Abstract
Bioluminescence (BLI) and fluorescence imaging (FI) allow for non-invasive detection of viable microorganisms from within living tissue and are thus ideally suited for in vivo probiotic studies. Highly sensitive optical imaging techniques detect signals from the excitation of fluorescent proteins, or luciferase-catalyzed oxidation reactions. The excellent relation between microbial numbers and photon emission allow for quantification of tagged bacteria in vivo with extreme accuracy. More information is gained over a shorter period compared to traditional pre-clinical animal studies. The review summarizes the latest advances in in vivo bioluminescence and fluorescence imaging and points out the advantages and limitations of different techniques. The practical application of BLI and FI in the tracking of lactic acid bacteria in animal models is addressed.
Keywords:
Abbreviations
BLI | = | bioluminescence |
CCD | = | charge-coupled device |
FI | = | fluorescence imaging |
FMNH2 | = | flavin mononucleotide |
GFP | = | green fluorescent protein |
GIT | = | gastro-intestinal tract |
IBS | = | irritable bowel syndrome |
IVIS | = | in vivo imaging system |
LAB | = | lactic acid bacteria |
MRI | = | magnetic resonance imaging |
NIR | = | near-infrared |
RFP | = | red fluorescent proteins |
Zn-DPA | = | zinc(II) dipicolyamine. |
Introduction
Lactic acid bacteria (LAB) have several health benefits and a number of claims have been made relating to probiotic properties, e.g. the alleviation of constipation, treatment and prevention of atopic dermatitis, treatment of irritable bowel syndrome (IBS), prevention of cardiovascular diseases and, more recently, the treatment of cancerous tumors.Citation1,2 The chance of finding a single strain that adheres to all of these properties is remote, hence the reason for so many strain combinations in probiotic preparations. Many of the strains are selected based on in vitro-attained phenotypic properties that adhere to the general definition of a probiotic and to a lesser extent the ability of cells to colonize the gastro-intestinal tract (GIT). It is thus not surprising that the probiotic market is dominated by a few well-studied species of the genera Lactobacillus and Bifidobacterium.Citation2 It is only in recent years that reports have been published on the probiotic properties of LAB from genera such as Streptococcus, Leuconostoc, Lactococcus and Enterococcus.Citation3-5 Enterococci are usually not included in probiotic preparations, due to their association with abnormal physiological conditions, underlying disease and immunosuppression.Citation6 Despite the few negative reports, clinical trials have shown that Enterococcus faecium SF68 is effective in the prevention and treatment of diarrheaCitation7 and lowers the cholesterol levels in serum.Citation8 Enterococcus faecium CRL 183 lowered cholesterol levels by 43% in in vitro testsCitation9 and E. faecium Fargo 688→ alleviated symptoms of IBS.Citation10,11 Enterococcus mundtii ST4SA colonised the GIT of mice, competed against Clostridium sporogenes and Enterococcus faecalis for adherence to epithelial cells and mucus,Citation12 produces a broad-spectrum bacteriocinCitation3,13,14 and prevented symptoms of Salmonella infection in Wistar rats.Citation15 The more we learn about the survival of other genera of LAB in the GIT, the more probiotic properties may be discovered.
Survival of probiotic bacteria is usually evaluated based on the ability of the cells to adhere to the GIT. Most of these studies are performed in vitro on human cell lines such as Caco-2, HT-29 and HT29-MTX.Citation16 In vitro studies in GIT-models that simulate the human intestineCitation2 is a step closer to real conditions in the intestine, but remains an in vitro approach. Although in vitro studies provide valuable insight into the metabolism, adherence and antimicrobial activity of probiotic cells, the interaction between microbial and epithelial cells, and interaction among microorganisms in the GIT can only be studied in vivo. Cell interactions are best studied with labeled cells, i.e. cells that express genes encoding easily detectable reporter proteins. Tracking of probiotic strains through the GIT is possible, provided that the proteins are expressed constitutively and, preferably, without being influenced by changes in physiological conditions.Citation17
Many reporter proteins are unstable when expressed in the GIT or mature slowly once expressed.Citation18 The challenge is thus not so much to express a reporter protein in vivo, but to select a protein with the longest possible half-life. Overexpression of the reporter protein is also not a prerequisite, since low levels can be detected with the latest development in imaging technology. One of the most used detection systems is the Caliper in vivo image system (IVIS), equipped with a highly sensitive charge-coupled device (CCD) camera (Caliper Life Sciences, Hopkinton, MA, USA).
One of the best examples illustrating the value of a reporter system was the study on daptomycin and its effect against infections caused by Staphylococcus aureus.Citation19 The authors used a luciferase-labeled strain of S. aureus in a murine model. The same technology was used to study the spread of Listeria monocytogenes infection in a murine modelCitation20 and later by many other research groups to study the migration of bacteria in the GIT.Citation21-23 In this review, the advantages and disadvantages of different in vivo reporter systems are discussed, with emphasis on bioluminescent and fluorescent proteins.
Reporter Systems
Genes are selected as reporters when the characteristics of the expressed protein(s) allow easy detection of the cells that carry the reporter in a complex microbial environment.Citation24 A number of reporter molecules with improved activity have been developed over the past 2 decades.Citation25 Light emitted by these molecules is detected using advanced photon detectors in CCD cameras mounted within light-tight specimen chambers.Citation26 Macroscopic in vivo biophonic imaging is a highly sensitive, non-invasive, non-toxic and quantitative optical technique that is based on the detection of light produced by either fluorescent proteins or by enzyme-catalyzed oxidation reactions (bioluminescence). As opposed to traditional pre-clinical animal studies, in vivo optical imaging techniques such as BLI and FI enable researchers to tag bacteria and determine their cell numbers in vivo without taking biopsies or killing the animals.Citation27
Examples of useful imaging systems that are commercially available, including the various features of each, are listed in . By using sensitive light detection imaging systems such as the IVIS, the production of light from within living tissue is detected and quantified as it passes through different tissue types, including the skin, muscle and bone. In vivo bioluminescence and fluorescence imaging are usually performed using a planar imaging technique.Citation27 Firstly, a reference image is acquired under weak illumination. This is followed by illuminating the subject with light passing through a filter that is set to the excitation wavelength of a specified fluorophore. The emitted light signal is then captured in complete darkness using particular bandwidth emission filters. Bioluminescence imaging is performed in the same manner, without the need for a second illumination step. The exposure times required can vary from a second to several minutes, depending on the strength and location of the biophotonic signal within the animal. The resulting images are displayed in pseudocolour superimposed on the reference photographic images.
Table 1. Commercially available imaging systems
Before transforming a gene construct encoding bioluminescence or fluorescence into a microbial cell, it is important to access the suitability of the reporter gene for the specific experiment. If a bioluminescent phenotype is required, the choice of luciferase system usually depends on the microorganism of interest. An inherent advantage of the bacterial luciferase system is the ability to produce a bioluminescent signal without the need to add a substrate. The disadvantage is that lux genes are generally not optimally expressed in Gram-positive bacteria.Citation28,29 To circumvent the problem, Gram-positive ribosomal binding sites were cloned into the lux operon.Citation30
A vast array of reporter systems is available (). The most popular include bioluminescence with bacterial lux genes (lux), firefly and click beetle luciferase (luc), and fluorescence recorded in the case of green and red proteins.Citation22,31,32 Other well established imaging systems include magnetic resonance imaging (MRI) and positron emission tomography.Citation25,33
Table 2. Properties of selected fluorescent reporter proteins
Table 3. Imaging systems utilizing bioluminescent bacteria
In vivo Fluorescence
Fluorescence is defined as the emission of light from a chemical substrate when excited with a specific wavelength. Thus, to record fluorescence, the excitation and emission wavelengths have to be carefully selected, but also the conditions that support photostability and maturation of the proteinCitation27 A number of fluorescent proteins (FPs) and probes have been developed for biological and molecular research.
Fluorescent proteins
Fluorescent proteins are genetically encoded in vivo reporters that are easily imagedCitation34,35 and have been used to tag other proteins, eukaryotic and prokaryotic cells.Citation36,37 A comparison of 3 of the most common and widely used reporter systems that confer identifiable characteristics is listed in . For a FP to be effectively used as a reporter molecule in in vivo optical imaging, it has to maintain emission levels when constantly illuminated and has to reach peak intensity within a specific time, a concept referred to as maturation time of the chromophore.Citation38
Table 4. Comparison of Lux, GFP and mCherry
Green fluorescent protein (GFP) is encoded by a gene originally isolated from the jellyfish Aequoria victoria.Citation39 Osamu Shimomura, Roger Tsien and Martin Chalfie were awarded the Nobel Prize in Chemistry for the impact GFP technology had on research in life sciences. The first GFP gene was cloned and expressed in other organisms, including E. coli, during the early 1990s.Citation40 Since then, numerous derivatives of GFP with enhanced fluorescence and the potential to be expressed in different organisms were constructed. Cells expressing GFP, and derivatives thereof, emit light in the blue, green and yellow range of the spectrum. The protein emits light when excited by long-wavelength UV or blue light, without the requirement for an exogenous substrate or complex nutrients.Citation31,41 Green fluorescent proteins have been expressed in a number of LAB, including Lactobacillus sakei, Lactobacillus fructosus, Lactobacillus delbrueckii subsp. lactis and E. faecalis.Citation42,43 GFP was also be used to detect Lactobacillus spp. in the GIT of chickens.Citation42 Recently, Vibrio cholera cells expressing GFP were used to visualize gastro-intestinal infection in zebrafish larvae.Citation44 Similarly, Rieu and co-workersCitation45 infected zebrafish larvae with cells of Lactobacillus casei expressing GFP to study GIT colonisation.
A variety of fluorescent proteins have been discovered and developed since the first application of GFP as a marker of gene expression in the nematode Caenorhabditis elegans.Citation46 This includes the discovery of the DsRed FP from the coral Discosoma sp and the development of a range of far-red FPs termed the ‘mFruits’.Citation34 The use of the DsRed fluorescent protein in live-cell imaging was hampered due to its slow maturation time (caused by its tetrameric form) and low photo stability.Citation47 The ‘mFruit’ FPs, developed in the laboratory of Roger Tsien are based on molecular-directed evolution of the DsRed FP.Citation34 Characteristics that render FPs most suitable for optical imaging are listed in .
To improve maturation time, brightness and photo-stability, optimized variants of red fluorescent proteins (RFP) were developed, of which mCherry, encoded by the mCherry gene, is the best known derivative.Citation34,48,49 The protein has a monomeric structure which matures from a nascent polypeptide into a folded fluorescent form within 15 min. Maximal fluorescence is reached in less than 2 h in E. coli and the protein matures 4 times faster than the DsRed protein it was derived from.Citation34
The monomeric structure of the mCherry fluorescent protein makes it ideal for protein fusions, as monomeric proteins tend to be the least disruptive to the function of the protein to which they are fused. The mCherry protein is non-toxic, and it can be expressed at high levels without adding any unwanted physiological stresses to host organisms.Citation32 The mCherry fluorophore is also highly resistant to photo bleaching, which means that the fluorophore will not lose its ability to fluoresce during continuous illumination.Citation50 The mCherry gene has been codon-optimized for optimal expression in various organisms,Citation32,34,35,51,52 including probiotic lactic acid bacteria (). Van Zyl and coworkersCitation52 used the mCherry gene to study the colonization of Lactobacillus plantarum and Enterococcus mundtii strains in the intestinal tract of mice.
Figure 1. Lactobacillus plantarum 423 dam::cat-mCherry cells expressing the red fluorescent mCherry gene in the GIT of mice ex vivo. The color bar indicates the relative signal intensity measured as photons/sec/cm-2/sr. Image modified from van Zyl.Citation52
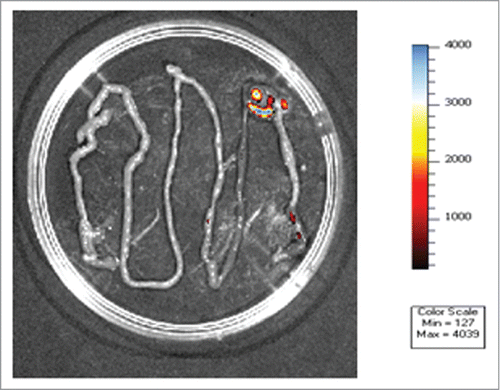
Alternatives to fluorescent proteins
Genetic engineering of microorganisms is often time consuming and requires a considerable level of expertise in molecular biology. Although genetically encoded markers are used in biological sciences, they are not always easy to apply. A second strategy employs an NIR molecular probe that consists of a fluorescent reporter group.Citation53 The authors used a synthetic zinc(II) dipicolyamine (Zn-DPA) coordination complex that binds to anionic surfaces of bacterial cells. Carbocyanine dye, acting as injectable near-infrared (NIR) probe, attaches selectively to an affinity group with 2 Zn-DPA units and stains the whole cell. The probe has an excitation wavelength of 794 nm and emission wavelength of 810 nm. By using in vitro fluorescence microscopy, the authors have shown that the probe can be used to stain the periphery of S. aureus cells. In addition, they also demonstrated that the probe could be used in vivo to target S. aureus in a mouse leg infection model.Citation53
Limitations and side effects of fluorescent proteins
In recent years, bacterial imaging has become an emerging technology with many applications in biological science. Despite this, there are numerous limitations and challenges that affect the application of molecular markers.Citation38 Optical imaging methods such as radio-imaging and MRI are much more developed than in vivo imaging of bacteria.Citation53 A clear drawback of optical imaging within live animals is the penetration of light through tissue. Although higher wavelength bacterial imaging systems such as NIR probes can penetrate readily through animal tissue, the imaging of bacterial cells in deeper tissues with higher background fluorescence is often problematic.Citation27 The deeper the tissue penetration, the more bacterial cells are required to produce a detectable fluorescent signal. Typically, for each centimeter of tissue depth, there is an approximate 10-fold loss in photon intensity.Citation54 Endogenous chromophores such as hemoglobin, keratin and collagen absorb light within tissues in the visible spectrum (400–760 nm).Citation55 Generally, the absorption of wavelengths longer than 600 nm (red part of the spectrum) by endogenously produced fluorophores is reduced, allowing the propagation of red light through several centimeters of tissue.Citation56,57
In many applications, the use of fluorescent proteins such as DsRed is hampered by a slow maturation time, photobleaching, oligomerization, brightness of the chromophore and cell toxicity.Citation34 According to Wiedemann and co-workers,Citation38 a critical characteristic of fluorescent proteins suitable for in vivo application is brightness.Citation38 Brightness is defined as the product of the ability of a chromophore to absorb excitation light (molar extinction coefficient) and re-emit photons (quantum yield of fluorescence).Citation17,27 Consequently, fluorescent proteins with a higher extinction coefficient and quantum yield have higher physical brightness. The physical brightness of a chromophore may also be affected by the translation efficiency of the marker protein. Brightness of the monomeric mRuby RFP was increased 5 – 8-fold by codon-optimization of the marker protein for expression in mammalian cells.Citation58,59 Furthermore, the usefulness of fluorescent proteins for imaging is significantly influenced by the total number of functional molecules expressed, the percentage of proteins that develop into mature chromophores, the turnover rate of nascent polypeptides into functional chromophores and the time required before photobleaching occurs.Citation34,38
Phototoxicity is one of the most adverse side effects FPs may have on living cells and tissue. Light of short and longer wavelengths induces a phototoxic effect in cells.Citation60,61 Many of the negative effects related to the exposure of living cells to UV and visible light can be avoided by the use of microscopic methods that use infrared light for imaging of FPs.Citation62
In vivo Bioluminescence
Bioluminescence, defined as the release of energy in the form of light due to a chemical reaction, has been described for many bacteria, marine crustaceans, fungi, fish and insects. Of these examples, a group of enzymes collectively named luciferases are the best studied and are used in the labeling of bacteria, eukaryotic cells and studies that involve gene expression.Citation26,54 Luciferase generates visible light that arises from the oxidation of a substrate in the presence of ATP.Citation63 The best studied bioluminescent systems used in in vivo imaging are those that originated from bacterium Photorhabdus luminescens, the firefly Photinus pyralis, click beetle Pyrophorus plagiopthalamus and the marine copepod Gaussia princeps.Citation27
Bacterial lux bioluminescence
Luminous bacteria are the most widely distributed light-emitting organisms, with the majority existing in seawater and the rest living in terrestrial or freshwater environments. The strains usually belong to one of 3 genera; Photobacterium, Vibrio or Photorhabdus. Marine species are usually planktonic and are members of the genera Photobacterium or Vibrio, whereas terrestrial bacteria are closely associated with fish, squid and nematodes and are grouped within the genus Photorhabdus.Citation64
A bioluminescent signal (light) forms in the presence of oxygen and when the reaction is catalyzed by luciferase. The expression of genes encoding bioluminescence is regulated by the lux operon.Citation65 The lux operon used in most molecular studies () originates from a Photorhabdus sp.Citation30,54 Bacterial luciferase is encoded by the structural genes luxA and luxB. Luciferase is thus a heterodimer composed of 2 different polypeptides known as α and β, respectively. Substrates for the bacterial luciferase are reduced flavin mononucleotide (FMNH2), O2 and long chain fatty aldehyde produced by the fatty acid reductase complex that is encoded by the luxCDE genes (). Two regulatory genes, luxR and luxI, encoding the regulatory protein and auto-inducer synthetase, respectively, are also required for light emission.
Figure 2. Arrangement of the luxCDABE open reading frames in Photobacterium spp, Vibrio spp. and Photorhabdus spp The luxI and luxR genes are adjacent to one another and luxI is the first of the 7 genes in the operon. luxI and luxR are required for activation of the luminescence genes.
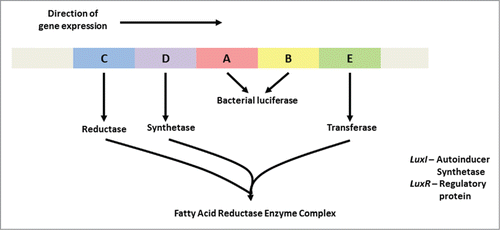
One of the most significant advantages of the bacterial luciferase system is the ability of the bacteria to synthesize the substrate (long chain aldehydes and FMNH2) required for light production. When eukaryotic bioluminescence systems such as the click beetle luciferase are expressed in bacteria, the substrate (luciferin) has to be added exogenously.Citation28 The optimum temperature of the P. luminescence lux operon lies within the same range as that of mammalian tissues, making it one of the most suitable bacterial luciferase systems for in vivo applications.Citation66
The bacterial luciferase system is predominantly used when bacterial infection models are studied.Citation30,67 An example of this is the cloning of the P. luminescence lux operon into infectious pathogens such as E. coli, Citrobacter rodentium, Listeria monocytogenes and Salmonella sppCitation20-23 Pathogenicity of the labeled bacteria remained unaffected and they were visualized throughout infection. Bacteria labeled with the lux genes were detected in the GIT of mice ().Citation52 Integration of the lux genes into the chromosome of bacteria significantly increases the stability of the enzyme in terms of the light emitted and is generally preferred over the use of plasmid expression.
Figure 3. Monitoring of the colonization of Listeria monocytogenes EGDe in the intestinal tract of mice. Strain EGDe contains the luxABCDE operon of Photorhabdus luminescence. The bioluminescence signal was measured transcutaneously 30 min after infection, using an IVIS system (Caliper Life Sciences). The image was acquired using an integration time of 1 min. The color bar indicates the relative signal intensity measured as photons/sec/cm-2/sr. Image modified from van Zyl.Citation52
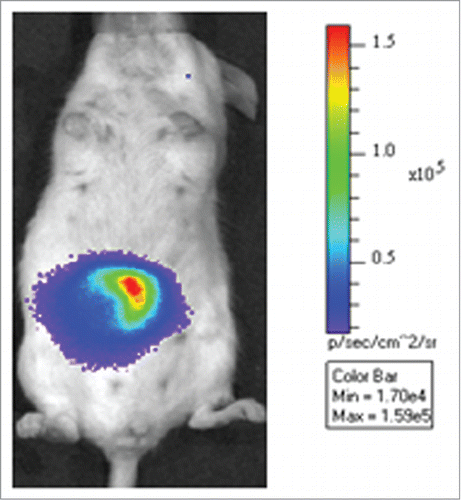
Firefly bioluminescence
Other than bacterial luciferase, the most commonly used luciferases for in vivo bioluminescence imaging is the luciferase (luc) genes from the North American firefly, P. pyralis and the click beetle P. plagiopthalamus. Expression in bacteria requires codon-optimization of the luc genes.Citation28 The firefly luciferase enzyme is approximately 62 kDa in size and is encoded by a single gene, luc.Citation68 The luminescence reaction involves oxidation of the substrate D-luciferin (D-(-)-2-(6’-hydroxy-2’benzothiazolyl) thiazone-4-carboxylic acid) in the presence of Mg-ATP. This results in the production of oxyluciferin, CO2 and light.Citation26,69
The P. pyralis luciferase enzyme produces light with an emission peak at 560 nm.Citation65 However, generation of light by firefly luciferase is affected by temperature, producing light at a peak of 610 nm at 37oC, rendering it suitable for in vivo applications.Citation26 Researchers have developed a number of luc genes with improved expression in bacterial and eukaryotic cells, with shifted emission spectra, by genetically modifying the wild type luc genes.Citation65
The amount of light emitted in relation to energy consumed by the firefly luciferase represents the most efficient bioluminescent reaction known and is, therefore, the most attractive choice for expression in mammalian cells.Citation27,70 Researchers have expressed the firefly luciferase successfully in muscle tissue and the nasal airways of mice.Citation71,72 Daniel and coworkersCitation28 tagged lactic acid bacteria with the P. pyralis luciferase using a plasmid expression system, revealing valuable information about the spatio-temporal persistence of the bacteria in mice.
Limitations and side-effects of luciferase systems in marker applications
One of the most significant drawbacks of the bacterial and firefly luciferases is the relation of bioluminescent light production to the metabolic activity of the microorganisms expressing the luciferase enzymes.Citation26 Luciferases rely heavily on the availability of microbial metabolites for continuous light emission. Bacterial luciferase relies on the bio-availability of FMNH2, whereas firefly luciferase requires ATP. This is important to note during in vivo infection studies, as a decrease or an absence of bioluminescence production by marked pathogens could be due to the bacteria being in stationary growth phase and not due to eradication of the infection.Citation20,29,30 Supporting this notion, an absence of bioluminescence production as a result of bacteria being in a phase of dormancy has been reported for lux-expressing Leishmania amazonesisCitation73 and Mycobacterium smegmatis.Citation32
Absorption of light by mammalian tissues is one of the main factors influencing the sensitivity and detection limits of optical imaging (). Hemoglobin serves as one of the most significant causes of light absorption in animals.Citation55 While hemoglobin absorbs light in the visible spectrum (400–760 nm), longer wavelengths of light (above 600 nm) propagate through mammalian tissue more readily.Citation56 Furthermore, melanin influences the absorption of light in animals with dark fur. Nude animals or animals with white fur are thus more suited for in vivo optical imaging studies.
Table 5. Factors that can affect the sensitivity of imaging systems Citation27
Since luciferases are oxygenases, molecular oxygen is a basic requirement for all luciferase enzymes to produce light. The lack of oxygen may thus limit the application of luciferases as reporters in anaerobic environments such as the GIT.Citation26 However, oxygen enters the GIT via diffusion from oxygen-rich tissue surrounding the lumen. A low level of oxygen was sufficient for production of detectable levels of bioluminescence by lux-expressing Citrobacter rodentium cells that colonized the murine GIT.Citation23
Expression of Reporter Genes in Lactic Acid Bacteria
Tracking of LAB in a complex environment such as the GIT is a challenge and requires the constitutive expression of reporter genes. Added to the challenge are the differences in transformation efficiency obtained for strains of the same species, as demonstrated by using the same electroporation protocol on different strains of L. crispatus.Citation74,75
Reporter genes are usually cloned into plasmids or integrated into the genome. By integration into the genome, gene expression is much more stable and antibiotic selection is not required, which is a big advantage in animal studies.Citation76 Integration into the genome also provides the added advantage of stabilizing the exogenous DNA by irreversible incorporation.Citation77 Target sequences for the genomic integration of reporter genes should be genes or regions that are non-essential to the host to ensure that the physiology of the host remains unchanged.Citation78 It is also important to make sure that recombinant strains only differ from the wild type by the newly acquired characteristics (fluorescence or bioluminescence). Complex experiments to prove that the host cell physiology has not been negatively affected, can be avoided by disrupting a non-functional genetic locus on the host chromosome. Prophage genes that are generally considered to be transcriptionally silent in the lysogenic phase are good examples of genetic loci that are often used for homologous recombination of foreign genes in LAB. Several LAB species harbour phage genes on their chromosomes, and heterologous DNA can be inserted, for example into the attB sites present in many prophages.Citation76 A more refined integration strategy makes use of 2 recombination events for integration. Following the integration of the entire plasmid, a second recombination event occurs to remove the plasmid backbone, while the gene of interest is irreversibly left behind in the host chromosome.Citation79 The event in which recombination occurs followed by looping out of the vector is determined by how the homologous sequences are arranged on the vector. Double recombination integration has been used with many LAB species, e.g., Lactobacillus casei, Lactobacillus plantarum and Lactobacillus acidophilus.Citation80
Since very few chromosomal sites have been investigated that will allow high levels of reporter gene expression in LAB, cloning of reporter genes into multiple copy number plasmids remains an attractive alternative for constitutive expression,Citation81 providing that the plasmids are maintained by the host.Citation28,82 Plasmid reporter gene expression levels are usually higher than those of some chromosomally integrated genes.Citation83
Translation efficiency of a protein in a heterologous host organism can be significantly increased by modifying the codon usage frequency, thus codon optimization.Citation84 To enhance the translational efficiency of a reporter gene, the gene can be chemically synthesized with an optimized codon usage for expression in specific LAB. Garcia-Cayuela and de CarinanosCitation35 constructed fluorescent protein vectors for analyzing the expression strength of different promoters in LAB and E. coli. The authors used a synthetic red fluorescent protein that had been codon-optimized for increased expression in L. plantarum and E. faecalis. Luciferase reporter genes from eukaryotes such as the firefly (P. pyralis) or click beetle (P. plagiopthalamus) have a codon usage that is not suitable for expression in bacteria. Nucleotide sequences have to be optimised to maximize expression of the luciferase reporter protein in LAB. Firefly luciferase expression in M. tuberculosis increased 30-fold in signal intensity after codon-optimization.Citation27
Conclusion
Several reporter systems are available to study the survival of lactic acid bacteria in the mammalian GIT. Many of the reporter genes are either not expressed in the GIT or the luminescence produced is too weak to be detected with optical imaging methods. The excitation and emission wavelengths have to be carefully selected. The advantage of bacterial luciferase is that it requires FMNH2, and not ATP, as in the case of firefly luciferase. This is important in studying the presence of pathogens in the GIT, as the bioluminescence signal is not linked to the metabolic activity (ATP levels) of the pathogen. Furthermore, expression of the firefly luciferase in bacteria requires codon-optimization.
Disclosure of Potential Conflicts of Interest
No potential conflicts of interest were disclosed.
References
- FAO/WHO. Guidelines for the Evaluation of Probiotics in Food. Joint FAO/WHO Working Group Report on Drafting Guidelines for the Evaluation of Probiotics in Food. 2002; ftp://ftp.fao.org/es/esn/food/wgreport2.pdf
- Dicks LMT, Botes M. Probiotic lactic acid bacteria in the gastro-intestinal tract: health benefits, safety and mode of action. Benef Microbes 2010; 1:11-29; PMID:21831747; http://dx.doi.org/10.3920/BM2009.0012
- Botes M, van Reenen CA, Dicks LMT. Evaluation of Enterococcus mundtii ST4SA and Lactobacillus plantarum 423 as probiotics using a gastro-intestinal model with infant milk formulations as substrate. Int J Food Microbiol 2008; 128:362-70; PMID:18963159; http://dx.doi.org/10.1016/j.ijfoodmicro.2008.09.016
- Sharma R, Bhaskar B, Sanodiya BS, Thakur GS, Jaiswal P, Yadav N, Sharma A, Bisen PS. Probiotic efficacy and potential of Streptococcus thermophiles modulating human health: A synoptic review. J Pharm Biol Sci 2014; 9:52–8
- Kekkonen RA, Kajasto E, Miettinen M, Veckman V, Korpela R, Julkunen I. Probiotic Leuconostoc mesenteroides ssp. cremoris and Streptococcus thermophiles induce IL-12 and IFN-γ production. World J Gastroenterol 2008; 14(8):1192-203; PMID:18300344; http://dx.doi.org/10.3748/wjg.14.1192
- Franz CMAP, Stiles ME, Schleifer K-H, Holzapfel WH. Enterococci in foods-a conundrum for food safety. Int J Food Microbiol 2003; 88:105-22; PMID:14596984; http://dx.doi.org/10.1016/S0168-1605(03)00174-0
- Lewenstein A, Frigerio G, Moroni M. Biological properties of SF68, a new approach for the treatment of diarrhoeal diseases. Curr Therap Res 1979; 26:967-81
- Agerbaek M, Gerdes LU, Richelsen B. Hypocholesterolemic effect of a new fermented milk product in healthy middle-aged men. Eur J Clin Nutr 1995; 49:346–52; PMID:7664720
- Rossi EA, Vendramini RC, Carlos IZ, Pei YC, Valdez GF. Development of a novel fermented soymilk product with potential probiotic properties. Eur Food Res Technol 1999; 209:305–7; http://dx.doi.org/10.1007/s002170050499
- Allen WD, Linggood MA, Porter P. Enterococcus organisms and their use as probiotics in alleviating irritable bowel syndrome symptoms. European Patent 1996; 0508701 (B1)
- Gardiner GE, Ross RP, Wallace JM, Scanlan FP, Jägers PPJM, Fitzgerald GF, Collins JK, Stanton C. Influence of a probiotic adjunct culture of Enterococcus faecium on the quality of cheddar cheese. J Agric Food Chem 1999; 47:4907–16; PMID:10606551; http://dx.doi.org/10.1021/jf990277m
- Ramiah K, van Reenen CA, Dicks LMT. Surface-bound proteins of Lactobacillus plantarum 423 that contribute to adhesion of Caco-2 cells and their role in competitive exclusion and displacement of Clostridium sporogenes and Enterococcus faecalis. Res Microbiol 2008; 159:470–5; PMID:18619532; http://dx.doi.org/10.1016/j.resmic.2008.06.002
- Ramiah K, ten Doeschate K, Smith R, Dicks LMT. Safety Assessment of Enterococcus mundtii ST4SA and Lactobacillus plantarum 423 determined in trials with Wistar rats. Probiot Antimicrob Prot 2009; 1:15–23; http://dx.doi.org/10.1007/s12602-009-9010-2
- Botes M, Loos B, van Reenen CA, Dicks LMT. Adhesion of the probiotic strains Enterococcus mundtii ST4SA and Lactobacillus plantarum 423 to Caco-2 cells under conditions simulating the intestinal tract, and in the presence of antibiotics and inflammatory medicaments. Arch Microbiol 2008; 190:573–84; PMID:18641972; http://dx.doi.org/10.1007/s00203-008-0408-0
- Dicks LMT, ten Doeschate K. Enterococcus mundtii ST4SA and Lactobacillus plantarum 423 alleviated symptoms of Salmonella Infection, as determined in Wistar rats challenged with Salmonella enterica serovar Typhimurium. Curr Microbiol 2010; 61:184–9; PMID:20127245; http://dx.doi.org/10.1007/s00284-010-9594-5
- Sambuy Y, De Angelis I, Ranaldi G, Scarino ML, Stammati A, Zucco F. The Caco-2 cell line as a model of the intestinal barrier: influence of cell and culture-related factors on Caco-2 cell functional characteristics. Cell Bio Toxicol 2005; 21:1–26; http://dx.doi.org/10.1007/s10565-005-0085-6
- Lehmann S, Garayoa EG, Blanc A, Keist R, Schibli R, Rudin M. Recoding intracellular molecular events from the outside: Glycosylphosphadtidylinositol-anchored avidin as a reporter protein for in vivo imaging. J Nucl Med 2011; 52:445–52; PMID:21321260; http://dx.doi.org/10.2967/jnumed.110.082412
- Lagendijk EL, Validov S, Lamers GEM, de Weert S, Bloemberg GV. Genetic tools for tagging Gram-negative bacteria with mCherry for visualization in vitro and in natural habitats, biofilm and pathogenicity studies. FEMS Microbiol Letts 2010; 305:81–90; http://dx.doi.org/10.1111/j.1574-6968.2010.01916.x
- Mortin, LI, Li T, Van Praagh AD, Zhang S, Zhang X, Alder JD. Rapid bactericidal activity of Daptomycin against methicillin-resistant and methicillin-susceptible Staphylococcus aureus Peritinitis in mice as measured with bioluminescent bacteria. Antimicrob Agents Chemother 2007; 5(51):1787–94; http://dx.doi.org/10.1128/AAC.00738-06
- Hardy J, Margolis JJ, Contag CH. Induced biliary excretion of Listeria monocytogenes. Infect Immun 2006; 74(2):1819–27; PMID:16495556; http://dx.doi.org/10.1128/IAI.74.3.1819-1827.2006
- Foucault ML, Thomas L, Goussard S, Branchini BR, Grillot-Courvalin C. In vivo bioluminescence imaging for the study of intestinal colonization by Escherichia coli in mice. Appl Environ Microbiol 2010; 76:264–74; PMID:19880653; http://dx.doi.org/10.1128/AEM.01686-09
- Rhee KJ, Cheng H, Harris A, Morin C, Kaper JB, Hecht G. Determination of spatial and temporal colonization of enteropathogenic E. coli and enterohemorrhagic E. coli in mice. Gut Microbes 2011; 2:34–41; PMID:21637016; http://dx.doi.org/10.4161/gmic.2.1.14882
- Wiles S, Pickard KM, Peng K, MacDonald TT, Frankel G. In vivo bioluminescence imaging of the murine pathogen Citrobacter rodentium. Infect Immun 2006; 74:5391–6; PMID:16926434; http://dx.doi.org/10.1128/IAI.00848-06
- Koo J, Kim Y, Kim J, Yeom M, Lee IC, Nam HG. A GUS/luciferase fusion reporter for plant gene trapping and for assay of promoter activity with luciferin-dependent control of the reporter protein stability. P Cell Phys 2007; 48(8):1121–31; http://dx.doi.org/10.1093/pcp/pcm081
- Maire E, Lelievre E, Brau D, Lyons A, Woodward M, Fafeur V, Vandenbunder B. Development of an ultralow-light-level luminescence image analysis system for dynamic measurements of transcriptional activity in living and migrating cells. Anal Biochem 2000; 280:118–27; PMID:10805529; http://dx.doi.org/10.1006/abio.2000.4503
- Contag CH, Bachmann MH. Advances in in vivo bioluminescence imaging of gene expression. Ann Rev Biomed Eng 2002; 4:235–7; http://dx.doi.org/10.1146/annurev.bioeng.4.111901.093336
- Andrue N, Zelmer A, Wiles S. Noninvasive biophotonic imaging for studies of infectious disease. FEMS Microbiol Rev 2011; 35:360–94; PMID:20955395; http://dx.doi.org/10.1111/j.1574-6976.2010.00252.x
- Daniel C, Poiret S, Dennin V, Boutillier D, Pot B. Bioluminescence imaging study of spatial and temporal persistence of Lactobacillus plantarum and Lactococcus lactis in living mice. Appl Environ Microbiol 2013; 79(4):1086–94; PMID:23204409; http://dx.doi.org/10.1128/AEM.03221-12
- Francis KP, Joh D, Bellinger-Kawahara C, Hawkinson MJ, Purchio TF, Contag PR. Monitoring of bioluminescent Staphylococcus aureus infections in living mice using a novel luxABCDE construct. Infect Immun 2000; 68:3594–600; PMID:10816517; http://dx.doi.org/10.1128/IAI.68.6.3594-3600.2000
- Francis KP, Yu J, Bellinger-Kawahara C, Joh D, Hawkinson MJ, Xiao G, Purchio TF, Caparon G, Lipsitch M, Contag PR. Visualizing pneumococcal infections in the lungs of live mice using bioluminescent Streptococcus pneumoniae transformed with a novel gram-positive lux transposon. Infect Immun 2001; 69:3350–8; PMID:11292758; http://dx.doi.org/10.1128/IAI.69.5.3350-3358.2001
- Pinheiro LB, Gibbs MD, Vesey G, Smith JJ. Fluorescent reference strains of bacteria by chromosomal integration of a modified green fluorescent protein gene. Appl Microbiol Biotechnol 2008; 77(6):1287–95; PMID:17994234; http://dx.doi.org/10.1007/s00253-007-1253-9
- Carroll P, Schreuder LJ, Muwanguzi-Karugaba J, Wiles S, Robertson BD, Ripoll J, Ward TH, Bancroft GJ, Schaible UE, Parish T. Sensitive detection of gene expression in Mycobacteria under replicating and non-replicating conditions using optimized far-red reporters. PLoS ONE 2010; 5(3):9823; http://dx.doi.org/10.1371/journal.pone.0009823
- Dothager RS, Flentie K, Moss B, Pan M, Kesarwala A, Piwnica-Worms D. Advances in bioluminescence imaging of live animals. Curr Opin Biotechnol 2009; 20:45–53; PMID:19233638; http://dx.doi.org/10.1016/j.copbio.2009.01.007
- Shaner NC, Campbell RE, Steinbach PA, Giepmans BN, Palmer AE, Tsien RY. Improved monomeric red, orange and yellow fluorescent proteins derived from Discosoma sp. red fluorescent protein. Nat Biotechnol 2004; 22(12): 1567–72; PMID:15558047; http://dx.doi.org/10.1038/nbt1037
- Garcia-Cayuela T, Gomez de Carinanos LP. Fluorescent protein vectors for promoter analysis in lactic acid bacteria and Escherichia coli. Appl Microbiol Biotechnol 2012; 96:171–81; PMID:22534822; http://dx.doi.org/10.1007/s00253-012-4087-z
- Frommer WB, Davidson MW, Campbell RE. Genetically encoded biosensors based on engineered fluorescent proteins. Chem Soc Rev 2009; 38:2833–41; PMID:19771330; http://dx.doi.org/10.1039/b907749a
- Chudakov DM, Lukyanov S, Lukyanov KA. Fluorescent protein as a toolkit for in vivo imaging. Trends Biotechnol 2005; 26:605–13; http://dx.doi.org/10.1016/j.tibtech.2005.10.005
- Wiedenmann J, Oswald F, Nienhaus GU. Fluorescent proteins for live cell imaging: opportunities, limitations and challenges. Life 2009; 61(11): 1029–42; PMID:19859977
- Shimomura O, Johnson FH, Saiga Y. Extraction, purification and properties of aequorin, a bioluminescent protein from the luminous hydromedusan, Aequorea. J Cell Compar Phys 1962; 59:223–39; http://dx.doi.org/10.1002/jcp.1030590302
- Prasher DC, Eckenrode VK, Ward WW, Prendergast FG, Cormier MJ. Primary structure of the Aequorea victoria green-fluorescent protein. Gene 1992; 111(2):229–33; PMID:1347277; http://dx.doi.org/10.1016/0378-1119(92)90691-H
- Ropp JD, Donahue CJ, Wolfgang-Kimball D, Hooley JJ, Chin JYW, Hoffman RA, Cuthbertson RA, Bauer KD. Aequorea green fluorescent protein analysis by flow cytometry. Cytometry 1995; 21:309–17; PMID:8608728; http://dx.doi.org/10.1002/cyto.990210402
- Yu Q, Dong S, Zhu W, Yang Q. Use of green fluorescent protein to monitor Lactobacillus in the gastro-intestinal tract of chicken. FEMS Microbiol Letts 2007; 275:207–13; http://dx.doi.org/10.1111/j.1574-6968.2007.00877.x
- Scott KP, Mercer DK, Richardson AJ, Melville CM, Glover LA, Flint HJ. Chromosomal integration of the green fluorescent protein gene in lactic acid bacteria and the survival of the marked strains in human gut simulations. FEMS Microbiol Letts 2000; 182:23–7; http://dx.doi.org/10.1111/j.1574-6968.2000.tb08867.x
- Runft DL, Mitchell KC, Abuaita BH, Allen JP, Bajer S, Ginsburg K, Neely MN, Withey JH. Zebrafish as a natural host model for Vibrio cholera colonization and transmission. Appl Environ Microbiol 2014; 80:1710–17; PMID:24375135; http://dx.doi.org/10.1128/AEM.03580-13
- Rieu A, Aoudia N, Jego G, Chluba J, Yousfi N, Briandet R, Deschamps J, Gasquet B, Monedero V, Garrido C, Guzzo J. The biofilm mode of life boosts the anti-inflammatory properties of Lactobacillus. Cell Microbiol 2014; 16:1836–53; PMID:25052472; http://dx.doi.org/10.1111/cmi.12331
- Chalfie M, Tu Y, Euskirchen G, Ward WW, Prasher DC. Green fluorescent protein as a marker for gene expression. Science 1994; 263(5148): 802–5; PMID:8303295; http://dx.doi.org/10.1126/science.8303295
- Bevis BJ, Glick BS. Rapidly maturing variants of the Discosoma red fluorescent protein (DsRed). Nat Biotechnol 2002; 20:83–7; PMID:11753367; http://dx.doi.org/10.1038/nbt0102-83
- Chapagain PP, Regmi CK, Castillo W. Fluorescent protein barrel fluctuations and oxygen diffusion pathways in mCherry. J Chem Phys 2011; 135(23):235101; PMID:22191901; http://dx.doi.org/10.1063/1.3660197
- Doherty GP, Bailey K, Lewis PJ. Stage-specific fluorescence intensity of GFP and mCherry during sporulation in Bacillus subtilis. BMC Res Notes 2010; 3:303–10; PMID:21073756
- Viegas MS, Martins TC, Seco F, do Carmo A. An improved and cost-effective methodology for the reduction of autofluorescence in direct immunofluorescence studies on formalin-fixed paraffin-embedded tissues. Eur J Histochem 2007; 51(1):59–66; PMID:17548270
- Leroch M, Mernke D, Koppenhoefer D, Schneider P, Mosbach A, Doehlemann G, Hahn M. Living colors in the gray mold pathogen Botrytis cinerea: Codon-optimized genes encoding green fluorescent protein and mcherry, which exhibit bright fluorescence. Appl Environ Microbiol 2011; 77(9):2887–97; PMID:21378036; http://dx.doi.org/10.1128/AEM.02644-10
- Van Zyl WF. Fluorescence and bioluminescence imaging of the intestinal colonization of Enterococcus mundtii ST4SA and Lactobacillus plantarum 423 in mice infected with Listeria monocytogenes EGde. MSc thesis, University of Stellenbosch, Stellenbosch, South Africa 2015.
- Leevy WM, Gammon ST, Jiang H. Optical imaging of bacterial infection in living mice using a fluorescent near infrared molecular probe. J Am Chem Soc 2006; 128:16476–7; PMID:17177377; http://dx.doi.org/10.1021/ja0665592
- Contag CH, Contag PR, Mullins JI, Spilman SD, Stevenson DK, Benaron DA. Photonic detection of bacterial pathogens in living hosts. Mol Microbiol 1995; 18:593–603; PMID:8817482; http://dx.doi.org/10.1111/j.1365-2958.1995.mmi_18040593.x
- Taroni P, Pifferi A, Torricelli A, Comelli D, Cubeddu R. In vivo absorption and scattering spectroscopy of biological tissues. Photochem Photobiol Sci 2003; 2:124–9; PMID:12664972; http://dx.doi.org/10.1039/b209651j
- Rice BW, Cable MD, Nelson MB. In vivo imaging of light-emitting probes. J Biomed Opt 2001; 6:432–40; PMID:11728202; http://dx.doi.org/10.1117/1.1413210
- Troy T, Jekic-McMullen D, Sambucetti L, Rice B. Qauntitative comparison of the sensitivity of detection of fluorescent and bioluminescent reporters in animal models. Mol Imaging 2004; 3:9–23; PMID:15142408; http://dx.doi.org/10.1162/153535004773861688
- Kredel S, Oswald F, Nienhaus K, Deuschle K, Roecker C, Wolff M, Heilker R, Nienhaus GU, Wiedenmann J. mRuby, a bright monomeric ref fluorescent protein for labelling of subcellular structures. Plos ONE 2009; 4:e4391; PMID:19194514; http://dx.doi.org/10.1371/journal.pone.0004391
- Gross LA, Baird GS, Hoffman RC, Baldridge KK, Tsien RY. The structure of the chromophore within DsRed, a red fluorescent protein from coral. Proc Natl Acad Sci USA 2000; 97:11990–5; PMID:11050230; http://dx.doi.org/10.1073/pnas.97.22.11990
- Morys M, Berger D. Accurate measurements of biologically effective ultraviolet radiation. Proc SPIE 1993; 2049:152–61; http://dx.doi.org/10.1117/12.163506
- Setlow RB, Grist E, Thompson K, Woodhead AD. Wavelengths effective in induction of malignant melanoma. Proc Natl Acad Sci 1993; 90:6666–70; PMID:8341684; http://dx.doi.org/10.1073/pnas.90.14.6666
- Tsutsui H, Karasawa S, Shimizu H, Nukina N, Miyawaki A. Semi-rational engineering of a coral fluorescent protein into an efficient highlighter. EMBO Rep 2005; 6:233–238; PMID:15731765; http://dx.doi.org/10.1038/sj.embor.7400361
- Wilson T, Hastings JW. Bioluminescence. Ann Rev Cell Dev Biol 1998; 14:197–230; http://dx.doi.org/10.1146/annurev.cellbio.14.1.197
- Lin LY, Meighen EA. Bacterial bioluminescence. Opin Struct Biol 2009; 5:798–809
- Hastings JW. Chemistries and colors of bioluminescent reactions: a review. Gene 1996; 173:5–11; PMID:8707056; http://dx.doi.org/10.1016/0378-1119(95)00676-1
- Szittner R, Meighen E. Nucleotide sequence, expression, and properties of luciferase coded by lux genes from a terrestrial bacterium. J Biol Chem 1990; 265:16581–7; PMID:2204626
- Brand AM, Smith R, de Kwaadsteniet M, Dicks LMT. Development of a murine model with optimal routes for bacterial infection and treatment, as determined with bioluminescent imaging in C57BL/6 Mice. Prob Antimicrob Prot 2011; 3:125–31; http://dx.doi.org/10.1007/s12602-011-9069-4
- De Wet JR, Wood KV, DeLuca M, Helsinki DR, Subramani S. Firefly luciferase gene: structure and expression in mammalian cells. Mol Cell Biol 1987; 7:725–37; PMID:3821727
- McElroy WD, DeLuca MA. Firefly and bacterial luminescence: basic science and applications. J Appl Biochem 1983; 5:197–209; PMID:6680120
- Ando Y, Niwa K, Yamada N. Firefly bioluminescence quantum yield and colour change by pH-sensitive green emission. Nat Photonics 2007; 2:44–7; http://dx.doi.org/10.1038/nphoton.2007.251
- Bloquel C, Trollet C, Pradines E, Seguin J, Scherman D, Bureau MF. Optical imaging of luminescence for in vivo quantification of gene electrotransfer in mouse muscle and knee. BMC Biotechnol 2006; 6:16; PMID:16524461; http://dx.doi.org/10.1186/1472-6750-6-16
- Doyle TC, Nawotka KA, Kawahara CB, Francis KP, Contag PR. Visualizing fungal infections in living mice using bioluminescent pathogenic Candida albicans strains transformed with the firefly luciferase gene. Microb Pathogenesis 2006; 40:82–90; http://dx.doi.org/10.1016/j.micpath.2005.11.003
- Lang T, Goyard S, Lebastard M, Milon G. Bioluminescent Leishmania expressing luciferase for rapid and high throughput screening of drugs acting on amastigote harbouring macrophages and for quantitative real-time monitoring of parasitism features in living mice. Cell Microbiol 2005; 7:383–92; PMID:15679841; http://dx.doi.org/10.1111/j.1462-5822.2004.00468.x
- Heravi RM, Nasiraii LR, Sankian M, Kermanshahi H, Varasteh AR. Optimization and comparison of two electrotransformation methods for lactobacilli. Biotechnol 2012; 11(1):50–54; http://dx.doi.org/10.3923/biotech.2012.50.54
- Beasley SS, Takala TM, Reunanean J, Apajalahti J, Saris PE. Characterization and electrotransformation of Lactobacillus crispatus isolated from chicken crop and intestine. Poult Sci 2004; 83:45–8; PMID:14761083; http://dx.doi.org/10.1093/ps/83.1.45
- Douglas GL, Klaenhammer TR. Directed chromosomal integration and expression of the reporter gene gusA3 in Lactobacillus acidophilus NCFM. Appl Environ Microbiol 2011; 77(20): 7365–71; PMID:21873486; http://dx.doi.org/10.1128/AEM.06028-11
- Heap JT, Ehsaan M, Cooksley CM, Ng Y, Cartman ST, Winzer K, Minton NP. Integration of DNA into bacterial chromosomes from plasmids without a counter selection marker. Nucl Acids Res 2012; 40(8) e59; PMID:22259038; http://dx.doi.org/10.1093/nar/gkr1321
- Rossi F, Capoaglio A, Dellaglio F. Genetic modification of Lactobacillus plantarum by heterologous gene integration in a not functional region of the chromosome. Appl Environ Microbiol 2008; 80:79–86
- Gosalbes MJ, Esteban CD, Galan JL, Perez-Martinez G. Integrative food-grade expression system based on the lactose regulon of Lactobacillus casei. Appl Environ Microbiol 2000; 66:4822–8; PMID:11055930; http://dx.doi.org/10.1128/AEM.66.11.4822-4828.2000
- Hols P, Ferain T, Garmyn D, Bernard N, Delcour J. Use of homologous expression-secretion signals and vector-free stable chromosomal integration in engineering of Lactobacillus plantarum for alpha-amylase and levanase expression. Appl Environ Microbiol 1994; 60(5):1401–3; PMID:8017927
- O’Sullivan DJ, Klaenhammer TR. High- and low- copy number Lactococcus shuttle cloning vectors with features for clone screening. Gene 1993; 137:227–31; PMID:Can't; http://dx.doi.org/10.1016/0378-1119(93)90011-Q
- Geoffroy M, Guyard C, Quatannens B, Pavan S, Lange M, Mercenier A. Use of green fluorescent protein to tag lactic acid bacterium strains under development as live vaccine vectors. Appl Environ Microbiol 2000; 66(1):383–91; PMID:10618252; http://dx.doi.org/10.1128/AEM.66.1.383-391.2000
- Martin MC, Alonso JC, Suarez JE, Alvarez MA. Genetic food-grade recombinant lactic acid bacterium strains by site-specific recombination. Appl Environ Microbiol 2000; 66(6): 2555–604; PMID:10831438; http://dx.doi.org/10.1128/AEM.66.6.2555-2564.2000
- Gupta S, Joshi L. Codon optimization. Computational Bioscience. Arizona State University. 2003
- Crawford MA, Zhu Y, Green CS. Antimicrobial effects of interferon-inducible CXC chemokines against Bacillus anthracis spores and bacilli. Infect Immun 2009; 77:1664–78; PMID:19179419; http://dx.doi.org/10.1128/IAI.01208-08
- Radhakrishnan GK, Yu Q, Harms JS, Splitter GA. Brucella TIR domain-containing protein mimics properties of the Toll-like receptor adaptor protein TIRAP. J Biol Chem 2009; 284:9892–8; PMID:19196716; http://dx.doi.org/10.1074/jbc.M805458200
- Cronin M, Sleator RD, Hill C, Fitzgerald GF, van Sinderin D. Development of luciferase-based reporter system to monitor Bifidobacterium breve UCC2003 persistence in mice. BMC Microbiol. 2008; 8:161; PMID:18816375; http://dx.doi.org/10.1186/1471-2180-8-161
- Andreu N, Zelmer A, Fletcher T. Optimisation of bioluminescent reporters for use with mycobacteria. PLoS One 2010; 5:e10777; PMID:20520722; http://dx.doi.org/10.1371/journal.pone.0010777
- Burkatovskaya M, Tegos GP, Swietlik E, Demidova TN, Castano AP, Hamblin MR. Use of chitosan bandage to prevent fatal infections developing from highly contaminated wounds in mice. Biomat 2006. 27: 4157–64; http://dx.doi.org/10.1016/j.biomaterials.2006.03.028
- Sedgley C, Applegate B, Nagel A, Hall D. Real-time imaging and quantification of bioluminescent bacteria in root canals in vitro. J Endodont 2004; 30:893–8; http://dx.doi.org/10.1097/01.DON.0000132299.02265.6C