T. M., & Winters, A. R. (2016). Quality characteristics of fresh and cooked ground beef can be improved by the incorporation of lean finely-textured beef (LFTB). Journal of Food Quality, 39(5), 465–475. https://doi.org/10.1111/jfq.12221
- Muhammad, A. I., Chen, W., Liao, X., Xiang, Q., Liu, D., Ye, X., & Ding, T. (2019). Effects of plasma-activated water and blanching on microbial and physicochemical properties of tiger nuts. Food and Bioprocess Technology, 12(10), 1721–1732. https://doi.org/10.1007/s11947-019-02323-w
- Muhammad, A. I., Li, Y., Liao, X., Liu, D., Ye, X., Chen, S., Hu, Y., Wang, J., & Ding, T. (2018). Effect of dielectric barrier discharge plasma on background microflora and physicochemical properties of tiger nut milk. Food Control, 96, 119–127. https://doi.org/10.1016/j.foodcont.2018.09.010
- Muhammad, A. I., Lv, R., Liao, X., Chen, W., Liu, D., & Ye, X. (2019). Modeling the inactivation of Bacillus cereus in tiger nut milk treated with cold atmospheric pressure plasma. Journal of Food Protection, 82(11), 1828–1836. https://doi.org/10.4315/0362-028X.JFP-18-586
- Ocloo, F. C. K., Okyere, A. A., & Asare, I. K. (2014). Physicochemical, functional and pasting properties of flour produced from gamma irradiated tiger nut (Cyperus esculentus L.). Radiation Physics and Chemistry, 103(4), 9–15. https://doi.org/10.1016/j.radphyschem.2014.05.010
- Oh, Y. J., Song, A. Y., & Min, S. C. (2017). Inhibition of salmonella typhimurium on radish sprouts using nitrogen-cold plasma. International Journal of Food Microbiology, 249, 66–71. https://doi.org/10.1016/j.ijfoodmicro.2017.03.005
- Painter, J. A., Hoekstra, R. M., Ayers, T., Tauxe, R. V., Braden, C. R., Angulo, F. J., & Griffin, P. M. (2013). Attribution of foodborne illnesses, hospitalizations, and deaths to food commodities by using outbreak data, United States, 1998-2008. Emerging Infectious Diseases, 19(3), 407–415. https://doi.org/10.3201/eid1903.111866
- Pillai, S. D., Venkateswaran, K., Cepeda, M., Soni, K., Mittasch, S., Maxim, J., & Osman, S. (2006). Electron beam (10 MeV) irradiation to decontaminate spacecraft components for planetary protection. IEEE Aerospace Conference Proceedings (pp. 1–15). https://doi.org/10.1109/aero.2006.1655743
- Ren, F., Perussello, C. A., Zhang, Z., Gaffney, M. T., Kerry, J. P., & Tiwari, B. K. (2018). Enhancement of phytochemical content and drying efficiency of onions (allium cepa L.) through blanching. Journal of the Science of Food and Agriculture, 98(4), 1300–1309. https://doi.org/10.1002/jsfa.8594
- Rosenquist, H., Smidt, L., Andersen, S. R., Jensen, G. B., & Wilcks, A. (2005). Occurrence and significance of Bacillus cereus and Bacillus thuringiensis in ready-to-eat food. FEMS Microbiology Letters, 250(1), 129–136. https://doi.org/10.1016/j.femsle.2005.06.054
- Selambakkannu, S., Bakar, K. A., Ming, T. T., & Sharif, J. (2011). Effect of gamma and electron beam irradiation on textile wastewater. Jurnal Sains Nuklear Malaysia, 23(2), 67–73.
- Singh, S., & Shalini, R. (2016). Effect of hurdle technology in food preservation: A review. Critical Reviews in Food Science and Nutrition, 56(4), 641–649. https://doi.org/10.1080/10408398.2012.761594
- Soni, A., Oey, I., Silcock, P., & Bremer, P. (2016). Bacillus spores in the food industry: A review on resistance and response to novel inactivation technologies. Comprehensive Reviews in Food Science and Food Safety, 15(6), 1139–1148. https://doi.org/10.1111/1541-4337.12231
- Tahergorabi, R., Matak, K. E., & Jaczynski, J. (2012). Application of electron beam to inactivate salmonella in food: Recent developments. Food Research International, 45(2), 685–694. https://doi.org/10.1016/j.foodres.2011.02.003
- Wells-Bennik, M. H. J., Eijlander, R. T., den Besten, H. M. W., Berendsen, E. M., Warda, A. K., Krawczyk, A. O., & Abee, T. (2016). Bacterial spores in food: Survival, emergence, and outgrowth. Annual Review of Food Science and Technology, 7(1), 457–482. https://doi.org/10.1146/annurev-food-041715-033144
- Yim, D. G., Jo, C., Cheol Kim, H., Seok Seo, K., & Nam, K. C. (2016). Application of electron-beam irradiation combined with aging for improvement of microbiological and physicochemical quality of beef loin. Korean Journal for Food Science of Animal Resources, 36(2), 215–222. https://doi.org/10.5851/kosfa.2016.36.2.215
- Zhang, Y., Moeller, R., Tran, S., Dubovcova, B., Akepsimaidis, G., Meneses, N., Drissner, D., & Mathys, A. (2018). Geobacillus and Bacillus spore inactivation by low energy electron beam technology: Resistance and influencing factors. Frontiers in Microbiology, 9(NOV), 1–10. https://doi.org/10.3389/fmicb.2018.02720
- Zhang, Z., Jiang, B., Liao, X., Yi, J., Hu, X., & Zhang, Y. (2012). Inactivation of Bacillus subtilis spores by combining high-pressure thermal sterilization and ethanol. International Journal of Food Microbiology, 160(2), 99–104. https://doi.org/10.1016/j.ijfoodmicro.2012.10.009. The above reference investigated the resistance of bacterial spore toward EB irradiation treatment and its influencing factors.