ABSTRACT
Extracellular vesicles (EVs) deliver bioactive macromolecules (i.e. proteins, lipids and nucleic acids) for intercellular communication in multicellular organisms. EVs are secreted by all cell types including immune cells. Immune cell-derived EVs modulate diverse aspects of the immune system to either enhance or suppress immune activities. The extensive effects of immune cell-derived EVs have become the focus of great interest for various nano-biomedical applications, ranging from the medical use of nanoplatform-based diagnostic agents to the development of therapeutic interventions as well as vaccine applications, and thus may be ideal for ‘immune-theranostic’. Here, we review the latest advances concerning the biological roles of immune cell-derived EVs in innate and acquired immunity. The intercellular communication amongst immune cells through their EVs is highlighted, showing that all immune cell-derived EVs have their unique function(s) in immunity through intricate interaction(s). Natural-killer (NK) cell-derived EVs, for example, contain potent cytotoxic proteins and induce apoptosis to targeted cancer cells. On the other hand, cancer cell-derived EVs bearing NK ligands may evade immune surveillance and responses. Finally, we discuss possible medical uses for the immune cell-derived EVs as a tool for immune-theranostic: as diagnostic biomarkers, for use in therapeutic interventions and for vaccination.
Introduction
Cells secrete extracellular vesicles (EVs) in vivo as well as in vitro. These vesicles are heterogeneous in size and originate from within the cell. In vivo, EVs are found in blood, urine, amniotic fluid, breast milk, seminal fluid, saliva and malignant effusions [Citation1,Citation2]. The EVs may be called exosomes, microvesicles (MVs), apoptotic bodies and nano-sized membrane vesicles, and they are excreted into the conditioned media by different cell cultures [Citation1,Citation2]. Owing to their diversified sources and functions, EVs have also been called ectosomes, oncosomes, shedding microvesicles, microparticles, etc. [Citation3] – all different terms with the same meaning, thereby, causing confusion. Nevertheless, two generic terms, exosomes and microvesicles, are currently the most commonly used in this field ()).
Figure 1. Biogenesis and molecular composition of a typical extracellular vesicle. (A) Extracellular vesicles may be classified into two major groups: microvesicles and exosomes, depending on their biogenesis pathways. (B) General composition including genetic materials, proteins and lipids in a typical EV. The proteins in EVs include transmembrane or lipid-bound extracellular proteins (e.g. tetraspanins, cell adhesion molecules), cytosolic proteins (e.g. MVE formation, signal transduction), intracellular proteins (e.g. HSP90), extracellular proteins (e.g. soluble secreted proteins, fibronectin), etc. EVs also contain cell-type specific components depending on the nature of the producer cell. MVE: multivesicular endosome.
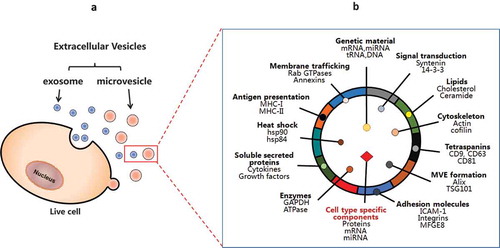
Exosomes are generated through fusion with the plasma membrane of specific endosomal compartments called ‘multivesicular bodies’ (MVB). Many reports have shown several traffic components, such as Tsg101, Alix and tetraspanins (CD63, CD9, CD81), that are enriched in exosomes; thus, it may be suggested that these distinctions can serve as exosomal markers [Citation4–Citation7]. Of note, a subpopulation of small EVs derived from plasma membrane may also contain CD9, CD63 and CD81. Thus, these molecules, not specific to exosomes, are considered as more generic EV markers of different subtypes [Citation8]. On the other hand, microvesicles (MV) are formed via an outward budding from the plasma membrane. Unlike exosomes, the molecular composition of microvesicles is less defined. Depending on the cell types, microvesicles seem to be enriched in matrix metalloproteinases (MMPs), glycoproteins, (e.g. GPIb, GPIIb-IIIa and P-selectin and integrins, etc.), and Mac-1; therefore they have been utilized as the markers for microvesicles [Citation9–Citation13]. Further differentiation between these two types of vesicles – exosomes and MV – is difficult because of their similarity in size and density. In general, EV is an acceptable term that encompasses all classes of secreted lipid membrane vesicles ()).
All EVs share a characteristic composition of biomolecules: proteins, lipids (e.g. cholesterol, ceramide) [Citation14–Citation16], and genetic material (e.g. mRNA, miRNA, tRNA and DNA) and low-molecular-weight metabolites, i.e. amino acids, ATP, glutathione, etc. [Citation17–Citation22]. ()). Their lipid and protein compositions have been extensively analysed by various techniques, including: Western blotting, FACS, immuno-EM and mass spectrometry [Citation23]. Databases containing proteins already detected in MVs are available: such as Vesiclepedia (http://www.microvesicles.org/), ExoCarta (http://exocarta.ludwig.edu.au/) and the Urinary Exosomes Protein Database (http://dir.nhlbi.nih.gov/papers/lkem/exosome/). EV proteomes include both a common set of membranes and cytosolic proteins and specific subsets of proteins, likely correlated to cell-type-associated functions. The latter group may be important in terms of their unique roles in organisms. Any given EV isolate is expected to contain four categories of proteins to define EVs and their functions, based on the recommendation of the International Society for Extracellular Vesicles [Citation5]. (1) The first group is transmembrane or lipid-bound extracellular proteins, which are enriched in EV isolates. These proteins include tetraspanins (CD9, CD63, CD81), integrins, cell adhesion molecules, growth factor receptors, heterotrimeric G proteins (GNA) and phosphatidylserine-binding MFGE8/lactadherin, etc. (2) The second group is cytosolic proteins with membrane- or receptor-binding capacity, which are also enriched in EV isolates, e.g. multivesicular endosome formation (Tsg101, annexins, Rabs), signal transduction or scaffolding proteins (syntenin). (3) The third group is intracellular proteins associated with compartments other than plasma membrane or endosomes that are absent or under-represented in EVs, such as endoplasmic reticulum proteins (HSP90B1, calnexin), Golgi components (GM130), mitochondria proteins (cytochrome C) and argonaute/RISC complex (AGO). (4) The fourth category is extracellular proteins that bind specifically or non-specifically to membranes and co-isolate with EVs, variable association with EVs, e.g. acetylcholinesterase (AChE), serum albumin, extracellular matrix proteins (fibronectin, collagen) and soluble secreted proteins (cytokines, growth factors, matrix metalloproteinases) [Citation5]. EVs also contain cell-type-specific components depending on the nature of the producer cell [Citation24]. For example, EVs from tumour cells contain tumour antigens, platelet-derived exosomes contain coagulation factors, and exosomes from dendritic cells express MHC-II-peptide complexes [Citation25].
In addition to the protein components, EVs contain a specific repertoire of microRNAs (miRNAs), long non-coding RNAs (lncRNAs) and mRNAs distinct from that of their cell of origin [Citation17,Citation26]. It has been demonstrated that embryonic stem-cell-derived microvesicles can deliver Wnt-3 mRNA, which can be translated into the corresponding proteins in target cells [Citation27]. Also, EVs can functionally transfer mRNA and microRNAs from one cell to another as a novel mechanism of genetic exchange between cells [Citation17]. These seminal studies, followed by the increasing number of publications reporting that miRNAs are differentially expressed in the blood of cancer patients compared with matched healthy individuals, inspired the hypothesis that miRNAs, inside EVs, could elicit ‘hormone-like’ functions within the tumour microenvironment. Toll-like receptor 8 (TLR8) has been identified as the first miRceptor (defined as a receptor for miRNAs) in Tumour-Associated Macrophages (TAMs) [Citation28]. This study identifies a completely new mechanism of action for miRNAs, as ligands and agonists of a receptor, triggering a pro-tumoral, inflammatory response [Citation29]. More recently, it has also been shown that miR-21, secreted by neuroblastoma cells in EVs, is taken up by TAMs and binds to TLR8 within TAM endosomes, triggering the NF-κB signalling pathway that transactivates miR-155 expression in TAMs and in EV secreted by TAMs. TAM-derived EVs transfer miR-155 back to neuroblastoma cells, where it targets TERF1, leading to increased resistance to cisplatin [Citation26]. More recently, it has been reported that prostate-cancer-derived exosomes are enriched for lncRNAs harbouring miRNA seed regions (especially for let-7 family members) and RNA-binding protein-binding motifs [Citation30]. This intriguing finding suggests a possible role for these non-coding RNAs in prostate carcinogenesis. While we are witnessing a blossoming of studies assessing the role of extracellular RNA (including RNA in EVs) as cancer biomarkers and as functional molecules within the tumour microenvironment, increasing caution is raised on the possible confounding effect of RNA in cell-culture-medium components such as fetal bovine serum (FBS) [Citation31]. This important point needs to be taken into consideration for a correct interpretation of the results of experiments with extracellular RNA. EVs can also transport genomic (gDNA) and mitochondrial DNA (mtDNA), ranging in size from 100 base pairs to 2.5 kilobase pairs, to recipient cells [Citation32]. The transferred DNA from EVs to cells not only increases the gDNA-coding mRNA and protein levels, but also induces function in recipient cells [Citation33]. It has been shown that transferred BCR/ABL DNA from leukaemia K562 EVs causes chronic myeloid leukaemia in animal studies [Citation34]. Tumour EVs carrying DNA, which reflects the genetic status of the disease, are potentially used as blood biomarkers for cancer [Citation35]. Cytotoxic agent ciprofloxacin induces Jurkat cells to release EVs with both gDNA and mtDNA associated with fibronectin on the EV surface [Citation36]. This EV-associated DNA could be a likely self-antigen, leading to an anti-DNA antibody response. Also, irinotecan (CPT-11) induces the release of intestinal DNA through the EV secretion, which triggers innate immune responses causing intestinal toxicity, the incidence of drug-induced diarrhoea [Citation37]. Thus, EVs transferring DNA can have both physiological and pathological impacts on recipient cells. Collectively, secreted EVs contain a wide variety of functional components. Owing to the complexity, it is quite intractable to study EVs’ compositions and functions. A recent report about methodological guidelines is practically useful to avoid many pitfalls and misconception in this field[Citation38].
All cell types are known to secrete EVs, including immune cells such as dendritic cells (DCs) [Citation39], mast cells (MCs) [Citation40], macrophages [Citation41,Citation42], B lymphocytes [Citation43] and T lymphocytes [Citation44,Citation45]. This allows intercellular communication in the bloodstream and lymph fluids, which in turn can exert numerous immune functions in physiology and pathology [Citation46–Citation48]. The impact of EVs’ biological functions on the immune system is now being recognized. In this review, we will focus on the current understanding of immune cell-derived EVs.
Current understanding of immune cell-derived EVs and their potential effects
Vertebrates have two immune response systems – the innate immune system and the acquired immune system. The innate immune system has been greatly advanced in all multicellular organisms, but the acquired immune system is only manifested in vertebrates [Citation49]. Natural-killer (NK) cells are an essential part of innate immunity, while B and T cells are the two necessary types of cells in the acquired immune system. B cells can recognize foreign antigens, but T cells require antigen-presenting cells (APCs) to identify the antigens. The professional APCs are dendritic cells (DCs), macrophages and B cells. APCs internalize foreign antigens, load these foreign antigens to MHC-I and MHC-II, respectively, and present them to CD8+ and CD4+ T cells for the T cell immune responses [Citation50]. Numerous reports have demonstrated that the cross-talk amongst immune cells is through interleukins, chemokines, interferons and other signalling molecules [Citation51,Citation52]. Recent studies have also shown that immune cells can secrete the immune active EVs that affect many physiological and pathological processes. These immune active EVs play important roles in innate and acquired immune responses, including antigen presentation, NK and T cell activations, T cell polarization toward Treg cells, immune suppression and anti-inflammatory effects. Therefore, the immune cell-derived EVs all take part in immune responses and regulations, and may potentially serve as a tool or target with great applications [Citation46].
Roles of immune cell-derived EVs in innate immunity
Immune cell-derived EVs are involved in the regulation of the innate immune responses. EVs released by NK cells, macrophages and dendritic cells have been described mainly as pro-inflammatory mediators via paracrine messengers acting on the innate immune system [Citation53].
NK cells, group 1 innate lymphoid cells, have multiple inhibitory receptors [e.g. killer immunoglobulin-like receptors (KIR)] and activating receptors (e.g. NKG2D), and the balance of these activating and inhibitory signals determines whether NK cells are activated or not. HLA-B-associated transcript 3 (BAT3), TNF superfamily members (TNF, TRAIL, Fas ligand (FasL)) and IL-15Ra expressed in DC-derived EVs, can bind directly to NK cell surface receptors (NKG2D receptor, TNF superfamily receptors, IL-15Ra receptor) to activate NK cells and enhance the cytotoxic activity of NK cells [Citation54–Citation57] (.①). Furthermore, human NK cells may release EVs that are cytotoxic against tumour cells and activated immune cells [Citation58,Citation59].
Figure 2. Immune cell-derived EVs modulate innate and acquired immune responses. Innate and acquired immune cells may communicate through released EVs by different ways, e.g. ① receptor–ligand interaction, ② pro-inflammatory mediators and cytokines, ③ anti-inflammatory mediators and cytokines, ④ direct antigen presentation, ⑤ cross-dressing and cross-presentation, ⑥ MC-EVs activate iDC and TC, ⑦ MØ-EVs activate APCs-mediated TC, ⑧ TC-EVs induce immune regulatory effects, ⑨ iDC-EVs induce immune suppression and ⑩ Treg-EVs induce immune suppression. The green/blue arrows indicate that secreted EVs exert a positive immune response(s) to targets, and red arrows indicate that EVs suppress or block the immune activities. NK, natural-killer cells; PN, polymorphonuclear neutrophils; mDC, mature dendritic cells; iDC, immature dendritic cells; APC, antigen-presenting cells; BC, B cells; TC, T cells; MC, mast cells; MØ, macrophages; Treg cell, regulatory T cells; EVs, extracellular vesicles; AID, autoimmune disease.
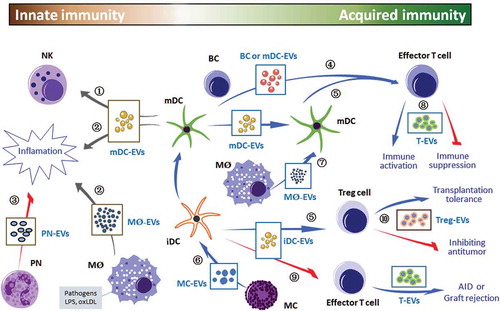
Macrophage-derived EVs can exert multiple functions. They could induce macrophage differentiation. Also, macrophage-derived EVs contain high levels of miR-223, which modulates the differentiation and proliferation of myeloid cells [Citation60]. The macrophages that are infected with Toxoplasma or Mycobacterium tuberculosis release pathogen-associated-molecular patterns-containing EVs to induce immune cell recruitment and pro-inflammatory cytokine secretion. The secreted pro-inflammatory cytokines include RANTES and TNF-α [Citation61,Citation62]. Moreover, intranasal injection of mice with EVs released by Mycobacterium tuberculosis-infected macrophages led to increased secretion of pro-inflammatory cytokine mediators (TNF-α and IL-12) and the recruitment of neutrophils and macrophages into the lungs of mice [Citation63]. Lipopolysaccharide (LPS)-stimulated macrophages secrete EVs, including the upregulated cytokines and miRNAs, which promote the occurrence of inflammation. In the innate and acquired immune responses, the high-throughput transcript sequencing analysis showed significant alteration of RNA species in EVs. The EVs secreted by LPS-stimulated macrophages sufficiently activate NF-κB pathways in naive immune cells, leading to the occurrence of inflammation [Citation41]. In the presence of oxidized low-density lipoprotein (oxLDL)-conjugated immune complex, macrophages release the EVs with increased expression of IL-1β, phosphatase and Hsp70, and these EVs can promote the formations of atherosclerotic plaques [Citation64] (.②). It has been reported that endotoxin-stimulated monocytes can release EVs that deliver a cell-death message via encapsulated caspase-1 [Citation65]. It is now clear that a multiple protein complex, inflammasome, is responsible for initiation of the inflammatory process through the activation of caspase-1 and the production of the pro-inflammatory cytokines IL-1β and IL-18 [Citation66]. Inflammasome-containing EVs from stimulated macrophages specifically enrich immune response-related proteins and signalling pathways. They can directly trigger the NF-κB signalling pathway, and subsequently amplify inflammatory signalling in neighbour cells [Citation67]. Thus, inflammasome-containing EVs play a vital role in activating the innate immune response in peripheral tissues.
Besides promoting inflammation, EVs released by innate immune cells also play a role in innate immunosuppressive effects. For example, activated polymorphonuclear neutrophils spread anti-inflammatory EVs by regulating endogenous pro-resolving lipid mediators or by inducing TGF-β secretion [Citation68] (.③).
Roles of immune cell-derived EVs in acquired immunity
More evidence has indicated that immune cell-derived EVs are involved in the acquired immune response. In the process of antigen presentation, APCs (such as DC or macrophages) deliver MHC-I and MHC-II molecules and directly stimulate the naive CD8+ and CD4+ T cells, respectively. Recent evidence has substantiated the involvement of EVs in the process of antigen presentation, i.e. the immune cell-derived EVs express MHC-I, MHC-II and T cell co-stimulatory molecules on their surfaces [Citation1], and these EVs can deliver the foreign antigens. One example is that macrophages infected with Mycobacterium tuberculosis release bacterial antigen-containing EVs [Citation69,Citation70]. Therefore, immunologists have utilized immune cell-derived EVs as media in antigen presentation and widely regarded this as an important mechanism of antigen presentation [Citation69,Citation70].
Ample evidence has indicated that APCs need to capture EVs during antigen presentation. Adhesion molecules and integrins on EVs and their lipid content may facilitate attachment and fusion with the plasma membrane of APCs [Citation53]. For example, mouse plasmacytoid DCs, which express Siglec-H, may capture EVs in vivo [Citation20]. Likewise, mature DCs (mDCs), which express Siglec-1 receptor, can catch Jurkat cell-derived EVs, and the capture was mostly inhibited by the blocking Siglec-1, a sugar-binding lectin [Citation71]. In general, EVs captured by APCs may convey stimulatory or suppressive signals to target cells (e.g. T cells) and contribute to antigen presentation. At present, there are three possible mechanisms bringing about immune cell-derived EVs mediated antigen presentation to T cells.
EV-mediated direct antigen presentation
APC-released EVs deliver MHC-I, MHC-II and T cell co-stimulatory molecules, which can directly activate CD8+ and CD4+ T cells in vitro [Citation72–Citation76]. Similarly, the DCs in ovalbumin (OVA)-treated mice release OVA-containing EVs, which are able to directly stimulate OVA specific CD8+ T cell lines [Citation72] (.④). Studies have shown that the EVs released by LPS-treated DCs can induce the activation of antigen-specific T cells both in vivo and in vitro [Citation77] (.④). In addition, the EVs secreted by monocyte-derived DCs contain viral antigen, which can activate T cells in vitro in the absence of DCs [Citation75] (.④). Moreover, B cell line released EVs can directly stimulate CD4+ T cell lines [Citation78] (.④). The direct effects of EVs on T cells, however, may not be the main mechanism underlying the activation of naive T cells in vivo. Studies have shown that the stimulatory activity of EVs alone on T cells is 10- to 20-fold lower than in the presence of APCs [Citation79].
Cross-dressing antigen presentation
Immune cell-derived EVs (e.g. DC-secreted EVs) contain antigen–MHC complexes [Citation80]. Following the capture and internalization, these antigen–MHC complexes are presented to T cells by APCs. This process is termed ‘cross-dressing’, indicating that the EVs can transfer antigen–MHC complexes between immune cells [Citation80–Citation83] (.⑤). However, some studies have shown that antigen–MHC complexes bearing EVs are unable to perform the ‘cross-dressing’ process [Citation84,Citation85]. Thus, further studies of this mechanism are required.
Cross-presentation
EV-transported antigens form a complex with MHC via APCs and present antigen peptide to T cells. In the presence of naive DCs, antigen-bearing EVs stimulate T cells to promote the activation of T cells [Citation86–Citation88]. DCs capture antigen-bearing EVs, which are subsequently presented to the internal MHC-I and MHC-II molecules, and then activate antigen-specific T cells [Citation80,Citation89] (.⑤). Mast cell-derived EVs in mice deliver antigens and activate naive DCs, thereby activating T cells in vitro [Citation90] (.⑥). Studies have shown that mast cell-derived OVA-bearing EVs can activate both DCs and OVA-specific T cell lines [Citation91] (.⑥). The EVs secreted by Mycobaterium tuberculosis-infected macrophages contain bacterial antigens that can activate APCs-mediated CD4+ and CD8+ T cells in mycobacterium-sensitive mice [Citation69] (.⑦). These mechanisms require the capture of EVs by receptor cells, and the process entails the interaction of receptors with ligands. Certain surface ligands and adhesion molecules have been shown to be involved in the process of capture, such as tetraspanins, ICAM-1 and phosphatidylserine [Citation77,Citation92,Citation93].
Besides been involved in the process of antigen cross-presentation, mast cell-derived EVs also take part in transferring specific cargo to recipient cells and mediate distinct effects [Citation40,Citation94]. For example, mast-cell-derived EVs transfer proteins (such as CD13, ribosomal protein S6 kinase, annexin V, CDC25, etc.) to splenocytes and T cells, and contribute to the immunostimulatory activities [Citation95]. Similarly, they may transfer OX40L ligand to promote the proliferation and differentiation of naive CD4+ cells to Th2 cells [Citation96]. Further, mast-cell-derived EVs are able to induce plasminogen activator inhibitor type 1 (PAI-1) expression in endothelial cells in vitro and promote the deposition of fibrin during the inflammation [Citation97]. Another example was that EVs from MCs transferred the CD117 (Kit protein) to adenocarcinoma cells, induced PI3K/AKT signalling and promoted migration and proliferation of cancer cells [Citation98].
T-cell-derived EVs can target many cell types, inducing a wide variety of immune-response effects ranging from immune activation to suppression [Citation99] (.⑧). Activated T-cell-secreted EVs enhance the immune response through action on autologous resting T cells [Citation23]. The EVs secreted by stimulated human CD3+ T cells work synergistically with IL-2 to promote the proliferation of autologous resting cells. Likewise, T cell-derived EVs are required for RANTES (CCL5)-dependent induction of T cell proliferation, support immunogenicity via gene regulation in targeted APCs [Citation18] and take part in IL-2 mediated immune-response signalling [Citation100]. In addition, T-cell-secreted EVs can activate MCs resulting in cell degranulation and induction of IL-8 and IL-24 [Citation101,Citation102]. The EVs secreted by activated T cells contain superfamily members TNF (FasL), which promotes tumour invasion in the lungs by increasing the expression of metalloproteinase matrix 9 [Citation103]. The EVs released by CTLs contain FasL, which can kill the target cells [Citation104]. Therefore, T-cell-secreted EVs are an important mediator of the immune responses that regulate the activity of immune cells and other cells.
Immune cell-derived EVs have immune-promotive as well as immunosuppressive effects, and immunosuppressive effects from EVs may also lead to immune tolerance. Immune tolerance is classified into central tolerance or peripheral tolerance depending on where the state is originally induced. Thymus and bone marrow induce central immune tolerance, and lymph nodes and other tissues induce peripheral immune tolerance. Recent research showed that human thymic EVs exhibit thymus-specific features including protein content, surface markers and microRNA profile. These thymic EVs play a role in T-cell selection and the induction of central tolerance [Citation105]. The presentation of tissue-restricted antigens (TRAs) in the thymic micromileus is vital to establish central tolerance. Subsequent studies showed that thymic epithelial cells (TECs) could produce thymic EVs bringing TRAs [Citation106] and MHC molecules [Citation107]. The thymic EVs from TECs become involved in the thymocyte negative selection process by delivering intact functional peptide–MHC complexes to APCs, such as thymic DCs, and induce central tolerance [Citation108].
Besides the central tolerance, immune cell-derived EVs also have peripheral immune tolerance effects. For example, the EVs secreted by immature or genetically modified or IL-10-treated DCs have been shown to induce immune suppression in transplanted model mice and in mice with autoimmune disease [Citation46,Citation109–Citation111] (.⑨). The expression of co-stimulatory molecules in the immature DCs had decreased, indicating the immunosuppressive effect of the EVs released by the immature DCs [Citation104]. Activated T-cell-secreted EVs expressing NKG2D, TCR, MHC, APO2 ligand, FasL and others are able to block NK cytotoxicity, inhibit T-cell stimulation, induce T-cell apoptosis and downregulate the T-cell stimulatory capacity of APCs, thus diminishing immune responses [Citation112–Citation115]. The presence of T-cell tolerance in mice with allergic dermatitis is attributed to the release of the EVs released by inhibitory CD8+ T cells [Citation116,Citation117]. Moreover, CD73 of T regulatory cell-derived EVs contributed to the suppressive role [Citation118].
EVs secreted from certain subtypes of T cells may participate in immunosuppression of anti-tumour immunity against tumours. For example, the EVs released by CD4+ T cells inhibited CD8+ cytotoxic T lymphocyte response and anti-tumour activity against OVA-expressing B16 melanoma [Citation44] (.⑩). Activated CD8+ T cell-derived FasL-expressed EVs could promote the invasion of murine melanoma cell line B16 and Lewis lung cancer cell line via Fas signalling pathway [Citation103]. Similarly, CD8+ CD25+ regulatory T cell-released EVs can suppress CD8+ T-cell-mediated cytotoxicity [Citation119]. EVs released by CD4+ CD25+ regulatory T cells have also been shown to inhibit the proliferation of T cells and prolong the survival time in a rat model, indicating the contributory effect of T-cell-secreted EVs on transplantation tolerance [Citation45] (.⑩). Therefore, certain EVs derived from immune cells may elicit immunosuppression against tumours, and this effect may be a potential target for the design of cancer immunotherapy [Citation120,Citation121].
Collectively, immune cell-derived EVs are involved in many aspects of innate and acquired immune responses. They may exert their functions alone by direct interaction with recipient cells, or may associate with cytokines and chemokines (such as TNF-α, IL-1ra, G-CSF, CCL2), possibly eliciting synergistic actions to regulate immune activities [Citation53]. As such, immune cell-derived EVs can mediate immune activation or immunosuppression, so as to promote inflammatory, autoimmune and other pathologic processes. The regulatory effect of immune cells-derived EVs has potential applications in the treatment of immune-mediated diseases, such as inflammation and graft rejection [Citation117,Citation122,Citation123].
Natural-killer cell-derived EVs (NK EVs)
NK cells play an important role in immuno-surveillance and host defence against cancer and pathogen infections [Citation124]. NK cells express a variety of germline-encoded activating and inhibitory cell surface receptors [Citation125]. The activation and/or inhibition signals are generated at the specialized immunological synapse formed between the NK and the target cell through the surface receptors and utilizing their cytotoxic ability to eliminate abnormal cells [Citation124]. The way that NK cells destroy target cells is to mobilize cytotoxic effector molecules stored in lytic granules upon stimulation [Citation126–Citation128]. The lytic granules have often been referred to as ‘secretory lysosomes’ because they have the characteristics of lysosomes. They are generated in the cytoplasm and released as a cargo of cytotoxic proteins, including perforin, granzymes, granulysin, FasL/CD178, TNF-related apoptosis-inducing ligand (TRAIL/CD253) and small anti-microbial peptides [Citation124]. Interestingly, human NK cells can release EVs in culture medium. The NK-derived EVs also contain typical NK markers and lytic proteins [Citation58,Citation59]. The relation between lytic granules/secretory lysosomes of NK cells and secreted NK EVs is intriguing, but needs to be further explored to fully understand their relation. Thus, isolation and characterization of NK EVs would provide key information to define their functions in immunity.
Characteristics of NK EVs
NK EVs have been isolated from NK92 cell culture [Citation129] and PBMC [Citation58]. Furthermore, a large-scale method to isolate NK EVs from ex vivo expansion of activated NK cell culture has been developed [Citation59]. This large-scale isolation of activated NK EVs might lead to new strategies for clinical exploitation. Isolated NK EVs are cytotoxic for cell lines derived from haematologic malignancies (Jurkat, K562, DAUDI) and a solid tumour (breast carcinoma SKBR3) [Citation58]. Activated NK EVs from ex vivo expansion cultures show cytotoxicity to other tested cancer cell lines including ALL (SupB15, NALM-6), neuroblastoma (CHLA-136, CHLA-255) and breast carcinoma (MCF7) [Citation59]. The degradation product of the common caspase substrate, PARP, could be detected in targeted cancer cell lines shortly after the NK EVs treatment. The pan-caspase inhibitor significantly reduced cytotoxicity. Furthermore, inhibitors of the apoptosis initiation group (caspase-2, -8, -9 and -10) effectively blocked EV-induced cytotoxicity, as did the inhibitors of caspase-3 (apoptosis execution group) and, to a lesser extent, those of caspase-6 and -12. Cleaved caspase-3, -7, -9 protein products can also be observed in the targeted cancer cells. Inhibitors of the inflammatory activation group (caspase-1, -4 and -13) had no detectable effect on cytotoxicity. The results suggest that the caspase members in the initiation and execution groups are involved, at least in part, in NK EV-mediated killing of cancer cells [Citation59].
One unique feature of isolated NK EVs is that they contain key cytotoxic proteins, such as perforin (PFN), granzyme A (Gzm-A), granzyme B (Gzm-B) and granulysin (GNLY). It is well known that human NK cells secrete lytic granules upon activation. The lytic granules essentially utilize these cytotoxic molecules as the major mechanisms for their cellular cytotoxicity [Citation130–Citation135]. This may be extrapolated to NK EV killing mechanisms (), which can be classified into three groups.
Figure 3. Mechanisms of NK-cell derived EVs interacting with target cells. NK cell-derived EVs express molecules involved in cytotoxicity: perforin-granzyme mediated fusion, receptor–ligand mediated reaction, granulysin mediated electrostatic interaction. The cytotoxic proteins of NK EVs may rely on the three mechanisms to activate the caspase cascade, and induce cell apoptosis. PFN, perforin; GzmA, granzyme A; GzmB, granzyme B; GNLY, granulysin; DISC, death-inducing signalling complex; BID, BH3-interacting domain.
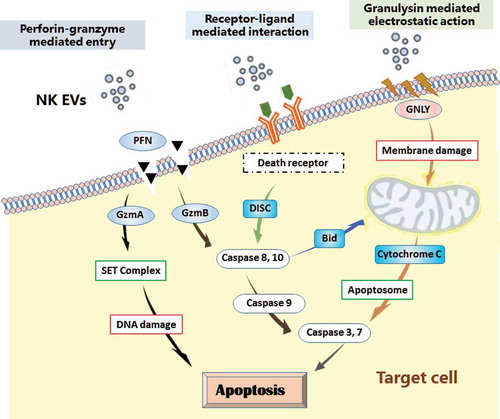
1) Perforin-granzyme mediated entry
Perforin is a pore-forming protein that allows delivery of the pro-apoptotic proteins into the cytoplasm of the target cells, subsequently inducing target cell death by apoptosis [Citation134,Citation135]. Perforin in lytic granules (pH ~5) is inactive. It is associated with its chaperone calreticulum, a Ca2+-binding protein that protects CTL from auto-digestion during biogenesis of the granules. Perforin becomes active in the synapse (pH ~7 and in the presence of calcium), oligomerizing with ~20 molecules into a large polyperforin pore complex [Citation136,Citation137]. NK EV-associated perforin may follow the same path to insert itself into a plasma membrane and generate pores so as to release cytotoxic proteins into the cytoplasm of target cells [Citation109]. In addition, following endocytosis, perforin may form pores on the endosomal membrane, releasing granzyme B into the target cell [Citation138]. In either case, NK EV-associated perforin and granzymes may activate upon fusion of NK EVs with the plasma or endosomal membrane of target cells, subsequently inducing apoptosis through caspase-dependent and -independent manners.
Granzyme A is a tryptase that induces caspase-independent cell death through histone digestion and by facilitating endogenous DNase access to DNA during granule-mediated apoptosis. Granzyme A can directly target the SET complex in ER for proteolysis. Proteolysis of SET releases the inhibition of DNase NM23-H1, resulting in the single-strand DNA nicks most commonly associated with granzyme A-mediated cell death [Citation139,Citation140]. Granzyme A can also target the mitochondria, leading to loss of mitochondrial transmembrane potential and the accumulation of reactive oxygen species (ROS) [Citation141], but this process does not lead to the release of cytochrome C, or other mitochondria-associated apoptotic molecules. Thus, granzyme A induces caspase-independent cell death pathways.
Granzyme B may be the most active member in the granzyme family. After entry into the target cell cytosol, granzyme B activates initiator caspases (such as caspases-8, -9, -10) and then promotes apoptosis through two main pathways. First, granzyme B can directly process effector caspases-3 and -7 to promote apoptosis [Citation139,Citation140,Citation142,Citation143]. Second, granzyme B-mediated proteolysis of the BH3-only protein BID exposes a myristolyation signal that induces the oligomerization of BAX and/or BAK in the mitochondrial outer membrane. That oligomerization event facilitates cytochrome C release into the cytosol, assembly of the apoptosome and effector caspases (e.g. caspases-3, -7) activation with the ensuing caspase cascade, subsequently leading to cell death.
2) Receptor–ligand mediated interaction
NK EVs-associated ligands (e.g. FasL) may interact with receptors (e.g. Fas or CD95) on the plasma membrane of target cells, and lead to trimerization of the receptors and subsequent formation of the death-inducing signalling complex (DISC) [Citation144]. DISC activates the initiator caspases (e.g. caspase-8, -10) and subsequently can induce the death signals in two directions: (a) direct activation of effector caspases-9, -3 and -7, without the involvement of mitochondria; (b) formation of apoptosomes (mitochondrial proteins, dATP and Apaf-1), leading to activation of effector caspases-3 and -7 [Citation143], consequently, dismantling the target cells.
3) Granulysin mediated action
There is no evidence to date for a specific granulysin receptor for cytotoxicity, but granulysin itself can bind to the membrane of target cell through electrostatic interaction based on the positive charge on its N-terminus [Citation142]. Granulysin is also able to go through the polyperforin pores. Granulysin appears to scissor through the cell membrane, causing ion fluxes [Citation145]. Cytosolic calcium levels increase while potassium levels decrease as a result of activation of calcium-dependent potassium pump [Citation145]. The increase in intracellular calcium, decrease in potassium and granulysin itself in the presence of ATP all contribute to mitochondrial damage [Citation146]. The damage of mitochondria results in loss of electrostatic potential, release of cytochrome C and activation of effector caspases (e.g. caspases-3, -7) [Citation130]. Granulysin can also activate a sphingomyelinase associated with the cell membrane to generate ceramide [Citation147]. Finally, granulysin can damage endoplasmic reticulum and activates caspase-7 to induce apoptosis in target cells [Citation131]. Thus, multiple mechanisms have been proposed for the granulysin-induced apoptosis. Taken together, it is conceivable that lytic molecules of NK EVs may rely on at least three mechanisms to activate the cell-death signalling network [Citation124,Citation148] (). Noticeably, it is possible that other cytotoxic factors are yet to be identified. The multiple killing mechanisms associated with NK EVs raise the possibility that NK EV-based delivery of cytotoxic molecules may be exploited as an entirely new approach to treat cancers and other diseases [Citation59].
EVs bearing NK ligands may evade immune surveillance and responses
The release of NK EVs is able to induce apoptosis of cancer cells. In opposition, cancer-derived EVs carrying NK ligands may neutralize immune surveillance and responses. NK cells attacking tumour cells are mediated by active receptors including NKG2D, NKp44, NKp46, NKp30 and DNAM-1 [Citation57]. Amongst them, NKG2D is one of the most-studied receptors [Citation125]. Ligands for human NKG2D consist of two groups of molecules, six UL16-binding proteins (ULBP1-6) and the MHC class I chain-related proteins A and B (MICA/B). While NKG2D ligands are absent from most normal cells, expression is induced upon stress and malignant transformation. NK cells may use NKG2D as a detector system to target inflamed and malignant cells and play a crucial role in anti-tumour immune surveillance [Citation149]. However, by shedding the NKG2D soluble ligands, tumour cells may evade immune responses. The shed NKG2D ligands bind to NK cell NKG2D receptors, creating competition for the receptor sites. This immune evasion through secreted EV-NKG2D ligands has been observed in prostate [Citation150], leukaemia [Citation151] and ovarian cancer [Citation152]. It has also been reported that human NKG2D ligand MICA*008 is shed by tumour cells in EVs, resulting in a marked loss of cytotoxic function of NK cells [Citation153,Citation154]. In sum, NK ligand-bearing cancer EVs selectively induce downregulation of NKG2D receptor expression and inhibit degranulation on NK cells, resulting in compromised cytotoxicity and reduced anti-tumour immune surveillance [Citation152]. Intriguingly, human placenta is also able to secrete EVs with NKG2D ligands. The released ligands downmodulate the related receptors for immunosuppressive function. The findings underline a role for NKG2D ligand-bearing placental EVs in fetal immune escape, rendering placenta as a unique immunosuppressive organ [Citation155]. Thus, EVs play versatile roles in many aspects of immune responses.
‘Theranostic’ applications of immune cell-derived EVs
The discovery of nano-sized EVs released by various cell types has profound relevance to basic and clinical research for immune modulation and autoimmune diseases [Citation156]. There are various nano-biomedical applications of EVs, ranging from nanoplatform-based diagnostics to the development of novel therapeutics. The ability of immune cell-derived EVs to either enhance or suppress immune activity makes them attractive candidates for several applications [Citation157]. They might constitute an ideal tool for ‘theranostics’ (i.e. therapy + diagnostic = theranostics) ().
Table 1. Immune cells-derived EVs-based immune-theranostic applications.
Diagnostic markers
EVs have been isolated and purified mainly from body fluids, such as blood, urine, ascites, saliva and bronchoalveolar lavage fluid. Many studies have shown significant differences in both qualitative and quantitative analyses of EVs in healthy individuals versus patients with certain diseases (e.g. cancer and renal disease) [Citation158]. These differences plus accessibility make EVs an excellent candidate biomarker. EVs are particularly suitable for this purpose. First, they travel through the bloodstream and can be easily isolated from bodily fluids such as plasma, serum and urine. Second, they receive surface markers from their cells of origin, and they may be easily identified after isolation. Third, they express unique markers, such as specific miRNA and mRNA [Citation123], or protein components from defined cell types [Citation8,Citation59].
Immune cell-derived EVs usually contain a unique biomarker for immune diagnosis. For example, in transplantation, Tregs cells identify the allogeneic antigen of donor and the receptor on the surface of DCs, and only after activation do Tregs cells secrete EVs. Therefore, it is feasible to evaluate the viability and function of Tregs cells by detecting the level of EVs secreted from Tregs cells in vivo for transplantation evaluation [Citation159]. Similarly, circulating immune cell-derived EVs can be disease-specific biomarkers of severity of inflammation. For example, the levels of circulating EVs from CD4+ and CD8+ T cells have been elevated in patients with chronic hepatitis C, and the levels of circulating EVs from iNKT cells and CD14+ macrophages/monocytes are elevated in patients with nonalcoholic fatty liver or nonalcoholic steatohepatitis. The level of these EVs in the sera is in correlation to the severity of the disease and is thus a viable diagnostic tool for the degree of these liver inflammatory conditions [Citation160,Citation161]. It is expected that the identification of novel biomarkers from immune cell-derived EVs will grow rapidly.
Therapeutic interventions
The ability of immune cell-derived EVs to modulate immune responses suggests they could be used as a therapeutic approach for a broad spectrum of diseases. In that regard, to date, pre-clinical studies of DC-derived EVs have demonstrated potent activity [Citation162].
DC-derived EVs potentially may be utilized as a therapy for immune regulation against HIV-1 infection. A low dosage release of DCs-derived EVs appears to be more beneficial against HIV-1 infection in comparison with a high dose in terms of immune response. Additionally, the release of the EVs with the capacity to modulate the immune system seemingly is not related to the DC maturation stage [Citation162]. DC-derived EVs have also been applied to brain diseases. Using electroporation, the siRNA against glyceraldehyde-3-phosphate dehydrogenase is fused with DC-derived EVs, which can effectively deliver in vivo and lead to deleted expression of the target gene in neurons, microglia and oligodendrocytes, resulting in a specific gene knockdown in the brain in a mouse model of Alzheimer’s disease [Citation163]. EVs can promote CNS myelination and may be used to regulate inflammation in brain trauma and neurodegenerative disorders [Citation164].
Different strategies for delivering therapeutic molecules using DC-derived EVs have been proposed for the treatment of autoimmune diseases [Citation165]. For example, IL-4, FasL or indoleamine-pyrrole 2,3-dioxygenase (IDO) can be gene transferred into DCs, and such DC-derived EVs have the capacity to ameliorate early-stage disease in a murine model of collagen induced arthritis [Citation166–Citation168]. The use of DC-derived EVs may be a better therapeutic compared with DCs for the treatment of autoimmune diseases such as rheumatoid arthritis [Citation169]. Immature bone marrow DCs are able to secrete tolerogenic exosomes, which are involved in the suppression of immune responses in a rat model of experimental autoimmune myasthenia gravis. These EVs upregulate levels of IDO/Treg and decrease synthesis of anti-R97-116 IgG, IgG2a and IgG2b antibodies [Citation170]. Moreover, DC-derived EVs can mediate the activation of CD4+ T cells through an endocrine mechanism, leading to improved cardiac function and wound healing after myocardial infarction [Citation171]. DC-derived EVs are also applicable to anti-cancer treatments. For example, DC-derived EVs-based phase I and II clinical trials have been conducted in advanced malignancies, and these agents have proven to be feasible and safe [Citation172]. A phase II trial confirmed the capacity of DC-derived EVs to boost the NK cell arm of anti-tumour immunity in patients with advanced non-small cell lung cancer (NSCLC) [Citation173].
Macrophages-derived EVs are able to modulate immune responses and are used in some disease treatments. For example, the plasmid encoding therapeutic protein (e.g. catalase or glial cell line-derived neurotrophic factor) has been applied to transfect macrophages [Citation174,Citation175]. After injection of transfected macrophages into mice with Parkinson disease, the EVs that contain modified genetic material are released, resulting in significant improvement in motor function [Citation174,Citation175]. After transplantation, transfer of EVs of autologous Treg cells prolongs survival and renal allograft function [Citation159]. Therefore, Treg-derived EVs might be a new approach to induce transplantation tolerance [Citation123].
Human NK cells secrete EVs that contain typical NK markers (e.g. CD56) and lytic proteins (e.g. granulysin, granzyme A, granzyme B, perforin, FasL) [Citation58,Citation59]. Thus, it is possible that NK EVs may be utilized as a novel approach to treat cancer [Citation157]. A study suggested that NK EVs increased the proliferation rate of NK cells and enhanced NK-mediated cytotoxicity to human melanoma cancer cells (B16F10/F cells) [Citation129]. In addition, a large-scale isolation of activated NK EVs from ex vivo expansion cultures has been developed and has been shown to be cytotoxic to several tested cancer cell lines including ALL (SupB15, NALM-6), neuroblastoma (CHLA-136, CHLA-255) and breast carcinoma (MCF7) [Citation59].
Vaccination
Immune-cell-derived EVs have immunomodulatory properties. Thus, engineering immune-cell-derived EVs containing specific biomolecules and small-molecule drugs that are able to be delivered to the target cells may stimulate the patient’s immune system to recognize and destroy tumour cells or pathogens. Studies have shown that DC-derived EVs in vivo and in vitro can induce antigen-specific CD4+ and CD8+ T-cell reactions and strengthen in vivo anti-tumour immunity [Citation81,Citation165]. Furthermore, EVs from DCs loaded with specific antigens and matured with the Toll-like receptor (TLR)-3 ligand poly(I:C) as inhibitory signal resulted in reduced tumour growth, limited metastasis burden and, most importantly, prolonged survival of a mouse model of human melanoma. These studies indicate that poly(I:C) is a promising candidate for the production of DC-derived EVs vaccines with immune stimulatory properties suitable for the immunotherapy of cancer [Citation176]. Overall, DC-derived EVs are promising vaccine candidates for the treatment of cancer.
In recent years, the immune cell-derived EVs as a vaccine against infectious diseases have gained widespread attention. A novel ovalbumin (OVA)-specific T cells-derived EVs has been generated in a chronic infection mouse model. The exosome-targeted T-cell-based vaccine counteracts T-cell anergy and converts CTL exhaustion via CD40L signalling through the mTORC1 pathway after i.v. infecting C57BL/6 mice with the OVA-expressing adenovirus AdVova [Citation177]. In addition, T-cell-based vaccines that comprise polyclonal CD4+ T cells armed with antigen-specific DC released exosomes may have applications in the treatment of viral infection, including HIV-1 [Citation178,Citation179].
Prospect
Immune-cell-derived EVs are a cellular product with multiple features that make them suitable for clinical applications. They can be prepared from individual patients for autologous use. Moreover, immune-cell-derived EVs have advantages over whole cell-based therapies in regard to manufacture, storage, transport, and transplantation. For example, NK EV preparations are stable in −80°C for at least one year [Citation59], making them readily available ‘off-the-shelf’. The administration of EVs instead of cells might also reduce some of the risks associated with cellular therapy (e.g. cytokine release syndrome, graft-versus-host disease) [Citation180]. Notably, NK EVs may be altered to improve targeting and cytotoxicity via engineering of NK cells [Citation181]. Finally, it is known that EVs can cross the blood–brain barrier [Citation182], and thus, they may be able to enter cancer sanctuary sites such as the central nervous system. Thus, the potential advantages of immune cell-derived EV-based applications versus whole cell-based applications provide a rationale to develop an EV-based immunotherapy.
Several key issues pertaining to the use of EVs in clinical require further exploration, such as standardization, optimization, quality control, development of release criteria standards, and further study of the biology and mechanism of EV communication with target cells [Citation3]. Overall, the potential of immune cell-derived EVs in immuno-theranostic applications is highly promising.
Acknowledgements
The authors thank Jennifer Liu for reviewing and editing. This study was supported in part by awards from NCI P30CA014089 (A.S.W.), the Alex’s Lemonade Stand Foundation, the Team Connor Foundation, the William Lawrence and Blanche Hughes Foundation, and the Whittier Foundation (A.J., R.C.S. and A.S.W.).
Disclosure statement
No potential conflict of interest was reported by the authors.
Additional information
Funding
References
- Colombo M, Raposo G, Théry C Biogenesis, secretion, and intercellular interactions of exosomes and other extracellular vesicles. Annu Rev Cell Dev Biol. 2014;30:1–19. PubMed PMID: 25288114. DOI:10.1146/annurev-cellbio-101512-122326
- Bobrie A, Colombo M, Raposo G, et al. Exosome secretion: molecular mechanisms and roles in immune responses. Traffic. 2011;12(12):1659–1668. Epub 2011/06/08. PubMed PMID: 21645191. DOI:10.1111/j.1600-0854.2011.01225.x
- Kalra H, Drummen GP, Mathivanan S Focus on extracellular vesicles: introducing the next small big thing. Int J Mol Sci. 2016;17(2):170. Epub 2016/02/06. PubMed PMID: 26861301; PMCID: PMC4783904. DOI:10.3390/ijms17020170
- Mathivanan S, Ji H, Simpson RJ Exosomes: extracellular organelles important in intercellular communication. J Proteomics. 2010;73(10):1907–1920. Epub 2010/07/01. PubMed PMID: 20601276. DOI:10.1016/j.jprot.2010.06.006
- Lötvall J, Hill AF, Hochberg F, et al., Minimal experimental requirements for definition of extracellular vesicles and their functions: a position statement from the International Society for Extracellular Vesicles. J Extracell Vesicles. 2014;3:26913. PubMed PMID: 25536934; PMCID: PMC4275645.
- Minciacchi VR, You S, Spinelli C, et al., Large oncosomes contain distinct protein cargo and represent a separate functional class of tumor-derived extracellular vesicles. Oncotarget. 2015;6(13):11327–11341. PubMed PMID: 25857301; PMCID: PMC4484459. DOI:10.18632/oncotarget.3598
- Keerthikumar S, Gangoda L, Liem M, et al., Proteogenomic analysis reveals exosomes are more oncogenic than ectosomes. Oncotarget. 2015;6(17):15375–15396. PubMed PMID: 25944692; PMCID: PMC4558158. DOI:10.18632/oncotarget.3801
- Kowal J, Arrasb G, Colomboa M, et al., Proteomic comparison defines novel markers to characterize heterogeneous populations of extracellular vesicle subtypes. Proc Natl Acad Sci U S A. 2016;113(8):E968–77. PMID: 26858453. PMCID: PMC4776515. DOI:10.1073/pnas.1521230113
- Martínez de Lizarrondo S, Roncal C, Calvayrac O, et al., Synergistic effect of thrombin and CD40 ligand on endothelial matrix metalloproteinase-10 expression and microparticle generation in vitro and in vivo. Arterioscler Thromb Vasc Biol. 2012;32(6):1477–1487. Epub 2012/04/05. PubMed PMID: 22492089. DOI:10.1161/ATVBAHA.112.248773
- Falati S, Liu Q, Gross P, et al., Accumulation of tissue factor into developing thrombi in vivo is dependent upon microparticle P-selectin glycoprotein ligand 1 and platelet P-selectin. J Exp Med. 2003;197(11):1585–1598. PubMed PMID: 12782720; PMCID: PMC2193915. DOI:10.1084/jem.20021868
- Mezouar S, Darbousset R, Dignat-George F, et al. Inhibition of platelet activation prevents the P-selectin and integrin-dependent accumulation of cancer cell microparticles and reduces tumor growth and metastasis in vivo. Int J Cancer. 2015;136(2):462–475. Epub 2014/06/09. PubMed PMID: 24889539. DOI:10.1002/ijc.28997
- Pluskota E, Woody NM, Szpak D, et al. Expression, activation, and function of integrin alphaMbeta2 (Mac-1) on neutrophil-derived microparticles. Blood. 2008;112(6):2327–2335. Epub 2008/05/28. PubMed PMID: 18509085; PMCID: PMC2532806. DOI:10.1182/blood-2007-12-127183
- Muralidharan-Chari V, Clancy JW, Sedgwick A, et al. Microvesicles: mediators of extracellular communication during cancer progression. J Cell Sci. 2010;123(Pt 10):1603–1611. PubMed PMID: 20445011; PMCID: PMC2864708. DOI:10.1242/jcs.064386
- Laulagnier K, Vincent-Schneider H, Hamdi S, et al., Characterization of exosome subpopulations from RBL-2H3 cells using fluorescent lipids. Blood Cells Mol Dis. 2005;35(2):116–121. PubMed PMID: 16023874. DOI:10.1016/j.bcmd.2005.05.010
- Trajkovic K, Hsu C, Chiantia S, et al., Ceramide triggers budding of exosome vesicles into multivesicular endosomes. Science. 2008;319(5867):1244–1247. PubMed PMID: 18309083. DOI:10.1126/science.1153124
- Llorente A, Skotland T, Sylvänne T, et al., Molecular lipidomics of exosomes released by PC-3 prostate cancer cells. Biochim Biophys Acta. 2013;1831(7):1302–1309. PubMed PMID: 24046871.
- Valadi H, Ekström K, Bossios A, et al. Exosome-mediated transfer of mRNAs and microRNAs is a novel mechanism of genetic exchange between cells. Nat Cell Biol. 2007;9(6):654–659. PubMed PMID: 17486113. DOI:10.1038/ncb1596
- Mittelbrunn M, Gutierrez-Vazquez C, Villarroya-Beltri C, et al., Unidirectional transfer of microRNA-loaded exosomes from T cells to antigen-presenting cells. Nat Commun. 2011;2:282. Epub 2011/04/21. PubMed PMID: 21505438; PMCID: 3104548. DOI:10.1038/ncomms1285
- Nolte-’T Hoen EN, Buermans HP, Waasdorp M, et al., Deep sequencing of RNA from immune cell-derived vesicles uncovers the selective incorporation of small non-coding RNA biotypes with potential regulatory functions. Nucleic Acids Res. 2012;40(18):9272–9285. Epub 2012/07/19. PubMed PMID: 22821563; PMCID: PMC3467056. DOI:10.1093/nar/gks658
- Montecalvo A, Larregina AT, Shufesky WJ, et al., Mechanism of transfer of functional microRNAs between mouse dendritic cells via exosomes. Blood. 2012;119(3):756–766. Epub 2011/10/28. PubMed PMID: 22031862; PMCID: 3265200. DOI:10.1182/blood-2011-02-338004
- van den Boorn JG, Dassler J, Coch C, et al., Exosomes as nucleic acid nanocarriers. Adv Drug Deliv Rev. 2013;65(3):331–335. Epub 2012/07/04. PubMed PMID: 22750807. DOI:10.1016/j.addr.2012.06.011
- Raimondo F, Morosi L, Chinello C, et al. Advances in membranous vesicle and exosome proteomics improving biological understanding and biomarker discovery. Proteomics. 2011;11(4):709–720. PubMed PMID: 21241021. DOI:10.1002/pmic.201000422
- Schorey JS, Cheng Y, Singh PP, et al. Exosomes and other extracellular vesicles in host-pathogen interactions. EMBO Rep. 2015;16(1):24–43. Epub 2014/12/08. PubMed PMID: 25488940; PMCID: PMC4304727. DOI:10.15252/embr.201439363
- Carrière J, Barnich N, Nguyen HT Exosomes: from functions in host-pathogen interactions and immunity to diagnostic and therapeutic opportunities. Rev Physiol Biochem Pharmacol. 2016;172:39–75. PubMed PMID: 27600934. DOI:10.1007/112_2016_7
- Choi DS, Kim DK, Kim YK, et al. Proteomics, transcriptomics and lipidomics of exosomes and ectosomes. Proteomics. 2013; 13 (10–11): 1554–1571. PubMed PMID: 23401200. DOI:10.1002/pmic.201200329
- Challagundla KB, Wise PM, Neviani P, et al., Exosome-mediated transfer of microRNAs within the tumor microenvironment and neuroblastoma resistance to chemotherapy. J Natl Cancer Inst. 2015;107(7). PubMed PMID: 25972604; PMCID: PMC4651042. DOI:10.1093/jnci/djv135
- Ratajczak J, Miekus K, Kucia M, et al., Embryonic stem cell-derived microvesicles reprogram hematopoietic progenitors: evidence for horizontal transfer of mRNA and protein delivery. Leukemia. 2006;20(5):847–856. PMID: 16453000.
- Fabbri M, Paone A, Calore F, et al. A new role for microRNAs, as ligands of Toll-like receptors. RNA Biol. 2013;10(2):169–174. Epub 2013/01/09. PubMed PMID: 23296026; PMCID: 3594274. DOI:10.4161/rna.23144
- Anastasiadou E, Slack FJ Cancer. Malicious exosomes. Science. 2014;346(6216):1459–1460. PubMed PMID: 25525233. DOI:10.1126/science.aaa4024
- Ahadi A, Brennan S, Kennedy PJ, et al. Long non-coding RNAs harboring miRNA seed regions are enriched in prostate cancer exosomes. Sci Rep. 2016;6:24922. Epub 2016/04/22. PubMed PMID: 27102850; PMCID: PMC4840345. DOI:10.1038/srep24922
- Tosar JP, Cayota A, Eitan E, et al., Ribonucleic artefacts: are some extracellular RNA discoveries driven by cell culture medium components? J Extracell Vesicles. 2017;6(1):1272832. Epub 2017/01/12. PubMed PMID: 28326168; PMCID: PMC5328325. DOI:10.1080/20013078.2016.1272832
- Thakur BK, Zhang H, Becker A, et al., Double-stranded DNA in exosomes: a novel biomarker in cancer detection. Cell Res. 2014;24(6):766–769. PMID: 24710597. DOI:10.1038/cr.2014.44
- Cai J, Han Y, Ren H, et al., Extracellular vesicle-mediated transfer of donor genomic DNA to recipient cells is a novel mechanism for genetic influence between cells. J Mol Cell Biol. 2013;5(4):227–238. PMID: 23580760. DOI:10.1093/jmcb/mjt011
- Cai J, Wu G, Tan X, et al., Transferred BCR/ABL DNA from K562 extracellular vesicles causes chronic myeloid leukemia in immunodeficient mice. PLoS One. 2014;9(8):e105200. PMID: 25133686. DOI:10.1371/journal.pone.0105200
- Balaj L, Lessard R, Dai L, et al., Tumour microvesicles contain retrotransposon elements and amplified oncogene sequences. Nat Commun. 2011;2:180. PMID: 21285958. DOI:10.1038/ncomms1180
- Németh A, Orgovan N, Sódar BW, et al., Antibiotic-induced release of small extracellular vesicles (exosomes) with surface-associated DNA. Sci Rep. 2017;7(1):8202. PMID: 28811610. DOI:10.1038/s41598-017-08392-1
- Lian Q, Xu J, Yan S, et al., Chemotherapy-induced intestinal inflammatory responses are mediated by exosome secretion of double-strand DNA via AIM2 inflammasome activation. Cell Res. 2017;27(6):784–800. PMID: 28409562. DOI:10.1038/cr.2017.54
- Coumans FAW, Brisson AR, Buzas EI, et al., Methodological guidelines to study extracellular vesicles. Circ Res. 2017;120(10):1632–1648. PMID: 28495994. DOI:10.1161/CIRCRESAHA.117.309417
- Sobo-Vujanovic A, Munich S, Vujanovic NL Dendritic-cell exosomes cross-present Toll-like receptor-ligands and activate bystander dendritic cells. Cell Immunol. 2014; 289 (1–2): 119–127. Epub 2014/04/08. PubMed PMID: 24759079; PMCID: PMC4045011. DOI:10.1016/j.cellimm.2014.03.016
- Vukman KV, Försönits A, Oszvald Á, et al. Mast cell secretome: soluble and vesicular components. Semin Cell Dev Biol. 2017;67:65–73. PMID: 28189858. DOI:10.1016/j.semcdb.2017.02.002
- McDonald MK, Tian Y, Qureshi RA, et al. Functional significance of macrophage-derived exosomes in inflammation and pain Pain. 2014;155(8):1527–1539. Epub 2014/04/30. PubMed PMID: 24792623; PMCID: PMC4106699. DOI:10.1016/j.pain.2014.04.029
- Lee HD, Kim YH, Kim DS Exosomes derived from human macrophages suppress endothelial cell migration by controlling integrin trafficking. Eur J Immunol. 2014;44(4):1156–1169. Epub 2013/12/18. PubMed PMID: 24338844. DOI:10.1002/eji.201343660
- Buschow SI, van Balkom BW, Aalberts M, et al. MHC class II-associated proteins in B-cell exosomes and potential functional implications for exosome biogenesis. Immunol Cell Biol. 2010;88(8):851–856. Epub 2010/05/11. PubMed PMID: 20458337. DOI:10.1038/icb.2010.64
- Zhang H, Xie Y, Li W, et al. CD4(+) T cell-released exosomes inhibit CD8(+) cytotoxic T-lymphocyte responses and antitumor immunity. Cell Mol Immunol. 2011;8(1):23–30. Epub 2010/12/13. PubMed PMID: 21200381; PMCID: PMC4002994. DOI:10.1038/cmi.2010.59
- Yu X, Huang C, Song B, et al., CD4+CD25+ regulatory T cells-derived exosomes prolonged kidney allograft survival in a rat model. Cell Immunol. 2013; 285 (1–2): 62–68. Epub 2013/06/28. PubMed PMID: 24095986. DOI:10.1016/j.cellimm.2013.06.010
- Zhang B, Yin Y, Lai RC, et al. Immunotherapeutic potential of extracellular vesicles. Front Immunol. 2014;5:518. Epub 2014/10/22. PubMed PMID: 25374570; PMCID: PMC4205852. DOI:10.3389/fimmu.2014.00518
- Srinivasan S, Vannberg FO, Dixon JB Lymphatic transport of exosomes as a rapid route of information dissemination to the lymph node. Sci Rep. 2016;6:24436. PMID: 27087234. DOI:10.1038/srep24436
- Milasan A, Tessandier N, Tan S, et al. Extracellular vesicles are present in mouse lymph and their level differs in atherosclerosis. J Extracell Vesicles. 2016;5:31427. PMID: 27664155. DOI:10.3402/jev.v5.31427
- Sirisinha S Evolutionary insights into the origin of innate and adaptive immune systems: different shades of grey. Asian Pac J Allergy Immunol. 2014;32(1):3–15. PubMed PMID: 24641285.
- Théry C, Amigorena S The cell biology of antigen presentation in dendritic cells. Curr Opin Immunol. 2001;13(1):45–51. PubMed PMID: 11154916.
- Ferrari D, McNamee EN, Idzko M, et al. Purinergic Signaling During Immune Cell Trafficking. Trends Immunol. 2016;37(6):399–411. Epub 2016/04/30. PubMed PMID: 27142306. DOI:10.1016/j.it.2016.04.004
- Akdis M, Aab A, Altunbulakli C, et al., Interleukins (from IL-1 to IL-38), interferons, transforming growth factor β, and TNF-α: receptors, functions, and roles in diseases. J Allergy Clin Immunol. 2016;138(4):984–1010. Epub 2016/08/28. PubMed PMID: 27577879. DOI:10.1016/j.jaci.2016.06.033
- Yanez-Mo M, Siljander PR, Andreu Z, et al., Biological properties of extracellular vesicles and their physiological functions. J Extracell Vesicles. 2015;4:27066. PubMed PMID: 25979354; PMCID: PMC4433489. DOI:10.3402/jev.v4.27066
- Simhadri VR, Reiners KS, Hansen HP, et al., Dendritic cells release HLA-B-associated transcript-3 positive exosomes to regulate natural killer function. PLoS One. 2008;3(10):e3377. Epub 2008/10/13. PubMed PMID: 18852879; PMCID: PMC2566590. DOI:10.1371/journal.pone.0003377
- Viaud S, Terme M, Flament C, et al., Dendritic cell-derived exosomes promote natural killer cell activation and proliferation: a role for NKG2D ligands and IL-15Ralpha. PLoS One. 2009;4(3):e4942. Epub 2009/03/26. PubMed PMID: 19319200; PMCID: 2657211. DOI:10.1371/journal.pone.0004942
- Munich S, Sobo-Vujanovic A, Buchser WJ, et al. Dendritic cell exosomes directly kill tumor cells and activate natural killer cells via TNF superfamily ligands. Oncoimmunology. 2012;1(7):1074–1083. Epub 2012/11/22. PubMed PMID: 23170255; PMCID: 3494621. DOI:10.4161/onci.20897
- Zhang J, Basher F, Wu JD NKG2D Ligands in Tumor Immunity: two Sides of a Coin. Front Immunol. 2015;6:97. PubMed PMID: 25788898; PMCID: 4349182. DOI:10.3389/fimmu.2015.00097
- Lugini L, Cecchetti S, Huber V, et al., Immune surveillance properties of human NK cell-derived exosomes. J Immunol. 2012;189(6):2833–2842. Epub 2012/08/21. PubMed PMID: 22904309. DOI:10.4049/jimmunol.1101988
- Jong AY, Wu CH, Li J, et al. Large-scale isolation and cytotoxicity of extracellular vesicles derived from activated human natural killer cells. J Extracell Vesicles. 2017;6(1):1294368. PubMed PMID: 28326171; PMCID: PMC5345580. DOI:10.1080/20013078.2017.1294368
- Ismail N, Wang Y, Dakhlallah D, et al., Macrophage microvesicles induce macrophage differentiation and miR-223 transfer. Blood. 2013;121(6):984–995. Epub 2012/11/09. PubMed PMID: 23144169; PMCID: PMC3567345. DOI:10.1182/blood-2011-08-374793
- Bhatnagar S, Shinagawa K, Castellino FJ, et al. Exosomes released from macrophages infected with intracellular pathogens stimulate a proinflammatory response in vitro and in vivo. Blood. 2007;110(9):3234–3244. Epub 2007/07/31. PubMed PMID: 17666571; PMCID: PMC2200902. DOI:10.1182/blood-2007-03-079152.
- Anand PK, Anand E, Bleck CK, et al. Exosomal Hsp70 induces a pro-inflammatory response to foreign particles including mycobacteria. PLoS One. 2010;5(4):e10136. Epub 2010/04/12. PubMed PMID: 20405033; PMCID: PMC2853569. DOI:10.1371/journal.pone.0010136
- Bhatnagar S, Schorey JS Exosomes released from infected macrophages contain Mycobacterium avium glycopeptidolipids and are proinflammatory. J Biol Chem. 2007;282(35):25779–25789. Epub 2007/06/25. PubMed PMID: 17591775; PMCID: PMC3636815. DOI:10.1074/jbc.M702277200
- Truman JP, Al Gadban MM, Smith KJ, et al., Differential regulation of acid sphingomyelinase in macrophages stimulated with oxidized low-density lipoprotein (LDL) and oxidized LDL immune complexes: role in phagocytosis and cytokine release. Immunology. 2012;136(1):30–45. PubMed PMID: 22236141; PMCID: PMC3372755. DOI:10.1111/j.1365-2567.2012.03552.x
- Sarkar A, Mitra S, Mehta S, et al. Monocyte derived microvesicles deliver a cell death message via encapsulated caspase-1. PLoS One. 2009:4(9):e7140. PMID:19779610. PMCID: PMC2744928. DOI:10.1371/journal.pone.0007140
- de Rivero Vaccari JP, Dietrich WD, Keane RW Activation and regulation of cellular inflammasomes: gaps in our knowledge for central nervous system injury. J Cereb Blood Flow Metab. 2014;34(3):369–375. PMID: 24398940. DOI:10.1038/jcbfm.2013.227
- Zhang Y, Liu F, Yuan Y, et al., Inflammasome-derived exosomes activate NF-κB signaling in macrophages. J Proteome Res. 2017;16(1):170–178. PMID: 27684284. DOI:10.1021/acs.jproteome.6b00599
- Gasser O, Schifferli JA Activated polymorphonuclear neutrophils disseminate anti-inflammatory microparticles by ectocytosis. Blood. 2004;104(8):2543–2548. Epub 2004/06/22. PubMed PMID: 15213101. DOI:10.1182/blood-2004-01-0361
- Giri PK, Kruh NA, Dobos KM, et al. Proteomic analysis identifies highly antigenic proteins in exosomes from M. tuberculosis-infected and culture filtrate protein-treated macrophages. Proteomics. 2010;10(17):3190–3202. PubMed PMID: 20662102; PMCID: PMC3664454. DOI:10.1002/pmic.200900840
- Giri PK, Schorey JS Exosomes derived from M. Bovis BCG infected macrophages activate antigen-specific CD4+ and CD8+ T cells in vitro and in vivo. PLoS One. 2008;3(6):e2461. Epub 2008/06/18. PubMed PMID: 18560543; PMCID: PMC2413420. DOI:10.1371/journal.pone.0002461
- Izquierdo-Useros N, Lorizate M, Puertas MC, et al., Siglec-1 is a novel dendritic cell receptor that mediates HIV-1 trans-infection through recognition of viral membrane gangliosides. PLoS Biol. 2012;10(12):e1001448. Epub 2012/12/18. PubMed PMID: 23271952; PMCID: PMC3525531. DOI:10.1371/journal.pbio.1001448
- Utsugi-Kobukai S, Fujimaki H, Hotta C, et al., Minami M. MHC class I-mediated exogenous antigen presentation by exosomes secreted from immature and mature bone marrow derived dendritic cells. Immunol Lett. 2003; 89 (2–3): 125–131. PubMed PMID: 14556969.
- Segura E, Amigorena S, Théry C Mature dendritic cells secrete exosomes with strong ability to induce antigen-specific effector immune responses. Blood Cells Mol Dis. 2005;35(2):89–93. PubMed PMID: 15990342. DOI:10.1016/j.bcmd.2005.05.003
- Luketic L, Delanghe J, Sobol PT, et al., Antigen presentation by exosomes released from peptide-pulsed dendritic cells is not suppressed by the presence of active CTL. J Immunol. 2007;179(8):5024–5032. PubMed PMID: 17911587.
- Admyre C, Johansson SM, Paulie S, et al. Direct exosome stimulation of peripheral human T cells detected by ELISPOT. Eur J Immunol. 2006;36(7):1772–1781. PubMed PMID: 16761310. DOI:10.1002/eji.200535615
- Hwang I, Shen X, Sprent J Direct stimulation of naive T cells by membrane vesicles from antigen-presenting cells: distinct roles for CD54 and B7 molecules. Proc Natl Acad Sci U S A. 2003;100(11):6670–6675. Epub 2003/05/12. PubMed PMID: 12743365; PMCID: PMC164505. DOI:10.1073/pnas.1131852100
- Segura E, Nicco C, Lombard B, et al. ICAM-1 on exosomes from mature dendritic cells is critical for efficient naive T-cell priming. Blood. 2005;106(1):216–223. Epub 2005/03/24. PubMed PMID: 15790784. DOI:10.1182/blood-2005-01-0220
- Raposo G, Nijman HW, Stoorvogel W, et al. B lymphocytes secrete antigen-presenting vesicles. J Exp Med. 1996;183(3):1161–1172. PubMed PMID: 8642258; PMCID: PMC2192324.
- Vincent-Schneider H, Stumptner-Cuvelette P, Lankar D, et al. Exosomes bearing HLA-DR1 molecules need dendritic cells to efficiently stimulate specific T cells. Int Immunol. 2002;14(7):713–722. PubMed PMID: 12096030.
- Montecalvo A, Shufesky WJ, Stolz DB, et al., Exosomes as a short-range mechanism to spread alloantigen between dendritic cells during T cell allorecognition. J Immunol. 2008;180(5):3081–3090. PubMed PMID: 18292531.
- Théry C, Duban L, Segura E, et al., Indirect activation of naïve CD4+ T cells by dendritic cell-derived exosomes. Nat Immunol. 2002;3(12):1156–1162. Epub 2002/11/11. PubMed PMID: 12426563. DOI:10.1038/ni854
- Chaput N, Schartz NE, André F, et al. Exosomes as potent cell-free peptide-based vaccine. II. Exosomes in CpG adjuvants efficiently prime naive Tc1 lymphocytes leading to tumor rejection. J Immunol. 2004;172(4):2137–2146. PubMed PMID: 14764679.
- Yewdell JW, Dolan BP Immunology: cross-dressers turn on T cells. Nature. 2011;471(7340):581–582. PubMed PMID: 21455165; PMCID: PMC3400133. DOI:10.1038/471581a
- Coppieters K, Barral AM, Juedes A, et al., No significant CTL cross-priming by dendritic cell-derived exosomes during murine lymphocytic choriomeningitis virus infection. J Immunol. 2009;182(4):2213–2220. PubMed PMID: 19201875. DOI:10.4049/jimmunol.0802578
- Wakim LM, Bevan MJ Cross-dressed dendritic cells drive memory CD8+ T-cell activation after viral infection. Nature. 2011;471(7340):629–632. PubMed PMID: 21455179; PMCID: PMC3423191. DOI:10.1038/nature09863
- Wolfers J, Lozier A, Raposo G, et al., Tumor-derived exosomes are a source of shared tumor rejection antigens for CTL cross-priming. Nat Med. 2001;7(3):297–303. PubMed PMID: 11231627. DOI:10.1038/85438
- Andre F, Schartz NE, Movassagh M, et al., Malignant effusions and immunogenic tumour-derived exosomes. Lancet. 2002;360(9329):295–305. PubMed PMID: 12147373. DOI:10.1016/S0140-6736(02)09552-1
- Napoletano C, Rughetti A, Landi R, et al., Immunogenicity of allo-vesicle carrying ERBB2 tumor antigen for dendritic cell-based anti-tumor immunotherapy. Int J Immunopathol Pharmacol. 2009;22(3):647–658. PubMed PMID: 19822081.
- Qazi KR, Gehrmann U, Domange Jordö E, et al., Antigen-loaded exosomes alone induce Th1-type memory through a B-cell-dependent mechanism. Blood. 2009;113(12):2673–2683. Epub 2009/01/27. PubMed PMID: 19176319. DOI:10.1182/blood-2008-04-153536
- Skokos D, Le Panse S, Villa I, et al., Mast cell-dependent B and T lymphocyte activation is mediated by the secretion of immunologically active exosomes. J Immunol. 2001;166(2):868–876. PubMed PMID: 11145662.
- Skokos D, Botros HG, Demeure C, et al. Mast cell-derived exosomes induce phenotypic and functional maturation of dendritic cells and elicit specific immune responses in vivo. J Immunol. 2003;170(6):3037–3045. PubMed PMID: 12626558.
- Véron P, Segura E, Sugano G, et al. Accumulation of MFG-E8/lactadherin on exosomes from immature dendritic cells. Blood Cells Mol Dis. 2005;35(2):81–88. PubMed PMID: 15982908. DOI:10.1016/j.bcmd.2005.05.001
- Théry C, Regnault A, Garin J, et al., Molecular characterization of dendritic cell-derived exosomes. Selective accumulation of the heat shock protein hsc73. J Cell Biol. 1999;147(3):599–610. PubMed PMID: 10545503; PMCID: PMC2151184.
- Benito-Martin A, Di Giannatale A, Ceder S, et al. The new deal: a potential role for secreted vesicles in innate immunity and tumor progression. Front Immunol. 2015; 6:66. PMID: 25759690.
- Skokos D, Goubran-Botros H, Roa M, et al. Immunoregulatory properties of mast cell-derived exosomes. Mol Immunol. 2002; 38(16–18):1359–1362. PMID: 12217408. DOI:10.1016/j.semcdb.2017.02.002
- Li F, Wang Y, Lin L, et al. Mast cell-derived exosomes promote Th2 cell differentiation via OX40L-OX40 ligation. J Immunol Res. 2016;2016:3623898. PMID: 27066504
- Al-Nedawi K, Szemraj J, Cierniewski CS Mast cell-derived exosomes activate endothelial cells to secrete plasminogen activator inhibitor type 1. Arterioscler Thromb Vasc Biol. 2005; 25(8):1744–1749. PMID: 15920032.
- Xiao H, Lässer C, Shelke GV, et al., Mast cell exosomes promote lung adenocarcinoma cell proliferation - role of KIT-stem cell factor signaling. Cell Commun Signal. 2014; 12:64. PMID: 25311367.
- Nolte-’T Hoen EN, Wauben MH Immune cell-derived vesicles: modulators and mediators of inflammation. Curr Pharm Des. 2012;18(16):2357–2368. PubMed PMID: 22390699.
- Wahlgren J, Karlson Tde L, Glader P, et al. Activated human T cells secrete exosomes that participate in IL-2 mediated immune response signaling. PLoS One. 2012;7(11):e49723. PubMed PMID: 23166755; PMCID: 3500321. DOI:10.1371/journal.pone.0049723
- Shefler I, Salamon P, Reshef T, et al. T cell-induced mast cell activation: a role for microparticles released from activated T cells. J Immunol. 2010;185(7):4206–4212. Epub 2010/09/01. PubMed PMID: 20810987. DOI:10.4049/jimmunol.1000409
- Shefler I, Pasmanik-Chor M, Kidron D, et al. T cell-derived microvesicles induce mast cell production of IL-24: relevance to inflammatory skin diseases. J Allergy Clin Immunol. 2014;133(1):217-24.e1-3. Epub 2013/06/12. PubMed PMID: 23768573. DOI:10.1016/j.jaci.2013.04.035
- Cai Z, Yang F, Yu L, et al., Activated T cell exosomes promote tumor invasion via Fas signaling pathway. J Immunol. 2012;188(12):5954–5961. Epub 2012/05/09. PubMed PMID: 22573809. DOI:10.4049/jimmunol.1103466
- Robbins PD, Morelli AE Regulation of immune responses by extracellular vesicles. Nat Rev Immunol. 2014;14(3):195–208. PubMed PMID: 24566916; PMCID: PMC4350779. DOI:10.1038/nri3622
- Skogberg G, Gudmundsdottir J, van der Post S, et al., Characterization of human thymic exosomes. PLoS One. 2013;8(7):e67554. PMID: 23844026. DOI:10.1371/journal.pone.0067554
- Skogberg G, Lundberg V, Berglund M, et al., Human thymic epithelial primary cells produce exosomes carrying tissue-restricted antigens. Immunol Cell Biol. 2015;93(8):727–734. PMID: 25776846. DOI:10.1038/icb.2015.33
- Wang GJ, Liu Y, Qin A, et al. Thymus exosomeslike particles induce regulatory T cells. J Immunol. 2008; 181:5242–5248. PMID: 18832678.
- Tang XJ, Sun XY, Huang KM, et al., Therapeutic potential of CAR-T cell-derived exosomes: a cell-free modality for targeted cancer therapy. Oncotarget. 2015;6(42):44179–44190. PMID: 26496034.
- Yang X, Meng S, Jiang H, et al. Exosomes derived from immature bone marrow dendritic cells induce tolerogenicity of intestinal transplantation in rats. J Surg Res. 2011;171(2):826–832. Epub 2010/06/08. PubMed PMID: 20828738. DOI:10.1016/j.jss.2010.05.021
- Li X, Li JJ, Yang JY, et al., Tolerance induction by exosomes from immature dendritic cells and rapamycin in a mouse cardiac allograft model. PLoS One. 2012;7(8):e44045. Epub 2012/08/29. PubMed PMID: 22952868; PMCID: PMC3430614. DOI:10.1371/journal.pone.0044045
- Cai Z, Zhang W, Yang F, et al., Immunosuppressive exosomes from TGF-β1 gene-modified dendritic cells attenuate Th17-mediated inflammatory autoimmune disease by inducing regulatory T cells. Cell Res. 2012;22(3):607–610. Epub 2011/12/13. PubMed PMID: 22157651; PMCID: PMC3292292. DOI:10.1038/cr.2011.196
- Alonso R, Mazzeo C, Rodriguez MC, et al., Diacylglycerol kinase α regulates the formation and polarisation of mature multivesicular bodies involved in the secretion of Fas ligand-containing exosomes in T lymphocytes. Cell Death Differ. 2011;18(7):1161–1173. Epub 2011/01/21. PubMed PMID: 21252909; PMCID: PMC3131963. DOI:10.1038/cdd.2010.184
- Hedlund M, Nagaeva O, Kargl D, et al. Thermal- and oxidative stress causes enhanced release of NKG2D ligand-bearing immunosuppressive exosomes in leukemia/lymphoma T and B cells. PLoS One. 2011;6(2):e16899. Epub 2011/03/03. PubMed PMID: 21364924; PMCID: 3045385. DOI:10.1371/journal.pone.0016899
- Busch A, Quast T, Keller S, et al. Transfer of T cell surface molecules to dendritic cells upon CD4+ T cell priming involves two distinct mechanisms. J Immunol. 2008;181(6):3965–3973. PubMed PMID: 18768851.
- Xie Y, Zhang H, Li W, et al., Dendritic cells recruit T cell exosomes via exosomal LFA-1 leading to inhibition of CD8+ CTL responses through downregulation of peptide/MHC class I and Fas ligand-mediated cytotoxicity. J Immunol. 2010;185(9):5268–5278. Epub 2010/09/29. PubMed PMID: 20881190. DOI:10.4049/jimmunol.1000386
- Ventimiglia LN, Alonso MA Biogenesis and function of T cell-derived exosomes. Front Cell Dev Biol. 2016;4:84. Epub 2016/08/17PubMed PMID: 27583248; PMCID: PMC4987406. DOI:10.3389/fcell.2016.00084
- Bryniarski K, Ptak W, Jayakumar A, et al., Antigen-specific, antibody-coated, exosome-like nanovesicles deliver suppressor T-cell microRNA-150 to effector T cells to inhibit contact sensitivity. J Allergy Clin Immunol. 2013;132(1):170–181. Epub 2013/06/04. PubMed PMID: 23727037. DOI:10.1016/j.jaci.2013.04.048
- Smyth LA, Ratnasothy K, Tsang JY, et al. CD73 expression on extracellular vesicles derived from CD4+ CD25+ Foxp3+ T cells contributes to their regulatory function. Eur J Immunol. 2013;43(9):2430–2440. Epub 2013/07/15. PubMed PMID: 23749427. DOI:10.1002/eji.201242909
- Xie Y, Zhang X, Zhao T, et al. Natural CD8⁺25⁺ regulatory T cell-secreted exosomes capable of suppressing cytotoxic T lymphocyte-mediated immunity against B16 melanoma. Biochem Biophys Res Commun. 2013;438(1):152–155. PMID: 23876314. DOI:10.1016/j.bbrc.2013.07.044
- Liu Y, Gu Y, Cao X The exosomes in tumor immunity. Oncoimmunology. 2015;4(9):e1027472. PubMed PMID: 26405598; PMCID: PMC4570093. DOI:10.1080/2162402X.2015.1027472
- Zhang X, Pei Z, Chen J, et al., Exosomes for Immunoregulation and Therapeutic Intervention in Cancer. J Cancer. 2016 25;7(9):1081–1087. PMID: 27326251. DOI:10.7150/jca.14866
- Sáenz-Cuesta M, Mittelbrunn M, Otaegui D Editorial: novel clinical applications of extracellular vesicles. Front Immunol. 2015;6:381. Epub 2015/07/24. PubMed PMID: 26257745; PMCID: PMC4513568. DOI:10.3389/fimmu.2015.00381
- Agarwal A, Fanelli G, Letizia M, et al. Regulatory T cell-derived exosomes: possible therapeutic and diagnostic tools in transplantation. Front Immunol. 2014;5:555. Epub 2014/11/05. PubMed PMID: 25414702; PMCID: PMC4220709. DOI:10.3389/fimmu.2014.00555
- Krzewski K, Coligan JE Human NK cell lytic granules and regulation of their exocytosis. Front Immunol. 2012;3:335. PubMed PMID: 23162553; PMCID: PMC3494098. DOI:10.3389/fimmu.2012.00335
- Watzl C, Urlaub D Molecular mechanisms of natural killer cell regulation. Front Biosci (Landmark Ed). 2012;17:1418–1432. Epub 2012/01/01. PubMed PMID: 22201812.
- Lettau M, Schmidt H, Kabelitz D, et al. Secretory lysosomes and their cargo in T and NK cells. Immunol Lett. 2007;108(1):10–19. Epub 2006/11/10. PubMed PMID: 17097742. DOI:10.1016/j.imlet.2006.10.001
- Peters PJ, Geuze HJ, Van der Donk HA, et al. Molecules relevant for T cell-target cell interaction are present in cytolytic granules of human T lymphocytes. Eur J Immunol. 1989;19(8):1469–1475. PubMed PMID: 2789142. DOI:10.1002/eji.1830190819
- Peters PJ, Borst J, Oorschot V, et al., Cytotoxic T lymphocyte granules are secretory lysosomes, containing both perforin and granzymes. J Exp Med. 1991;173(5):1099–1109. PubMed PMID: 2022921; PMCID: PMC2118839.
- Zhu L, Senthikumar K, Oh J, et al. The NK-derived exosomes enhance NK cell-based therapy to melanoma cancer. J Nucl Med. 2016;57(Supplement 2):1393.
- Kaspar AA, Okada S, Kumar J, et al., A distinct pathway of cell-mediated apoptosis initiated by granulysin. J Immunol. 2001;167(1):350–356. PubMed PMID: 11418670.
- Saini RV, Wilson C, Finn MW, et al. Granulysin delivered by cytotoxic cells damages endoplasmic reticulum and activates caspase-7 in target cells. J Immunol. 2011;186(6):3497–3504. Epub 2011/02/08. PubMed PMID: 21296981. DOI:10.4049/jimmunol.1003409
- Ewen CL, Kane KP, Bleackley RC A quarter century of granzymes. Cell Death Differ. 2012;19(1):28–35. PubMed PMID: 22052191; PMCID: PMC3252830. DOI:10.1038/cdd.2011.153
- Lieberman J Granzyme A activates another way to die. Immunol Rev. 2010;235(1):93–104. PubMed PMID: 20536557; PMCID: PMC2905780. DOI:10.1111/j.0105-2896.2010.00902.x
- Keefe D, Shi L, Feske S, et al. Perforin triggers a plasma membrane-repair response that facilitates CTL induction of apoptosis. Immunity. 2005;23(3):249–262. PubMed PMID: 16169498. DOI:10.1016/j.immuni.2005.08.001
- Voskoboinik I, Whisstock JC, Trapani JA Perforin and granzymes: function, dysfunction and human pathology. Nat Rev Immunol. 2015;15(6):388–400. PubMed PMID: 25998963. DOI:10.1038/nri3839
- Law RH, Lukoyanova N, Voskoboinik I, et al. The structural basis for membrane binding and pore formation by lymphocyte perforin. Nature. 2010;468(7322):447–451. PubMed PMID: 21037563. DOI:10.1038/nature09518
- Reboul CF, Whisstock JC, Dunstone MA A new model for pore formation by cholesterol-dependent cytolysins. PLoS Comput Biol. 2014;10(8):e1003791. Epub 2014/08/21. PubMed PMID: 25144725; PMCID: PMC4140638. DOI:10.1371/journal.pcbi.1003791
- Thiery J, Keefe D, Boulant S, et al., Perforin pores in the endosomal membrane trigger the release of endocytosed granzyme B into the cytosol of target cells. Nat Immunol. 2011;12(8):770–777. Epub 2011/06/19. PubMed PMID: 21685908; PMCID: PMC3140544. DOI:10.1038/ni.2050
- Fan Z, Beresford PJ, Oh DY, et al. Tumor suppressor NM23-H1 is a granzyme A-activated DNase during CTL-mediated apoptosis, and the nucleosome assembly protein SET is its inhibitor. Cell. 2003;112(5):659–672. PubMed PMID: 12628186.
- Cullen SP, Brunet M, Martin SJ Granzymes in cancer and immunity. Cell Death Differ. 2010;17(4):616–623. PubMed PMID: 20075940. DOI:10.1038/cdd.2009.206
- Martinvalet D, Dykxhoorn DM, Ferrini R, et al. Granzyme A cleaves a mitochondrial complex I protein to initiate caspase-independent cell death. Cell. 2008;133(4):681–692. PubMed PMID: 18485875; PMCID: PMC2840390. DOI:10.1016/j.cell.2008.03.032
- Cullen SP, Martin SJ Mechanisms of granule-dependent killing. Cell Death Differ. 2008;15(2):251–262. Epub 2007/11/02. PubMed PMID: 17975553. DOI:10.1038/sj.cdd.4402244
- Choi YH, Jin N, Kelly F, et al. Elevation of alanine aminotransferase activity occurs after activation of the cell-death signaling initiated by pattern-recognition receptors but before activation of cytolytic effectors in NK or CD8+ T cells in the liver during acute HCV infection. PLoS One. 2016;11(10):e0165533. Epub 2016/10/27. PubMed PMID: 27788241; PMCID: PMC5082795. DOI:10.1371/journal.pone.0165533
- Lavrik IN, Krammer PH Regulation of CD95/Fas signaling at the DISC. Cell Death Differ. 2012;19(1):36–41. Epub 2011/11/11. PubMed PMID: 22075988; PMCID: PMC3252827. DOI:10.1038/cdd.2011.155
- Anderson DH, Sawaya MR, Cascio D, et al., Granulysin crystal structure and a structure-derived lytic mechanism. J Mol Biol. 2003;325(2):355–365. Epub 2002/12/19. PubMed PMID: 12488100.
- Okada S, Li Q, Whitin JC, et al. Intracellular mediators of granulysin-induced cell death. J Immunol. 2003;171(5):2556–2562. Epub 2003/08/21. PubMed PMID: 12928406.
- Gamen S, Hanson DA, Kaspar A, et al. Granulysin-induced apoptosis. I. Involvement of at least two distinct pathways. J Immunol. 1998;161(4):1758–1764. Epub 1998/08/26. PubMed PMID: 9712041.
- Falschlehner C, Emmerich CH, Gerlach B, et al. TRAIL signalling: decisions between life and death. Int J Biochem Cell Biol. 2007; 39 (7–8): 1462–1475. Epub 2007/02/14. PubMed PMID: 17403612. DOI:10.1016/j.biocel.2007.02.007
- Mincheva-Nilsson L, Baranov V Cancer exosomes and NKG2D receptor-ligand interactions: impairing NKG2D-mediated cytotoxicity and anti-tumour immune surveillance. Semin Cancer Biol. 2014;28:24–30. PMID: 24602822. DOI:10.1016/j.semcancer.2014.02.010
- Lundholm M, Schröder M, Nagaeva O, et al. Prostate tumor-derived exosomes down-regulate NKG2D expression on natural killer cells and CD8+ T cells: mechanism of immune evasion. PLoS One. 2014;9(9):e108925. PMID: 25268476. DOI:10.1371/journal.pone.0108925
- Reiners KS, Topolar D, Henke A, et al., Pogge von Strandmann E. Soluble ligands for NK cell receptors promote evasion of chronic lymphocytic leukemia cells from NK cell anti-tumor activity. Blood. 2013;121(18):3658–3665. PMID: 23509156. DOI:10.1182/blood-2013-01-476606
- Labani-Motlagh A, Israelsson P, Ottander U, et al., Differential expression of ligands for NKG2D and DNAM-1 receptors by epithelial ovarian cancer-derived exosomes and its influence on NK cell cytotoxicity. Tumour Biol. 2016;37(4):5455–5466. PMID: 26563374. DOI:10.1007/s13277-015-4313-2
- Ashiru O, Boutet P, Fernández-Messina L, et al., Natural killer cell cytotoxicity is suppressed by exposure to the human NKG2D ligand MICA*008 that is shed by tumor cells in exosomes. Cancer Res. 2010;70(2):481–489. PMID: 20068167. DOI:10.1158/0008-5472.CAN-09-1688
- Ashiru O, López-Cobo S, Fernández-Messina L, et al. A GPI anchor explains the unique biological features of the common NKG2D-ligand allele MICA*008. Biochem J. 2013;454(2):295–302. PMID: 23772752. DOI:10.1042/BJ20130194
- Hedlund M, Stenqvist AC, Nagaeva O, et al., Human placenta expresses and secretes NKG2D ligands via exosomes that down-modulate the cognate receptor expression: evidence for immunosuppressive function. J Immunol. 2009;183(1):340–351. PMID: 19542445. DOI:10.4049/jimmunol.0803477
- Tan L, Wu H, Liu Y, et al. Recent advances of exosomes in immune modulation and autoimmune diseases. Autoimmunity. 2016;49(6):357–365. Epub 2016/06/03. PubMed PMID: 27259064. DOI:10.1080/08916934.2016.1191477
- Fais S NK cell-released exosomes: natural nanobullets against tumors. Oncoimmunology. 2013;2(1):e22337. Epub 2013/03/14. PubMed PMID: 23482694; PMCID: 3583913. DOI:10.4161/onci.22337
- Chaput N, Thery C Exosomes: immune properties and potential clinical implementations. Semin Immunopathol. 2011;33(5):419–440. Epub 2010/12/22. PubMed PMID: 21174094. DOI:10.1007/s00281-010-0233-9
- Scottà C, Esposito M, Fazekasova H, et al. Differential effects of rapamycin and retinoic acid on expansion, stability and suppressive qualities of human CD4(+)CD25(+)FOXP3(+) T regulatory cell subpopulations. Haematologica. 2013;98(8):1291–1299. Epub 2012/12/14. PubMed PMID: 23242600; PMCID: PMC3729911. DOI:10.3324/haematol.2012.074088
- Julich H, Willms A, Lukacs-Kornek V, et al. Extracellular vesicle profiling and their use as potential disease specific biomarker. Front Immunol. 2014;5:413. Epub 2014/09/01. PubMed PMID: 25225495; PMCID: PMC4150251. DOI:10.3389/fimmu.2014.00413
- Kornek M, Lynch M, Mehta SH, et al. Circulating microparticles as disease-specific biomarkers of severity of inflammation in patients with hepatitis C or nonalcoholic steatohepatitis. Gastroenterology. 2012;143(2):448–458. Epub 2012/04/24. PubMed PMID: 22537612; PMCID: PMC3404266. DOI:10.1053/j.gastro.2012.04.031
- Ellwanger JH, Crovella S, Dos Reis EC, et al. Exosomes are possibly used as a tool of immune regulation during the dendritic cell-based immune therapy against HIV-I. Med Hypotheses. 2016;95:67–70. Epub 2016/09/15. PubMed PMID: 27692171. DOI:10.1016/j.mehy.2016.09.005
- Alvarez-Erviti L, Seow Y, Yin H, et al., Delivery of siRNA to the mouse brain by systemic injection of targeted exosomes. Nat Biotechnol. 2011;29(4):341–345. Epub 2011/03/20. PubMed PMID: 21423189. DOI:10.1038/nbt.1807
- Pusic KM, Pusic AD, Kraig RP Environmental enrichment stimulates immune cell secretion of exosomes that promote CNS myelination and may regulate inflammation. Cell Mol Neurobiol. 2016;36(3):313–325. Epub 2016/03/18. PubMed PMID: 26993508; PMCID: PMC4860060. DOI:10.1007/s10571-015-0269-4
- Damo M, Wilson DS, Simeoni E, et al. TLR-3 stimulation improves anti-tumor immunity elicited by dendritic cell exosome-based vaccines in a murine model of melanoma. Sci Rep. 2015;5:17622. PubMed PMID: 26631690; PMCID: PMC4668567. DOI:10.1038/srep17622
- Kim SH, Bianco NR, Shufesky WJ, et al. Effective treatment of inflammatory disease models with exosomes derived from dendritic cells genetically modified to express IL-4. J Immunol. 2007;179(4):2242–2249. PubMed PMID: 17675485.
- Ruffner MA, Kim SH, Bianco NR, et al. B7-1/2, but not PD-L1/2 molecules, are required on IL-10-treated tolerogenic DC and DC-derived exosomes for in vivo function. Eur J Immunol. 2009;39(11):3084–3090. PubMed PMID: 19757438; PMCID: PMC3075967. DOI:10.1002/eji.200939407
- Bianco NR, Kim SH, Ruffner MA, et al. Therapeutic effect of exosomes from indoleamine 2,3-dioxygenase-positive dendritic cells in collagen-induced arthritis and delayed-type hypersensitivity disease models. Arthritis Rheum. 2009;60(2):380–389. PubMed PMID: 19180475; PMCID: PMC3491653. DOI:10.1002/art.24229
- Schorey JS, Bhatnagar S Exosome function: from tumor immunology to pathogen biology. Traffic. 2008;9(6):871–881. Epub 2008/03/12. PubMed PMID: 18331451. DOI:10.1111/j.1600-0854.2008.00734.x
- Li XL, Li H, Zhang M, et al., Exosomes derived from atorvastatin-modified bone marrow dendritic cells ameliorate experimental autoimmune myasthenia gravis by up-regulated levels of IDO/Treg and partly dependent on FasL/Fas pathway. J Neuroinflamm. 2016;13:8. Epub 2016/01/12. PubMed PMID: 26757900; PMCID: PMC4710023. DOI:10.1186/s12974-016-0475-0
- Liu H, Gao W, Yuan J, et al., Exosomes derived from dendritic cells improve cardiac function via activation of CD4(+) T lymphocytes after myocardial infarction. J Mol Cell Cardiol. 2016;91:123–133. Epub 2015/12/30. PubMed PMID: 26746143. DOI:10.1016/j.yjmcc.2015.12.028
- Pitt JM, André F, Amigorena S, et al. Dendritic cell-derived exosomes for cancer therapy. J Clin Invest. 2016;126(4):1224–1232. Epub 2016/04/01. PubMed PMID: 27035813; PMCID: PMC4811123. DOI:10.1172/JCI81137
- Besse B, Charrier M, Lapierre V, et al., Dendritic cell-derived exosomes as maintenance immunotherapy after first line chemotherapy in NSCLC. Oncoimmunology. 2016;5(4):e1071008.doi: 10.1080/2162402X.2015.1071008. Epub 2015/08/12. PubMed PMID: 27141373; PMCID: PMC4839329.
- Haney MJ, Zhao Y, Harrison EB, et al. Specific transfection of inflamed brain by macrophages: a new therapeutic strategy for neurodegenerative diseases. PLoS One. 2013;8(4):e61852. Epub 2013/04/19. PubMed PMID: 23620794; PMCID: PMC3631190. DOI:10.1371/journal.pone.0061852
- Zhao Y, Haney MJ, Gupta R, et al. GDNF-transfected macrophages produce potent neuroprotective effects in Parkinson’s disease mouse model. PLoS One. 2014;9(9):e106867. Epub 2014/09/17. PubMed PMID: 25229627; PMCID: PMC4167552. DOI:10.1371/journal.pone.0106867
- Ohno S, Drummen GP, Kuroda M Focus on extracellular vesicles: development of extracellular vesicle-based therapeutic systemss. Int J Mol Sci. 2016;17(2):172. Epub 2016/02/06. PubMed PMID: 26861303; PMCID: PMC4783906. DOI:10.3390/ijms17020172
- Wang R, Xu A, Zhang X, et al., Novel exosome-targeted T-cell-based vaccine counteracts T-cell anergy and converts CTL exhaustion in chronic infection via CD40L signaling through the mTORC1 pathway. Cell Mol Immunol. 2017;14(6):529–545. PubMed PMID: 27264687. DOI:10.1038/cmi.2016.23
- Xu A, Freywald A, Xiang J Novel T-cell-based vaccines via arming polyclonal CD4(+) T cells with antigen-specific exosomes. Immunotherapy. 2016;8(11):1265–1269. PubMed PMID: 27993084. DOI:10.2217/imt-2016-0094
- Mannan S Cancer vaccine clinical trials1. Immunotherapy. 2016;8(11):1263–1264. PubMed PMID: 27993086. DOI:10.2217/imt-2016-0126
- Shah NN, Baird K, Delbrook CP, et al., Acute GVHD in patients receiving IL-15/4-1BBL activated NK cells following T-cell-depleted stem cell transplantation. Blood. 2015;125(5):784–792. PubMed PMID: 25452614; PMCID: PMC4311226. DOI:10.1182/blood-2014-07-592881
- Tian Y, Li S, Song J, et al. A doxorubicin delivery platform using engineered natural membrane vesicle exosomes for targeted tumor therapy. Biomaterials. 2014;35(7):2383–2390. Epub 2013/12/19. PubMed PMID: 24345736. DOI:10.1016/j.biomaterials.2013.11.083
- Zhuang X, Xiang X, Grizzle W, et al., Treatment of brain inflammatory diseases by delivering exosome encapsulated anti-inflammatory drugs from the nasal region to the brain. Mol Ther. 2011;19(10):1769–1779. Epub 2011/09/13. PubMed PMID: 21915101; PMCID: PMC3188748. DOI:10.1038/mt.2011.164