ABSTRACT
A corollary of the increased use of computers at primary school is a decrease in handwriting time, which may adversely affect the ability to recognise and discriminate letters. Our purpose was to examine if in-classroom handwriting and touch typewriting tuition make a difference in the recognition and discrimination of letters in novel readers. 81 Dutch primary school children (4.0 till 6.1 years), participated in this study. They were assigned to either a handwriting, a touch typewriting or an alphabet tuition control group. During three weeks they received two 20-minutes classroom-based tuition sessions weekly. All children were assessed on a recognition and discrimination letter test before and after the tuition sessions. Children recognised and discriminated more letters after tuition, irrespective of the type of training they had received. The novice readers among primary school children did not learn to recognise and discriminate letters better after classroom-based handwriting than after touch typewriting, or alphabet tuition.
1. Introduction
In recent years, the use of computers, digital tablets, and thus keyboards in primary school classrooms has dramatically increased at the expense of the time that children spend on handwriting (Mangen & Balsvik, Citation2016). This adversely affects handwriting skill and legibility. However, the reduction in handwriting tuition or penmanship practice is not restricted to handwriting; it has been found to affect children’s basic fine motor skills as well (Sülzenbrück et al., Citation2011; see also Seo, Citation2018). In fact, there is much controversy among researchers whether the acquisition of handwriting skills may even influence children’s further or broader development (Wollscheid et al., Citation2016). Ding et al. (Citation2020), for example, found a reciprocal relationship between handwriting fluency and spelling accuracy in Chinese children. Further to this point, many researchers have argued that the reduced time spend on practicing handwriting in the classroom affects children’s reading skills, including their ability to recognise, discriminate and memorise letters (Arndt, Citation2016; Longcamp et al., Citation2005, Citation2006; Mangen & Balsvik, Citation2016; Preminger et al., Citation2004). Also practitioners are increasingly worried about children’s reading skills, based on a general assumption that learning to write promotes reading. The assumption underpins various teaching programmes at primary schools internationally [e.g. The Spalding method (Citationn.d.) in the US and Australia; Reading to learn (Citationn.d.) and The THRASS Institute—Teaching Handwriting, Reading and Spelling Skills (Citationn.d.) in Australia; A Programme That Grows with Your Children—Jolly Phonics (Citationn.d.) in the UK and Pennenstreken (Citationn.d.) in the Netherlands].
The supposed relation between learning to write and reading skills is consistent with the premises of embodied cognition theories. These increasingly influential theories posit that the development of cognitive skills such as numeracy, mathematics and reading are grounded in action, rather than that they solely originate from cognitive (or brain) processes. They hold that perception and cognition cannot be separated from bodily action, and that perceptual and cognitive skills grow out of or are constituted by action (Barsalou, Citation2008; Chemero, Citation2013; Mangen & Balsvik, Citation2016). In reading, for example, learning to copy or produce the shape or topology of a letter by handwriting is thought to promote the recognition and identification of that letter (James & Engelhardt, Citation2012; Kiefer & Trumpp, Citation2012; Mangen & Velay, Citation2010). Evidence that reading skills are indeed grounded in writing skills via letter topology was first presented in research by Longcamp et al. (Citation2005). The authors did this by comparing children’s ability to recognise letters following a weekly 30-minute tuition in handwriting or typewriting across three weeks. The three- to five-year-olds were asked to either write or type twice a series of twelve letters, which were verbally and visually presented. Only the older children in this group (and not the younger children) who received handwriting tuition learnt to recognise more letters than the children who received typewriting tuition. Longcamp et al. (Citation2005) concluded that the embodied linkage between reading and bodily action is restricted to handwriting. It would not encompass typewriting, presumably because unlike handwriting, typewriting movements do not share the topological characteristics with the (visual) letter shapes (see also James & Engelhardt, Citation2012; Kersey & James, Citation2013).
Later observations by Kiefer et al. (Citation2015) did not confirm the advantage of handwriting over typewriting tuition in recognising letters. Four-to-six-year-old children received handwriting or typewriting tuition through letter games. Eight letters were taught over a four-week period, with children practicing four times a week for 25 minutes. Tuition increased children’s handwriting and typing skills, and more so than in the study by Longcamp et al. (Citation2005). Importantly, after tuition, both groups had improved letter recognition, but this improvement did not differ between the handwriting and typewriting groups. This contrasts with the hypothesis that the benefits of handwriting for letter recognition is through topological similarity, because such similarity is absent for typewriting movements, letter recognition should not be facilitated by typewriting. One possibility may be that the benefits of handwriting, if any, are only short-lived, and thus may have been concealed by the longer duration of the tuition in Kiefer et al.’s study (i.e. 400 minutes in Kiefer et al. (Citation2015) versus 90 minutes in Longcamp et al. (Citation2005)). Alternatively, the letter games in Kiefer et al. (Citation2015) involved story telling during which the target letter shapes were also presented visually and combined with an explication of the corresponding sound and lip-movements. In addition, the children were encouraged to find other words starting with the target letter. Because no control group was present that only received visual-verbal tuition, Kiefer et al.’s (Citation2015) study does not rule out that the increased letter recognition followed from this visual-verbal practice rather than from handwriting and/or typewriting. Recently, Zemlock et al. (Citation2018) did include a group to control for the effect of practicing visual recognition of letters independent of handwriting. They compared the effects of handwriting tuition of letters and digits on letter recognition, discrimination and identification in three- to seven-year-old children. The children received twelve tuition sessions in small groups across six weeks. They either practiced copying letters or digits, each letter or digit four times or they drew mazes with the exit showing a letter or a digit (i.e. visual practice of letters or digits only). The letters and digits were never pronounced. Zemlock et al. (Citation2018) found that both the letter and digit writing tuition groups showed enhanced letter recognition compared to the visual practice group. This implies that a topological similarity between handwriting movements and letter forms may be not a prerequisite for increased letter recognition. Zemlock et al. (Citation2018) argued that it is the visual guidance of the fine movement forms per se (i.e. high demand for visual control while writing letters and digits) rather than topological similarity that facilitated the recognition of letter forms. Finally, it must be noted that each studies include aspects that make them less representative for classroom-based tuition, for example setting a fixed number of letter repetitions and/or removing verbal support are unlikely to happen in regular classes. To also make the findings representative for classroom-based tuition, it is pertinent to impose as few experimental constraints on the method of tuition as possible.
If indeed a mere increase in movement demands promotes visual recognition of letters, then this may also be a conceivable effect for typewriting. In this respect, both Longcamp et al. (Citation2005) and Kiefer et al. (Citation2015) had children type in a hunt-and-peck fashion, that is, typing all letters with one and the same preferred finger. Accordingly, in both studies movement control requirements were reduced since no specific linkages were present between letter shape and typing movements. By contrast, in touch typewriting, proficient typists touch a limited number of designated keys with each of eight fingers with the hands in the same initial position, i.e. touch typewriting involves a degree of specificity of the finger movements to letter shapes that is absent in hunt-and-peck typing. Consequently, for novices, touch typewriting increases the attentional control demands of individual finger movements compared to hunt-and-peck typing.
The aim of this study was to further investigate the purported superiority of handwriting tuition for learning to recognise and discriminate letters in primary school children compared to touch typewriting tuition. Because we were not only interested in testing hypotheses based on embodied cognition but also in the degree to which tuition in handwriting and typewriting can actually make a difference in schools, we conducted a classroom-based study. Two groups of children, without previous formal reading tuition, either practiced handwriting or touch typewriting in small classroom groups. In addition, a control group followed the typical Dutch tuition method for alphabet learning that present letters visually and verbally, using rhymes and words. Children look at letter shapes, listen and repeat phonemes and words of the target letter, but do not write or type. To gauge reading skills (Le Roux et al., Citation2017), we used the letter recognition test previously used by Longcamp et al. (Citation2005) and Kiefer et al. (Citation2015), in which children selected the target letter between forms with similar but divergent shapes as the target letter (e.g. its mirror image). Additionally, we conducted a letter discrimination test, in which children distinguished the target letter between other letters—as in reading (Bara et al., Citation2004; Citation2011).
Following embodied cognition, we hypothesised that children’s letter recognition and discrimination ability would benefit both from handwriting and touch typewriting tuition compared to alphabet tuition only. We expected this advantage to be larger for handwriting tuition because of the higher degree of specificity (i.e. topological similarity) between handwriting movements and letter shapes.
2. Material and methods
2.1 Participants
Dutch primary school children who had not received any formal reading and writing tuition at school were eligible for the study (grades 1 and 2). Power analysis (G*Power 3.1) showed that a minimum number of 66 participants was needed (i.e. based on the initially planned ANOVA with repeated measures within-between interaction, effect size f = .25, α = .05 and 1−β = .95). To recruit children, four primary schools were contacted. In the Netherlands, there is no division between private and public schools, and the vast majority of primary schools are government funded. Consequently, a school’s student composition typically reflects the social-economical characteristics of the neighbourhood. The participating schools were from middle-class neighbourhoods in medium-sized cities in the Netherlands. After school teachers and the board of two schools agreed to participate, a recruitment letter, which included an information letter and informed consent form, was send to the parents. The parents of 83 children gave written consent for participation of their children. Children were then screened for motor disability, using the Movement Assessment Battery for Children-2, Dutch version test (M-ABC-2-NL, see below), and/or visual disabilities that may affect fine motor movements (i.e. writing and/or typing). No child was excluded for these reasons. Finally, two children left school shortly after the pretest (both from the alphabet control group) and were excluded from analysis. There were no further dropouts. The local university’s ethical committee approved the study.
The final sample consisted of a total of 81 children (mean age = 5.1 years, SD = 0.6). They were randomly assigned to one of the three groups (i.e. handwriting, typewriting and alphabet control group), which were matched for pretest scores on the recognition and discrimination tests. Children were first categorised as scoring above chance on neither, one or both the recognition and discrimination tests, and then proportionately distributed across the three groups (see Section 2.3). There were no significant differences in age, grade, and manual dexterity scores (subscale of the M-ABC-2-NL, see Section 2.2) between the three groups (respectively, F (2, 78) = .34, p = .71, X2(2) = .18, p = .91, and X2(2) = 2.05, p = .36, ).
Table 1. Participants characteristics as a function of group.
2.2 Material and equipment
2.2.1 Letter recognition and discrimination tests
During the pre- and posttests, the children performed the letter recognition, and the letter discrimination tests individually, and in randomised order (a computer programme pointed out which test came first). The two tests included the same twelve target letters as the letters that were introduced during tuition (i.e. c, e, f, h, j, k, m, n, r, s, t, z). The same printed block lowercase letters were used for the tests and for the lessons. The choice was based on the letters having an asymmetrical shape, such that the mirror image could be used as a distractor in the letter recognition test. In the letter recognition test (Longcamp et al., Citation2005; Citation2006), the twelve target letters were shown on a monitor. One target letter was presented together with three distractors (i.e. the mirror image of the target letter, the target letter with an extra stripe, and the mirror image of this modified target letter, (a)). In the letter discrimination test, the twelve lowercase target letters were also shown together with three distractor letters. Distractor letters were letters from the alphabet other than the target letter and including the other eleven target letters ((b)).
2.2.2 Movement Assessment Battery for children-2, Dutch version
During the pretest, the fine motor abilities were assessed by using selected items of the Movement Assessment Battery for Children-2, Dutch version (M-ABC-2-NL, Henderson et al., Citation2007; Smits-Engelsman, Citation2010). The M-ABC-2-NL is a standardised test to determine motor development. Dutch norm scores are available (Smits-Engelsman, Citation2010). Items include manual dexterity, ball skills and static and dynamic balance. For the present study, the children only performed the three items for the manual dexterity for the first age band, that is, putting coins in a box, stringing beads and drawing between lines. Reliability of the manual dexterity norm scores was reported fair to excellent (Griffiths et al., Citation2018; Van der Linde et al., Citation2013).
2.3 Procedure and design
A pretest, intervention, posttest design was used. The pretest was carried out at all schools, three to six weeks before the first lesson, while the posttest was conducted between one and three days after the final lesson. The period between pretest and the start of tuition was organisationally needed to test and assign children to groups. Children were not encouraged to read at home during this period and the remainder of the study. Yet, if they did so, or had done so before the start of the study, was not verified. In the two tests, children were individually assessed on letter recognition and discrimination by the main experimenter (first author) or a research assistant. Letter recognition and discrimination tests included 24 trials with four alternatives.
In both the recognition and discrimination test, the experimenter pronounced the target letter as a phoneme, and asked the child to point out the corresponding letter among the four shapes (in the recognition test) or the four letters (in the discrimination test) shown on the monitor. The shape or letter pointed out by the child was recorded. In both tests, all twelve target letters were assessed twice in a random order (i.e. total of 24 letters per test), each time with another configuration of neighbouring distractors. Type of distractor, the location and order of the presentation were randomised across trials. We used the Excel function “ASELECT” to randomise the order of the tests, the letters, and distractors in both the pre- and posttest.
Based on four alternatives, a binominal distribution test indicated that to perform above chance, children had to recognise or discriminate at least 11 of 24 items correctly. Based on this, children were divided into three pretest performance categories: performance in both the recognition and discrimination tests were above chance (n = 41; 50.6%); one of the two tests was above chance (n = 27; 33.3%), or both tests were performed at chance (n = 13; 16.0%). Next, children from each category were randomly assigned to the handwriting, typewriting or alphabet control group using a randomiser algorithm (also using the Excel function “ASELECT”). Subsequently, these groups were controlled for the number of children with a delay according to M-ABC-2-NL assessment (see ). It took a second round of randomisation for the four children with delay to be equally distributed across groups. This resulted in 30 children (10 girls, 2 lefthanded) being placed in the handwriting group, 29 children (12 girls, 5 lefthanded) in the typewriting group and 22 children (11 girls, 2 lefthanded) in the alphabet control group ().
2.4 Tuition
2.4.1 General procedure
Children received classroom-based tuition twice a week for a period of three weeks. Each lesson took 20 minutes and was performed in groups of two to four children. Children sat on standard adjustable school furniture. The children in the handwriting and touch typewriting groups sat at tables, while children of the alphabet control group sat in a small circle without tables. The main experimenter or the research assistant led the lessons. For each group, the letters that were practiced were presented visually and verbally in the same fixed order across lessons. In the first lesson, j and f were taught, in the second s, t and h, in the third e, r and k, in the fourth z and n, and in the fifth lesson c and m. From the second lesson onwards, the new letters and all the previously introduced letters were practiced (similarly to Kiefer et al., Citation2015). In the sixth lesson, all twelve letters were rehearsed. In each group, the lesson started with the experimenter or assistant naming the letters, that is, pronouncing them as phonemes. At the same time the letter was shown visually, printed in A4 size. In addition, the letter was contextualised as the first letter of a spoken word or as a letter clearly apparent within the spoken word. Children were encouraged to pronounce the letter and think of words of their own, which contained the target letter. On the child’s request, a letter was shown again during practice. Practice was standardised across groups with respect to the time-on-task. That is, the practice time was fixed (20 minutes), and the number of letters and words each child wrote, typed, or pronounced was free to vary.Footnote1 The rationale behind standardisation of time-on-task was to prioritise the representativeness of the classroom-based tuition relative to regular teaching methods at schools (e.g. Hurschler Lichtsteiner et al., Citation2018), which is typically organised in fixed time slots rather than in number of practice repetitions. Additionally, this allowed individual children to practice in their own pace, resulting in more repetitions among both the handwriting and typewriting group in the more skilled children. However, a minimum criterion for all children was set. They had to write or type at least 30 letters within a lesson, with the two or three newly introduced letters at least 5 times and all the previously introduced letters at least twice. For reference, this minimum per lesson was higher than the total number of repetitions in the Longcamp et al. (Citation2005) and Zemlock et al. (Citation2018) studies.
2.4.2 Handwriting tuition
In the handwriting tuition group, after the target letters were visually and verbally presented, the children were instructed to trace the letter once over a letter, which was printed in plain lines on a worksheet. They were then told to write the novel target letter on the worksheet. A standard worksheet taken from a regular Dutch handwriting method (“Schrijven leer je zo”) was used. It had no lines, and one example of the target letter was shown. Children wrote with regular pencils and could write the letter in any size they chose. Children were told to write the shape of letter correctly; the experimenter indicated were to start and how to write the letter (i.e. by showing the prescribed sequence and direction of strokes, as indicated in the manual). When the child did not adhere to these instructions (i.e. incorrect form or sequence), the experimenter or assistant would show the child how to write the letter shape correctly. From the second lesson onwards, after practicing all the newly introduced target letters, the children were asked to rehearse the target letters learned in the previous lessons, separately or in words. If children wanted to write words, the experimenter wrote these words as an example on the worksheets, so the children could copy them.
2.4.3 Touch typewriting tuition
In the touch typewriting group, children used laptops with Qwerty keyboards with all letter keys blinded with coloured stickers. After the target letters were visually and verbally presented, the experimenter or research assistant wrote the letter on the corresponding sticker and explained what finger to use. During practice, a row of letters was presented on the monitor. The children were asked to copy the letters beneath the example row using the correct finger. In the first and subsequent lessons, children always started with the left index finger for the f and the right index finger for the j. In the second lesson, after rehearsing the f and j, the new target letters were introduced and rehearsed. From the third session onwards, after rehearsing the f and j and the new target letters, the children were instructed to rehearse all letters that were learned in previous sessions, separately or in words. When the children typed the wrong letter, the experimenter or research assistant deleted it using the backspace key, and the child was asked to type the correct letter. When the child used the wrong finger (as identified by the experimenter, the research assistant, or the children themselves), the letter was deleted, and the child was given the opportunity to type the correct letter with the correct finger. Children were required to type for 20 minutes, regardless of their typewriting speed and the number of letters or words they typed. Yet, the experimenter and assistant made sure the children typed at least all the new and previously rehearsed letters in each lesson. In comparison, the fast typing children would likely make more letter repetitions than the fast writers within a lesson.
2.4.4 Alphabet tuition
In the alphabet tuition control group, in addition to the visual and verbal presentation of the target letter, the experimenter or assistant read rhymes about the target letters from a children’s book for reading (‘Het alfabet van Anders’, Oomen, Citation2002). The children pronounced the target letters (as a phoneme) and they watched pictures and talked about words that contained the target letter. Also, the experimenter or research assistant provided words that included the target letters. When talking about a letter or when a child asked for it, the letter was shown again using the A-4 page. Every child was given the opportunity to find and pronounce new words containing the target letters. The lessons in the alphabet group lasted for 20 minutes. Each lesson started with the rhymes about the novel target letters, and from the second session onwards, thereafter the previously introduced letters were rehearsed. The rehearsed letters were pronounced by the children together. When a target letter was discussed (either new or previously rehearsed), every child was given the opportunity to pronounce the letter or a word containing the letter, so that the children pronounced the letters or words containing the letters at least twice in each session. In addition, the children listened to the other children rehearsing the letters and words.
2.5 Data analysis and statistics
The number of correct answers (out of 24) on the recognition and discrimination tests was counted. Next, difference scores with respect to the number of correct answers between pre- and posttest were calculated for both tests. Thereafter, the difference scores on the recognition and discrimination tests were compared with two separate ANCOVAs with difference scores as dependent variable and pretest score as covariate. This ANCOVA was not initially planned but performed to take the observed inter-individual differences in reading skills at the start of the study into account, because these differences potentially affect the amount of improvement that can be achieved with tuition. Effect sizes were expressed using partial eta-squared (ηp2), with values of 0.01, 0.06, and 0.14 interpreted as small, medium, and large effects, respectively (Cohen, Citation2013). In addition, for both the recognition and discrimination tests, McNemar’s tests were conducted to examine whether the number of children that performed above chance in the pretest compared to the posttest increased across the three groups.
3. Results
Eight children missed one of the six lessons (i.e. three in the handwriting group, three in the typewriting group and two in the alphabet control group). However, they were included in the analyses since the pattern of results were similar with and without inclusion of the children who missed one lesson..Footnote2
shows the average number of correct answers for the recognition and discrimination tests. All three groups improved recognition and discrimination of letters from the pretest to the posttest, yet the magnitude of these improvements did not appear to differ between groups. Accordingly, one sample t-tests confirmed that the difference scores on the recognition and discrimination tests showed exceeded zero for all three groups (t’s > 2.89; p’s < .007). Additionally, the ANCOVAs for the difference scores of the letter recognition and discrimination tests revealed significant effects for the pretest score co-variate, F (1,77) = 24.2, p < .001, η2p = .24 and F (1,77) = 77.8, p < .001, η2p = .50, respectively, but not for group (resp. F (2,77) = 0.33, p < .718, η2p = .009 and F (2,77) = 0.82, p < .444, η2p = .01; ). A lower pretest score was associated with a higher difference score after training both for recognition test, Pearson’s r = − .48, p < .001, and for the discrimination test, Pearson’s r = −.71, p < .001.
Figure 2. Difference between pre- and posttest scores with standard errors for the recognition and discrimination test.
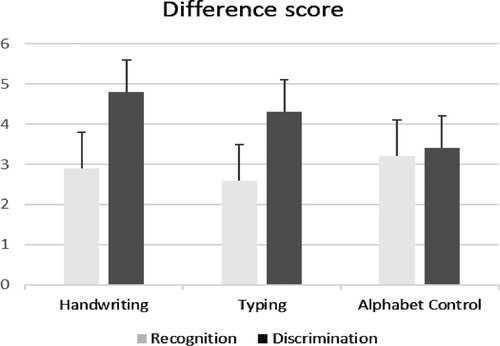
Because the absence of evidence is not equal to evidence of absence (Stefan et al., Citation2019), we performed a Bayesian repeated measured ANCOVA using JASP (see Van den Bergh et al., Citation2020). In our model, H₀ stands for the absence of a group effect in difference scores, H₁ for the presence of a group effect and in difference scores between the pre- and posttests. Using the Bayesian ANCOVA, allows an estimation of the model’s parameters based on prior distribution hypotheses, and to calculate the ratio between two conditional probabilities (Perret & Solier, Citation2022). For recognition, the model with the pretest score as a predictor is about 28,770 times more likely than the model with group as a predictor (). shows the model-averaged results for the recognition difference score. It shows for the two predictors (pretest score and groups) the prior and posterior inclusion probabilities and the inclusion Bayes factor. The difference score is 3465 times more likely for the model that includes the recognition pretest score than under the model that includes the training group.
Table 2. Model comparison for the recognition difference scores models.
Table 3. Results from averaging over the recognition models in .
Similarly, the discrimination test difference score is 1.920e +11 times more likely under the model with the pretest score as a predictor than under the model with group as predictor (). Also, the discrimination difference score is 3.983e +10 times more likely under the model that includes the discrimination pretest scores than under a model that includes the group ().
Table 4. Model comparison for the discrimination difference scores models.
Table 5. Results from averaging over the discrimination models in .
Finally, we examined the number of children within each group that performed above chance level on the recognition and discrimination tests in the pretest and posttest. It must be noted that perusal of the data showed that for the letter recognition test, the three incorrect alternatives (i.e. distractor options) were not equally strong. The two alternatives with the additional stripe were chosen less frequently (i.e. approximately 10% of the answers) than the mirror shape of the target letter (or the target letter). Consequently, rather than four, the recognition test effectively had two alternatives. For two alternatives, the binominal distribution test indicated that children must recognise at least 17 of the 24 items correctly for reliable above chance performance. Following this, we found on the pretest that 11 children (13.6%) performed above change in both the recognition and discrimination tests, 50 children (61.7%) in one of the two tests, while 20 children (24.7%) scored at chance on both tests.
shows the number of children in each of three group performing at and above chance (i.e. for the recognition test based on the modified criteria) in the pretest and posttest. First, a McNemar’s test was performed comparing pretest and posttest for all children, irrespective of tuition group membership. This indicated a significant increase in the number of children that performed above chance in the posttest compared to the pretest for both the recognition and discrimination test, χ² = 18.2, p < .001 and χ² = 17.0, p < .001, respectively. Because the number of participants in individual cells was too small to conduct McNemar’s tests within groups, we performed Chi-squared tests instead to determine differences between groups in the distribution of children performing at chance or above chance. This was done for the two tests for the pretest and posttest separately. For the recognition test, this did not reveal significant differences between the groups on the pre-test and posttest, χ² = 2.16, p = .34, and χ² = 3.90, p = .14, respectively. Similarly, for the discrimination test no significant effects were found on the pretest and posttest, χ² = .11, p = .95, χ² = 2.03, p = 0.36. This indicates that the increase in the number of children that performed above chance on the posttest is not different across tuition groups.
Table 6. Number of children performing at and above chance on the pre- and posttest recognition (a) and discrimination (b).
4. Discussion
In the current computerised era, primary school children spend increasingly more time typewriting at the expense of handwriting. Some practitioners and scientists have raised concerns that the reduced time handwriting adversely affects the learning and/or ability to read. For example, Longcamp et al. (Citation2005) compared improvement in letter recognition in children who received handwriting or typewriting tuition. They reported that both handwriting and typewriting benefit letter recognition, but crucially handwriting tuition was more effective than typewriting. However, a subsequent study did not substantiate this superiority of handwriting tuition (Kiefer et al., Citation2015). To provide a more powerful comparison between the effects of handwriting and typewriting tuition, the present study taught four- and five-year-old children to touch type. Touch typewriting entails an increased degree of specificity between finger movements and letter shapes compared to hunt-and-peck typing, and thus requires more visual control of fine movements (Zemlock et al., Citation2018). Also, a control group that received regular visual-verbal alphabet tuition was included to ascertain that any benefit of handwriting and/or typing tuition on letter recognition and discrimination could indeed be attributed to the engagement in arm and hand motor actions. We found that children, dependent on their initial reading abilities, indeed improved letter recognition and discrimination with handwriting and touch typewriting practice. Importantly, similar significant improvements were also found in the alphabet control group, suggesting that the handwriting and touch typewriting movements did not result in superior reading abilities compared to traditional visual-verbal alphabet tuition. This been said, these findings do demonstrate that classroom-based tuition in handwriting and touch typewriting did result in similar levels of reading ability as traditional visual-verbal alphabet tuition plus, presumably, either more proficient handwriting or touch typewriting skills.
Our leading hypothesis was that children would show additional benefits from handwriting and typewriting in learning to recognise and discriminate letters compared to alphabet tuition only. Embodied cognition approaches have suggested that these benefits would depend on the degree of specificity of the handwriting and typing movements to the letter shapes (James & Engelhardt, Citation2012; Kersey & James, Citation2013; Longcamp et al., Citation2005; Zemlock et al., Citation2018). Clearly, this degree of specificity is higher for handwriting than touch typewriting, but even in touch typewriting it is not completely absent as in hunt-and-peck typing, nor is the requirement for attentional control. The anticipated advantage for handwriting relative to touch typewriting was not found. However, we must be careful to conclude that the high degree of specificity or topological similarity of handwriting to the letter shapes does not have additional benefits for reading abilities. Because time-on-task rather than number of practice trials was controlled, it cannot be ruled that any advantage of handwriting over touch typewriting was concealed by more letter repetitions during touch typewriting tuition. Yet, for practitioners these classroom-based observations are significant; with equal tuition durations, touch typewriting goes not at the expense of reading abilities relative to handwriting. This been said, additional studies are needed in which the number of letter conditions is more precisely controlled to further scrutinise the degree of specificity hypothesis from embodied cognition theories.
In this respect, it is pertinent that our findings do not provide evidence that handwriting and/or touch typewriting movements facilitate the discrimination or recognition of letters over and above the visual-verbal alphabet tuition group. This indicates that among the largely novice readers in our study (i.e. the children had not received any formal reading tuition before the experiment), learning to produce arm and hand movements that were to some degree specific to the letter shapes did not promote visual recognition and discrimination of letters above verbal plus visual tuition. This was not only true for the comparably weak specificity in touch typewriting, but also for the strong topological specificity in handwriting (cf. Longcamp et al., Citation2005). Apparently, also the increased control demands for novice writing and touch typing did not further increase letter recognition and discrimination compared to alphabet training (Zemlock et al., Citation2018). Longcamp et al. (Citation2005) suggested that older children’s (i.e. approx. 4 years of age) letter recognition benefitted more from handwriting tuition than recognition by younger children (i.e. approx. 3 years of age). Possibly, because manual skills of older children are further developed. In our study, the individual differences in the letter recognition and discrimination at the start of the experiment were considerable: indeed additional analyses revealed that in the pretest, children in Dutch grade 2 had better letter recognition and discrimination than children in grade 1.Footnote3 However, analyses also showed that grade itself was not a significant co-variate, and thus did not significantly affect the observed improvements from pretest to posttest in the three tuition groups.Footnote4 Yet, initial reading ability (i.e. pretest score) was associated with improvement on both the recognition and the discrimination test. The higher the initial reading abilities, the less the children profited from the handwriting, touch typewriting and alphabet lessons. This seems a logical finding, the lessons may simply not have been sufficiently challenging for these children to further improve reading ability. The current results do not entirely rule out embodied cognition approaches as an explanatory framework for the present findings. In all three tuition groups, children were shown and asked to pronounce the target letters. That is, also children in the handwriting and touch typewriting groups were encouraged to repeat the letters verbally or (if they wanted) to make words with them. The children in the alphabet control group were actively pronouncing letters and thinking of words that contained the target letters (i.e. to resemble the typical school tuition, the children in the alphabet tuition did not practice handwriting or typewriting). Accordingly, each group actively practiced looking, producing and listening, and thus were engaged in multimodal perception. Previous research has shown that multimodal visual-auditory information can enhance visual recognition of letters in beginner readers (for overview on the letter-speech sound association, see e.g. Blomert & Froyen, Citation2010). In this respect, it is significant that producing sound not only creates auditory information, but is in itself a motor act. Consequently, it would be premature to entirely refute the general conjecture that learning to read is grounded in action. Thus, our findings suggest that in children without any previous formal reading tuition, visual-verbal alphabet tuition suffices for learning to recognise and discriminate letters. At the same time, also handwriting and touch typewriting tuition did achieve this learning to recognise and discriminate letters, but not more so than alphabet tuition only. Ultimately, therefore, the present findings do not support the presumption that a decrease in handwriting practice would lead to a decline in reading skills for single letters.
4.1 Strength and limitations of the study
A clear strength of the present study is that it was classroom-based. The way the letters were taught, including practice duration, feedback and time-on-task, was very similar to the tuition and practice children typically receive at primary school, albeit that in this study attention for the individual child was increased (i.e. in Dutch schools children regularly practice in small groups, often under guidance of volunteering parents, but also frequently practice with the entire class together). This high representativeness together with the current study’s adequate statistical power allows strong confidence about the generalisability of the findings to the classroom. As mentioned above, it must be noticed that we took duration or time-on-task as the main constraint on tuition and not the number of repetitions. Based on our experiment, we have no direct measures of the number of repetitions needed to learn the letters. We made sure that the number of repetitions of the letters in all groups was greater than in Longcamp et al. (Citation2005) and Zemlock et al. (Citation2018), but for future research it is important to delineate the relationship between number of repetitions (and time-on-task, we would argue) on improvements in reading ability. Besides that, an issue remains with respect to the initial reading skills of the current participants (see also Wollscheid et al., Citation2016). Although children had not received formal reading tuition at school, about half of the children did possess some initial reading skills. Also, it remains unclear to what extend participation in the study induced reading practice at home. It is notable that this initial ability was stronger for discriminating letter shapes. The number of children that had some rudimentary recognition was clearly lower. However, here it must be kept in mind that while we used the same recognition test as in previous research (e.g. Longcamp et al., Citation2005), two of the distractors (i.e. the (non-) mirror letters with a stripe extra) appeared insufficiently strong. This effectively may have changed the 4-choice into a 2-choice recognition task, and thus lowered the sensitivity of the test. In future studies (e.g. addressing recognition in complete novice readers) tests should be used that better control for distractor difficulty and number of repetitions of the letters. Furthermore, the results concern the ability to read isolated letters and did not include the ability to read words.
5. Conclusions
Four- to five-year-old children were not found to learn to recognise and discriminate isolated letters better after handwriting than after touch typewriting tuition, and also not compared to regular alphabet tuition. This suggests that the decrease in time spend with handwriting related to increased use of digital tools in primary schools is unlikely to significantly decrease the recognition of letters as long as children are taught to use touch typewriting and/or regular alphabet tuition is provided.
Acknowledgements
We like to thank all children and teachers who participated in our research for their time and enthusiasm. A special thanks to Elsbeth van der Boon for her research assistance.
Disclosure statement
No potential conflict of interest was reported by the author(s).
Data availability statement
The data that support the findings of this study are openly available at https://osf.io/8efgc/.
Notes
1 We did not register the exact number of letters written, typed or pronounced by individual children. Having additional assistants and/or multiple video cameras in the classroom was considered too intrusive for the normal classroom procedures.
2 We performed the same ANCOVAs as reported in the text, but then excluding the children who missed of one of the lessons. This showed similar statistical patterns; no main effects of groups for the difference score in recognition, F(2, 69) = 0.44, p = 0.645 and discrimination, F(2, 69) = 0.91, p = 0.408.
3 Unpaired t-tests showed significant effects for recognition, t(79) = 4.74, p < .001, and discrimination, t(79) = 3.93, p < .001. Note that formal tuition in reading starts in Dutch grade 3.
4 Two separate ANCOVAs with grade as co-variate showed no significant effects for recognition, F(1,76) = 1.78, p = .186 and for discrimination, F(1,76) = 2.1, p = .152.
References
- A programme that grows with your children - Jolly Phonics. (n.d.). Jolly Learning. Distracted March 25, 2021, from https://www.jollylearning.co.uk/jolly-phonics/.
- Arndt, P. A. (2016). Computer usage for learning how to read and write in primary school. Trends in Neuroscience and Education, 5(3), 90–98. https://doi.org/10.1016/j.tine.2016.07.003
- Bara, F., & Gentaz, E. (2011). Haptics in teaching handwriting: The role of perceptual and visuo-motor skills. Human Movement Science, 30(4), 745–759. https://doi.org/10.1016/j.humov.2010.05.015
- Bara, F., Gentaz, E., Colé, P., & Sprenger-Charolles, L. (2004). The visuo-haptic and haptic exploration of letters increases the kindergarten-children’s understanding of the alphabetic principle. Cognitive Development, 19(3), 433–449. https://doi.org/10.1016/j.cogdev.2004.05.003
- Barsalou, L. W. (2008). Grounded cognition. Annual Review of Psychology, 59(1), 617–645. https://doi.org/10.1146/annurev.psych.59.103006.093639
- Bergh, V. D., Van Doorn, D., Marsman, J., Draws, M., Van Kesteren, T., Derks, E. J., Dablander, K., Gronau, F., Kucharský, Q. F., Gupta, I., Sarafoglou, A. R. K. N., Voelkel, A., Stefan, J. G., Ly, A., Hinne, A., Matzke, M., & Wagenmakers, D., & J, E. (2020). A tutorial on conducting and interpreting a Bayesian ANOVA in JASP. L’Année psychologique, 120(1), 73–96. https://doi.org/10.3917/anpsy1.201.0073
- Blomert, L., & Froyen, D. (2010). Multi-sensory learning and learning to read. International Journal of Psychophysiology, 77(3), 195–204. https://doi.org/10.1016/j.ijpsycho.2010.06.025
- Chemero, A. (2013). Radical embodied cognitive science. Review of General Psychology, 17(2), 145–150. https://doi.org/10.1037/a0032923
- Cohen, J. (2013). Statistical power analysis for the behavioral sciences. Taylor and Francis.
- Ding, Y., Li, L., & Wu, X. (2020). The reciprocal relationship between handwriting fluency and spelling accuracy in Chinese: A longitudinal study. Frontiers in Psychology, 11(620). https://doi.org/10.3389/fpsyg.2020.00620
- Griffiths, A., Toovey, R., Morgan, P. E., & Spittle, A. J. (2018). Psychometric properties of gross motor assessment tools for children: A systematic review. BMJ Open, 8(10), e021734. https://doi.org/10.1136/bmjopen-2018-021734
- Henderson, S. E., Sugden, D. A., & Barnett, A. L. (2007). Movement assessement battery for children-2. 2nd ed. (MovementABC-2) (Examiner’s Manual). Harcourt Assessment.
- Hurschler Lichtsteiner, S., Wicki, W., & Falmann, P. (2018). Impact of handwriting training on fluency, spelling and text quality among third graders. Reading and Writing, 31(6), 1295–1318. https://doi.org/10.1007/s11145-018-9825-x
- James, K. H., & Engelhardt, L. (2012). The effects of handwriting experience on functional brain development in pre-literate children. Trends in Neuroscience and Education, 1(1), 32–42. https://doi.org/10.1016/j.tine.2012.08.001
- Kersey, A. J., & James, K. H. (2013). Brain activation patterns resulting from learning letter forms through active self-production and passive observation in young children. Frontiers in Psychology, 4(567), 1–15. https://doi.org/10.3389/fpsyg.2013.00567.
- Kiefer, M., Schuler, S., Mayer, C., Trumpp, N. M., Hille, K., & Sachse, S. (2015). Handwriting or typewriting? The influence of Penor keyboard-based writing training on reading and writing performance in preschool children. Advances in Cognitive Psychology, 11(4), 136–146. https://doi.org/10.5709/acp-0178-7.
- Kiefer, M., & Trumpp, N. M. (2012). Embodiment theory and education: The foundations of cognition in perception and action. Trends in Neuroscience and Education, 1(1), 15–20. https://doi.org/10.1016/j.tine.2012.07.002
- Le Roux, M., Geertsema, S., Jordaan, H., & Prinsloo, D. (2017). Phonemic awareness of English second language learners. South African Journal of Communication Disorders, 64(1), 1–10. https://doi.org/10.4102/sajcd.v64i1.164
- Longcamp, M., Boucard, C., Gilhodes, J.-C., & Velay, J.-L. (2006). Remembering the orientation of newly learned characters depends on the associated writing knowledge: A comparison between handwriting and typing. Human Movement Science, 25(4–5), 646–656. https://doi.org/10.1016/j.humov.2006.07.007
- Longcamp, M., Zerbato-Poudou, M.-T., & Velay, J.-L. (2005). The influence of writing practice on letter recognition in preschool children: A comparison between handwriting and typing. Acta Psychologica, 119(1), 67–79. https://doi.org/10.1016/j.actpsy.2004.10.019
- Mangen, A., & Balsvik, L. (2016). Pen or keyboard in beginning writing instruction? Some perspectives from embodied cognition. Trends in Neuroscience and Education, 5(3), 99–106. https://doi.org/10.1016/j.tine.2016.06.003
- Mangen, A., & Velay, J.-L.. (2010). Digitizing literacy: Reflections on the Haptics of writing. In M. H. Zadeh (Ed.), Advances in haptics (pp. 385–402). IN-TECH. https://doi.org/10.5772/8710
- Oomen, F. (2002). Het alfabet van Anders (1ste editie). Rubinstein Publishing BV.
- Pennenstreken. (n.d.). Uitgeverij Zwijsen. Distracted March 25, 2021, from https://www.zwijsen.nl/lesmethodes/pennenstreken/?gclid = EAIaIQobChMI_JyduobL7wIVyPZRCh31OgwNEAAYASAAEgIAefD_BwE.
- Perret, C., & Solier, C. (2022). Application of a Bayesian approach for exploring the impact of syllable frequency in handwritten picture naming. Journal of Cognitive Psychology, 1–14. https://doi.org/10.1080/20445911.2022.2029459
- Preminger, F., (Tamar) Weiss, P. L., & Weintraub, N. (2004). Predicting Occupational performance: Handwriting versus keyboarding. The American Journal of Occupational Therapy, 58(2), 193–201. https://doi.org/10.5014/ajot.58.2.193
- Reading to learn. (n.d.). https://readingtolearn.com.au/. Distracted March 25, 2021, from https://readingtolearn.com.au/.
- Seo, S. M. (2018). The effect of fine motor skills on handwriting legibility in preschool age children. Journal of Physical Therapy Science, 30(2), 324–327. https://doi.org/10.1589/jpts.30.324
- Smits-Engelsman BCM. (2010). Nederlandse bewerking van de Movement Assessment Battery for children, handleiding. Pearson.
- Stefan, A. M., Gronau, Q. F., Schönbrodt, F. D., & Wagenmakers, E. J. (2019). A tutorial on Bayes factor design analysis using an informed prior. Behavior Research Methods, 51(3), 1042–1058. https://doi.org/10.3758/s13428-018-01189-8
- Sülzenbrück, S., Hegele, M., Rinkenauer, G., & Heuer, H. (2011). The death of handwriting: Secondary effects of frequent computer Use on basic motor skills. Journal of Motor Behavior, 43(3), 247–251. https://doi.org/10.1080/00222895.2011.571727
- The spalding method. (n.d.). https://www.spaldingeducation.org/. Distracted March 25, 2021, from https://www.spaldingeducation.org/.
- The THRASS Institute – Teaching Handwriting, Reading and Spelling Skills. (n.d.). https://www.thrass.com.au/. Distracted March 25, 2021, from https://www.thrass.com.au/.
- Van der Linde, B. W., van Netten, J. J., Otten, B. E., Postema, K., Geuze, R. H., & Schoemaker, M. M. (2013). Development and psychometric properties of the DCDDaily: A new test for clinical assessment of capacity in activities of daily living in children with developmental coordination disorder. Clinical Rehabilitation, 27(9), 834–844. https://doi.org/10.1177/0269215513481227
- Wollscheid, S., Sjaastad, J., & Tømte, C. (2016). The impact of digital devices vs. Pen(cil) and paper on primary school students’ writing skills – A research review. Computers & Education, 95(C), 19–35. https://doi.org/10.1016/j.compedu.2015.12.001.
- Zemlock, D., Vinci-Booher, S., & James, K. H. (2018). Visual–motor symbol production facilitates letter recognition in young children. Reading and Writing, 31(6), 1255–1271. http://doi.org/10.1007/s11145-018-9831-z