ABSTRACT
Oral Prevotella are known as anaerobic commensals on oral mucosae and in dental plaques from early life onwards, including pigmented P. melaninogenica, P. nigrescens, and P. pallens and non-pigmented Prevotella species. Many Prevotella species contribute to oral inflammatory processes, being frequent findings in dysbiotic biofilms of periodontal diseases (P. intermedia, P. nigrescens), cariotic lesions (P. denticola, Alloprevotella (formerly Prevotella) tannerae), endodontic infections (P. baroniae, P. oris, P. multisaccharivorax), and other clinically relevant oral conditions. Over the years, several novel species have been recovered from the oral cavity without knowledge of their clinical relevance. Within this wide genus, virulence properties and other characteristics like biofilm formation seemingly vary in a species- and strain-dependent manner, as shown for the P. intermedia group organisms (P. aurantiaca, P. intermedia, P. nigrescens, and P. pallens). Oral Prevotella species are identified in various non-oral infections and chronic pathological conditions. Here, we have updated the knowledge of the genus Prevotella and the role of Prevotella species as residents and infectious agents of the oral cavity, as well as their detection in non-oral infections, but also gathered information on their potential link to cancers of the head and neck, and other systemic disorders.
Introduction
Anaerobic bacteria constitute a significant part of oral microbial communities and are fundamental for oral homeostasis. The size of the core community of the mouth is estimated to be larger than those of the gut and skin [Citation1]. Among the oral microbiota, Bacteroidetes is one of the major phylae and Prevotella its largest genus [Citation2]. This genus, described by Shah and Collins in 1990 [Citation3], consists of pigmented or non-pigmented, Gram-negative, strictly anaerobic, short rod-shaped bacteria. Besides 12 former Bacteroides reclassified as Prevotella species [Citation3], a high number of Prevotella species of oral origin from humans have been described. A recent whole-genome analysis of Prevotella genomes available in public repositories demonstrated variable genome lengths (2.37–4.26 Mb) and G + C contents (36.4–56.1%) between the 25 human Prevotella species included in the analysis [Citation4], indicating a wide diversity within the genus. In addition, a closely related novel genus Alloprevotella consists of two oral species, A. tannerae (formerly Prevotella tannerae) and A. rava (formerly known as Prevotella oral taxon 302) [Citation5]. Knowledge of such a variety of species and their impact on the oral microbiota and human well-being is of concern.
Many Prevotella species are common residents on various surfaces of the mouth, often with a commensal relation to their host. One example is P. melaninogenica, a ubiquitous species in the oral cavity, where it colonizes mucosal surfaces of infants from the early months of life onwards [Citation6,Citation7]. Other early-colonizing oral Prevotella species are P. nigrescens and P. pallens [Citation8]. While the latter species of the P. intermedia group is without any specific connection to oral diseases, P. nigrescens and P. intermedia, in particular, show moderate pathogenicity, being present in periodontitis-associated subgingival complexes [Citation9]. A concept of dysbiotic biofilms, where commensals can behave as opportunistic pathogens in susceptible hosts, is useful to describe oral infection-driven inflammatory diseases like periodontitis [Citation10]. Cooperative or competitive interactions between bacteria regulate the composition of biofilms and their properties. Since Prevotella is a highly diverse genus [Citation4], some of its species could behave as true commensals but some as potential pathobionts, which trigger inflammatory mediators with harmful consequences not only in the mouth but also at extra-oral sites. For instance, proinflammatory interleukin-17 produced in response to P. nigrescens could aggravate the injury in arthritis-affected joints [Citation11].
The hematogenous spread of oral bacteria, including Prevotella, can occur after dental procedures [Citation12]. A novel perspective is the blood microbiome, where oral bacteria seem to have a significant impact [Citation13], which raises a question on their potential involvement in systemic inflammatory conditions. Due to pathological processes present in the mouth, such as periodontitis, caries, and endodontic infections among others (), many inflammatory agents produced by the host can migrate via the blood circulation to extra-oral sites [Citation14]. Indeed, translocated oral, vaginal, and intestinal Prevotella species have harmful consequences in a huge variety of clinically relevant infections at the head and neck area and the gastrointestinal, lower respiratory, and urogenital tracts, skin and soft tissues, central nervous system, and blood [Citation15]. Moreover, there is evidence that Prevotella may have a role in malignancies as well as in non-infectious chronic conditions like rheumatic diseases and neurodegenerative disorders.
Figure 1. Oral Prevotella species detected in clinical specimens from oral and non-oral diseases/infections.
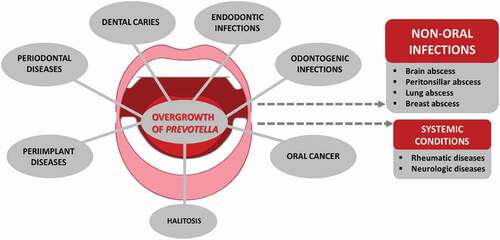
Many Prevotella organisms of oral origin seem to be important players in the gastrointestinal and respiratory tracts during health and disease [Citation16]. Prevotella constitutes a significant part of oral microbial communities; however, species-level data are still scarce due to a considerable expansion within the genus during the past two decades. In this narrative review, we focus on the presence and properties of individual species in the oral cavity, by gathering the knowledge of various Prevotella species as oral residents and disease-associated agents, as well as their potential impact on some systemic conditions.
Prevotella species in the developing anaerobic microbiota and as commensals of the mouth
While the facultative genera Streptococcus, Gemella, and Granulicatella predominate in saliva of 3–4-day-old neonates, the genus Prevotella is still rare and its relative abundance low [Citation17]. However, the anaerobic organisms of the genera Veillonella and Prevotella start their establishment in the mouth within 1–2 months of life, as shown in culture-based studies from the 90ʹs. Thorough anaerobic techniques and longitudinal study designs revealed the time of colonization and succession of anaerobes in the oral cavity, with P. melaninogenica as a common anaerobic finding on oral mucosae and in saliva of predentate infants [Citation6,Citation7]. For pioneer commensals, the clonal diversity seems to be typical, and up to seven simultaneous clones have been shown for early-colonizing anaerobes, P. melaninogenica and Fusobacterium nucleatum [Citation6,Citation18]. This wide antigenic variety and frequent turnover of strains would allow escape from the host immune response; thus, these species can persistently colonize the habitat and elicit natural immunity. In particular, the key role of F. nucleatum in successive colonization of other species in oral biofilms is of interest. Besides P. melaninogenica, the frequency of oral pigmented Prevotella organisms, including P. denticola, P. loescheii, P. nigrescens, and P. pallens, and non-pigmented Prevotella species remarkably increased during the first years of life [Citation6,Citation19]. The composition of parents’ oral microbiota with potential pathogens and frequent contacts reflect the developing microbiota of their children [Citation8,Citation20], which may affect their oral health later in life. At the clonal level, knowledge of intra-familial transmission of oral Prevotella between mother-child pairs or between spouses includes P. melaninogenica, P. pallens, P. nigrescens, and P. intermedia [Citation19,Citation21,Citation22]. Within the P. intermedia group, recoveries of similar clones of P. nigrescens and P. pallens from maternal saliva and oral samples of their young children were mainly from periodontally healthy mothers [Citation19]. A recent review [Citation23] underlined the necessity of large-scale, detailed longitudinal studies to reveal the spatial diversity and acquisition patterns of the early oral microbiota for understanding conformities of a symbiotic host–microbiota relationship. Crielaard and co-workers [Citation24] explored temporal changes in the microbiome that occur due to the natural development of the dentition along with age by analyzing the salivas of children with the deciduous, early mixed, late mixed, and permanent dentition. The genus Prevotella was among the three predominant taxa in saliva, and its relative abundance was steadily increased due to this age-driven developmental maturation [Citation24]; however, no species-level data were available. In 240 Kuwaiti children, stratified into five age groups (<6, 6–9, 10–12, 13–15, and 16–18 years of age), the salivary carriage rates of P. intermedia and P. nigrescens were clearly distinct [Citation25]. While the rates of P. intermedia remained low, varying from 2.5% in the youngest group to 6.7%, 15%, 10%, and 8.3% in the oldest group, the corresponding rates for P. nigrescens were much higher, i.e. 15%, 31.7%, 62.5%, 50%, and 46.7%. A similar trend was reported from saliva and subgingival samples collected from 119 healthy children aged 2–15 years in Japan [Citation26]. A recent study compared the bacterial composition of subgingival plaque samples from the offspring of two parent groups, including 18 parents with generalized severe (grade C) periodontitis and 18 parents without periodontal disease [Citation20]. In the core microbiome of their children aged 6–12 years, P. maculosa, P. melaninogenica, P. oris, and P. oulorum belonged to the core microbiome, determined as being present in at least 80% of the children, irrespective of the parent group. Instead, in the core microbiome of children of diseased parents, a much wider selection of Prevotella taxa was found, including P. histicola, P. nanceiensis, P. nigrescens, P. pleuritidis, P. saccharolytica, P. scopos, P. veroralis, and A. tannerae. Furthermore, Prevotella abundance differed between children of parents with and without periodontitis. The most abundant Prevotella species in children of periodontitis parents were P. shahii and P. scopos, with over 6- and 4-fold difference, respectively, compared to children of periodontally healthy parents. In addition, P. denticola, P. maculosa, and P. pleuritidis had over 3-fold difference, and P. oris, P. oulorum, P. saccharolytica, P. salivae, and P. veroralis over 2-fold difference [Citation20].
In healthy adults, the detection rates of the genus Prevotella are high in saliva and dental plaque [Citation17,Citation27]. In saliva, the richness of Prevotella diversity was especially high [Citation26]. A study looking for the intraoral distribution of bacterial species in 225 systemically healthy individuals showed P. melaninogenica in high proportions in saliva and at the dorsum and lateral sites of the tongue [Citation28]. In the oropharynx of young adults, tonsillar crypts are colonized by a variety of Prevotella species, among those P. buccae, P. dentalis, P. denticola, P. fusca, P. micans, P. oralis, P. oris, P. pallens, P. salivae, and P. veroralis, typical oral species, but also P. nanceiensis and P. pleuritidis, less known residents of the oral cavity or oropharynx [Citation29]. In the literature, the species-level data on Prevotella organisms have long been limited to P. intermedia and/or P. nigrescens due to their clinical relevance in oral pathologies, but also ignorance of commensals. However, this attitude is no longer valid, and knowledge of their prevalence and abundance is rapidly increasing.
In sequencing studies with limited numbers of participants, representing various age and ethnical backgrounds, P. melaninogenica appears among the core oral bacteriome in individuals with healthy oral tissues [Citation30,Citation31]. Less abundant core species included P. denticola, P. oris, and A. tannerae, while P. multiformis was recognized at low prevalence but relatively high abundance [Citation31]. However, also some differences have been reported; a comparison of bacterial communities in saliva of a total of 2,815 individuals showed significantly higher relative abundances of the genus Prevotella and P. melaninogenica, in particular, in orally healthy Japanese than South Korean study participants [Citation32]. A recent study compared the tongue microbiome of 10 urban and 10 rural women, aged 20–45 years, in Indonesia [Citation33]. In the urban group, Prevotella and Leptotrichia were among the most abundant genera, and P. melaninogenica, P. histicola, P. pallens, and P. salivae were the most discriminative Prevotella species between the urban and rural groups, probably connected to different environmental and lifestyle factors. When comparing bacterial communities of the buccal mucosae of 20 young healthy individuals who smoked 15–24 cigarettes daily to those of 20 non-smokers, increased abundance of several species, including P. salivae, P. melaninogenica and P. histicola, was observed in smokers [Citation34]. In studies dealing with Finnish periodontitis-free individuals, the most frequent pigmented Prevotella species were P. melaninogenica, P. intermedia/P. nigrescens and P. loescheii [Citation35], whereas P. intermedia (sensu stricto) has been a rare finding [Citation19,Citation22,Citation36]. Interestingly, P. jejuni, which is a pigment-producing species closely related to P. melaninogenica, but not originally accounted for as an oral species, proves to be present in oral specimens [Citation33,Citation37,Citation38]. Recoveries from a general population-based study, looking for PCR-based carriage rates of periodontal pathogens in saliva of 1,294 adults living in southern Finland, only 13.9% were positive for P. intermedia [Citation39], which was more frequent in women than in men and in smokers than in non-smokers. Similarly, a low detection rate of 10% for P. intermedia was reported from subgingival plaques of 504 Australian adults, mainly composed of university staff [Citation40]. With regard to other Prevotella species, such information based on large study populations is limited. In the salivary microbiome of more than 2,300 inhabitants aged ≥40 years of the town of Hisayama, a demographically representative of Japan, P. melaninogenica was recovered as a core bacterial species, and other common Prevotella species were P. denticola, P. histicola and P. salivae [Citation41]. Another study based on the same study material focused on the bacterial composition on the tongue dorsum in 506 community-dwelling elderly, accounting for half of the age group of 70–80 years living in the town [Citation42]. P. melaninogenica was the second most predominant finding, but also P. histicola and P. pallens were among the predominant findings on the tongue dorsum.
Properties of oral Prevotella organisms
Species-level differences within the P. intermedia group
Here, this specific Prevotella group was selected as an example to demonstrate the wide heterogeneity of properties of biological and medical relevance even within closely related species. With this comparison, we emphasize the importance of obtaining species-level information for a better understanding of their potential roles as commensals, pathobionts, or ‘true’ pathogens.
The P. intermedia group is composed of four phylogenetically close species, i.e. P. intermedia, P. nigrescens, P. pallens, and P. aurantiaca. Their shared biochemical characteristics are the ability to decompose tryptophane into indole, moderate saccharolytic activities, and production of pigment [Citation43–45]. All four species are residents of the oral cavity, which represents their predominant site of isolation. When Shah and Gharbia [Citation43] used DNA–DNA hybridization to propose the separation of the phenotypically identical P. intermedia and P. nigrescens into two genetically distinct species, the division was not only based on their different homology groups but also on their dissimilar virulence properties. While P. intermedia was associated with periodontal infections, P. nigrescens was found at both healthy and diseased periodontal sites and as a core species mediating fluctuations in the subgingival microbiota [Citation22,Citation43,Citation46]. P. pallens recoveries come mainly from healthy children and periodontitis-free women [Citation19] and, in addition, it seems to be a resident species on healthy esophageal mucosae [Citation47]. The newest member of the P. intermedia group, P. aurantiaca, was isolated from the periodontal pocket of a patient with periodontitis [Citation45]. However, the literature is scarce regarding its involvement in oral or systemic infections.
In relation to periodontal health, the physiological and hormonal changes during pregnancy exhibit a direct impact on periodontal tissues [Citation48]. P. intermedia and P. nigrescens can take up and utilize sex steroid hormones (estrogen and progesterone) as substitutes for vitamin K, the essential growth factor, in temperature- and concentration-dependent manners [Citation49]. The impact of estradiol on the growth characteristics, proteolytic enzyme activities, and biofilm-related pathogenicity within the P. intermedia group organisms has been examined in vitro, partially simulating the clinical conditions of pregnancy-associated gingivitis [Citation50,Citation51]. P. nigrescens, P. pallens, and P. aurantiaca increased their numbers in the planktonic phase with elevated estradiol levels, and P. intermedia, P. nigrescens, and P. pallens exhibited higher protein concentrations in the presence of estradiol, presenting the ability to form biofilms. In addition, P. intermedia enhanced polysaccharide production and coaggregation capabilities with F. nucleatum, indicating their potential inter-species differences in the pathogenesis of pregnancy-associated gingivitis [Citation50]. Estradiol affected the virulence of P. intermedia, P. nigrescens, P. pallens, and P. aurantiaca, as seen in their bacterial dipeptidyl peptidase IV (DPPIV) enzyme activity in static biofilms, in a strain- and dose-dependent manner [Citation51,Citation52]. Variations in the distribution of virulence-associated genes among Prevotella species seem to regulate their intra-oral colonization and relation to disease pathogenesis; for example, beta-lactam resistance genes significantly altered the frequency and distribution of certain strains of P. intermedia, P. nigrescens, and A. tannerae at different sites in the oral cavity [Citation53].
Virulence factors
Properties of Prevotella organisms vary due to the wide diversity of this anaerobic genus. As having a Gram-negative cell structure, lipopolysaccharide (LPS) is a potential virulence factor of Prevotella species. For instance, LPS of P. intermedia stimulates the expression of IL-8 in human gingival fibroblasts, invades the human oral epithelial cells (KB cells), impairs phagocytic and chemotactic activities in human dental stem cells, and stimulates alveolar bone resorption [Citation54–56]. P. intermedia and P. nigrescens produce mannose polysaccharide, which allows these species to participate in chronic inflammatory processes, including the modification of human leukocyte phagocytosis and invasion of host defense barriers [Citation57,Citation58].
Other virulence factors are connected to fimbriae, outer membrane vesicles, protein secretion systems (enzymes and toxins), resistance to oxidative stress, and pH modification [Citation59–61]. Indeed, P. intermedia carries a Type IX secretion system, allowing it to release its proteases, hemolysins, and other virulence factors on outer membrane proteins, thus dysregulating host cell responses [Citation62]. As regards P. intermedia and P. nigrescens, the pathways of energy metabolism, carbohydrate and lipid metabolism, and amino acid metabolism differ between disease- and health-related samples [Citation61]. Regarding surface structures, various types of P. intermedia fimbriae mediate their adherence to host erythrocytes to facilitate their agglutination and create a fimbriae-associated hemagglutinin activity essential for P. intermedia colonization in vivo [Citation63]. Host cellular adhesion is an essential part of bacterial virulence; P. intermedia and P. nigrescens demonstrated representative adhesion capabilities to invade epithelial cells, with special tendency to bind the epithelial cell lamellipodia [Citation64].
The catalytic action of proteolytic enzymes (cysteine and serine proteinases) produced by oral Prevotella species is considered a significant virulence factor, contributing to periodontal tissue destruction through the cleavage of cellular peptides, which leads to the degradation of collagenous matrix in the periodontium [Citation65–67]. Prevotella strains isolated from oral infectious lesions, e.g. purulent periodontal pockets and peri-implantitis sites, demonstrate higher proteolytic enzyme activities than resident Prevotella of healthy sites [Citation66,Citation68]. As part of host-bacterial interactions, the degradation of immunoglobulins by total proteases of P. intermedia and P. nigrescens is strongly linked to the causality of periodontal disease originated from polymicrobial infections [Citation69].
DPPIV is a serine protease of bacterial and host origin. Bacterial DPPIV is an endopeptidase that hydrolyzes the penultimate proline or alanine dipeptides from the N-terminal polypeptide chains, while the host CD26 is a transmembrane glycoprotein encoded by the DPPIV gene and is expressed on the surface of different cell types, including human gingival keratinocytes and fibroblasts [Citation70–72]. The CD26/DPPIV plays a key role in many physiological and pathological processes, such as glucose metabolism and chemokine regulation, but also acts as a tumor marker for various types of cancers [Citation73–75], and has a strong association with the severity of disease and comorbidity in patients with COVID-19 [Citation76,Citation77]. In bacteremia induced by periodontitis-associated species (Porphyromonas gingivalis, Tannerella forsythia, and P. intermedia), DPPIV shows a significant capability of modulating blood glucose levels, potentially deteriorating the health condition of diabetes, and is considered a powerful biomarker for the existence of periodontopathogens in subgingival biofilms [Citation78]. Via an indirect pathway, the expression of virulence by P. melaninogenica is significantly regulated by the proteases and cell surface proteins secreted by P. gingivalis and T. forsythia as virulence factors [Citation79].
Hemagglutinating bacteria use certain agglutinins that enable red blood cells to be clumped by serum antibodies, while the hemolytic activity of bacteria is their ability to produce hemolysins for the breakdown of red blood cells and release hemoglobin [Citation80]. Both properties are considered virulence characteristics of bacteria with fimbriae. P. intermedia and P. nigrescens have high hemagglutinating and hemolytic activities that crucially benefit their growth and persistence, as well as to synergistically support the virulence of other pigmented species, e.g. providing haem to P. gingivalis in subgingival plaque through a mutualistic proteolytic conjunctive action by P. intermedia [Citation81–83]. As a metabolic mechanism, oral Prevotella species require haem as an indispensable nutrient for their persistence and to express virulence [Citation84]. P. intermedia contributes to haem acquisition by another proteolytic enzyme, interpain A or albuminase, to degrade hemoglobin in inflamed periodontal pockets under special conditions of low redox potential and high pH [Citation83,Citation85]. Unlike P. intermedia, the lactoferrin-binding protein identified in P. nigrescens from a periodontal pocket is seemingly an active player in its iron acquisition mechanism [Citation86]. As part of the essential iron-scavenging utilization, the iron-saturated human transferrin-binding capacity of P. nigrescens allows it to survive and proliferate in deep periodontal pockets [Citation87]. Although considered a low magnitude of virulence, P. melaninogenica is another example of Prevotella species exhibiting a hemagglutinating activity and agglutinating erythrocytes [Citation88].
Prevotella in oral (dysbiotic) biofilms
In comparison with the planktonic phase of growth, bacteria living in oral biofilms are shielded against the disruptive mechanical and chemical forces (e.g. tooth brushing, salivary flow, and antimicrobials) and are protected from phagocytic immune cells and the complement system to recognize them [Citation89–92]. Thus, biofilm formation is among the significant virulence aspects of oral Prevotella species to establish a tolerable environment, optimal for their growth and proliferation [Citation91]. Various Prevotella species, including P. intermedia, P. nigrescens, and P. denticola, can take part in both sugar and protein metabolisms, allowing them to survive in both health- and disease-associated conditions, and in supra- and subgingival areas [Citation59,Citation93]. Prevotella species usually colonize the dental biofilm in micro-colonies, being visible on the top layers of the biofilm [Citation94]. Yet, there are variations in biofilm formation capabilities of Prevotella species in in vitro conditions; P. loescheii, P. oris, and P. nigrescens demonstrate better biofilm forming abilities than P. intermedia, P. melaninogenica, P. pallens, and P. oulorum [Citation95]. A dysbiotic shift in biofilms occurs with a change in the environment, for example, with an increase in dietary sugars or in inflammatory proteins, leading to common destructive diseases, caries, and periodontitis. An inflammation-induced protein-rich environment allows the proliferation of amino acid-degrading Prevotella in subgingival biofilms [Citation96]. Systemic diseases connected to a chronic inflammatory state, such as rheumatoid arthritis, induce Prevotella to enrich in the oral microbiota as well [Citation97]. Indeed, enriched Prevotella can neutralize the acidic stress by fermenting glutamate and aspartate into ammonia, allowing acid-sensitive P. gingivalis and Treponema denticola to become more dominant in subgingival biofilms [Citation98]. In addition to these indirect synergistic effects, cell-to-cell contacts with Prevotella stimulate biofilm formation: P. intermedia and F. nucleatum [Citation99] and P. oris or P. nigrescens and P. gingivalis [Citation100,Citation101]. Not only whole cells but also metabolites of Prevotella can modify cellular response against bacteria. Short-chain fatty acids of P. loescheii inhibit murine T- and B-cell proliferation and cytokine production by splenic T cells [Citation102]. In particular, volatile propionic, butyric, and isovaleric acids are able to induce DNA fragmentation and apoptosis in B cells [Citation103]. Soluble sonic extracts of P. loescheii induce a dose-dependent inhibition of human peripheral blood lymphocyte and leukemic cell line (BALL-1) proliferations [Citation104]. These findings indicate that Prevotella organisms within biofilms can regulate host residence and immune cell response, without being in need of a direct contact with the host. Although Prevotella species are considered to be aerotolerant [Citation105], coaggregation with F. nucleatum seems to be the major determinant for Prevotella to survive in aerated environments [Citation90]. Taken together, Prevotella species not only contribute to the biofilm’s structural maintenance but also benefit from the multispecies character of oral biofilms.
Host cell response to oral Prevotella
Amongst the vast number of bacteria–host cell interaction studies, only few studies include Prevotella as a test organism and/or demonstrate cellular response against Prevotella species other than P. intermedia. This species is common in periodontitis-affected gingival tissues, indicating its direct interactions with periodontal resident and immune cells [Citation106]. P. intermedia is a strong inducer of epithelial anti-microbial peptides [human beta-defensins (hBD-1, −2, −3)] and IL-8, especially when incubated with human gingival keratinocytes with a multiplicity of infection (MOI) of 1,000 [Citation107]. Such an effect was not observed when P. intermedia was incubated with a MOI of 200, meaning that the cellular response against P. intermedia requires colonization and proliferation of P. intermedia. Not only P. intermedia but also its glycoproteins, but not its LPS, can activate IL-8, granulocyte colony-stimulating factor, and granulocyte-macrophage colony-stimulating factor protein expressions of gingival keratinocytes [Citation108]. Thus, it is possible that oral Prevotella species do not only aggravate the disease extension and progression but also protect the healthy homeostasis in a species-dependent manner.
Cellular response against Prevotella is not limited to gingival keratinocytes. Indeed, P. intermedia activates COX-2-dependent PGE2 expression in periodontal ligament fibroblasts in a dose- and time-dependent manner [Citation109]. P. nigrescens LPS can activate PGE2 expression together with transforming growth factor production in bone marrow mononuclear cells and induce osteoclastogenesis [Citation110]. A similar dose-dependent cellular response was observed when mouse odontoblast-like cells and macrophages were incubated with P. intermedia LPS, which ended up elevating vascular endothelial growth factor protein expressions [Citation111]. In fact, mouse studies produced evidence that P. nigrescens can induce Th17 response via toll-like receptor-2 and IL-1 activation [Citation112]. Human dental pulp cells also react against P. intermedia LPS by producing IL-10 receptor [Citation113]. P. nigrescens LPS increases cell surface fibronectin expression of gingival fibroblasts in inflamed gingiva [Citation114]. Yet, the clinical significance of elevated cell surface fibronectin in periodontal disease pathogenesis is not well understood. Finally, P. intermedia can stimulate CD4(+) T cell subsets to produce cytokines interferon-γ and IL-2 [Citation115]. Since these cytokines are expressed by Th1 lymphocytes, it is possible that P. intermedia regulates T lymphocyte differentiation.
As seen here, the main gap in the literature is within the interactions between human cellular response and oral health-associated Prevotella commensals. Further studies are needed to demonstrate how different Prevotella species contribute to a healthy oral equilibrium.
Prevotella involvement in oral conditions of clinical relevance
Besides being a core species in various locations of the oral cavity, many members of the genus Prevotella are common findings in clinical specimens collected from oral infections (). In early studies, Bacteroides melaninogenicus subspecies intermedius was connected to plaque-induced gingival inflammation and periodontitis [Citation116,Citation117] as well as to specific types of gingival diseases, such as pregnancy gingivitis and necrotizing ulcerative gingivitis [Citation36,Citation118]. After the description of the genus Prevotella [Citation3] and separation between P. intermedia and P. nigrescens [Citation43], it was soon observed that these species differ as regards to their site specificity, surface properties, enzyme activities, and pathogenicity [Citation46,Citation119]. While the majority of P. intermedia originated from deepened periodontal pockets, only a few strains came from endodontic infections, and P. nigrescens was common at healthy gingival sites [Citation46,Citation119]. In subgingival biofilms, however, both species belong to the orange complex with a moderate association with periodontal disease [Citation9].
The recoveries of specific Prevotella species from a variety of oral diseases have been gathered in .
Table 1. Detection of specific Prevotella species (validly published) of oral origin in oral and non-oral diseases/conditions
Periodontal and peri-implant diseases
In periodontitis-free pregnant women, mainly P. nigrescens was found to be associated with pregnancy-related gingivitis [Citation36]. Interestingly, the estrogen level plays a role in the severity of inflammation at the gingival margin developed against plaque bacteria [Citation120]. During pregnancy, the symbiotic composition is interrupted by the shifts towards anaerobes, including abundant P. nigrescens, P. oris, and P. gingivalis in plaque, and Prevotella oral taxon 313 and P. melaninogenica in saliva [Citation121]. This transitional state of pregnancy-related dysbiosis usually returns to pre-gestational levels few months postpartum [Citation120,Citation121].
Chronic inflammation in gingival tissues can expose susceptible individuals to the initiation of periodontitis via alterations in subgingival bacterial communities. P. oulorum levels have been shown to be highly correlated with bleeding on probing scores during the transition from periodontal health to gingivitis [Citation122]. Prevotella species can contribute to microbial dysbiosis and inflammation-regulated tissue destruction from its initiation to maturation. P. denticola, P. multiformis, and P. oralis have been connected to the severity of periodontal inflammation, correlating with increased age [Citation123]. This kind of disrupted homeostasis may then result in the onset of disease as abundant P. denticola, P. intermedia, and P. oralis have been associated with deepening periodontal pockets [Citation122,Citation124]. Indeed, periodontitis is induced by bacterial infection, where Prevotella organisms as immunostimulatory oral bacteria can play a role as potential pathobionts or pathogens [Citation10].
Proteomic and metagenomic studies have indicated a more complex microbial diversity in periodontitis patients and elevated abundances of Prevotella species; among salivary findings are P. baroniae, P. enoeca, P. denticola, P. histicola, P. intermedia, P. melaninogenica, P. nigrescens, P. pallens, P. veroralis, A. tannerae, and A. rava, but also P. copri and P. stercorea, rather known as inhabitants of the gut [Citation125–128]. Questions may arise on how well the salivary microbiota represents the subgingival microbiota. However, the abundance of multiple subgingival plaque-related bacteria in saliva highly correlates with the progression of periodontitis [Citation125].
Non-surgical periodontal therapy significantly decreased the levels of periodontitis-associated anaerobes, including P. denticola, P. nigrescens, and A. tannerae, in subgingival biofilms [Citation129]. However, one should bear in mind that these bacteria do not fully disappear from the oral cavity; for example, P. intermedia, P. melaninogenica, P. nigrescens, and P. veroralis were detected in high abundances in saliva of periodontitis patients after 1 week, and P. intermedia and P. veroralis even after 8 weeks, of completed periodontal treatment [Citation128]. Thus, high abundances of salivary Prevotella may contribute to the recurrence of periodontitis via bacterial recolonization of subgingival sites.
Although periodontal and peri-implant microbiomes share similarities in bacterial lineages, they represent microbiologically distinct ecosystems in health or disease [Citation130,Citation131]. In non-smokers, microbial abundances are reduced in peri-implant mucositis compared to peri-implant health, and a few health-associated species disappear along with a gain of various new species [Citation131]. The species richness and consistency are similar between peri-implant mucositis and peri-implantitis. Several Prevotella taxa were identified among altered microbial communities. P. scopos, together with several unnamed taxa (HOT293, HOT299, HOT305 HOT306, and HOT315), were unique Prevotella findings during the shift from health to peri-implant mucositis. Then, P. shahii, P. multisaccharivorax, and unnamed taxa (HOT304 and HOT475) were recognized in peri-implant mucositis, Prevotella HOT526 during the shift from peri-implant mucositis to peri-implantitis, and Prevotella HOT515 and HOT820 in peri-implantitis [Citation131]. Also, P. intermedia, P. nigrescens, and P. denticola have been reported at high numbers from peri-implantitis samples [Citation132–134]. In peri-implantitis patients, deep peri-implant pockets, peri‐implant disease status, and partial edentulism were found to be associated with a high abundance of Gram‐negative anaerobic taxa, including members of the genus Prevotella [Citation135]. The local environment affects the peri-implant biofilm composition; in comparison to shallow pockets, higher levels of P. nigrescens and P. oris, together with F. nucleatum and Anaeroglobus geminatus, were detected in deep pockets around dental implants. In 18 partially dentate and implant‐rehabilitated Chinese adults suffering from both periodontitis and peri-implantitis, the levels of P. denticola, P. fusca, and P. multiformis were markedly abundant in submucosal samples, whereas P. dentalis was more abundant in subgingival samples [Citation136].
Dental caries and root canal infections
High abundance of the genus Prevotella is associated with dental caries both in adults and children [Citation137–140]. Intense and prolonged carbohydrate exposure alters the diversity of the microbial community by promoting the growth of surviving microorganisms under various acidic conditions, and the superficial parts are more acidic than the deepest sites of the caries lesion [Citation138]. At a pH range of 4.5–5.0, lactobacilli act as anchor species, Prevotella taxa being the anchor species at a mid-range pH of 5.5–6.0. While P. multisaccharivorax and P. histicola were found in more acidic layers of dentinal caries, P. oulorum and P. nigrescens together with A. tannerae were prominent in mildly acidic and neutral pH regions of the caries lesion. Despite these species-specific variations regarding the pH gradient, approximately 60% of the dentinal caries-associated bacterial taxa, including eight Prevotella species, P. baroniae, P. buccae, P. dentalis, P. intermedia, P. maculosa, P. marshii, P. oralis, and P. pleuritidis, were non-discriminatory for pH changes, and thus, belonged to the substantial core microbiota [Citation138]. This indicates that the presence of Prevotella in deep dentinal caries lesions reflects a suitable ecological niche rather than a contribution to caries progression [Citation137].
According to a US study on dental caries experienced during early childhood, the diversity of caries-free sites and enamel caries lesions proved to be rather similar, whereas more taxa and enzyme activities were observed in dentin caries lesions, where Scardovia wiggsiae was most abundant, and also P. denticola, P. oris, and P. oulorum were among the most abundant species [Citation141]. In an Irish study on the microbiome of severe early childhood caries (EEC) [Citation140], higher relative abundance of P. histicola was detected both in deep dentinal caries lesions and in saliva from the same caries-active children, whereas P. melaninogenica and P. salivae dominated only in saliva. In a Chinese study, salivary levels of P. denticola and Streptococcus mutans were significantly higher in children with severe EEC than in caries-free children [Citation142]. In supragingival plaque samples, collected from caries-active Kuwaiti children, the relative abundance of P. melaninogenica was significantly elevated [Citation143].
Comparison between Romanian adolescents with a high prevalence of dental caries and Swedish adolescents with or without caries yielded a core microbiome of 24 species, including three Prevotella and both Alloprevotella species [Citation144]. In Romanian adolescents with a high prevalence of caries and minimal dental care, the biofilm composition was dominated by ‘traditional’ caries pathogens, such as S. mutans and Streptococcus sobrinus, whereas their role was less pronounced in Swedish adolescents, who were under caries prevention programs. Interestingly, Swedish adolescents were typically characterized by an intake of sweets and having P. maculosa and P. nigrescens [Citation144].
In supragingival plaque of elderly, the prevalence and relative abundance of P. multisaccharivorax was significantly increased and associated with root caries, suggesting its role as a true root caries pathogen [Citation145,Citation146]. Instead, P. intermedia was implicated as being probiotic, since it was highly prevalent and abundant at healthy, caries-free sites [Citation146]. In a Japanese elderly population (n = 506), P. melaninogenica, P. histicola, and P. pallens were among 21 predominant OTUs in the tongue microbiota [Citation42]. P. histicola, together with Veillonella atypica, Streptococcus salivarius, and Streptococcus parasanguinis, was more predominant in elderly with fewer teeth, a higher plaque index, and more dental caries experience.
Progressing caries lesions eventually reach the dental pulp, leading to pulpitis and pulpal necrosis. In Brazilian children, P. intermedia (96.9%), P. nigrescens (56.2%), and P. denticola (53.1%) were among the most prevalent species in primary teeth exhibiting pulp necrosis [Citation147]. The microbiome of infectious root canals in their apical portions was primarily characterized as having Prevotella (17.9%) and Bacteroidaceae G-1 (14.3%), P. oris being the most numerous species [Citation148]. Interestingly, elevated levels of Prevotella and Porphyromonas were found in symptomatic cases. In addition, P. baroniae, P. intermedia, P. multisacchariavorax, and A. tannerae predominate in apical periodontitis [Citation149,Citation150], and P. buccae and P. intermedia in radicular and residual cysts [Citation151].
Odontogenic infections
Odontogenic infections are typically of endodontic or periodontal origin. In acute apical abscesses, the most prevalent Prevotella taxa include P. multisaccharivorax, P. intermedia, P. baroniae, and A. tannerae, as well as occasional recoveries of P. oralis and P. nigrescens [Citation152–156]. Significant variations in the microbiome can be, at least in part, explained by geographic/ethnic differences; for example, P. nigrescens, A. tannerae, and F. nucleatum were more prevalent in endodontic abscess samples from the USA than in those from Brazil [Citation153]. Of the 138 clinical strains isolated from periodontal abscesses, the majority represented Prevotella taxa [Citation157]. Of those, 1/3 were identified as P. intermedia, and around 1/10 as P. nigrescens, P. melaninogenica, P. dentalis, P. denticola, and P. buccae . Occasional recoveries included P. fusca, P. histicola, P. multiformis, P. oris, P. oulorum, P. pallens, P. salivae, P. veroralis, and A. rava. Recently, the microbiome compositions between abscess pus and the corresponding periodontal pocket (coronally from the abscess) and periodontally healthy gingival crevice samples were compared [Citation158]. Based on the beta-diversity analyses, periodontal abscess pus and the periodontal pocket shared similar compositions. In comparison to the healthy gingival crevice, P. intermedia and P. heparinolytica, together with P. gingivalis, were the predominant findings and significantly more abundant in periodontal abscesses [Citation158]. In 50 German patients hospitalized for severe odontogenic abscesses, the frequency of Prevotella was 17.7% in saliva (collected prior to the abscess incision) and 27.2% in the pus samples, being the most abundant genus in odontogenic abscesses [Citation159]. In spreading odontogenic infections, members of the genus Prevotella are common, especially P. buccae and P. oris [Citation160–162].
Alveolar osteitis, accompanied by poorly integrated blood clot in the alveolar socket, is a common complication after tooth extraction, resulting in severe postoperative pain. Prevotella has been found to be the most frequent genus in sockets with and without alveolar osteitis (22% and 18%, respectively) [Citation163]. Prevotella recoveries from alveolar osteitis sites were only P. nanceiensis, P. pleuritidis, and P. veroralis, and occasionally, P. copri, P. multiformis, and P. oulorum. P. melaninogenica and P. intermedia were over-represented but P. loescheii and P. salivae were under-represented at alveolar osteitis sites compared to sockets without complications. In tooth sockets without complications, P. bivia and P. marshii, and occasional P. aurantiaca, P. baroniae, P. bergensis, and P. oralis were found [Citation163].
Diseases on oral mucosae
Oral lichen planus (OLP) is characterized as a chronic inflammatory condition affecting the oral mucosa with white striated, non-erosive or erosive lesions. In 13 OLP cases, the abundance of P. melaninogenica was significantly higher in comparison to samples from healthy mucosa [Citation164]. Based on FISH analyses, epithelial and lamina propria invasion by P. melaninogenica was observed in OLP samples. As a novel aspect, P. melaninogenica was confirmed to activate the NF-kB signaling pathway after adhering to and invading macrophages, thus, indicating its role in the pathogenesis of OLP [Citation164]. In recurrent aphthous stomatitis, Prevotella and Alloprevotella seem to be among the 10 most abundant genera, and the formation of aphthous ulcers may be due to the colonization of Escherichia coli and/or Alloprevotella [Citation165]. Similarly, oral leukoplakia as a potentially malignant disorder was recently linked to salivary Prevotella, which was among the top 10 abundant taxa and among the most significant discriminating genera for leukoplakia [Citation166].
Sjögren’s syndrome
Sjögren’s syndrome (SS), an autoimmune disease, typically affects the salivary and lacrimal glands. In a Norwegian study, the composition of the salivary microbiome was examined in 45 women divided into primary-SS (p-SS), dry mouth (non-SS), and healthy control groups [Citation167]. The mean relative abundance of the genus Prevotella was around 30%, and P. histicola, P. melaninogenica, and P. salivae were among the core microbiomes in all three groups. However, dysbiotic shifts in the salivary microbiota, including significantly reduced abundance of P. pallens and detection of P. nanceiencis and P. intermedia, were observed only in women with disturbed saliva secretion [Citation167]. In a Korean study, comparing 25 women with p-SS and 25 controls with or without dry mouth, P. melaninogenica was highly associated (OR 22.4) with Sjögren’s syndrome [Citation168]. Labial salivary gland biopsies with focal sialoadenitis revealed a high presence of bacteria, including P. melaninogenica, within the ductal cells and in the area of infiltration. In order to understand the bacterial involvement in the etiopathogenesis of Sjögren’s syndrome and in the functional and phenotypic changes in salivary glands, the authors used a human submandibular gland tumor cell model [Citation168]. According to the results, P. melaninogenica increased and P. histicola decreased interferon (IFN)-λ production. Moreover, P. melaninogenica induced the deregulation of submandibular gland cells.
Halitosis
Halitosis (oral malodor) is a common and multifactorial condition associated with volatile sulphur compounds (VSCs) of intra-oral or extra-oral origin. In preschool-aged children with halitosis, different Prevotella taxa have been reported; relative proportions of P. pallens and A. rava were dominant in tongue-coating samples [Citation169], whereas the prevalence and relative abundance of salivary P. shahii were higher in halitosis children compared to children with normal VSC concentrations [Citation170]. In Scottish adults with halitosis, P. melaninogenica, P. pallens, and P. veroralis were the predominant species on the tongue dorsum [Citation171]. The comparison between the tongue microbiome of periodontally healthy Chinese individuals with and without intraoral halitosis revealed significantly higher relative percentages of Prevotella and Alloprevotella in halitosis samples [Citation172]. Moreover, both genera correlated with increased VSC (here: H2S and CH3SH) values. This observation was in line with a Korean study where higher abundances of Prevotella and Alloprevotella in saliva correlated with amine metabolites, suggesting a causative mechanism via cadaverine and putrescine pathways [Citation173].
Prevotella in squamous cell carcinoma of the head and neck
The impact of the oral microbiota on cancer development has drawn remarkable attention among researchers. Dysbiotic bacterial communities are currently considered to play a role in carcinogenesis and cancer progression. Although the highest interest has been directed to F. nucleatum and P. gingivalis [Citation174,Citation175], there is considerable evidence available on the impact of various oral Prevotella species and A. tannerae on many cancer types in humans.
In the head and neck, squamous cell carcinoma (SCC), developing from the mucosal epithelium in the oral cavity, pharynx, and larynx, is among the most common cancer types globally [Citation176]. Typical risk factors include an exposure to carcinogen-containing products (tobacco, alcohol, pollutants) as well as certain infectious agents (human papilloma and Epstein–Barr viruses). It has been shown that smokers have an altered bacterial composition on buccal mucosae, including P. salivae, P. melaninogenica, and P. histicola [Citation34]. The authors suggested that smoking with increased nitrate intake favors nitrate-reducing species, which could be exposed to cancerous changes over the course of time. Also, poor oral hygiene, which favors the growth of moderate periodontal pathogens, e.g. P. intermedia and A. tannerae, may be linked to an increased risk for oral SCC [Citation177]. Interestingly, P. intermedia was recognized at significantly elevated levels from tumor sites located in the gingiva but also in the specific mucosa of the tongue, or buccal and mouth floor mucosae [Citation178].
Recently, Gopinath and co-workers [Citation179] studied the oral bacteriome of oral SCC patients, collecting three types of samples from the oral cavity: ‘whole mouth fluid’ (including components of saliva, mucosal transudate, inflammatory exudate, and gingival crevicular fluid), a swab of the cancer surface, and cancer tissue from the body of the tumor. First, it was shown that the oral bacteriome, based on the whole mouth fluid sample, and its metabolic activity in cancer patients are clearly distinct from those of healthy controls. Further, the authors found that the bacteriome differed significantly between the surface and deeper part of the tumor. Prevotella organisms were located in deep cancerous tissue, and their increase in abundance, being in line with previous reports, could indicate a role in cancer progression [Citation179]. Studies presenting Prevotella species-level data have found P. intermedia, P. loescheii, and P. salivae enriched in cancer tissues [Citation178,Citation180,Citation181]. Altered salivary microbiomes with increased abundance of P. multisaccharivorax [Citation182], P. melaninogenica, P. nanceiensis, P. nigrescens, and P. pallens [Citation183], and P. intermedia, P. oris, and A. tannerae [Citation177] have been reported from oral SCC patients. In the latter study, however, P. melaninogenica and P. histicola were slightly more abundant and P. pallens equally abundant in controls [Citation177]. Interestingly, reduced proportions of mucosa-associated P. melaninogenica and P. histicola were observed in the salivary microbiota after surgical tongue resection compared to pre-surgery salivary samples of tongue cancer patients [Citation184].
In tonsillar crypts, the genus Prevotella, in addition to Veillonella and Fusobacterium, showed the highest abundance in patients with tonsillar SCC, differing from tonsillar findings in obstructive sleep apnea patients used as controls [Citation185]. These bacterial alterations were interpreted as being connected to conditions induced by tumor growth. At the species level, P. melaninogenica was among the top-10 tumor-predictive species, and this was seen to be consistent with its increased presence in saliva, as reported in previous studies [Citation185]. In contrast, an Indian study, investigating alterations in the salivary microbiota of oropharyngeal and hypopharyngeal SCC patients, reported reduced abundances of P. melaninogenica, P. nanceiensis, P. nigrescens, P. pallens, and A. tannerae both in oropharyngeal and hypopharyngeal cancer patients [Citation186]. Instead, the study reported, for the first time, the presence and increased abundance of P. copri, an intestinal Prevotella species, in these cancer types.
In the throat, the three most frequent genera with proportions higher than 0.01%, in descending order, were Streptococcus, Fusobacterium, and Prevotella [Citation187]. Bacterial community compositions and abundances differed between tissue samples (two collection sites: upper and lower epiglottis) from laryngeal cancer patients and those from controls with vocal cord polyps. Concerning Prevotella, increased prevalences of P. intermedia, P. nigrescens, P. oris, and A. tannerae were found in cancer patients [Citation187]. Another Chinese study investigated bacterial communities on mucous membranes of the vocal cords [Citation188], targeting to reveal differences between glottic laryngeal SCC and adjacent non-tumor tissues and vocal cord polyp tissues (controls). The genera Fusobacterium, Alloprevotella, and Prevotella proved to be enriched in tumor tissue.
A follow-up study looked for changes in microbial community profiles of the larynx prior to laryngectomy and 1 week and 24 weeks thereafter [Citation189]. Smoking and alcohol consumption strongly affected the community structure; a positive association was found between the drinking index and abundance of Prevotella in cancer patients prior to laryngectomy. The abundance of Alloprevotella was significantly reduced at 24 weeks post-surgery. It has been speculated whether alcohol consumption influences the microbial community structure of the throat, which could then lead to laryngeal carcinogenesis.
Non-oral infections with emphasis on oral Prevotella
Abscesses and soft tissue infections
Oral bacteria are commonly reported from various types of abscesses at different body sites, including the brain and lungs. A part of brain abscesses was polymicrobial, including microaerophilic and anaerobic species, especially Aggregatibacter aphrophilus, Streptococcus intermedius, and F. nucleatum [Citation190,Citation191]. The role of F. nucleatum as one of the primary pathogens in brain abscesses with strictly anaerobic species was suggested to be due to its key role in coaggregation and biofilm formation as seen in oral infections [Citation191]. Hematogenous spread of oral pathogens and dysbiotic biofilms through the skull bone may be an important infectious route for their access into the venous system of the brain. Thus, bacterial brain abscesses could be of oral or sinus origin. These comprehensive studies on the microbiology of brain abscesses, using advanced molecular methods, recognized various Prevotella species in pus specimens as follows: P. baroniae, P. denticola, P. heparinolytica P. intermedia, P. nigrescens, P. oris, P. pleuritidis, and A. tannerae as well as not-yet-named Prevotella HOT 314 and HOT 317 [Citation190,Citation191].
Polymicrobial findings, clustering F. nucleatum with other anaerobic species typical in oral biofilms, encouraged a Norwegian research group to take a thorough look at bacterial recoveries from pleural empyemas, due to similarities between the brain and lungs as being highly oxygenated organs, and to compare the bacterial compositions of these purulent infections [Citation192]. Pleural fluid samples were examined using massive parallel sequencing of partial 16S rRNA and rpoB genes, i.e. the same method as used for brain abscesses [Citation191]. Again, F. nucleatum and S. intermedius were dominant findings in empyema specimens similarly to previous brain specimens. By analyzing 27 empyemas with poorly described etiology and 25 brain abscesses where oral or sinus origin was assumed, several anaerobic species, among those P. oris, P. pleuritidis, Prevotella HMT 317, and A. tannerae, were recovered from both brain abscesses and pleural empyemas, while P. buccae findings were only from the latter one [Citation192].
In the oropharynx during recurrent tonsillitis, a shift in bacterial composition has occurred, resulting in an increased abundance of P. melaninogenica/P. histicola [Citation29]. Oral members of the genus Prevotella are among the polymicrobial consortia of peritonsillar abscesses with or without complications [Citation193,Citation194]. In a study on abscesses of the head and neck region, 33 clinical Prevotella isolates were identified as P. melaninogenica, P. oralis, P. buccae, P. intermedia, and P. denticola with a biochemical test kit [Citation195]. In breast abscesses, P. buccae, P. bivia, and P. bergensis have been among anaerobic bacteria [Citation196]. Interestingly, a metronidazole-resistant P. buccae strain was reported from an infected breast cyst [Citation197]. Also, the genus Prevotella seems to play a role in hidradenitis suppurativa (acne inversa) [Citation198,Citation199]. This is a chronic inflammatory skin disease, where purulent lesions are formed in sweltering regions, including the underarms and under the breasts. Anaerobes predominated in 149 samples from lesions compared to 175 samples from unaffected skinfolds, and especially Gram-negative, strictly anaerobic bacteria were enriched; Prevotella and Porphyromonas were detected nearly ubiquitously and were the most abundant bacterial genera in lesions where P. intermedia, P. nigrescens, and P. melaninogenica were named [Citation198]. In osteomyelitic bone specimens collected from 17 diabetic foot infections, Prevotella proved to be the most abundant genus [Citation200]. P. denticola, P. fusca, and P. intermedia, typical Prevotella species of oral origin, and P. jejuni, were detected, and all of them positively correlated with the duration of the foot infection.
Some novel Prevotella species without knowledge of their preferred habitat have been among recoveries, especially from pus specimens. For example, the description of P. pleuritidis was performed based on a single strain from pleural fluid [Citation201], and since then, its presence was reported also from lung and liver abscesses [Citation202,Citation203]. Noteworthy are the frequently abundant findings of P. pleuritidis among the oral microbiota of the study populations from the United Arab Emirates and Sweden: in subgingival biofilms of heavy smokers [Citation204] and in saliva of early-onset rheumatoid arthritis patients [Citation205], respectively. In addition, increased proportions of P. pleuritidis subgingivally have been reported from systemic lupus erythematosus patients with periodontitis [Citation206] and from post-extraction sockets with alveolar osteitis [Citation163]. These results strongly indicate that P. pleuritidis is an oral species. The strains of three individuals characterized for the description of P. nanceiensis originated from the pus of a lung abscess, broncho-alveolar fluid, and blood [Citation207]. Since then, P. nanceiensis has been recovered from the adenoid specimens of children with middle ear effusion [Citation208], health-associated tongue coating microbiome of children [Citation170], as well as from saliva of children with a gluten-free diet due to celiac disease [Citation209], and from saliva [Citation128] and alveolar osteitis [Citation163] of adults, indicating P. nanceiensis as being of oral origin. In the description of two novel Prevotella species, the P. vespertina strain originated from an unspecified abscess located at the upper respiratory tract [Citation210] and the P. illustrans strain from an oropharyngeal abscess [Citation211]. The eight strains described as P. bergensis were isolated from infections of the skin and soft-tissue abscesses [Citation212], and recently, it was found in a breast abscess [Citation196]. The original description of P. timonensis was based on a single strain from a breast abscess [Citation213]. Among 84 clinical P. timonensis strains collected in a university hospital during a 10-year period, most strains came from cutaneous/soft tissue and bone infections, but also from genitourinary tract specimens [Citation214]. Despite being originally isolated from a breast abscess, it was assumed that the habitat of P. timonensis is below the waistline. In contrast, P. jejuni, originally isolated from the jejunum of a celiac child, seems to be among the resident members of the oral cavity [Citation33,Citation37,Citation38].
Potential involvement of oral Prevotella in chronic pathological conditions outside the mouth
Rheumatic diseases
To date, P. gingivalis is widely considered a major oral species associated with rheumatoid arthritis (RA), a common autoimmune disease. However, by comparing subgingival microbiota and its abundance and diversity between new-onset RA, chronic RA, and healthy individuals, it was shown that the overall exposure to P. gingivalis did not differ between the RA and control groups, whereas Prevotella (and Leptotrichia) species were characteristic for separating the newly-onset RA group regardless of periodontal status [Citation215]. Based on these results, Scher and co-workers [Citation215] appealed for further studies on these anaerobic taxa. Since then, the relative abundance of Prevotella has been shown to be increased in saliva of patients with early RA but also at-risk individuals, and an interesting observation was a low abundance for P. gingivalis also here [Citation216]. Indeed, there is now increasing evidence available on specific Prevotella organisms in this destructive inflammatory disease of synovial joints. Significant differences were observed in subgingival bacterial communities in RA patients who had high bacterial loads and diverse microbiotas, even in periodontitis-free individuals, in comparison to controls [Citation217]. Among elevated Prevotella findings were P. melaninogenica, P. denticola, P. histicola, P. nigrescens, P. oulorum, and P. maculosa. Concerning P. melaninogenica, the finding is in agreement with its increased abundance in rheumatoid and osteoarthritis individuals detected by Chen et al. [Citation218], but in contrast to that reported by Lehenaff et al. [Citation219]. Recently, a Swedish study group [Citation205] focused on species-level differences of the oral microbiota between 61 untreated early-onset RA patients and their healthy age- and sex-matched controls, also taking their periodontal status into account. P. pleuritidis, P. dentalis, P. intermedia, two Prevotella clones (HMT 317 and HMT 300), and A. tannerae were among the top 20 most influential taxa for separating the cases and controls. Influential Prevotella species in saliva of early-onset RA individuals without deepened periodontal pockets were P. denticola and P. nigrescens, and in those with deepened pockets (pocket depth >6 mm) were P. dentalis, P. intermedia, and P. melaninogenica [Citation205]. Although P. pleuritidis was more abundant in early-onset RA patients, it was not associated with periodontal status.
The link between systemic lupus erythematosus (SLE), which is an autoimmune disease affecting joints, and periodontitis was studied in 52 SLE patients and their age- and sex-matched controls by Correa and co-workers [Citation206]. It is assumed that this systemic disease alters the composition of subgingival biofilms, exposing them to the development of periodontitis via dysbiotic microbiota and deterioration of SLE. Indeed, SLE patients had a reduced microbial diversity and higher pathogen load, among those P. nigrescens, P. oris, and P. oulorum, regardless of the periodontal status. The relative abundances of P. nigrescens correlated with higher SLE index scores (SLE damage and activity), P. oris with SLE duration, P. melaninogenica with increased C-reactive protein levels, P. denticola with elevated neutrophils, and P. oulorum with elevated lymphocytes [Citation206].
Neurological diseases
The impact of oral health on neurodegenerative diseases has emerged along with research interest in the potential inflammatory etiology of Alzheimer's disease (AD), and some evidence exists on Prevotella organisms in this context. Using a longitudinal study design, serum IgG levels to seven periodontal pathogens, including P. intermedia, were analyzed in 158 study participants who were cognitively intact at baseline and were then followed for around 10 years [Citation220]. Of the 81 individuals experiencing either AD or mild cognitive impairment, significantly increased IgG levels to P. intermedia and F. nucleatum in particular were found prior to the diagnosis of AD. A recent study using serum antibody data from the NHANES study population showed that AD’s mortality risk was increased in individuals with high IgG levels for several periodontal pathogens, including P. intermedia, P. nigrescens, and P. melaninogenica [Citation221]. The link to periodontitis-associated bacteria was stronger for older adults.
In pioneer studies from the beginning of the 21st century, intestinal microorganisms, especially anaerobic bacterial taxa, were suggested to play a potential role in autism, a neurodevelopmental disorder [Citation222,Citation223]. Interestingly, while non-clostridial anaerobic and microaerophilic bacteria were common in aspirates collected from the small intestine of autistic children, they were absent from their controls [Citation222]. At the phylum level, important differences between the autistic and neurotypical children have been observed especially within Firmicutes, Bacteroidetes, Actinobacteria, and Proteobacteria [Citation223]. Now, a few studies have looked for the role of the oral microbiota in this context, and only very limited data are available on Prevotella organisms. Qiao and co-workers [Citation224] analyzed bacterial taxa at the genus level in dental plaque and saliva of children with and without autism spectrum disorder (ASD). Microbial alterations were observed, including decreased rates of the genera Prevotella and Alloprevotella in saliva, but also a reduction of the family Prevotellaceae co-occurrence network in dental plaque of ASD children [Citation224]. Recently, the tongue microbiome was examined to assess whether there could be a link between the bacterial composition on the tongue surface and ASD [Citation225]. The genus Prevotella and the species P. melaninogenica were among the most abundant findings in studied children; however, no significant differences were detected between ASD and control children. The microbiology of these neurodevelopmental disorders is still at an early phase to be assessed.
The potential role of bacterial communities of the oral cavity and oropharynx in individuals with psychiatric disorders is of current research interest. In salivary samples from young adults with and without depression, the most prevalent were Neisseria spp. and P. nigrescens, but distinct bacterial abundance patterns were found between the cases and controls [Citation226]. Smoking was recognized as a contributing factor for alterations in the salivary microbiome. While P. nigrescens was more abundant in depressive individuals, several other known species like P. nanceiensis, P. oris, and A. rava were reduced and more abundant in controls [Citation226]. A similar reduced abundance of the genus Prevotella in the oropharyngeal microbiome was reported for individuals with schizophrenia and mania as well as with major depressive disorder in comparison to non-psychiatric controls [Citation227]. The altered beta-diversity in individuals with schizophrenia and mania was independent of smoking. No significant associations were observed between the levels of identified bacterial taxa and psychiatric symptoms.
Some fragmentary information is available on the involvement of Prevotella organisms in vascular conditions in the intracranial region. Regarding ischemic stroke, the genus Prevotella was found to be enriched in the patient group with poor treatment outcome [Citation228]. DNA of P. intermedia has been recovered from ruptured intracranial aneurysm walls [Citation229] and in culture of cerebrospinal fluid from a patient of intracranial mycotic aneurysm [Citation230]. A metronidazole-resistant P. loescheii strain has been isolated from subdural hematoma [Citation231].
Summary
Research into the genus Prevotella has increased considerably over the past years. Prevotella has a wide intra-genus variation, which leads to a variety of properties between Prevotella species. Interest is focused on their involvement as commensals and potentially pathogenic organisms at different locations of the human body. In the oral cavity, Prevotella are important members of bacterial communities on various mucosal surfaces and in saliva as well as in dental biofilms above and below the gumline. However, knowledge of individual Prevotella species other than P. intermedia and P. nigrescens and their role in keeping homeostasis or interfering in dysbiotic biofilms is still limited. As regards P. melaninogenica, on the one hand, it belongs to the core oral bacteriome in individuals with healthy oral tissues but, on the other hand, it seems to be involved in many pathogenic conditions inside and outside the mouth. Is it a bystander or an active player in these cases? Also, the influence of behavioral and lifestyle factors on oral Prevotella is not clear. For example, an interesting question whether diet could have an impact on oral Prevotella species remains to be answered. Furthermore, increasingly attractive is the potential link of oral Prevotella organisms to systemic conditions not usually connected to microbes.
Disclosure statement
No potential conflict of interest was reported by the author(s).
Additional information
Funding
References
- Costello EK, Lauber CL, Hamady M, et al. Bacterial community variation in human body habitats across space and time. Science. 2009;326(5960):1694–24.
- Dewhirst FE, Chen T, Izard J, et al. The human oral microbiome. J Bacteriol. 2010;192(19):5002–5017.
- Shah HN, Collins DM. Prevotella, a new genus to include Bacteroides melaninogenicus and related species formerly classified in the genus Bacteroides. Int J Syst Bacteriol. 1990;40(2):205–208.
- Tett A, Pasolli E, Masetti G, et al. Prevotella diversity, niches and interactions with the human host. Nat Rev Microbiol. 2021;19(9):585–599.
- Downes J, Dewhirst FE, Tanner ACR, et al. Description of Alloprevotella rava gen. nov., sp. nov., isolated from the human oral cavity, and reclassification of Prevotella tannerae Moore et al. 1994 as Alloprevotella tannerae gen. nov., comb. nov. Int J Syst Evol Microbiol. 2013;63(Pt_4):1214–1218.
- Könönen E, Asikainen S, Saarela M, et al. The oral gram-negative anaerobic microflora in young children: longitudinal changes from edentulous to dentate mouth. Oral Microbiol Immunol. 1994;9(3):136–141.
- Könönen E, Kanervo A, Takala A, et al. Establishment of oral anaerobes during the first year of life. J Dent Res. 1999;78(10):1634–1639.
- Könönen E. Development of oral bacterial flora in young children. Ann Med. 2000;32(2):107–112.
- Socransky SS, Haffajee AD, Cugini MA, et al. Microbial complexes in subgingival plaque. J Clin Periodontol. 1998;25(2):134–144.
- Jiao Y, Hasegawa M, Inohara N. The role of oral pathobionts in dysbiosis during periodontitis development. J Dent Res. 2014;93(6):539–546.
- Kleinstein SE, Nelson KE, Freire M. Inflammatory networks linking oral microbiome with systemic health and disease. J Dent Res. 2020;99(10):1131–1139.
- Bahrani-Mougeot FK, Paster BJ, Coleman S, et al. Diverse and novel oral bacterial species in blood following dental procedures. J Clin Microbiol. 2008;46(6):2129–2132.
- Emery DC, Cerajewska TL, Seong J, et al. Comparison of blood bacterial communities in periodontal health and periodontal disease. Front Cell Infect Microbiol. 2021;10:577485.
- Kitamoto S, Nagao-Kitamoto H, Hein R, et al. The bacterial connection between the oral cavity and the gut diseases. J Dent Res. 2020;99(9):1021–1029.
- Conrads G, Nagy E, Könönen E. Bacteroides, Porphyromonas, Prevotella, Fusobacterium, and other anaerobic gram-negative rods. In: Carroll KC, et al. editors. Manual of clinical microbiology. 12thed. Washington DC: American Society for Microbiology; 2019:995–1023.
- Könönen E, Gursoy UK. Oral Prevotella species and their connection to events of clinical relevance in gastrointestinal and respiratory tracts. Front Microbiol. 2022;12:798763.
- Xu X, He J, Xue J, et al. Oral cavity contains distinct niches with dynamic microbial communities. Environ Microbiol. 2015;17(3):699–710.
- Haraldsson G, Holbrook WP, Könönen E. Clonal persistence of oral Fusobacterium nucleatum in infancy. J Dent Res. 2004;83(6):500–504.
- Könönen E, Wolf J, Mättö J, et al. The Prevotella intermedia group organisms in young children and their mothers as related to maternal periodontal status. J Periodontal Res. 2000;35(6):329–334.
- Monteiro MF, Altabtbaei K, Kumar PS, et al. Parents with periodontitis impact the subgingival colonization of their offspring. Sci Rep. 2021;11(1):1357.
- Könönen E, Saarela M, Karjalainen J, et al. Transmission of oral Prevotella melaninogenica between a mother and her young child. Oral Microbiol Immunol. 1994;9(5):310–314.
- Mättö J, Saarela M, von Troil-Lindén B, et al. Distribution and genetic analysis of oral Prevotella intermedia and Prevotella nigrescens. Oral Microbiol Immunol. 1996;11(2):96–102.
- Kaan AMM, Kahharova D, Zaura E. Acquisition and establishment of the oral microbiota. Periodontol 2000. 2021;86(1):123–141.
- Crielaard W, Zaura E, Schuller AA, et al. Exploring the oral microbiota of children at various developmental stages of their dentition in the relation to their oral health. BMC Med Genomics. 2011;4(1):22.
- Rotimi VO, Salako NO, Divia M, et al. Prevalence of periodontal bacteria in saliva of Kuwaiti children at different age groups. J Infect Public Health. 2010;3(2):76–82.
- Ooshima T, Nishiyama N, Hou B, et al. Occurrence of periodontal bacteria in healthy children: a 2-year longitudinal study. Community Dent Oral Epidemiol. 2003;31(6):417–425.
- Keijser BJ, Zaura E, Huse SM, et al. Pyrosequencing analysis of the oral microflora of healthy adults. J Dent Res. 2008;87(11):1016–1020.
- Mager DL, Ximenez-Fyvie LA, Haffajee AD, et al. Distribution of selected bacterial species on intraoral surfaces. J Clin Periodontol. 2003;30:644–654.
- Jensen A, Fagö-Olsen H, Sørensen CH, et al. Molecular mapping to species level of the tonsillar crypt microbiota associated with health and recurrent tonsillitis. PLoS One. 2013;8:e56418.
- Bik EM, Long CD, Armitage GC, et al. Bacterial diversity in the oral cavity of 10 healthy individuals. ISME J. 2010;4(8):962–974.
- Al-Hebshi NN, Abdulhaq A, Albarrag A, et al. Species-level core oral bacteriome identified by 16S rRNA pyrosequencing in a healthy young Arab population. J Oral Microbiol. 2016;8(1):31444.
- Takeshita T, Matsuo K, Furuta M, et al. Distinct composition of the oral indigenous microbiota in South Korean and Japanese adults. Sci Rep. 2014;4(1):6990.
- Widyarman AS, Theodorea CF, Udawatte NS, et al. Diversity of oral microbiome of women from urban and rural areas of Indonesia: a pilot study. Front Oral Health. 2021;2. 738306.
- Karabudak S, Ari O, Durmaz B, et al. Analysis of the effect of smoking on the buccal microbiome using next-generation sequencing technology. J Med Microbiol. 2019;68(8):1148–1158.
- Könönen E. Pigmented Prevotella species in the periodontally healthy oral cavity. FEMS Immunol Med Microbiol. 1993;6(2–3):201–205.
- Gürsoy M, Haraldsson G, Hyvönen M, et al. Does the frequency of Prevotella intermedia increase during pregnancy? Oral Microbiol Immunol. 2009;24(4):299–303.
- Gürsoy M, Harju I, Matomäki J, et al. Performance of MALDI-TOF MS for identification of oral Prevotella species. Anaerobe. 2017;47:89–93.
- Haran JP, Bradley E, Zeamer AL, et al. Inflammation-type dysbiosis of the oral microbiome associates with the duration of COVID-19 symptoms and long COVID. JCI Insight. 2021;6(20):e152346.
- Könönen E, Paju S, Pussinen PJ, et al. Population-based study of salivary carriage of periodontal pathogens in adults. J Clin Microbiol. 2007;45(8):2446–2451.
- Hamlet SM, Cullinan MP, Westerman B, et al. Distribution of Actinobacillus actinomycetemcomitans, Porphyromonas gingivalis and Prevotella intermedia in an Australian population. J Clin Periodontol. 2001;28(12):1163–1171.
- Takeshita T, Kageyama S, Furuta M, et al. Bacterial diversity in saliva and oral health-related conditions: the Hisayama Study. Sci Rep. 2016;6(1):22164.
- Asakawa M, Takeshita T, Furuta M, et al. Tongue microbiota and oral health status in community-dwelling elderly adults. mSphere. 2018;3(4):e00332–18.
- Shah HN, Gharbia SE. Biochemical and chemical studies on strains designated Prevotella intermedia and proposal of a new pigmented species, Prevotella nigrescens sp. nov. Int J Syst Bacteriol. 1992;42(4):542–546.
- Könönen E, Eerola E, Frandsen EV, et al. Phylogenetic characterization and proposal of a new pigmented species to the genus Prevotella: prevotella pallens sp. nov. Int J Syst Bacteriol. 1998;48(1):47–51.
- Sakamoto M, Suzuki N, Okamoto M. Prevotella aurantiaca sp. nov., isolated from the human oral cavity. Int J Syst Evol Microbiol. 2010;60(3):500–503.
- Gharbia SE, Haapasalo M, Shah HN, et al. Characterization of Prevotella intermedia and Prevotella nigrescens isolates from periodontic and endodontic infections. J Periodontol. 1994;65(1):56–61.
- Pei Z, Bini EJ, Yang L, et al. Bacterial biota in the human distal esophagus. Proc Natl Acad Sci U SA. 2004;101(12):4250–4255.
- Cornejo Ulloa P, Krom BP, van der Veen MH. Sex steroid hormones as a balancing factor in oral host microbiome interactions. Front Cell Infect Microbiol. 2021;11:714229.
- Kornman KS, Loesche WJ. Effects of estradiol and progesterone on Bacteroides melaninogenicus and Bacteroides gingivalis. Infect Immun. 1982;35(1):256–263.
- Fteita D, Könönen E, Söderling E, et al. Effect of estradiol on planktonic growth, coaggregation, and biofilm formation of the Prevotella intermedia group bacteria. Anaerobe. 2014;27:7–13.
- Fteita D, Könönen E, Gürsoy M, et al. Does estradiol have an impact on the dipeptidyl peptidase IV enzyme activity of the Prevotella intermedia group bacteria? Anaerobe. 2015;36:14–18.
- Fteita D, Musrati AA, Könönen E, et al. Dipeptidyl peptidase IV and quorum sensing signaling in biofilm-related virulence of Prevotella aurantiaca. Anaerobe. 2017;48:152–159.
- De Lima BR, Nicoloso GF, Fatturi-Parolo CC, et al. Prevotella strains and lactamic resistance gene distribution in different oral environments of children with pulp necrosis. Int Endod J. 2018;51(11):1196–1204.
- Wilson M. Biological activities of lipopolysaccharides from oral bacteria and their relevance to the pathogenesis of chronic periodontitis. Sci Prog. 1995;78:19–34.
- Dorn BR, Leung KL, Progulske-Fox A. Invasion of human oral epithelial cells by Prevotella intermedia. Infect Immun. 1998;66(12):6054–6057.
- Hieke C, Kriebel K, Engelmann R, et al. Human dental stem cells suppress PMN activity after infection with the periodontopathogens Prevotella intermedia and Tannerella forsythia. Sci Rep. 2016;15(6):39096.
- Yamane K, Yamanaka T, Yamamoto N, et al. A novel exopolysaccharide from a clinical isolate of Prevotella nigrescens: purification, chemical characterization and possible role in modifying human leukocyte phagocytosis. Oral Microbiol Immunol. 2005;20(1):1–9.
- Yamanaka T, Yamane K, Furukawa T, et al. Comparison of the virulence of exopolysaccharide-producing Prevotella intermedia to exopolysaccharide non-producing periodontopathic organisms. BMC Infect Dis. 2011;11(1):228.
- Takahashi N, Yamada T. Glucose metabolism by Prevotella intermedia and Prevotella nigrescens. Oral Microbiol Immunol. 2000;15(3):188–195.
- Byrne DP, Wawrzonek K, Jaworska A, et al. Role of the cysteine protease interpain A of Prevotella intermedia in breakdown and release of haem from haemoglobin. Biochem J. 2009;425(1):257–264.
- Zhang Y, Zhen M, Zhan Y, et al. Population-genomic insights into variation in Prevotella intermedia and Prevotella nigrescens isolates and its association with periodontal disease. Front Cell Infect Microbiol. 2017;21(7):409.
- Veith PD, Glew MD, Gorasia DG, et al. The Type IX secretion system and its role in bacterial function and pathogenesis. J Dent Res. 2021;10:220345211051599.
- Leung K, Nesbitt WE, Okamoto M, et al. Identification of a fimbriae-associated haemagglutinin fromPrevotella intermedia. Microb Pathog. 1999;26(3):139–148.
- Gursoy UK, Könönen E, Uitto VJ. Prevotella intermedia ATCC 25611 targets host cell lamellipodia in epithelial cell adhesion and invasion. Oral Microbiol Immunol. 2009;24(4):304–309.
- Kumagai Y, Yagishita H, Yajima A, et al. Molecular mechanism for connective tissue destruction by dipeptidyl aminopeptidase IV produced by the Periodontal Pathogen Porphyromonas gingivalis. Infect Immun. 2005;73(5):2655–2664.
- Yanagisawa M, Kuriyama T, Williams DW, et al. Proteinase activity of Prevotella species associated with oral purulent infection. Curr Microbiol. 2006;52(5):375–378.
- Yost S, Duran-Pinedo AE. The contribution of Tannerella forsythia dipeptidyl aminopeptidase IV in the breakdown of collagen. Mol Oral Microbiol. 2018;33(6):407–419.
- Neilands J, Wickström C, Kinnby B, et al. Bacterial profiles and proteolytic activity in peri-implantitis versus healthy sites. Anaerobe. 2015;35:28–34.
- Jansen HJ, Grenier D, Van der Hoeven JS. Characterization of immunoglobulin G-degrading proteases of Prevotella intermedia and Prevotella nigrescens. Oral Microbiol Immunol. 1995;10(3):138–145.
- Abbott CA, Baker E, Sutherland GR, et al. Genomic organization, exact localization, and tissue expression of the human CD26 (dipeptidyl peptidase IV) gene. Immunogenetics. 1994;40(5):331–338.
- Augustyns K, Bal G, Thonus G, et al. The unique properties of dipeptidyl-peptidase IV (DPP IV/CD26) and the therapeutic potential of DPP IV inhibitors. Curr Med Chem. 1999;6(4):311–327.
- Nemoto E, Sugawara S, Takada H, et al. Increase of CD26/dipeptidyl peptidase IV expression on human gingival fibroblasts upon stimulation with cytokines and bacterial components. Infect Immun. 1999;67(12):6225–6233.
- Uematsu T, Tanaka H, Yamaoka M, et al. Effects of oral squamous cell carcinoma-derived TGF-beta1 on CD26/DPPIV expression in T cells. Anticancer Res. 2004;24(2B):619–624.
- Metzemaekers M, Van Damme J, Mortier A, et al. Regulation of chemokine activity - A focus on the role of dipeptidyl peptidase IV/CD26. Front Immunol. 2016;11(7):483.
- Deacon CF. Metabolism of GIP and the contribution of GIP to the glucose-lowering properties of DPP-4 inhibitors. Peptides. 2020;125:170196.
- Bassendine MF, Bridge SH, McCaughan GW, et al. COVID-19 and comorbidities: a role for dipeptidyl peptidase 4 (DPP4) in disease severity? J Diabetes. 2020;12(9):649–658.
- Posadas-Sánchez R, Sánchez-Muñoz F, Guzmán-Martín CA, et al. Dipeptidylpeptidase-4 levels and DPP4 gene polymorphisms in patients with COVID-19. Association with disease and with severity. Life Sci. 2021;1(276):119410.
- Ohara-Nemoto Y, Nakasato M, Shimoyama Y, et al. Degradation of incretins and modulation of blood glucose levels by periodontopathic bacterial dipeptidyl peptidase 4. Infect Immun. 2017;18(85):e00277–17.
- Kondo Y, Sato K, Nagano K, et al. Involvement of PorK, a component of the type IX secretion system, in Prevotella melaninogenica pathogenicity. Microbiol Immunol. 2018;62(9):554–566.
- Hrv R, Devaki R, Kandi V. Comparison of hemagglutination and hemolytic activity of various bacterial clinical isolates against different human blood groups. Cureus. 2016;11(8):e489.
- Okamoto M, Maeda N, Kondo K, et al. Hemolytic and hemagglutinating activities of Prevotella intermedia and Prevotella nigrescens. FEMS Microbiol Lett. 1999;15(178):299–304.
- Silva TA, Noronha FS, de Macêdo Farias L, et al. In vitro activation of the hemolysin in Prevotella nigrescens ATCC 33563 and Prevotella intermedia ATCC 25611. Res Microbiol. 2004;155(1):31–38.
- Byrne DP, Potempa J, Olczak T, et al. Evidence of mutualism between two periodontal pathogens: co-operative haem acquisition by the HmuY haemophore of Porphyromonas gingivalis and the cysteine protease interpain A (InpA) of Prevotella intermedia. Mol Oral Microbiol. 2013;28(3):219–229.
- Smalley JW, Silver J, Birss AJ, et al. The haem pigment of the oral anaerobes Prevotella nigrescens and Prevotella intermedia is composed of iron (III) protoporphyrin IX in the monomeric form. Microbiology (Reading). 2003;149(7):1711–1718.
- Byrne DP, Manandhar SP, Potempa J, et al. Breakdown of albumin and haemalbumin by the cysteine protease interpain A, an albuminase of Prevotella intermedia. BMC Microbiol. 2015;15(1):185.
- de Lillo A, Fierro JF. Identification of a lactoferrin-binding protein in Prevotella nigrescens. FEMS Microbiol Lett. 1997;150(1):61–64.
- Duchesne P, Grenier D, Mayrand D. Binding and utilization of human transferrin by Prevotella nigrescens. Infect Immun. 1999;67(2):576–580.
- Haraldsson G, Meurman JH, Könönen E, et al. Properties of hemagglutination by Prevotella melaninogenica. Anaerobe. 2005;11(5):285–289.
- Huang R, Li M, Gregory RL. Bacterial interactions in dental biofilm. Virulence. 2011;2(5):435–444.
- Bradshaw DJ, Marsh PD, Watson GK, et al. Role of Fusobacterium nucleatum and coaggregation in Anaerobe survival in Planktonic and biofilm oral microbial communities during Aeration. Infect Immun. 1998;66(10):4729–4732.
- Sanz M, Beighton D, Curtis MA, et al. Role of microbial biofilms in the maintenance of oral health and in the development of dental caries and periodontal diseases. Consensus report of group 1 of the Joint EFP/ORCA workshop on the boundaries between caries and periodontal disease. J Clin Periodontol. 2017;44(Suppl 18):S5–S11.
- Takahashi N. Oral microbiome metabolism: from “Who are they?” to “What are they doing?”. J Dent Res. 2015;94(12):1628–1637.
- Saito K, Takahashi N, Horiuchi H, et al. Effects of glucose on formation of cytotoxic end-products and proteolytic activity of Prevotella intermedia, Prevotella nigrescens and Porphyromonas gingivalis. J Periodontal Res. 2001;36(6):355–360.
- Zijnge V, van Leeuwen MB, Degener JE, et al. Oral biofilm architecture on natural teeth. PLoS One. 2010;5(2):e9321.
- Albaghdadi SZ, Altaher JB, Drobiova H, et al. In vitro characterization of biofilm formation in Prevotella species. Front Oral Health. 2021; 2: 724194.
- Bamashmous S, Kotsakis GA, Kerns KA, et al. Human variation in gingival inflammation. Proc Natl Acad Sci U S A. 2021;118(27):e2012578118.
- Zhang X, Zhang D, Jia H, et al. The oral and gut microbiomes are perturbed in rheumatoid arthritis and partly normalized after treatment. Nat Med. 2015;21(8):895–905.
- Takahashi N. Acid-neutralizing activity during amino acid fermentation by Porphyromonas gingivalis, Prevotella intermedia and Fusobacterium nucleatum. Oral Microbiol Immunol. 2003;18(2):109–113.
- Okuda T, Kokubu E, Kawana T, et al. Synergy in biofilm formation between Fusobacterium nucleatum and Prevotella species. Anaerobe. 2012;18(1):110–116.
- Sato T, Nakazawa F. Coaggregation between Prevotella oris and Porphyromonas gingivalis. J Microbiol Immunol Infect. 2014;47(3):182–186.
- Barbosa GM, Colombo AV, Rodrigues PH, et al. Intraspecies variability affects heterotypic biofilms of Porphyromonas gingivalis and Prevotella intermedia: evidences of strain-dependence biofilm modulation by physical contact and by released soluble factors. PLoS One. 2015;10(9):e0138687.
- Kurita-Ochiai T, Fukushima K, Ochiai K. Volatile fatty acids, metabolic by-products of periodontopathic bacteria, inhibit lymphocyte proliferation and cytokine production. J Dent Res. 1995;74(7):1367–1373.
- Kurita-Ochiai T, Ochiai K, Fukushima K. Volatile fatty acid, metabolic by-product of periodontopathic bacteria, induces apoptosis in WEHI 231 and RAJI B lymphoma cells and splenic B cells. Infect Immun. 1998;66(6):2587–2594.
- Terao N, Saito S, Hayakawa M, et al. Suppressive effect of soluble factor(s) derived from Prevotella loescheii ATCC 15930 on proliferation of human lymphocytes. Oral Microbiol Immunol. 1992;7(4):230–234.
- Silva VL, Carvalho MA, Nicoli JR, et al. Aerotolerance of human clinical isolates of Prevotella spp. J Appl Microbiol. 2003;94(4):701–707.
- Hillmann G, Dogan S, Geurtsen W. Histopathological investigation of gingival tissue from patients with rapidly progressive periodontitis. J Periodontol. 1998;69(2):195–208.
- Ji S, Kim Y, Min BM, et al. Innate immune responses of gingival epithelial cells to nonperiodontopathic and periodontopathic bacteria. J Periodontal Res. 2007;42(6):503–510.
- Sugiyama A, Uehara A, Iki K, et al. Activation of human gingival epithelial cells by cell-surface components of black-pigmented bacteria: augmentation of production of interleukin-8, granulocyte colony-stimulating factor and granulocyte-macrophage colony-stimulating factor and expression of intercellular adhesion molecule. J Med Microbiol. 2002;51(1):27–33.
- Guan SM, Fu SM, He JJ, et al. Prevotella intermedia induces Prostaglandin E 2 via multiple signaling Pathways. J Dent Res. 2011;90(1):121–127.
- Chung YH, Chang EJ, Kim SJ, et al. Lipopolysaccharide from Prevotella nigrescens stimulates osteoclastogenesis in cocultures of bone marrow mononuclear cells and primary osteoblasts. J Periodontal Res. 2006;41(4):288–296.
- Botero TM, Mantellini MG, Song W, et al. Effect of lipopolysaccharides on vascular endothelial growth factor expression on mouse pulp cells and macrophages. Eur J Oral Sci. 2003;111(3):228–234.
- de Aquino SG, Abdollahi-Roodsaz S, Koenders MI, et al. Periodontal pathogens directly promote autoimmune experimental arthritis by inducing a TLR2- and IL-1-driven Th17 response. J Immunol. 2014;192(9):4103–4111.
- Tokuda M, Nagaoka S, Torii M. Interleukin-10 receptor expression in human dental pulp cells in response to lipopolysaccharide from Prevotella intermedia. J Endod. 2002;28(3):177–180.
- Kubo K, Kamada T, Okamoto H, et al. Lipopolysaccharide increases cell surface-associated fibronectin in fibroblasts in vitro. Oral Microbiol Immunol. 1996;11(1):29–34.
- Leung KP, Torres BA. Prevotella intermedia Stimulates Expansion of Vβ-Specific CD4 + T Cells. Infect Immun. 2000;68(9):5420–5424.
- Slots J. Subgingival microflora and periodontal disease. J Clin Periodontol. 1979;6(5):351–382.
- Zambon JJ, Reynolds HS, Slots J. Black-pigmented Bacteroides spp. in the human oral cavity. Infect Immun. 1981;32(1):198–203.
- Loesche WJ, Syed SA, Laughon BE, et al. The bacteriology of acute necrotizing ulcerative gingivitis. J Periodontol. 1982;53(4):223–230.
- Maeda N, Okamoto M, Kondo K, et al. Incidence of Prevotella intermedia and Prevotella nigrescens in periodontal health and disease. Microbiol Immunol. 1998;42(9):583–589.
- Gürsoy M, Gürsoy UK, Sorsa T, et al. High salivary estrogen and risk of developing pregnancy gingivitis. J Periodontol. 2013;84(9):1281–1289.
- Balan P, Chong YS, Umashankar S, et al. Keystone species in pregnancy gingivitis: a snapshot of oral microbiome during pregnancy and postpartum period. Front Microbiol. 2018;9:2360.
- Kistler JO, Booth V, Bradshaw DJ, et al. Bacterial community development in experimental gingivitis. PLoS One. 2013;8(8):e71227.
- Nadkarni MA, Chhour KL, Browne GV, et al. Age-dependent changes in Porphyromonas gingivalis and Prevotella species/phylotypes in healthy gingiva and inflamed/diseased sub-gingival sites. Clin Oral Investig. 2015;19(4):911–919.
- Nadkarni MA, Browne GV, Chhour KL, et al. Pattern of distribution of Prevotella species/phylotypes associated with healthy gingiva and periodontal disease. Eur J Clin Microbiol Infect Dis. 2012;31(11):2989–2999.
- Kageyama S, Takeshita T, Asakawa M, et al. Relative abundance of total subgingival plaque-specific bacteria in salivary microbiota reflects the overall periodontal condition in patients with periodontitis. PLoS One. 2017;12(4):e0174782.
- Sun X, Li M, Xia L, et al. Alteration of salivary microbiome in periodontitis with or without type-2 diabetes mellitus and metformin treatment. Sci Rep. 2020;10(1):15363.
- Sun B, Liu B, Gao X, et al. Metagenomic analysis of saliva reveals disease-associated microbiotas in patients with periodontitis and Crohn’s disease-associated periodontitis. Front Cell Infect Microbiol. 2021;11:719411.
- Diao J, Yuan C, Tong P, et al. Potential roles of the free salivary microbiome dysbiosis in periodontal diseases. Front Cell Infect Microbiol. 2021;11:711282.
- Johnston W, Rosier BT, Artacho A, et al. Mechanical biofilm disruption causes microbial and immunological shifts in periodontitis patients. Sci Rep. 2021;11(1):9796.
- Dabdoub SM, Tsigarida AA, Kumar PS. Patient-specific analysis of periodontal and peri-implant microbiomes. J Dent Res. 2013;92(12 Suppl):168S–175S.
- Tsigarida AA, Dabdoub SM, Nagaraja HN, et al. The influence of smoking on the peri-implant microbiome. J Dent Res. 2015;94(9):1202–1217.
- Maruyama N, Maruyama F, Takeuchi Y, et al. Intraindividual variation in core microbiota in peri-implantitis and periodontitis. Sci Rep. 2014;4(1):6602.
- Komatsu K, Shiba T, Takeuchi Y, et al. Discriminating microbial community structure between peri-Implantitis and periodontitis with integrated metagenomic, metatranscriptomic, and network analysis. Front Cell Infect Microbiol. 2020;10:596490.
- Grischke J, Szafrański SP, Muthukumarasamy U, et al. Removable denture is a risk indicator for peri-implantitis and facilitates expansion of specific periodontopathogens: a cross-sectional study. BMC Oral Health. 2021;21(1):173.
- Polymeri A, van der Horst J, Buijs MJ, et al. Submucosal microbiome of peri-implant sites: a cross-sectional study. J Clin Periodontol. 2021;48(9):1228–1239.
- Yu XL, Chan Y, Zhuang L, et al. Intra-oral single-site comparisons of periodontal and peri-implant microbiota in health and disease. Clin Oral Implants Res. 2019;30(8):760–776.
- Tanner AC, RL K Jr, Holgerson PL, et al. Microbiota of severe early childhood caries before and after therapy. J Dent Res. 2011;90(11):1298–1305.
- Kianoush N, Adler CJ, Nguyen KA, et al. Bacterial profile of dentine caries and the impact of pH on bacterial population diversity. PLoS One. 2014;9(3):e92940.
- Schulze-Schweifing K, Banerjee A, Wade WG. Comparison of bacterial culture and 16S rRNA community profiling by clonal analysis and pyrosequencing for the characterization of the dentine caries-associated microbiome. Front Cell Infect Microbiol. 2014;4:164.
- Hurley E, Barrett MPJ, Kinirons M, et al. Comparison of the salivary and dentinal microbiome of children with severe-early childhood caries to the salivary microbiome of caries-free children. BMC Oral Health. 2019;19(1):13.
- Kressirer CA, Chen T, Lake Harriman K, et al. Functional profiles of coronal and dentin caries in children. J Oral Microbiol. 2018;10(1):1495976.
- Zhang L, Sun T, Zhu P, et al. Quantitative analysis of salivary oral bacteria associated with severe early childhood caries and construction of caries assessment model. Sci Rep. 2020b;10(1):6365.
- Qudeimat MA, Alyahya A, Karched M, et al. Dental plaque microbiota profiles of children with caries-free and caries-active dentition. J Dent. 2021;104:103539.
- Johansson I, Witkowska E, Kaveh B, et al. The microbiome in populations with a low and high prevalence of caries. J Dent Res. 2016;95(1):80–86.
- Preza D, Olsen I, Aas JA, et al. Bacterial profiles of root caries in elderly patients. J Clin Microbiol. 2008;46(6):2015–2021.
- Chen L, Qin B, Du M, et al. Extensive description and comparison of human supra-gingival microbiome in root caries and health. PLoS One. 2015;10(2):e0117064.
- Tavares WL, Neves de Brito LC, Teles RP, et al. Microbiota of deciduous endodontic infections analysed by MDA and checkerboard DNA-DNA hybridization. Int Endod J. 2011;44(3):225–235.
- de Brito LCN, Doolittle-Hall J, Lee C-T, et al. The apical root canal system microbial communities determined by next-generation sequencing. Sci Rep. 2020;10(1):10932.
- Rôças IN, JF S Jr. Prevalence of new candidate pathogens Prevotella baroniae, Prevotella multisaccharivorax and as-yet-uncultivated Bacteroidetes clone X083 in primary endodontic infections. J Endod. 2009;35(10):1359–1362.
- Nardello LCL, Amado PPP, Franco DC, et al. Next-generation sequencing to assess potentially active bacteria in endodontic infections. J Endod. 2020;46(8):1105–1112.
- Tek M, Metin M, Sener I, et al. The predominant bacteria isolated from radicular cysts. Head Face Med. 2013;9(1):25.
- Xia T, Baumgartner JC, David LL. Isolation and identification of Prevotella tannerae from endodontic infections. Oral Microbiol Immunol. 2000;15(4):273–275.
- Baumgartner JC, JF S Jr, Xia T, et al. Geographical differences in bacteria detected in endodontic infections using polymerase chain reaction. J Endod. 2004;30(3):141–144.
- JF S Jr, Rôças IN. The microbiota of acute apical abscesses. J Dent Res. 2009;88(1):61–65.
- George N, Flamiatos E, Kawasaki K, et al. Oral microbiota species in acute apical endodontic abscesses. J Oral Microbiol. 2016;8(1):30989.
- Rôças IN, JF S Jr. Frequency and levels of candidate endodontic pathogens in acute apical abscesses as compared to asymptomatic apical periodontitis. PLoS One. 2018;13(1):e0190469.
- Chen J, Miao X, Xu M, et al. Intra-genomic heterogeneity in 16S rRNA genes in strictly anaerobic clinical isolates from periodontal abscesses. PLoS One. 2015;10(6):e0130265.
- Chen J, Wu X, Zhu D, et al. Microbiota in human periodontal abscess revealed by 16S rDNA sequencing. Front Microbiol. 2019;10:1723.
- Böttger S, Zechel-Gran S, Schmermund D, et al. Microbiome of odontogenic abscesses. Microorganisms. 2021;9(6):1307.
- Riggio MP, Aga H, Murray CA, et al. Identification of bacteria associated with spreading odontogenic infections by 16S rRNA gene sequencing. Oral Surg Oral Med Oral Pathol Oral Radiol Endod. 2007;103(5):610–617.
- Fihman V, Raskine L, Petitpas F, et al. Cervical necrotizing fasciitis: 8-years’ experience of microbiology. Eur J Clin Microbiol Infect Dis. 2008;27(8):691–695.
- Shi H, Yang Z, Li H, et al. Efficacy of needle aspiration in patients with oral-maxillofacial abscesses: a retrospective study of 15 consecutive patients. Am J Otolaryngol. 2022;43(1):103216.
- Aguilar-Durán L, Figueiredo R, Seminago R, et al. A metagenomic study of patients with alveolar osteitis after tooth extraction. A preliminary case-control study. Clin Oral Investig. 2019;23(11):4163–4172.
- Zheng SW, Xu P, Cai LT, et al. The presence of Prevotella melaninogenica within tissue and preliminary study on its role in the pathogenesis of oral lichen planus. Oral Dis. 2021 Mar 29.
- Yang Z, Cui Q, An R, et al. Comparison of microbiomes in ulcerative and normal mucosa of recurrent aphthous stomatitis (RAS)-affected patients. BMC Oral Health. 2020;20(1):128.
- Gopinath D, Menon RK, Wie CC, et al. Salivary bacterial shifts in oral leukoplakia resemble the dysbiotic oral cancer bacteriome. J Oral Microbiol. 2020;13(1):1857998.
- Rusthen S, Kristoffersen AK, Young A, et al. Dysbiotic salivary microbiota in dry mouth and primary Sjögren’s syndrome patients. PLoS One. 2019;14(6):e0218319.
- Alam J, Lee A, Lee J, et al. Dysbiotic oral microbiota and infected salivary glands in Sjögren’s syndrome. PLoS One. 2020;15(3):e0230667.
- Zhang Y, Zhu C, Feng X, et al. Microbiome variations in preschool children with halitosis. Oral Dis. 2021;27(4):1059–1068.
- Ren W, Xun Z, Wang Z, et al. Tongue coating and the salivary microbial communities vary in children with halitosis. Sci Rep. 2016;6(1):24481.
- Riggio MP, Lennon A, Rolph HJ, et al. Molecular identification of bacteria on the tongue dorsum of subjects with and without halitosis. Oral Dis. 2008;14(3):251–258.
- Ye W, Zhang Y, He M, et al. Relationship of tongue coating microbiome on volatile sulfur compounds in healthy and halitosis adults. J Breath Res. 2020;14(1):016005.
- Jo JK, Seo SH, Park SE, et al. Identification of salivary microorganisms and metabolites associated with halitosis. Metabolites. 2021;11(6):362.
- Karpinski TM. Role of oral microbiota in cancer development. Microorganisms. 2019;7(1):20.
- Teles FRF, Alawi F, Castilho RM, et al. Association or causation? Exploring the oral microbiome and cancer links. J Dent Res. 2020;99(13):1411–1424.
- Johnson DE, Burtness B, Leemans CR, et al. Head and neck squamous cell carcinoma. Nat Rev Dis Primers. 2020;6(1):92.
- Hsiao JR, Chang CC, Lee WT, et al. The interplay between oral microbiome, lifestyle factors and genetic polymorphisms in the risk of oral squamous cell carcinoma. Carcinogenesis. 2018;39(6):778–787.
- Yang K, Wang Y, Zhang S, et al. Oral microbiota analysis of tissue pairs and saliva samples from patients with oral squamous cell carcinoma - a pilot study. Front Microbiol. 2021;12:719601.
- Gopinath D, Menon RK, Wie CC, et al. Differences in the bacteriome of swab, saliva, and tissue biopsies in oral cancer. Sci Rep. 2021;11(1):1181.
- Perera M, Al-Hebshi NN, Perera I, et al. Inflammatory bacteriome and oral squamous cell carcinoma. J Dent Res. 2018;97(6):725–732.
- Zhang L, Liu Y, Zheng HJ, et al. The oral microbiota may have influence on oral cancer. Front Cell Infect Microbiol. 2020;9:476.
- Chen MY, Chen JW, Wu LW, et al. Carcinogenesis of male oral submucous fibrosis alters salivary microbiomes. J Dent Res. 2021;100(4):397–405.
- Rai AK, Panda M, Das AK, et al. Dysbiosis of salivary microbiome and cytokines influence oral squamous cell carcinoma through inflammation. Arch Microbiol. 2021;203(1):137–152.
- Kageyama S, Nagao Y, Ma J, et al. Compositional shift of oral microbiota following surgical resection of tongue cancer. Front Cell Infect Microbiol. 2020;10:600884.
- De Martin A, Lütge M, Stanossek Y, et al. Distinct microbial communities colonize tonsillar squamous cell carcinoma. Oncoimmunology. 2021;10(1):1945202.
- Panda M, Rai AK, Rahman T, et al. Alterations of salivary microbial community associated with oropharyngeal and hypopharyngeal squamous cell carcinoma patients. Arch Microbiol. 2020;202(4):785–805.
- Gong H, Shi Y, Zhou X, et al. Microbiota in the throat and risk factors for laryngeal carcinoma. Appl Environ Microbiol. 2014;80(23):7356–7363.
- Dong Z, Zhang C, Zhao Q, et al. Alterations of bacterial communities of vocal cord mucous membrane increases the risk for glottic laryngeal squamous cell carcinoma. J Cancer. 2021;12(13):4049–4063.
- Hsueh C-Y, Gong H, Cong N, et al. Throat microbial community structure and functional changes in postsurgery laryngeal carcinoma patients. Appl Environ Microbiol. 2020;86(24):e01849–20.
- Al Masalma M, Lonjon M, Richet H, et al. Metagenomic analysis of brain abscesses identifies specific bacterial associations. Clin Infect Dis. 2012;54(2):202–210.
- Kommedal Ø, Wilhelmsen MT, Skrede S, et al. Massive parallel sequencing provides new perspectives on bacterial brain abscesses. J Clin Microbiol. 2014;52(6):1990–1997.
- Dyrhovden R, Nygaard RM, Patel R, et al. The bacterial aetiology of pleural empyema. A descriptive and comparative metagenomic study. Clin Microbiol Infect. 2019;25(8):981–986.
- Fernández-Canigia L, Cejas D, Gutkind G, et al. Detection and genetic characterization of beta-lactamases in Prevotella intermedia and Prevotella nigrescens isolated from oral cavity infections and peritonsillar abscesses. Anaerobe. 2015;33:8–13.
- Klug TE, Greve T, Hentze M. Complications of peritonsillar abscess. Ann Clin Microbiol Antimicrob. 2020;19(1):32.
- Bancescu G, Didilescu A, Bancescu A, et al. Antibiotic susceptibility of 33 Prevotella strains isolated from Romanian patients with abscesses in head and neck spaces. Anaerobe. 2015;35:41–44.
- Cobo F, Guillot V, Navarro-Marí JM. Breast abscesses caused by anaerobic microorganisms: clinical and microbiological characteristics. Antibiotics. 2020;9(6):341.
- Cobo F, Rodríguez-Granger J, Sampedro A, et al. Infected breast cyst due to Prevotella buccae resistant to metronidazole. Anaerobe. 2017;48:177–178.
- Guet-Revillet H, Jais JP, Ungeheuer MN, et al. The microbiological landscape of anaerobic infections in hidradenitis suppurativa: a prospective metagenomic study. Clin Infect Dis. 2017;65(2):282–291.
- Ring HC, Sigsgaard V, Thorsen J, et al. The microbiome of tunnels in hidradenitis suppurativa patients. J Eur Acad Dermatol Venereol. 2019;33(9):1775–1780.
- Zou M, Cai Y, Hu P, et al. Analysis of the composition and functions of the microbiome in diabetic foot osteomyelitis based on 16S rRNA and metagenome sequencing technology. Diabetes. 2020;69(11):2423–2439.
- Sakamoto M, Ohkusu K, Masaki T, et al. Prevotella pleuritidis sp. nov., isolated from pleural fluid. Int J Syst Evol Microbiol. 2007;57(8):1725–1728.
- Jayasimhan D, Wu L, Huggan P. Fusobacterial liver abscess: a case report and review of the literature. BMC Infect Dis. 2017;17(1):440.
- Asif AA, Roy M, Ahmad S. Rare case of Prevotella pleuritidis lung abscess. BMJ Case Rep. 2020;13(9):e235960.
- Al Bataineh MT, Dash NR, Elkhazendar M, et al. Revealing oral microbiota composition and functionality associated with heavy cigarette smoking. J Transl Med. 2020;18(1):421.
- Esberg A, Johansson L, Johansson I, et al. Oral microbiota identifies patients in early onset rheumatoid arthritis. Microorganisms. 2021;9(8):1657.
- Corrêa JD, Calderaro DC, Ferreira GA, et al. Subgingival microbiota dysbiosis in systemic lupus erythematosus: association with periodontal status. Microbiome. 2017;5(1):34.
- Alauzet C, Mory F, Carlier JP, et al. Prevotella nanceiensis sp. nov., isolated from human clinical samples. Int J Syst Evol Microbiol. 2007;57(10):2216–2220.
- Ari O, Karabudak S, Kalcioglu MT, et al. The bacteriome of otitis media with effusion: does it originate from the adenoid? Int J Pediatr Otorhinolaryngol. 2019;126:109624.
- Francavilla R, Ercolini D, Piccolo M, et al. Salivary microbiota and metabolome associated with celiac disease. Appl Environ Microbiol. 2014;80(11):3416–3425.
- Buhl M, Marschal M. Prevotella vespertina sp. nov., isolated from an abscess of a hospital patient. Int J Syst Evol Microbiol. 2020;70(8):4576–4582.
- Buhl MEJ, Meier-Kolthoff JP, Marschal M. Prevotella illustrans sp. nov., derived from human oropharyngeal abscess puncture fluid. Int J Syst Evol Microbiol. 2021;71(12).
- Downes J, Sutcliffe IC, Hofstad T, et al. Prevotella bergensis sp. nov., isolated from human infections. Int J Syst Evol Microbiol. 2006;56(3):609–612.
- Glazunova OO, Launay T, Raoult D, et al. Prevotella timonensis sp. nov., isolated from a human breast abscess. Int J Syst Evol Microbiol. 2007;57(4):883–886.
- Aberkane S, Pradel B, Dumont Y, et al. Clinical sources and antimicrobial susceptibility of Prevotella timonensis at the university hospital of Montpellier, France. Anaerobe. 2018;50:19–21.
- Scher JU, Ubeda C, Equinda M, et al. Periodontal disease and the oral microbiota in new-onset rheumatoid arthritis. Arthritis Rheum. 2012;64(10):3083–3094.
- Kroese JM, Brandt BW, Buijs MJ, et al. Differences in the oral microbiome in patients with early rheumatoid arthritis and individuals at risk of rheumatoid arthritis compared to healthy individuals. Arthritis Rheumatol. 2021;73(11):1986–1993.
- Corrêa JD, Fernandes GR, Calderaro DC, et al. Oral microbial dysbiosis linked to worsened periodontal condition in rheumatoid arthritis patients. Sci Rep. 2019;9(1):8379.
- Chen B, Zhao Y, Li S, et al. Variations in oral microbiome profiles in rheumatoid arthritis and osteoarthritis with potential biomarkers for arthritis screening. Sci Rep. 2018;8(1):17126.
- Lehenaff R, Tamashiro R, Nascimento MM, et al. Subgingival microbiome of deep and shallow periodontal sites in patients with rheumatoid arthritis: a pilot study. BMC Oral Health. 2021;21(1):248.
- Sparks Stein P, Steffen MJ, Smith C, et al. Serum antibodies to periodontal pathogens are a risk factor for Alzheimer’s disease. Alzheimers Dement. 2012;8(3):196–203.
- Beydoun MA, Beydoun HA, Hossain S, et al. Clinical and bacterial markers of periodontitis and their association with incident all-cause and Alzheimer’s disease dementia in a large national survey. J Alzheimers Dis. 2020;75(1):157–172.
- Finegold SM, Molitoris D, Song Y, et al. Gastrointestinal microflora studies in late-onset autism. Clin Infect Dis. 2002;35(Suppl 1):S6–S16.
- Finegold SM. State of the art; microbiology in health and disease. Intestinal bacterial flora in autism. Anaerobe. 2011;17:367–368.
- Qiao Y, Wu M, Feng Y, et al. Alterations of oral microbiota distinguish children with autism spectrum disorders from healthy controls. Sci Rep. 2018;8(1):1597.
- Abdulhaq A, Halboub E, Homeida HE, et al. Tongue microbiome in children with autism spectrum disorder. J Oral Microbiol. 2021;13(1):1936434.
- Wingfield B, Lapsley C, McDowell A, et al. Variations in the oral microbiome are associated with depression in young adults. Sci Rep. 2021;11(1):15009.
- Yolken R, Prandovszky E, Severance EG, et al. The oropharyngeal microbiome is altered in individuals with schizophrenia and mania. Schizophr Res. 2021;234:51–57.
- Chang Y, Woo HG, Jeong JH, et al. Microbiota dysbiosis and functional outcome in acute ischemic stroke patients. Sci Rep. 2021;11(1):10977.
- Pyysalo MJ, Pyysalo LM, Pessi T, et al. Bacterial DNA findings in ruptured and unruptured intracranial aneurysms. Acta Odontol Scand. 2016;74(4):315–320.
- Park HR, Chang J, Kim S, et al. First case of intracranial mycotic aneurysm caused by Prevotella intermedia associated with chronic sinusitis in a Korean adult. Case Rep Neurol. 2020;12(1):121–126.
- Sandoe JA, Struthers JK, Brazier JS. Subdural empyema caused by Prevotella loescheii with reduced susceptibility to metronidazole. J Antimicrob Chemother. 2001;47(3):366–367.
- Piccolo M, De Angelis M, Lauriero G, et al. Salivary microbiota associated with immunoglobulin A nephropathy. Microb Ecol. 2015;70:557–565.
- Downes J, Sutcliffe I, Tanner ACR, et al. Prevotella marshii sp. nov. and Prevotella baroniae sp. nov., isolated from the human oral cavity. Int J Syst Evol Microbiol. 2005;55(4):1551–1555.
- Böttger S, Zechel-Gran S, Schmermund D, et al. Clinical relevance of the microbiome in odontogenic abscesses. Biology (Basel). 2021;10(9):916.
- Boyanova L, Kolarov R, Gergova G, et al. Trends in antibiotic resistance in Prevotella species from patients of the University Hospital of Maxillofacial Surgery, Sofia, Bulgaria, in 2003–2009. Anaerobe. 2010;16(5):489–492.
- van Winkelhoff AJ, Winkel EG, Barendregt D, et al. Beta-lactamase producing bacteria in adult periodontitis. J Clin Periodontol. 1997;24(8):538–543.
- Lareyre F, Cohen C, Declemy S, et al. A fatal aortic arch rupture due to descending necrotizing mediastinitis in a 24-year-old woman. Vasc Endovascular Surg. 2017;51(6):408–412.
- Montagner F, Jacinto RC, Correa Signoretti FG, et al. Beta-lactamic resistance profiles in Porphyromonas, Prevotella, and Parvimonas species isolated from acute endodontic infections. J Endod. 2014;40(3):339–344.
- Willems A, Collins MD. 16S rRNA gene similarities indicate that Hallella seregens (Moore and Moore) and Mitsuokella dentalis (Haapasalo et al.) are genealogically highly related and are members of the genus Prevotella: emended description of the genus Prevotella (Shah and Collins) and description of Prevotella dentalis comb. nov. Int J Syst Bacteriol. 1995;45(4):832–836.
- Zheng H, Xu L, Wang Z, et al. Subgingival microbiome in patients with healthy and ailing dental implants. Sci Rep. 2015;5(1):10948.
- Moore LV, Johnson JL, Moore WE. Descriptions of Prevotella tannerae sp. nov. and Prevotella enoeca sp. nov. from the human gingival crevice and emendation of the description of Prevotella zoogleoformans. Int J Syst Bacteriol. 1994;44(4):599–602.
- Li X, Du H, Song Z, et al. Polymicrobial anaerobic meningitis detected by next-generation sequencing: case report and review of the literature. Front Med (Lausanne). 2022;9:840910.
- Downes J, Wade WG. Prevotella fusca sp. nov. and Prevotella scopos sp. nov., isolated from the human oral cavity. Int J Syst Evol Microbiol. 2011;61(4):854–858.
- Downes J, Hooper SJ, Wilson MJ, et al. Prevotella histicola sp. nov., isolated from the human oral cavity. Int J Syst Evol Microbiol. 2008;58(8):1788–1791.
- Pietrobon G, Tagliabue M, Stringa LM, et al. Leukoplakia in the oral cavity and oral microbiota: a comprehensive review. Cancers (Basel). 2021;13(17):4439.
- Marqués A F, Maestre Vera JR, Mateo Maestre M, et al. Septic arthritis of the knee due to Prevotella loescheii following tooth extraction. Med Oral Patol Oral Cir Bucal. 2008;13(8):E505–507.
- Steingruber I, Bach CM, Czermak B, et al. Infection of a total Hip arthroplasty with Prevotella loescheii. Clin Orthop Relat Res. 2004;418:222–224.
- Downes J, Sutcliffe IC, Booth V, et al. Prevotella maculosa sp. nov., isolated from the human oral cavity. Int J Syst Evol Microbiol. 2007;57(12):2936–2939.
- Hsiao WW, Li KL, Liu Z, et al. Microbial transformation from normal oral microbiota to acute endodontic infections. BMC Genomics. 2012;13(1):345.
- Downes J, Liu M, Könönen E, et al. Prevotella micans sp. nov., isolated from the human oral cavity. Int J Syst Evol Microbiol. 2009;59(4):771–774.
- Sakamoto M, Huang Y, Umeda M, et al. Prevotella multiformis sp. nov., isolated from human subgingival plaque. Int J Syst Evol Microbiol. 2005;55(2):815–819.
- Buonavoglia A, Lanave G, Camero M, et al. Next-generation sequencing analysis of root canal microbiota associated with a severe endodontic-periodontal lesion. Diagnostics (Basel). 2021;11(8):1461.
- Sakamoto M, Umeda M, Ishikawa I, et al. Prevotella multisaccharivorax sp. nov., isolated from human subgingival plaque. Int J Syst Evol Microbiol. 2005;55(5):1839–1843.
- Downes J, Tanner ACR, Dewhirst FE, et al. Prevotella saccharolytica sp. nov., isolated from the human oral cavity. Int J Syst Evol Microbiol. 2010;60:2458–2461.
- Sakamoto M, Suzuki M, Huang Y, et al. Prevotella shahii sp. nov. and Prevotella salivae sp. nov., isolated from the human oral cavity. Int J Syst Evol Microbiol. 2004;54(3):877–883.