ABSTRACT
The effect of domestic cooking techniques such as boiling, frying, microwaving, and freezing on the phenolic profile of spinach, and mustard was studied using high performance liquid chromatography with diode array detection (HPLC-DAD). Nitric oxide, superoxide anion scavenging activity, and anti-choline esterase activity were studied using spectroscopic methods. Results showed 12 phenolic compounds in spinach and 13 in mustard leaves. Microwaving significantly enhanced the amounts of most of the phenolic compounds. Conversely, boiling has an enervating effect on the amounts of phenolic compounds. Raw spinach and mustard leaves have the highest nitric oxide inhibition activities of 42.4 and 62.7%, respectively, followed by microwaving and freezing. Similarly, the highest % superoxide anion scavenging activity was determined in raw spinach and mustard. The applied cooking techniques had deleterious effects on anti-cholinesterase activities. In conclusion, different processing conditions affected phenolic availability, and the antioxidant and anti-cholinesterase activities of the selected vegetable leaves.
1. Introduction
There is an enormous diversity of chemicals found across the plant kingdom that are referred to as “phenolic compounds” (PCs). These compounds can be identified by the presence of an aromatic ring with a hydroxyl group (Cartea et al., Citation2010). The molecular structure of PCs gives them redox characteristics (Aboul-Enein et al., Citation2013) and hence is linked to their antioxidant action (Kruk et al., Citation2022). PCs may be very important to enervate oxidative stress in several ways such as neutralization of reactive oxygen species (ROS), dissolution of peroxides, or suppression of singlet and triplet oxygen (Cartea et al., Citation2010). The shikimic acid pathway forms PCs as secondary metabolites (Shende et al., Citation2024). Leafy vegetables possess a diverse variety of PCs with antioxidant benefits (Zeb, Citation2020). The identification of plant-based natural antioxidants that can be utilized in disease prevention and health promotion has received the attention of researchers over the last decade. PCs have been observed as the most important families of phytochemicals with antioxidant activity (Jahangir et al., Citation2009).
Both raw and cooked/processed forms of green leafy vegetables are consumed by humans. Different cooking techniques are frequently used to inactivate microorganisms and enzymes, hence improving food safety and quality. Additionally, cooking aims to improve palatability and inactivate antinutritional compounds in food (Perdana et al., Citation2014; Williams et al., Citation2013). Boiling, frying, microwaving, and freezing are some of the cooking methods that are commonly applied in domestic settings. Cooking could significantly alter the nutritional value of green leafy vegetables, particularly their phytochemical contents (Thi & Hwang, Citation2015; Zhao et al., Citation2019). The cell wall composition is altered and degraded during food processing, which can increase the bioavailability of polyphenols (Ribas-Agustí et al., Citation2018). Bioactive metabolites have the potential to enter the bloodstream, thereby mitigating oxidative stress in the surrounding tissues (Palafox‐Carlos et al., Citation2011). Different thermal processing techniques can affect the phytochemical concentrations to varying degrees. Recently, it was observed that different processing techniques alter the carotenoid composition of Brassica leaves (Mehmood & Zeb, Citation2023b). Therefore, it is important to carefully analyse the technique for the processing of green leafy vegetables. It is also warranted to know the effects of these processing conditions on the antioxidant activities and medicinal properties.
In this paper, the impact of different cooking techniques on the phenolic composition of Spinacia oleracea L. (oriental spinach) and Brassica campestris L. (field mustard) is reported. This study aimed to fill the gap in the literature by identifying safe and appropriate domestic cooking methods that could preserve or retain the nutritional compounds and medicinal properties of selected leafy vegetables.
2. Materials and methods
2.1. Sampling
Fresh leaves (5 kg) of Spinacea oleracea L. (oriental spinach) and Brassica campestris L. (field mustard) were obtained from the local market at Batkhela, District Malakand, Khyber Pakhtunkhwa, Pakistan (latitude = 34.6138° N, longitude = 71.9283° E and altitude = 648.4 m).
2.2. Processing techniques
The vegetable leaves were processed through domestic cooking techniques such as boiling, frying, microwaving, and freezing. A portion of untreated 200 g of samples of spinach and mustard was taken as raw samples. The leaves (200 g each) were boiled in a stainless pot for 10 min. In sunflower oil, frying was undertaken for 5 min at 180°C. Similarly, the leaves were heated for 15 min in a microwave (Dawlance Microwave oven, Pakistan) at 850 W. Using a deep freezer (PEL Arctic pro, Pakistan) freezing was performed for 120 h.
2.3. Analysis of phenolic compounds
Phenolic compounds were extracted by mixing the ground paste (1 g) of the sample’s leaves with 10 mL methanol-water (9:1). Using a vortex mixer, the entire mixture was agitated for 2 h before being filtered with Whatman filter paper (pore size 5.0 μm) and then ultrafiltered through a PTFE syringe filter (pore size 0.45 μm). An HPLC vial (2.0 mL) was used to collect the filtrate. An Agilent HPLC-1260 infinity system was used for the separation of phenolic compounds. The system was equipped with a reversed-phase column (Agilent Zorbax Eclipse C-18) maintained at 25°C with a specification of 4.6 × 100 mm, 3.5 μm. Separation was achieved using binary gradient chromatography, and detection was carried out using a diode array detector (DAD). Binary elution was performed as previously reported (Zeb, Citation2015). The chromatograms within a spectral range (200–600 nm) were obtained at 280, 320, and 360 nm to confirm the identity of most of the eluted compounds. To identify and quantify each phenolic compound in the sample extract, reference standards were used. The phenolic compounds were expressed as mg/100 g of extract.
2.4. Antioxidant activities
2.4.1. Superoxide anion scavenging activity
The activity was performed by using the method of Robak and Gryglewski (Citation1988). Briefly, 0.1 M phosphate buffer (pH 7.4), NBT (150 μM), PMS (60 μM), and NADH (468 μM) were prepared. After the preparation of reagents, 1 ml of each was added to 1 ml extract of sample and was incubated for 5 min at room temperature. The absorbance of the sample was measured at 560 nm using a spectrophotometer (Shimadzu-1700, Japan). Superoxide scavenging activity was expressed as % activity.
2.4.2. Nitric oxide scavenging activity
Nitric oxide scavenging activity was performed by using the Griess Illosvoy reaction (Badami et al., Citation2003). The reagents SNP (10 mM) and Griess Reagent were prepared in 0.1 M of phosphate buffer (pH 7.2). SNP (3 mL) was taken in a test tube and 100 μL of sample extract was added followed by an incubation period of 150 min at room temperature. The, Griess reagent (3 mL) was added, and the absorbance was measured at 546 nm against the blank. Nitric oxide scavenging activity was expressed as % NO.
2.5. Anti-choline esterase activity
Using Ellman’s assay (Ellman et al., Citation1961), the anti-choline esterase activity was determined. Sample extract (50 μL) was mixed with DTNB (50 µL), to which 0.03 µL/mL of the enzyme was added. The whole mixture was incubated at 30°C for 15 min, followed by the addition of the enzyme substrate (50 µL). The absorbance of the mixture was recorded at 412 nm at the start and after four min against the blank. Calculations of enzyme activity and % inhibition were made using the rate of change of absorption with time and the activity was expressed as % Ach. E.
2.6. Statistical analysis
All experiments were carried out in replicates (n = 3). GraphPad Prism 10.0.2 was used to analyse one-way ANOVA with multiple variable comparisons using Dunnett’s multiple comparison test at p < .05. Principal component analysis (PCA) was determined by using Minitab Statistical Software (Version 18.0, Minitab Inc.). Variables (identified phenolic compounds) are stored in the PCA biplot against the observations (cooking techniques) using loading factors in the dimensional spaces specified by principal components.
3. Results
3.1. Phenolic compounds in spinach leaves
Twelve phenolic compounds were identified in spinach leaves as shown in . The first compound detected in spinach leaves was p-hydroxybenzoic acid. Peak 2 was isorhamnetin-3-(caffeoyl-diglucoside)-7-rhamnoside, apigenin was eluted at 15.4 min, Kaempferol was peak 3, and kaempferol-3-coumaroyl sinapoyl diglucoside was peak 4. Apigenin-2-pentoxide-8-hexoside, quercetin-3-(sinapoyldiglucoside)-7-glucoside, diometin-7-rutionside, quercetin-3-(p-coumaroyl-diglucoside)-7-glucoside, kaemferol-3-(p-coumaroyl-diglucoside)-7-glucoside, quercetin-3-sinapolysophoroside-7-glucoside, and quercetin-3,4-diglucoside-3-(6-feruloy-glucoside) were the compounds eluted till 33 min to elute.
Table 1. Effects of cooking techniques on the phenolic composition (mg/100 g) of spinach leaves.
3.2. Phenolic compounds in mustard leaves
Thirteen phenolic compounds were identified in mustard leaves as shown in . Briefly, p-hydroxybenzoic acid was at peak 1, peak 2 represents caffeic acid, and peak 3 was caffeoyl feruloyl quinic acid. Flavonoid glycosides constituting apigenin-7-glucoside, quercetin-3,7-diglucoside, kaempferol-3-sinapoylsophotrioside, kaemferol-3-p-coumaroylsinapoyldiglucoside, kaempferol-feruloylglucoside diglucoside, isorhamnetin-3-caffeoyl-7-rhamnoside, kaempferol 3-(caffeoyldiglucoside)-7-rhamnoside, luteolin-3-glucoside, isorhamnetin-3,7-diglucoside and quercetin-3-(sinapoyldiglucoside)-7-glucoside.
Table 2. Effects of cooking techniques on the phenolic composition (mg/100 g) of mustard leaves.
3.3. Effects of cooking on the phenolic composition of spinach leaves
also shows the effects of various cooking techniques, boiling, frying, microwaving, and freezing on the phenolic composition of spinach leaves. Microwave heating has been found to significantly increase (p < .05) the amount of p-hydroxybenzoic acid to 4076.1 mg/100 g, followed by frying (1282.5 mg/100 g), when compared to its raw counterpart (249.4 mg/100 g). The amount of p-hydroxybenzoic acid was significantly reduced to 176.6 mg/100 g, during boiling whereas the amount was further reduced to 156.1 mg/100 g during freezing. In the raw leaves, isorhamnetin-3-(caffeoyl-diglucoside)-7-rhamnoside was 68.0 mg/100 g with microwaving and was significantly increased to 1127.4 mg/100 g, whereas its amounts were reduced significantly in other treatments; boiling (7.3 mg/100 g), frying (2.6 mg/100 g) and freezing (14.7 mg/100 g). In the raw samples, the concentrations of apigenin and kaempferol were 90.8 and 16.0 mg/100 g, respectively. During microwaving, the concentrations of these compounds increased significantly to 1634.8 and 1376.7 mg/100 g, respectively. The amount of kaempferol-3-coumaroyl sinapoyl diglucoside was found to be 20.3 mg/100 g in raw samples, rising to 429.1 mg/100 g while microwaving, then 70.8 mg/100 g during frying, and 67.7 mg/100 g during freezing. When microwaved, the amounts of apigenin-2-pentoxide-8-hexoside and quercetin-3-(sinapoyldiglucoside)-7-glucoside significantly increased from 160.1 and 146.2 mg/100 g, respectively, to 1840.7 and 508.0 mg/100 g, respectively whereas other treatments hurt the concentration of these compounds. Diometin-7-rutionside was found to be 10.5 mg/100 g in the raw samples, which increased to 135.5 mg/100 g after microwave cooking, followed by frying (32.7 mg/100 g), while other treatments hurt its amounts. The concentrations of kaempferol-3-(p-coumaroyl-diglucoside) and quercetin-3-(p-coumaroyl-diglucoside)-7-glucoside were 9.1 and 108.1 mg/100 g, respectively in the raw samples. During frying, however, these amounts dramatically increased to 212.5 and 247.0 mg/100 g, respectively, whereas other treatments have been found to increase their quantity. The amount of quercetin-3-sinapolysophoroside-7-glucoside in the raw samples was 87.6 mg/100 g, but it significantly increased when fried (95.1 mg/100 g). Boiling considerably enhanced the contents of quercetin-3,4-diglucoside-3-(6-feruloyl-glucoside) to 294.0 mg/100 g when compared to the raw samples (91.9 mg/100 g), while other procedures greatly decreased its concentration.
3.4. Effects of cooking on the phenolic composition of mustard leaves
also shows the effects of various cooking techniques, boiling, frying, microwaving, and freezing on the phenolic composition of mustard leaves. The amount of p-hydroxy benzoic acid was 325.8 mg/100 g in the raw sample which was significantly (p < .05) enhanced during frying (6155.1 mg/100 g), microwaving (3899.1 mg/100 g), boiling (1471.1 mg/100 g) and freezing (604.5 mg/100 g). The amount of caffeic acid was not detected in the raw sample, whereas during frying, the amount was determined to be 226.7 mg/100 g, followed by microwaving (150.1 mg/100 g). Caffeoylferuloylquinic acid was determined only in microwaving (235.0 mg/100 g), whereas apigenin-7-glucoside was quantified both in microwaving (460.0 mg/100 g) and freezing (12.6 mg/100 g). Similarly, in the raw sample, quercetin-3,7-diglucoside was determined to be 13.3 mg/100 g, which was increased during microwaving (1217.2 mg/100 g), whereas in other treatments, it was not determined. Kaempferol-3-sinapoylsophotrioside and kaemferol-3-p-coumaroylsinapoyldiglucoside were determined to be 408.9 and 833.8 mg/100 g, respectively, during microwave treatment, while they were not reported in other treatments. The amount of kaempferol-feruloyl glucoside diglucoside was 5.3 mg/100 g in the raw samples, and significantly increased to 587.1 mg/100 g during microwaving, then decreased to 91.4 mg/100 g during freezing, 34.8 mg/100 g during frying, and 15.0 mg/100 g during boiling. In raw samples, the amount of isorhamnetin-3-caffeoyl-7-rhamnoside was 20.4 mg/100 g, whereas in microwaving, it was increased to 320.8 mg/100 g. During microwaving, the amount of kaempferol 3-(caffeoyldiglucoside)-7-rhamnoside was 377.2 mg/100 g, whereas other treatments had a deleterious effect on its concentration. The concentration of luteolin-3-glucoside was detected only in the microwave treatment and 134.9 mg/100 g. When fried, isorhamnetin-3,7-diglucoside considerably increased from the raw sample’s 125.5 to 233.6 mg/100 g. Quercetin-3-(sinapoyldiglucoside)-7-glucoside was determined to be 589.1 mg/100 g, in the raw sample, whereas the amount was significantly reduced in other treatments such as microwaving (68.1 mg/100 g), boiling (38.0 mg/100 g), frying (23.5 mg/100 g) and freezing (not detected).
3.5. Antioxidant activities
3.5.1. Nitric oxide scavenging activity
shows the effect of cooking techniques on the nitric oxide radical scavenging activity of spinach and mustard extracts. Raw spinach and mustard have been observed to possess the highest inhibition activities at 42.4 and 62.7%, respectively. The IC50 value, for raw spinach and mustard extracts were determined to be 77.9 and 59.1 μg/mL, respectively. In treated spinach, the highest inhibition activity was reported in microwaving (35.5%, IC50 = 78.5 μg/mL), followed by freezing (32.4%, IC50 = 93.2 μg/mL), boiling (31.4%, IC50 = 114.4 μg/mL) and frying (26.6%, IC50 = 91.8 μg/mL). In treated mustard, the highest activity was observed in microwaving (49.4%, IC50 = 23.4 μg/mL), followed by freezing (40.9%, IC50 = 47.4 μg/mL), frying (38.5%, IC50 = 82.2 μg/mL) and boiling (27.1%, IC50 = 117.2 μg/mL).
3.5.2. Superoxide anion scavenging activity
shows the effect of cooking techniques on the superoxide anion scavenging activity of spinach and mustard extracts. Raw spinach and mustard showed the highest % inhibition activity of superoxide anions at 44.5 and 56.1%, respectively. The IC50 values, for raw spinach and mustard extracts were determined to be 64.1 and 29.3 μg/mL, respectively. In treated spinach, the highest inhibition activity was observed in microwaving (35.6%, IC50 = 158.4 μg/mL), followed by freezing (34.8%, IC50 = 118.3 μg/mL), boiling (34.3%, IC50 = 90.4 μg/mL) and frying (29.6%, IC50 = 64.8 μg/mL). However, in treated mustard, the highest % inhibition activity was reported in microwaving (52.0%, IC50 = 14.3), followed by boiling (40.5%, IC50 = 38.2), frying (33.3%, IC50 = 64.3) and freezing (32.8%, IC50 = 146.4).
3.6. Anti-choline esterase activity
shows the effect of cooking techniques on the anti-choline esterase activity of spinach and mustard extracts. Raw spinach and mustard showed the highest % inhibition activity which was determined to be 53.7 and 20.6%, respectively. The IC50 values, for raw spinach and mustard extracts were determined to be 497.9 and 535.9 μg/mL, respectively. In treated spinach, the highest activity was observed in frying (24.6%, IC50 = 361.1 μg/mL), followed by microwaving (23.1%, IC50 = 83.9 μg/mL), boiling (20.5%, IC50 = 160.3 μg/mL) and freezing (7.6%, IC50 = 160.8 μg/mL). In treated mustard, the highest activity was determined in microwaving (14.1%, IC50 = 124.2 μg/mL), followed by boiling (12.9%. IC50 = 75.1 μg/mL), frying (6.4%, IC50 = 198.7 μg/mL) and freezing (5.6%, IC50 = 85.5 μg/mL).
3.7. Principal component analysis
represents the biplots of the loadings (cooking treatments) and scores (phenolic compounds) of spinach. The biplot loadings explained the similarities and differences between the raw and cooking treatments. Principal components (PC1 = 99.1 and PC2 = 0.6%) explained 99.7% of the variation in the treated samples. Quercetin-3-sinapolysophoroside-7-glucoside, kaemferol-3-(p-coumaroyl-diglucoside)-7-glucoside and quercetin-3,4-diglucoside-3-(6-feruloyl-glucoside) were scored in the first quadrant, showing the least significant difference with each other, whereas in the second quadrant, only quercetin-3-(p-coumaroyl-diglucoside)-7-glucoside was scored which shows qualitative difference from other compounds, and the studied treatments had no significant effect on its concentration. Similarly, the compounds scored in the third quadrant showed the least significant difference from each other. Apigenin-2-pentoxide-8-hexoside and p-hydroxybenzoic acid were scored in the fourth quadrant, which showed the significant effect of frying on their concentration when compared with other treatments.
Figure 4. The biplot of principal component analysis (PCA) of phenolic compounds of spinach leaves represents the loadings (cooking treatments) and scores (phenolic compounds) in the dimensional spaces determined by the principal components.
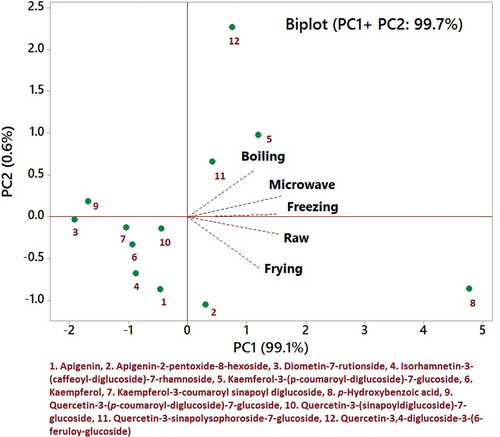
shows the biplot of loadings (cooking treatments) and scores (phenolic compounds) of mustard, in the dimensional spaces specified by the principal components that explained 98.3% of the variation (PC1 = 81.7 and PC2 = 16.6%). In the first quadrant, only p-hydroxybenzoic acid was scored, indicating a significant effect of various treatments. Isorhamnetin-3-caffeoyl-7-rhamnoside, kaempferol 3-(caffeoyldiglucoside)-7-rhamnoside and luteolin-3-glucoside were allocated in the second quadrant, which showed the least significant difference (similar) from each other, whereas isorhamnetin-3,7-diglucoside and quercetin-3-(sinapoyldiglucoside)-7-glucoside had significant effects upon treatments. In the third quadrant, the majority of compounds were allocated very close to each other, indicating less variation with each other and the effect of the studied treatments.
4. Discussion
Spinach and mustard leaves are extensively consumed as cooked food from the kitchen to food industries; hence, they are considered essential components of our daily food menu. In domestic as well as industrial settings, these vegetables are usually processed through common cooking techniques, such as boiling, frying, microwaving, and freezing. Thus, this study provides important information on considering the selected vegetables processing. PCs are water soluble and may leech into the surrounding water; therefore, the amount of PCs may be affected during boiling (Traoré et al., Citation2017; Yao & Ren, Citation2011). Such findings corroborate this study, as most of the PCs of spinach and mustard were degraded after boiling. During frying, the amount of PCs considerably increased. However, in the spinach sample, the amount of isorhamnetin-3-(caffeoyl-diglucoside)-7-rhamnoside and quercetin-3-(sinapoyldiglucoside)-7-glucoside was significantly decreased during frying. Similarly, the amount of PCs was significantly increased in mustard leaves after frying except quercetin-3,7-diglucoside, isorhamnetin-3-caffeoyl-7-rhamnoside and quercetin-3-(sinapoyldiglucoside)-7-glucoside. According to Fellows (Citation2000), frying is usually carried out at high temperatures which may vaporize the water contents and soften the food matrix (Hossain et al., Citation2017), hence the compounds may be concentrated. Zeb (Citation2021) also concluded that PCs might play a crucial role in the enhancement of the thermal stability of frying oil during cooking. During microwaving the amount of most of the PCs in spinach and mustard was significantly increased. Similar findings were reported by Bembem et al. (Citation2014), who reported a significant increase in the phenolic contents of mustard leaves. In different studies, Subramaniam et al. (Citation2017) and Moorthy et al. (Citation2020) reported that microwave heating enhanced the bioavailability of PCs through cell wall disruption. The findings in this study are also paralleled with those of Sultana et al. (Citation2008), and Mehmood and Zeb (Citation2023a), who reported a net increase in the phenolic contents of spinach leaves. Similarly, Kamiloglu (Citation2020) observed a significant decrease in the phenolic acid and flavonoid contents of spinach leaves during freezing, similar to this study, as the amounts of PCs were significantly decreased during freezing. The antioxidant potential of leafy vegetables has been demonstrated by antioxidant assays, and their potential could be triggered by polyphenol and flavonoid contents (Bergman et al., Citation2001; Oh et al., Citation2016). In this study, the antioxidant activities of the treated samples were significantly decreased compared to those of their raw forms. According to Podsędek (Citation2007), the antioxidant activity of vegetables may be affected during cooking which brings quality changes, antioxidant breakdown, or leaching of antioxidant compounds during heat treatments. Zhang and Hamauzu (Citation2004) also reported that such compounds are heat sensitive and might be decreased during such treatments. In a previous study, Mehmood and Zeb (Citation2023a) reported that the antioxidant potential of spinach leaves is affected by various heat treatments, particularly during boiling and frying, while microwaving significantly increased the antioxidant activity of spinach leaves. This study is in agreement with previous findings as a significant increase was observed in the superoxide anion scavenging and nitric oxide scavenging activity of both spinach and mustard leaves when microwaved. According to Thi and Hwang (Citation2015), the superoxide anion scavenging activity of water spinach was significantly increased after boiling, which could also be compared to this study as they observed the effects of steaming and boiling on the superoxide anion scavenging activity of water spinach and did not report the effects of other cooking techniques. In this study, the effect of cooking techniques on the anti-choline esterase activity of spinach and mustard was also observed. It was concluded that the applied treatments have deleterious effects on anti-choline esterase activity. The additional sensory analysis of the samples after each treatment would clarify our understanding about the potential acceptability of the final product.
5. Conclusion
Selected vegetables might gain an advantage from microwave heating having more phenolic compounds and antioxidant potential. Compared to other treatments, boiling has detrimental effects on spinach and mustard leaves’ phenolic profiles and antioxidant potentials. Chromatography revealed 12 and 13 phenolic compounds in spinach and mustard leaves, respectively. Among the selected treatments, microwave treatment had enhanced the phenolic composition, whereas declined by boiling. Nitric oxide inhibition activities were highest in microwave and freezing treatments. The applied treatments could also have a deleterious impact on the anti-choline esterase activity of selected leafy vegetables.
Acknowledgment
The authors are grateful to the Department of Biochemistry, University of Malakand for providing the facilities to conduct this work.
Disclosure statement
No potential conflict of interest was reported by the author(s).
Data availability statement
The manuscript contains all the data created during the study.
References
- Aboul-Enein, H. Y., Berczynski, P., & Kruk, I. (2013). Phenolic compounds: The role of redox regulation in neurodegenerative disease and cancer. Mini Reviews in Medicinal Chemistry, 13(3), 385–8. https://doi.org/10.2174/138955713804999766
- Badami, S., Gupta, M. K., & Suresh, B. (2003). Antioxidant activity of the ethanolic extract of striga orobanchioides. Journal of Ethnopharmacology, 85(2–3), 227–230. https://doi.org/10.1016/S0378-8741(03)00021-7
- Bembem, K., Sadana, B., & Bains, K. (2014). Effect of domestic cooking methods on the nutritive and antioxidative components of mustard leaves (Brassica juncea). International Journal of Food, Agriculture & Veterinary Sciences, 4(1), 24–31.
- Bergman, M., Varshavsky, L., Gottlieb, H. E., & Grossman, S. (2001). The antioxidant activity of aqueous spinach extract: Chemical identification of active fractions. Phytochemistry, 58(1), 143–152. https://doi.org/10.1016/S0031-9422(01)00137-6
- Cartea, M. E., Francisco, M., Soengas, P., & Velasco, P. (2010). Phenolic compounds in Brassica vegetables. Molecules, 16(1), 251–280. https://doi.org/10.3390/molecules16010251
- Ellman, G. L., Courtney, K. D., Andres, V. Jr., & Featherstone, R. M. (1961). A new and rapid colorimetric determination of acetylcholinesterase activity. Biochemical Pharmacology, 7(2), 88–95. https://doi.org/10.1016/0006-2952(61)90145-9
- Fellows, P. (2000). Food Processing Technology: Principles and Practice (3rd ed., pp. 369–380). Cambridge: Woodhead Publishing Limited.
- Hossain, A., Khatun, M. A., Islam, M., & Huque, R. (2017). Enhancement of antioxidant quality of green leafy vegetables upon different cooking method. Preventive Nutrition and Food Science, 22(3), 216. https://doi.org/10.3746/pnf.2017.22.3.216
- Jahangir, M., Kim, H. K., Choi, Y. H., & Verpoorte, R. (2009). Health‐affecting compounds in Brassicaceae. Comprehensive Reviews in Food Science and Food Safety, 8(2), 31–43. https://doi.org/10.1111/j.1541-4337.2008.00065.x
- Kamiloglu, S. (2020). Industrial freezing effects on the content and bioaccessibility of spinach (Spinacia oleracea L.) polyphenols. Journal of the Science of Food & Agriculture, 100(11), 4190–4198. https://doi.org/10.1002/jsfa.10458
- Kruk, J., Aboul-Enein, B. H., Duchnik, E., & Marchlewicz, M. (2022). Antioxidative properties of phenolic compounds and their effect on oxidative stress induced by severe physical exercise. The Journal of Physiological Sciences, 72(1), 1–24. https://doi.org/10.1186/s12576-022-00845-1
- Mehmood, A., & Zeb, A. (2023a). Effects of different cooking techniques on the carotenoids composition, phenolic contents, and antioxidant activity of spinach leaves. Journal of Food Measurement and Characterization, 17(5), 4760–4774. https://doi.org/10.1007/s11694-023-01962-0
- Mehmood, A., & Zeb, A. (2023b). Effects of different processing conditions on the carotenoid’s composition, phenolic contents, and antioxidant activities of Brassica campestris leaves. Heliyon, 9(11), e21191. https://doi.org/10.1016/j.heliyon.2023.e21191
- Moorthy, P., Rajan, M., Sathyanarayanan, S., Muniyandi, K., Sivaraj, D., Sasidharan, S. P., & Thangaraj, P. (2020). Effect of different cooking methods of Hibiscus surratensis L. leaf vegetable on nutritional, anti-nutritional composition, and antioxidant activities. Journal of Culinary Science & Technology, 18(1), 13–28. https://doi.org/10.1080/15428052.2018.1502110
- Oh, S., Kim, K., & Choi, M. (2016). Antioxidant activity of different parts of dolsan leaf mustard. Food Science & Biotechnology, 25(5), 1463–1467. https://doi.org/10.1007/s10068-016-0227-z
- Palafox‐Carlos, H., Ayala‐Zavala, J. F., & González‐Aguilar, G. A. (2011). The role of dietary fiber in the bioaccessibility and bioavailability of fruit and vegetable antioxidants. Journal of Food Science, 76(1), R6–R15. https://doi.org/10.1111/j.1750-3841.2010.01957.x
- Perdana, J., den Besten, H. M., Aryani, D. C., Kutahya, O., Fox, M. B., Kleerebezem, M., Boom, R. M., & Schutyser, M. A. (2014). Inactivation of lactobacillus plantarum WCFS1 during spray drying and storage assessed with complementary viability determination methods. Food Research International, 64, 212–217. https://doi.org/10.1016/j.foodres.2014.06.029
- Podsędek, A. (2007). Natural antioxidants and antioxidant capacity of brassica vegetables: A review. LWT - Food Science & Technology, 40(1), 1–11. https://doi.org/10.1016/j.lwt.2005.07.023
- Ribas-Agustí, A., Martín-Belloso, O., Soliva-Fortuny, R., & Elez-Martínez, P. (2018). Food processing strategies to enhance phenolic compounds bioaccessibility and bioavailability in plant-based foods. Critical Reviews in Food Science and Nutrition, 58(15), 2531–2548. https://doi.org/10.1080/10408398.2017.1331200
- Robak, J., & Gryglewski, R. J. (1988). Flavonoids are scavengers of superoxide anions. Biochemical Pharmacology, 37(5), 837–841. https://doi.org/10.1016/0006-2952(88)90169-4
- Shende, V. V., Bauman, K. D., & Moore, B. S. (2024). The shikimate pathway: Gateway to metabolic diversity. Natural Product Reports, 41(4), 604–648. https://doi.org/10.1039/D3NP00037K
- Subramaniam, S., Rosdi, M. H. B., & Kuppusamy, U. R. (2017). Customized cooking methods enhance antioxidant, antiglycemic, and insulin-like properties of momordica charantia and moringa oleifera. Journal of Food Quality, 2017, 1–9. https://doi.org/10.1155/2017/9561325
- Sultana, B., Anwar, F., & Iqbal, S. (2008). Effect of different cooking methods on the antioxidant activity of some vegetables from Pakistan. International Journal of Food Science & Technology, 43(3), 560–567. https://doi.org/10.1111/j.1365-2621.2006.01504.x
- Thi, N. D., & Hwang, E.-S. (2015). Effects of different cooking methods on bioactive compound content and antioxidant activity of water spinach (ipomoea aquatica). Food Science & Biotechnology, 24(3), 799–806. https://doi.org/10.1007/s10068-015-0104-1
- Traoré, K., Parkouda, C., Savadogo, A., Ba/Hama, F., Kamga, R., & Traoré, Y. (2017). Effect of processing methods on the nutritional content of three traditional vegetables leaves: Amaranth, black nightshade and jute mallow. Food Science and Nutrition, 5(6), 1139–1144. https://doi.org/10.1002/fsn3.504
- Williams, D. J., Edwards, D., Hamernig, I., Jian, L., James, A. P., Johnson, S. K., & Tapsell, L. C. (2013). Vegetables containing phytochemicals with potential anti-obesity properties: A review. Food Research International, 52(1), 323–333. https://doi.org/10.1016/j.foodres.2013.03.015
- Yao, Y., & Ren, G. (2011). Effect of thermal treatment on phenolic composition and antioxidant activities of two celery cultivars. LWT - Food Science & Technology, 44(1), 181–185. https://doi.org/10.1016/j.lwt.2010.07.001
- Zeb, A. (2015). Phenolic profile and antioxidant potential of wild watercress (Nasturtium officinale L.). Springer Plus, 4(1), 714. https://doi.org/10.1186/s40064-015-1514-5
- Zeb, A. (2020). Concept, mechanism, and applications of phenolic antioxidants in foods. Journal of Food Biochemistry, 44(9), e13394. https://doi.org/10.1111/jfbc.13394
- Zeb, A. (2021). A comprehensive review on different classes of polyphenolic compounds present in edible oils. Food Research International, 143, 110312. https://doi.org/10.1016/j.foodres.2021.110312
- Zhang, D., & Hamauzu, Y. (2004). Phenolics, ascorbic acid, carotenoids and antioxidant activity of broccoli and their changes during conventional and microwave cooking. Food Chemistry, 88(4), 503–509. https://doi.org/10.1016/j.foodchem.2004.01.065
- Zhao, C., Liu, Y., Lai, S., Cao, H., Guan, Y., San Cheang, W., Liu, B., Zhao, K., Miao, S., Riviere, C., Capanoglu, E., & Xiao, J. (2019). Effects of domestic cooking process on the chemical and biological properties of dietary phytochemicals. Trends in Food Science & Technology, 85, 55–66. https://doi.org/10.1016/j.tifs.2019.01.004