ABSTRACT
Short-chain fatty acids (SCFAs) are produced by microbial fermentation of dietary fiber in the gut. Butyrate is a particularly important SCFA with anti-inflammatory properties and is generally present at lower levels in inflammatory diseases associated with gut microbiota dysbiosis in mammals. We aimed to determine if SCFAs are produced by the zebrafish microbiome and if SCFAs exert conserved effects on zebrafish immunity as an example of the non-mammalian vertebrate immune system. We demonstrate that bacterial communities from adult zebrafish intestines synthesize all three main SCFA in vitro, although SCFA were below our detectable limits in zebrafish intestines in vivo. Immersion in butyrate, but not acetate or propionate, reduced the recruitment of neutrophils and M1-type pro-inflammatory macrophages to wounds. We found conservation of butyrate sensing by neutrophils via orthologs of the hydroxycarboxylic acid receptor 1 (hcar1) gene. Neutrophils from Hcar1-depleted embryos were no longer responsive to the anti-inflammatory effects of butyrate, while macrophage sensitivity to butyrate was independent of Hcar1. Our data demonstrate conservation of anti-inflammatory butyrate effects and identify the presence of a conserved molecular receptor in fish.
Introduction
Short-chain fatty acids (SCFAs) are microbial metabolites produced in the gut by the anaerobic fermentation of dietary fiber and protein in the large intestine.Citation1 The most abundant SCFAs are acetate, butyrate, and propionate. In addition to providing the host with an energy source, microbially derived SCFAs exert anti-inflammatory effects through inhibition of histone deacetylates (HDAC) and activation of G-protein-coupled receptors (GPCRs).Citation2 Most research on SCFAs has reported their anti-inflammatory properties in mammals.Citation3–5 However, the anti-inflammatory mechanism responsible for the anti-inflammatory effects of SCFA administration has not been reported in fish species to date.
Zebrafish are an important model of vertebrate gut physiology with key experimental advantages including high fecundity, transparency, and well-developed gut digestive function by 6 d post fertilization (dpf).Citation6 There is a high degree of intestinal immune conservation across vertebrates, including the sensitivity of zebrafish intestinal epithelial cell progenitors to butyrate.Citation7–9 However, SCFA production has not been previously observed in the intestines of zebrafish, and it is unclear if the intestinal lumen of the zebrafish intestine provides a suitable niche for SCFA production.Citation9 Previous genomic investigation has identified the presence of butyrate metabolic genes in the zebrafish gut metagenome; however, the key bacterial enzyme for butyrate synthesis butyryl coenzyme A (CoA):acetate-CoA transferase has not been specifically detected.Citation9,Citation10 Acetate, butyrate, and propionate have been measured in several species of teleosts.Citation11–15
We have used conservation between mammalian intestinal function and immunity to create zebrafish models of human intestinal inflammation.Citation16,Citation17 Key pattern recognition molecule families, such as the Toll-like receptors and Nod-like receptors, are evolutionarily ancient, and have conserved roles in zebrafish intestinal immunity.Citation7,Citation8,Citation18,Citation19 However, conservation of host molecules responsible for sensing SCFAs has not been explored in teleosts. Mammals utilize a wide range of molecules to sense SCFAs including G protein-coupled receptors (GPRs) GPR81 (also known as HCAR1) which is primarily present on immune cells and GPR109A which is expressed on intestinal epithelial cells. Microbially derived SCFAs also exert direct effects on host physiology through histone deacetylase (HDAC) inhibition.Citation20–22
In this study, we investigated whether SCFAs are produced in the zebrafish intestine, and if the anti-inflammatory effects and sensing of SCFAs are conserved in zebrafish. We find that the pattern of SCFA production by the zebrafish intestinal microbiota is different from that seen in mammals, but that the anti-inflammatory effects mechanisms of butyrate are conserved across vertebrate species and development regardless of the ability of their endogenous microbiota to produce measurable butyrate.
Methods
Zebrafish handling
Adult zebrafish were housed at the Centenary Institute (Sydney Local Health District AEC Approval 17–036) and Duke University School of Medicine (Duke Institutional Animal Care and Use Committee Protocol Approval A115-16-05). Adult zebrafish experimentation was approved by the Institutional Animal Care and Use Committees of Duke University approval A115-16-05. Zebrafish adults were reared, housed, and fed as previously described.Citation23 All zebrafish embryo research experiments and procedures were completed in accordance with Sydney Local Health District animal ethics guidelines under approval 17–036. Zebrafish embryos were obtained by natural spawning and embryos were maintained and raised in E3 media at 28ºC.
SCFA quantification from adult zebrafish
Adult zebrafish were euthanized with 200–300 mg/L of ethyl 3-aminobenzoate methanesulfonate (tricaine) (Sigma, E10521) prior to dissection. For each sample, intestines dissected from five adults (90+ dpf) EK WT zebrafish males (roughly 0.2 g total) were pooled and homogenized using a Precellys 24 High-Powered Bead Homogenizer at 5500 rpm for 3 cycles at 20 seconds per cycle with a 10 second delay between cycles. Samples were then acidified with HCl to a pH below 3, pelleted by centrifugation, and the supernatant was harvested. Filtered supernatant was stored at −80°C until quantification.
SCFA quantification as carried out on an Agilent 7890B GC FID, with an HP-FFAP capillary column (25 m length, ID 0.2 mm, film thickness 0.33 µm). Concentrations were determined using a linear model fit of a standard curve that encompasses the sample concentration range. Standardized concentrations used for each C2-C5 SCFA were as follows: 0.2, 0.5, 1, 2, 4, and 8 mM.
In vitro synthesis of SCFA by zebrafish gut commensals
Freshly dissected intestines from four adults (6 months old) EK WT zebrafish males and frozen mouse fecal pellets were homogenized under reducing conditions to preserve the anaerobes. Samples were handled in a Coy anaerobic chamber and used to inoculate tubes containing brain-heart infusion (BHI) media (Thermo Scientific, OXOID) or Gifu anaerobic media (HIMEDIA), both supplemented with deoxygenated hemin and vitamin K to a final concentration of 12.5 mg/L of hemin and 2.5 mg/L of vitamin K.
Tubes were incubated in a sealed anaerobic jar with a Gas-Pak (Becton and Dickinson) to maintain anaerobic conditions at 28°C for 24 hours. Samples were then acidified to a pH below 3 with HCl, pelleted by centrifugation, and the supernatant was harvested. Filtered supernatant was stored at −80°C until quantification with methods identical to those listed above in Section 2.2.
Drug treatments
Embryos were treated with 30 mM sodium acetate (Sigma; S2889), 30 mM sodium butyrate (Sigma; B5887), 30 mM sodium propionate (Sigma; P1880), 50 µg/mL dexamethasone (Sigma; D4902), or 100 mM 6-aminocaproic acid (Sigma; A2504). Drug stocks were dissolved in DMSO or PBS and added by pipetting directly into E3 media containing zebrafish embryos.
Tail wounding experiment
Caudal fin amputation was performed on 5 dpf embryos unless otherwise indicated. Zebrafish embryos were anesthetized with tricaine. Embryos were cut posterior to the notochord using a sterile scalpel. Wounded embryos were then recovered to fresh E3 and kept at 28ºC.
Imaging
Live zebrafish embryos were anesthetized using tricaine, mounted on 3% methylcellulose (Sigma, M0512), and imaged using a Leica M205FA. ImageJ software was used to quantify the fluorescent pixel count within 100 µm of the wound site.
Additional high resolution and time-lapse microscopy was carried out on anesthetized embryos embedded in 1% low melt agarose in a 96-well plate with a Leica SP8 confocal microscope or Deltavision Elite microscope.
Neutrophil tracking
Time-lapse images were processed and analyzed using ImageJ. Neutrophils were tracked using the Trackmate plugin in ImageJ software and further quantified using Chemokine and Migration tool software (Ibidi).
Germ-free derivation and microdissection of embryos
Germ-free zebrafish were created and maintained as previously described.Citation24 The gut and body of 5 dpf embryos were separated using a 25-gauge needle and added to Trizol LS (Invitrogen; 10296010) for RNA extraction.
RNA extraction, cDNA synthesis and quantitative PCR (qPCR)
10–20 zebrafish embryos were pooled and lysed using a 25-gauge needle in Trizol LS for RNA extraction. cDNA was synthesized using a High-capacity reverse transcription kit (ThermoFisher Scientific, 4368814). qPCR was carried out using Power UP SYBR green master mix (ThermoFisher Scientific, 4385610) on a CFX96 Real-Time system (BioRad). Primer pairs (5`- 3`); 18s TCGCTAGTTGGCATCGTTTATG and CGGAGGTTCGAAGACGATCA; hcar1 CATCGTCATCTACTGCTCCAC and GCTAACACAAACCGCACA.
gRNA synthesis and CRISPR injections
gRNA templates for hcar1-2 (5`- 3`): Target 1 TAATACGACTCACTATAGGTACCGGCGGCTCGATTGGGTTTTAGAGCTAGAAATAGC, Target 2 TAATACGACTCACTATAGGAGCAACTCTCGCTTCACTGTTTTAGAGCTAGAAATAGC, Target 3 TAATACGACTCACTATAGGGATTCGAGAGATGTTACTGTTTTAGAGCTAGAAATAGC. gRNA was synthesized as previously described.Citation25
A 1:1 solution of gRNA and 500 µg/mL of Cas9 nuclease V3 (Integrated DNA Technology) was prepared with phenol red dye (Sigma, P0290). Freshly laid eggs were collected from breeding tanks and the solution was injected in the yolk sac of the egg before the emergence of the first cell with a FemtoJet 4i (Eppendorf).
Histone acetylation detection
Protein lysates were prepared from 6 dpf embryos as described previously.Citation26 Protein lysates of equal concentration were separated on SDS-PAGE, transferred onto PVDF membrane and probed with anti-rabbit acetylated histone 3 at lysine 9 (H3K9; Cell Signaling), anti-rabbit acetylated histone 4 at lysine 5 (H4K5; Cell Signaling), and anti-mouse beta-actin (Sigma).
Statistics
All statistical analyses (t-tests and ANOVA where appropriate) were performed using GraphPad Prism8. Outliers were removed using ROUT, with Q = 1%. All data are representative of at least two biological replicates.
Results
Zebrafish gut commensals are capable of producing SCFA ex vivo
We initially tried to detect SCFAs in whole intestines and their contents dissected from conventionally reared adult zebrafish using gas chromatography but levels of acetate, butyrate, or propionate were below our limit of detection of 0.00132 mmol SCFA per g of tissue.
We next sought to determine if the conventional zebrafish gut microbiota had the capacity to produce SCFAs using ex vivo culture on two rich media, BHI and Gifu. We found that microbial communities cultured from conventionally reared adult zebrafish intestines were able to synthesize acetate under both aerobic and anaerobic conditions (). Butyrate and propionate were only detected under anaerobic conditions. The highest concentrations of SCFA were detected under anaerobic conditions in BHI where acetate, propionate, and butyrate were present in a roughly 90:5:5 ratio.
Figure 1. In vitro synthesis of SCFA by zebrafish gut microbiota. Concentrations of short-chain fatty acids (SCFA) synthesized by conventional microbiota harvested from adult zebrafish intestines. SCFA content of nutrient media used to culture microbes is provided under “Negative”. Asterisks indicate measurements within the range of standards. Error bars are shown as mean ± SE, n = 2. Bars without an asterisk indicate concentrations that were outside the standard range but were detectable.
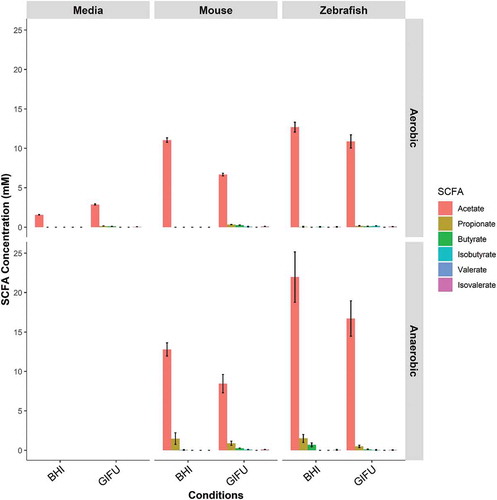
Butyrate reduces the recruitment of zebrafish neutrophils to a wound
We first observed the effect of SCFAs (acetate, butyrate, and propionate) on neutrophil migration following a tail wound injury using Tg(lyzC:DsRed)nz50 and Tg(lyzC:GFP)nz117 transgenic zebrafish lines where neutrophils are fluorescently labeled (). We observed a significant reduction in the number of recruited neutrophils at 6 hours post wounding (hpw) in embryos exposed to butyrate by immersion (). There were no changes seen with acetate or propionate, but dexamethasone, a corticosteroid anti-inflammatory used as a positive control, reduced neutrophil recruitment as expected.
Figure 2. Butyrate reduces the recruitment of zebrafish neutrophils to a wound. (a) Cartoon describing the standard cut site transecting the dorsal aorta and cardinal vein of a 5 dpf zebrafish embryo, and the fin cut site used for live imaging studies. (b) Neutrophil counts at 6 hpw. Each dot represents a single embryo. Embryos were immersed in 30 mM SCFAs or 50 µg/mL dexamethasone. (c) Velocity of wound-recruited neutrophils calculated from live imaging studies. Each dot represents the average of 10 neutrophils from a single embryo. (C) Meandering index (Total distance/Euclidean distance) of wound-recruited neutrophils calculated from live imaging studies. Each dot represents the average of 10 neutrophils from a single embryo. (d) Neutrophil count at 6 hpw in 2 dpf zebrafish.
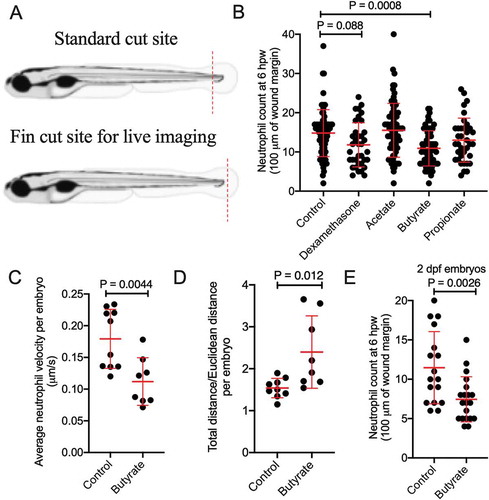
We next assessed the quality of neutrophil recruitment by intravital imaging. We observed reduced neutrophil velocity () and increased meandering index (total distance traveled/Euclidean distance) in butyrate-treated embryos ().
We next sought to determine if butyrate sensitivity is dependent on intestinal maturity by repeating the tail wound experiment using 2 dpf embryos, a developmental stage prior to significant intestinal morphogenesis,Citation27 and found neutrophil recruitment was overall reduced compared to 5 dpf, but still further inhibited by butyrate immersion ().
Butyrate reduces the proportion of tnfa positive macrophages at the wound site
Next, we examined the effect of SCFAs on macrophage recruitment and polarization following wounding. Tg(mfap4:tdTomato)xt12 transgenic zebrafish were used to visualize macrophage numbers.Citation28 Relative to control embryos, macrophage recruitment was reduced by butyrate and increased by propionate treatment at 6 hpw (). Consistent with a lack of effect on neutrophil recruitment, acetate treatment did not affect the number of recruited macrophages, and as the positive control, anti-inflammatory dexamethasone significantly reduced macrophage recruitment. These changes were maintained at 24 hpw when inflammation is in the resolution phase ().
Figure 3. Butyrate reduces macrophage recruitment to the wound site and pro-inflammatory differentiation. (a) Macrophage fluorescent area at 6 hpw. (b) Macrophage fluorescent area at 24 hpw. (c) Total tnfa promotor fluorescent area at the wound site after 24 hpw. (d) Representative images of double transgenic red macrophage, green tnfa promoter activity embryos tail wounds at 24 hpw. Scale bar represents 100 µm. (D) Quantification of wound site tnfa expressing macrophages at 24 hpw.
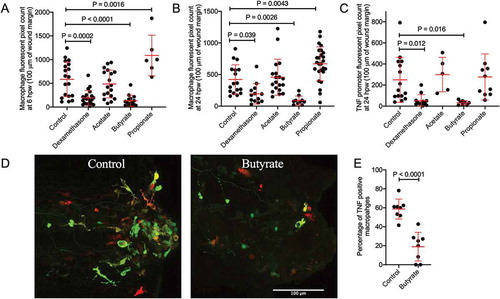
We next used the TgBAC(tnfa:gfp)pd1026 line to monitor inflammatory gene expression.Citation29 As expected, since macrophages are the primary producers of tnfa in zebrafish embryos, total tnfa promotor fluorescence area at 24 hpw reflected the trend of macrophages recruitment in each treatment condition with butyrate, but not acetate or propionate, reducing tnfa promoter expression (). We next crossed the TgBAC(tnfa:gfp)pd1026 and Tg(mfap4:tdTomato)xt12 lines to monitor macrophage inflammatory polarization as defined by inflammatory tnfa promotor expression ().Citation30 Butyrate treatment reduced the percentage of TgBAC(tnfa:gfp)pd1026 positive macrophages ().
Butyrate does not have toxic effects as measured by hemostatic indices
Butyrate has been previously shown to reduce the proliferation of zebrafish intestinal epithelial cells.Citation9 This raises the possibility that inhibition of leukocyte recruitment by butyrate immersion was due to toxicity. Changes to zebrafish hemostasis have been observed in models of toxicity and inflammation.Citation31,Citation32
We used Tg(fabp10a:fgb-EGFP)mi4001, where fibrin clots are visualized by GFP deposition, and Tg(−6.0itga2b:eGFP)la2, where thrombocytes are GFP-labeled, transgenic zebrafish lines to monitor hemostasis following transection of the dorsal aorta and posterior cardinal vein.Citation33,Citation34 We stabilized clots with aminocaproic acid as a positive control.Citation35 Fibrinogen accumulation in Tg(fabp10a:fgb-EGFP)mi4001 embryos was unchanged at the wound site in response to butyrate treatment; however, we noted that propionate treatment caused increased fibrinogen accumulation (Supplementary Figure 1A). No changes were observed in thrombocytes accumulation in the Tg(−6.0itga2b:eGFP)la2 line following any of the SCFA treatments (Supplementary Figure 1B).
Characterization of the zebrafish hydrocarboxylic acid receptor 1
Hydrocarboxylic acid receptor 1 (HCAR1) is an important receptor for butyrate in mammals.Citation20 We identified a conserved region of zebrafish chromosome 10, human chromosome 12, and mouse chromosome 5 containing density regulated re-initiation and release factor (denr), coiled-coil domain-containing 62 (ccdc62), huntingtin interacting protein 1 related a (hip1ra) loci and the single exon hcar family (). Two copies of the putative zebrafish hcar1 (with 93% identity and lacking sufficient divergence to differentiate by PCR) were identified as annotated by the single-entry NM_001163295.1 (Danio rerio hydroxycarboxylic acid receptor 1–2 (hcar1-2), mRNA) suggesting the possibility of a tandem duplication event (Supplementary File). Kuei et al. previously annotated what we refer as hcar1a as gpr81-2 and hcar1b as gpr81-1.Citation36 The predicted 933/936 bp transcript of hcar1a/hcar1b is expected to give rise to a 310/311 amino acid protein with 89% amino acid identity. The Hcar1a/Hcar1b hypothetical proteins contain seven predicted transmembrane helix domains characteristic of a GPCR and an E-value of 7.05−157/3.21−166 for the HCAR subfamily.Citation37 The predicted zebrafish proteins have approximately 43% identity to the human HCAR1, HCAR2, and HCAR3; and mouse HCAR1 and HCAR2 proteins.
Figure 4. Characterization of zebrafish hydrocarboxylic acid receptor 1 and responsiveness to butyrate. (a) Synteny diagram illustrating HCAR1 in a conserved region of human chromosome 12, mouse chromosome 5, and zebrafish chromosome 10. (b) Quantification of hcar1 expression in dissected gut and body of zebrafish embryos. Each dot represents a biological replicate of at least 10 embryos. (c) Quantification of hcar1 expression in 5 dpf embryos injected with hcar1-targeting Crispr-Cas9 complexes. Each dot represents a biological replicate of at least 10 embryos. (d) Morphology of the control and crispant embryos. Scale bar represents 100 µm. (e) Quantification of neutrophil area at 6 hpw in control and crispant embryos exposed to butyrate by immersion. (E) Quantification of macrophage area at 6 hpw in control and crispant embryos exposed to butyrate at 6 hpw. (f) Total tnfa promotor-driven fluorescent area at the wound site at 24 hpw.
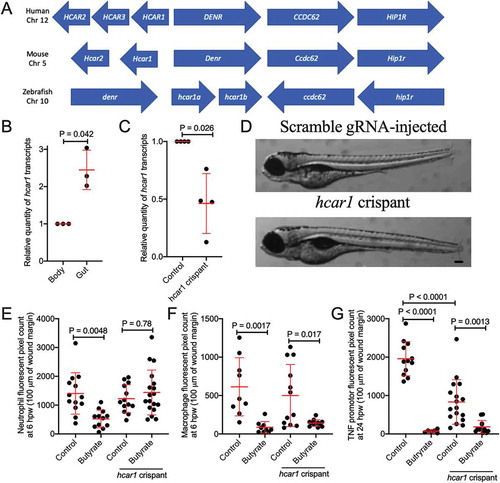
Mammalian HCAR1 is expressed by and has anti-inflammatory signaling effects in intestinal epithelial cells and leukocytes.Citation20,Citation38 Compared to other immune cells, hcar1 expression is enriched in zebrafish neutrophils.Citation39,Citation40 We sought to characterize expression of hcar1 in the intestines of zebrafish embryos using microdissection of 5 dpf embryos. We found increased hcar1 expression in dissected guts compared to the rest of the embryo by RT-qPCR analysis (). Interestingly, there were no significant changes observed with the absence of microbial colonization in germ-free embryos (Supplementary Figure 2).
The anti-inflammatory effects of butyrate on neutrophils, but not macrophages, are dependent on Hcar1
To determine if butyrate acts through the Hcar1 receptor, we next used CRISPR/Cas9 technology to knockdown hcar1 expression in zebrafish embryos. We utilized three target sites in hcar1a, two of which had strong homology to sequences in hcar1b (Supplementary File). We confirmed ~50% transcript depletion by RT-qPCR for a shared hcar1a/hcar1b sequence (). Embryo development was morphologically normal compared to embryos injected with control scrambled guide RNA-Cas9 complex ().
Knockdown of hcar1 abrogated the effect of butyrate treatment on neutrophil recruitment to tail wound injury (). As expected from the lack of expression on macrophages, hcar1 knockdown did not affect the reduced macrophage recruitment () and tnfa promotor expression () induced by butyrate immersion.
Zebrafish histone deacetylases are sensitive to exogenous butyrate.Citation41 This inhibitory effect on histone deacetylases is critical to the anti-inflammatory effects of butyrate on mammalian macrophages.Citation42 We observed that the increased acetylation of H3K9 and H4K5 histones in butyrate-treated embryos was not sensitive to hcar1 knockdown (Supplementary Figure 3).
Discussion
This study shows for the first time that commensal microbiota residing in the zebrafish intestine are capable of producing SCFAs. Experimentally, we demonstrate that the effects, and sensing, of butyrate are conserved between zebrafish and mammals. Out of the three main SCFAs, only the anti-inflammatory effect of butyrate was found to be conserved in zebrafish embryos. We applied the commonly used tail wounding model to demonstrate an anti-inflammatory effect of butyrate on zebrafish neutrophils and macrophages. Using Crispr-Cas9 targeted mutagenesis, we also identified conserved butyrate responsiveness of the zebrafish Hcar1 receptor.
The anti-inflammatory effects of butyrate have been established in numerous in vivo and in vitro studies of mammalian hosts but not in fish.Citation43 Our zebrafish tail wound model demonstrates conservation of this property in a bony fish. Bony fish diverge from mammals approximate 420 million years ago suggesting that the sensing of microbially derived SCFAs has been conserved from a common ancestor.
Our finding that immune cells are responsive to butyrate even before intestinal lumen formation in early embryonic development is surprising as 2 dpf embryos are usually contained within relatively impervious chorions that prevent microbial colonization of the embryo. This suggests that the ability to sense xenobiotic SCFAs is programmed alongside the ability to sense more traditional microbially associated molecular patterns via pattern recognition molecules.
The HCAR1/GPR81 butyrate receptor is expressed by many mammalian innate immune cells.Citation20,Citation38 Expression in zebrafish is strongest in granulocytes.Citation39,Citation40 Our knockdown experiments further demonstrate Hcar1 is necessary for the butyrate sensitivity of neutrophils but not macrophages. Thus, the butyrate-Hcar1signaling axis that influences neutrophil behavior is evolutionarily ancient.
Human macrophages in the presence of butyrate have been shown to differentiate into an M2 phenotype which exhibits anti-microbial and tissue reparative properties.Citation44,Citation45 These effects are independent of HCAR1 signal transduction and are believed to be an effect of butyrate acting as a histone deacetylase inhibitor.Citation42,Citation46 SCFAs can permeate cell membranes through passive diffusion or through specific transporters such as the proton-coupled monocarboxylate-transporter 1 (MCT1) and sodium-coupled monocarboxylate-transporter 1 (SMCT1).Citation47–50 Consistent with this literature, we show butyrate reduces the expression of pro-inflammatory tnfa by zebrafish macrophages and increases histone acetylation independent of Hcar1 expression. Our data suggest the HCAR1-independent immunosuppressive actions of butyrate may be conserved across vertebrate evolution.
Our data demonstrate that, under nutrient-rich in vitro conditions, gut commensal microbiota from adult zebrafish are capable of synthesizing the three most important SCFAs: acetate, propionate, and butyrate. However, the ratio of acetate, propionate, and butyrate produced under anaerobic conditions in BHI media (90:5:5) differed from the ratio typically observed in mammalian colons (60:20:20).Citation1 This may be due to the differing bacterial communities found in zebrafish and mammalian intestines. The most abundant bacterial phyla in the adult zebrafish intestine are Proteobacteria and Fusobacteria, whereas mouse and human intestines are dominated by members of phyla Bacteroidetes and Firmicutes.Citation51 Considering the SCFA production we observed in vitro, our inability to detect SCFA in vivo was surprising. We anticipate this could be due to rapid host or microbial metabolism of SCFA produced within the zebrafish gut, or the composition of the diet fed to the zebrafish tested in this experiment. Zebrafish are omnivores and were fed protein-rich diets in the Duke aquaculture facility. A diet with more SCFA substrates such as carbohydrates and fiber may yield detectable SCFA production in situ.
Interestingly, we observed increased macrophage and fibrinogen clot accumulation at the wound site following propionate treatment, indicative of a pro-inflammatory effect. Although this is at odds with anti-inflammatory effects of propionate in mammals,Citation52–54 it is consistent with evidence of an immunostimulatory effect of propionate in teleosts.Citation55,Citation56
Overall this manuscript provides further evidence of conserved mechanisms of host–microbe interaction within vertebrates. We present evidence that immunological sensitivity to butyrate is conserved across vertebrates. Furthermore, there is conservation of the molecular machinery that senses butyrate even down to the responsiveness of individual leukocyte lineages.
Disclosure of Potential Conflicts of Interest
No potential conflicts of interest were disclosed.
Supplemental Material
Download Zip (773 KB)Acknowledgments
The authors acknowledge the facilities and the scientific and technical assistance of the BioImaging Facility and Sydney Cytometry at Centenary Institute.
Supplementary material
Supplemental data for this article can be accessed on the publisher’s website.
Additional information
Funding
References
- den Besten G, van Eunen K, Groen AK, Venema K, Reijngoud DJ, Bakker BM. The role of short-chain fatty acids in the interplay between diet, gut microbiota, and host energy metabolism. J Lipid Res. 2013;54(9):2325–2340. doi:10.1194/jlr.R036012.
- Tan J, McKenzie C, Potamitis M, Thorburn AN, Mackay CR, Macia L. The role of short-chain fatty acids in health and disease. Adv Immunol. 2014;121:91–119.
- Tedelind S, Westberg F, Kjerrulf M, Vidal A. Anti-inflammatory properties of the short-chain fatty acids acetate and propionate: a study with relevance to inflammatory bowel disease. World J Gastroenterol. 2007;13(20):2826–2832. doi:10.3748/wjg.v13.i20.2826.
- Zheng N, Gao Y, Zhu W, Meng D, Walker WA. Short chain fatty acids produced by colonizing intestinal commensal bacterial interaction with expressed breast milk are anti-inflammatory in human immature enterocytes. PLoS One. 2020;15(2):e0229283. doi:10.1371/journal.pone.0229283.
- Baxter NT, Schmidt AW, Venkataraman A, Kim KS, Waldron C, Schmidt TM. Dynamics of human gut microbiota and short-chain fatty acids in response to dietary interventions with three fermentable fibers. mBio. 2019;10(1):e02566-18.
- Matty MA, Oehlers SH, Tobin DM. Live imaging of host-pathogen interactions in zebrafish larvae. Methods Mol Biol. 2016;1451:207–223.
- Oehlers SH, Flores MV, Chen T, Hall CJ, Crosier KE, Crosier PS. Topographical distribution of antimicrobial genes in the zebrafish intestine. Dev Comp Immunol. 2011;35:385–391.
- Oehlers SH, Flores MV, Hall CJ, Swift S, Crosier KE, Crosier PS. The inflammatory bowel disease (IBD) susceptibility genes NOD1 and NOD2 have conserved anti-bacterial roles in zebrafish. Dis Model Mech. 2011;4(6):832–841. doi:10.1242/dmm.006122.
- Kaiko GE, Ryu SH, Koues OI, Collins PL, Solnica-Krezel L, Pearce EJ, Oltz EM, Stappenbeck TS. The colonic crypt protects stem cells from microbiota-derived metabolites. Cell. 2016;165:1708–1720.
- Gaulke CA, Beaver LM, Armour CR, Humphreys IR, Barton CL, Tanguay RL, Ho E, Sharpton TJ. An integrated gene catalog of the zebrafish gut microbiome reveals significant homology with mammalian microbiomes. bioRxiv. 2020 Jun 15. 153924.
- Romano N, Simon W, Ebrahimi M, Fadel AHI, Chong CM, Kamarudin MS. Dietary sodium citrate improved oxidative stability in red hybrid tilapia (Oreochromis sp.) but reduced growth, health status, intestinal short chain fatty acids and induced liver damage. Aquaculture. 2016;458:170–176. doi:10.1016/j.aquaculture.2016.03.014.
- Titus E, Ahearn GA. Short-chain fatty acid transport in the intestine of a herbivorous teleost. J Exp Biol. 1988;135:77–94.
- Mountfort DO, Campbell J, Clements KD. Hindgut fermentation in three species of marine herbivorous fish. Appl Environ Microbiol. 2002;68(3):1374–1380. doi:10.1128/AEM.68.3.1374-1380.2002.
- Hao YT, Wu SG, Jakovlić I, Zou H, Li WX, Wang GT. Impacts of diet on hindgut microbiota and short-chain fatty acids in grass carp (Ctenopharyngodon idellus). Aquaculture Research. 2017;48(11):5595–5605. doi:10.1111/are.13381.
- Ebrahimi M, Daeman NH, Chong CM, Karami A, Kumar V, Hoseinifar SH, Romano N. Comparing the effects of different dietary organic acids on the growth, intestinal short-chain fatty acids, and liver histopathology of red hybrid tilapia (Oreochromis sp.) and potential use of these as preservatives. Fish Physiol Biochem. 2017;43(4):1195–1207. doi:10.1007/s10695-017-0365-0.
- Oehlers SH, Flores MV, Hall CJ, Wang L, Ko DC, Crosier KE, Crosier PS. A whole animal chemical screen approach to identify modifiers of intestinal neutrophilic inflammation. FEBS J. 2017;284:402–413.
- Oehlers SH, Flores MV, Hall CJ, Crosier KE, Crosier PS. Retinoic acid suppresses intestinal mucus production and exacerbates experimental enterocolitis. Dis Model Mech. 2012;5(4):457–467. doi:10.1242/dmm.009365.
- Koch BEV, Yang S, Lamers G, Stougaard J, Spaink HP. Intestinal microbiome adjusts the innate immune setpoint during colonization through negative regulation of MyD88. Nat Commun. 2018;9(1):4099. doi:10.1038/s41467-018-06658-4.
- Cheesman SE, Neal JT, Mittge E, Seredick BM, Guillemin K. Epithelial cell proliferation in the developing zebrafish intestine is regulated by the Wnt pathway and microbial signaling via Myd88. Proc Natl Acad Sci U S A. 2011;108(Suppl 1):4570–4577. doi:10.1073/pnas.1000072107.
- Ranganathan P, Shanmugam A, Swafford D, Suryawanshi A, Bhattacharjee P, Hussein MS, Koni PA, Prasad PD, Kurago ZB, Thangaraju M, et al. GPR81, a cell-surface receptor for lactate, regulates intestinal homeostasis and protects mice from experimental colitis. J Immunol. 2018;200:1781–1789.
- Arpaia N, Campbell C, Fan X, Dikiy S, van der Veeken J, deRoos P, Liu H, Cross JR, Pfeffer K, Coffer PJ, et al. Metabolites produced by commensal bacteria promote peripheral regulatory T-cell generation. Nature. 2013;504(7480):451–455. doi:10.1038/nature12726.
- Furusawa Y, Obata Y, Fukuda S, Endo TA, Nakato G, Takahashi D, Nakanishi Y, Uetake C, Kato K, Kato T, et al. Commensal microbe-derived butyrate induces the differentiation of colonic regulatory T cells. Nature. 2013;504(7480):446–450. doi:10.1038/nature12721.
- Murdoch CC, Espenschied ST, Matty MA, Mueller O, Tobin DM, Rawls JF. Intestinal Serum amyloid A suppresses systemic neutrophil activation and bactericidal activity in response to microbiota colonization. PLoS Pathog. 2019;15(3):e1007381. doi:10.1371/journal.ppat.1007381.
- Melancon E, Gomez De La Torre Canny S, Sichel S, Kelly M, Wiles TJ, Rawls JF, Eisen JS, Guillemin K. Best practices for germ-free derivation and gnotobiotic zebrafish husbandry. Methods Cell Biol. 2017;138:61–100.
- Wu RS, Lam II, Clay H, Duong DN, Deo RC, Coughlin SR. A rapid method for directed gene knockout for screening in G0 zebrafish. Dev Cell. 2018;46(1):112–25 e4. doi:10.1016/j.devcel.2018.06.003.
- Watchon M, Yuan KC, Mackovski N, Svahn AJ, Cole NJ, Goldsbury C, Rinkwitz S, Becker TS, Nicholson GA, Laird AS, et al. Calpain inhibition is protective in machado-joseph disease zebrafish due to induction of autophagy. J Neurosci. 2017;37(32):7782–7794. doi:10.1523/JNEUROSCI.1142-17.2017.
- Ng AN, de Jong-curtain TA, Mawdsley DJ, White SJ, Shin J, Appel B, Dong PDS, Stainier DYR, Heath JK. Formation of the digestive system in zebrafish: III. Intestinal epithelium morphogenesis. Dev Biol. 2005;286(1):114–135. doi:10.1016/j.ydbio.2005.07.013.
- Walton EM, Cronan MR, Beerman RW, Tobin DM. The macrophage-specific promoter mfap4 allows live, long-term analysis of macrophage behavior during mycobacterial infection in zebrafish. PLoS ONE. 2015;10:e0138949.
- Marjoram L, Alvers A, Deerhake ME, Bagwell J, Mankiewicz J, Cocchiaro JL, Beerman RW, Willer J, Sumigray KD, Katsanis N, et al. Epigenetic control of intestinal barrier function and inflammation in zebrafish. Proc Natl Acad Sci U S A. 2015;112(9):2770–2775. doi:10.1073/pnas.1424089112.
- Nguyen-Chi M, Laplace-Builhe B, Travnickova J, Luz-Crawford P, Tejedor G, Phan QT, Duroux-Richard I, Levraud J-P, Kissa K, Lutfalla G, et al. Identification of polarized macrophage subsets in zebrafish. Elife. 2015;4:e07288. doi:10.7554/eLife.07288.
- McLeish JA, Chico TJ, Taylor HB, Tucker C, Donaldson K, Brown SB. Skin exposure to micro- and nano-particles can cause haemostasis in zebrafish larvae. Thromb Haemost. 2010;103(04):797–807. doi:10.1160/TH09-06-0413.
- Hortle E, Johnson KE, Johansen MD, Nguyen T, Shavit JA, Britton WJ, Tobin DM, Oehlers SH. Thrombocyte inhibition restores protective immunity to mycobacterial infection in zebrafish. J Infect Dis. 2019;220:524–534.
- Vo AH, Swaroop A, Liu Y, Norris ZG, Shavit JA. Loss of fibrinogen in zebrafish results in symptoms consistent with human hypofibrinogenemia. PLoS ONE. 2013;8(9):e74682. doi:10.1371/journal.pone.0074682.
- Lin HF, Traver D, Zhu H, Dooley K, Paw BH, Zon LI, Handin RI. Analysis of thrombocyte development in CD41-GFP transgenic zebrafish. Blood. 2005;106(12):3803–3810. doi:10.1182/blood-2005-01-0179.
- Liu Y, Kretz CA, Maeder ML, Richter CE, Tsao P, Vo AH, Huarng MC, Rode T, Hu Z, Mehra R, et al. Targeted mutagenesis of zebrafish antithrombin III triggers disseminated intravascular coagulation and thrombosis, revealing insight into function. Blood. 2014;124(1):142–150. doi:10.1182/blood-2014-03-561027.
- Kuei C, Yu J, Zhu J, Wu J, Zhang L, Shih A, Mirzadegan T, Lovenberg T, Liu C. Study of GPR81, the lactate receptor, from distant species identifies residues and motifs critical for GPR81 functions. Mol Pharmacol. 2011;80(5):848–858. doi:10.1124/mol.111.074500.
- Marchler-Bauer A, Bo Y, Han L, He J, Lanczycki CJ, Lu S, Chitsaz F, Derbyshire MK, Geer RC, Gonzales NR, et al. CDD/SPARCLE: functional classification of proteins via subfamily domain architectures. Nucleic Acids Res. 2017;45(D1):D200–D3. doi:10.1093/nar/gkw1129.
- Hoque R, Farooq A, Ghani A, Gorelick F, Mehal WZ. Lactate reduces liver and pancreatic injury in Toll-like receptor- and inflammasome-mediated inflammation via GPR81-mediated suppression of innate immunity. Gastroenterology. 2014;146(7):1763–1774. doi:10.1053/j.gastro.2014.03.014.
- Rougeot J, Torraca V, Zakrzewska A, Kanwal Z, Jansen HJ, Sommer F, Spaink HP, Meijer AH. RNAseq profiling of leukocyte populations in zebrafish larvae reveals a cxcl11 chemokine gene as a marker of macrophage polarization during mycobacterial infection. Frontiers in Immunology. 2019;10:832. doi:10.3389/fimmu.2019.00832.
- Athanasiadis EI, Botthof JG, Andres H, Ferreira L, Lio P, Cvejic A. Single-cell RNA-sequencing uncovers transcriptional states and fate decisions in haematopoiesis. Nat Commun. 2017;8(1):2045. doi:10.1038/s41467-017-02305-6.
- Martin CC, McGowan R. Genotype-specific modifiers of transgene methylation and expression in the zebrafish, Danio rerio. Genet Res. 1995;65(1):21–28. doi:10.1017/S0016672300032973.
- Chang PV, Hao L, Offermanns S, Medzhitov R. The microbial metabolite butyrate regulates intestinal macrophage function via histone deacetylase inhibition. Proc Natl Acad Sci U S A. 2014;111:2247–2252.
- Inan MS, Rasoulpour RJ, Yin L, Hubbard AK, Rosenberg DW, Giardina C. The luminal short-chain fatty acid butyrate modulates NF-kappaB activity in a human colonic epithelial cell line. Gastroenterology. 2000;118(4):724–734. doi:10.1016/S0016-5085(00)70142-9.
- Ji J, Shu D, Zheng M, Wang J, Luo C, Wang Y, Guo F, Zou X, Lv X, Li Y, et al. Microbial metabolite butyrate facilitates M2 macrophage polarization and function. Sci Rep. 2016;6(1):24838. doi:10.1038/srep24838.
- Schulthess J, Pandey S, Capitani M, Rue-Albrecht KC, Arnold I, Franchini F, Chomka A, Ilott NE, Johnston DGW, Pires E, et al. The short chain fatty acid butyrate imprints an antimicrobial program in macrophages. Immunity. 2019;50(2):432–45 e7. doi:10.1016/j.immuni.2018.12.018.
- Davie JR. Inhibition of histone deacetylase activity by butyrate. J Nutr. 2003;133(7):2485S–93S. doi:10.1093/jn/133.7.2485S.
- Miyauchi S, Gopal E, Fei YJ, Ganapathy V. Functional identification of SLC5A8, a tumor suppressor down-regulated in colon cancer, as a Na+-coupled transporter for short-chain fatty acids. J Biol Chem. 2004;279(14):13293–13296. doi:10.1074/jbc.C400059200.
- Ritzhaupt A, Wood IS, Ellis A, Hosie KB, Shirazi-Beechey SP. Identification and characterization of a monocarboxylate transporter (MCT1) in pig and human colon: its potential to transport L-lactate as well as butyrate. J Physiol. 1998;513:719–732.
- Borthakur A, Priyamvada S, Kumar A, Natarajan AA, Gill RK, Alrefai WA, Dudeja PK. A novel nutrient sensing mechanism underlies substrate-induced regulation of monocarboxylate transporter-1. Am J Physiol Gastrointest Liver Physiol. 2012;303(10):G1126–33. doi:10.1152/ajpgi.00308.2012.
- Alarcon P, Manosalva C, Conejeros I, Carretta MD, Munoz-Caro T, Silva LMR, Taubert A, Hermosilla C, Hidalgo MA, Burgos RA. d(-) lactic acid-induced adhesion of bovine neutrophils onto endothelial cells is dependent on neutrophils extracellular traps formation and CD11b expression. Front Immunol. 2017;8:975.
- Rawls JF, Mahowald MA, Ley RE, Gordon JI. Reciprocal gut microbiota transplants from zebrafish and mice to germ-free recipients reveal host habitat selection. Cell. 2006;127:423–433.
- Provost KA, Smith M, Miller-Larsson A, Gudleski GD, Sethi S. Bacterial regulation of macrophage bacterial recognition receptors in COPD are differentially modified by budesonide and fluticasone propionate. PLoS One. 2019;14(1):e0207675. doi:10.1371/journal.pone.0207675.
- Ciarlo E, Heinonen T, Herderschee J, Fenwick C, Mombelli M, Le Roy D, Roger T. Impact of the microbial derived short chain fatty acid propionate on host susceptibility to bacterial and fungal infections in vivo. Sci Rep. 2016;6:37944.
- Park JW, Kim HY, Kim MG, Jeong S, Yun CH, Han SH. Short-chain fatty acids inhibit staphylococcal lipoprotein-induced nitric oxide production in murine macrophages. Immune Netw. 2019;19:e9.
- Hoseinifar SH, Zoheiri F, Caipang CM. Dietary sodium propionate improved performance, mucosal and humoral immune responses in Caspian white fish (Rutilus frisii kutum) fry. Fish Shellfish Immunol. 2016;55:523–528. doi:10.1016/j.fsi.2016.06.027.
- Safari R, Hoseinifar SH, Kavandi M. Modulation of antioxidant defense and immune response in zebra fish (Danio rerio) using dietary sodium propionate. Fish Physiol Biochem. 2016;42(6):1733–1739. doi:10.1007/s10695-016-0253-z.