Abstract
Numerous arthropod species represent potential targets for gene-drive-based population suppression or replacement, including those that transmit diseases, damage crops, or act as deleterious invasive species. Containment measures for gene drive research in arthropods have been discussed in the literature, but the importance of developing safe and effective standard operating procedures (SOPs) for these types of experiments has not been adequately addressed. Concisely written SOPs link safe work practices, containment measures, institutional training, and research-specific protocols. Here we discuss information to be considered by principal investigators, biosafety officers, and institutional biosafety committees as they work together to develop SOPs for experiments involving gene drive in arthropods, and describe various courses of action that can be used to maintain the effectiveness of SOPs through evaluation and revision. The information provided herein will be especially useful to investigators and regulatory personnel who may lack extensive experience working with arthropods under containment conditions.
1. Gene drive systems are diverse
Gene drive systems hold great promise for introducing beneficial traits into natural arthropod populations in order to control invasive species and reduce disease transmission for the protection of human health, endangered species, and agriculture within a medically, ecologically, and/or economically useful timeframe [Citation1]. Nonetheless, even laboratory-based gene drive research has raised social, political, and environmental concerns on an international scale [Citation2–6]. Proof-of-principle experiments using CRISPR/Cas9 (clustered regularly interspaced short palindromic repeats/CRISPR-associated protein 9) as a mechanistic component for gene drive based on homology-driven DNA repair have recently demonstrated that these synthetic genetic elements can successfully invade laboratory populations of yeast, fruit flies, and mosquitoes [Citation7–10].
Although Cas9-based gene drive has received significant attention due to its ease of design [Citation11,12], other naturally occurring and experimentally derived gene drive systems which do not utilize programmable nucleases technology may be also be proposed. These alternative technologies have a wide array of genetic properties governing how quickly they may spread through populations and how long they might persist (see Champer et al. [Citation1] and Marshall and Akbari [Citation13]). For example, underdominance-based gene drives (UD) using translocations or toxin-antidote systems are unable to spread when they are rare in a population, but can spread rapidly when they become the predominant genotype [Citation14–16]. A form of gene drive referred to as Maternal Effect Dominant Embryonic Arrest (MEDEA) drives the rapid spread of genes in a population using a maternal toxin and a zygotic rescue, such that embryos without the zygotic rescue gene succumb to the toxin [Citation17]. Unlike Cas9-based gene drive (or another programmable site-specific nuclease), neither UD nor MEDEA rely on DNA break repair to fix ‘cargo’ genes conferring pathogen-resistance or another beneficial trait into a population. Other forms of gene drive aim to reduce population size by causing the production of males to be favored [Citation18–20]. With these examples we emphasize that the concept of gene drive is not mechanistically linked to any particular technology, and we can expect even more examples of gene drive in the coming years. Importantly, each form of gene drive presents a different set of biological constraints. Therefore, a case-by-case basis evaluation of containment measures, protocols, and procedures commensurate with any identified hazards posed by each specific combination of gene drive mechanism and host organism should be conducted.
2. Laboratory containment of gene drive organisms
Soon after the successful demonstration of Cas9-based gene drive in Drosophila melanogaster, Akbari et al. [Citation21] proposed a series of recommendations to limit the potential accidental spread of gene drive containing organisms in nature, and identified the following four types of confinement strategies: molecular confinement (splitting gene drive into multiple components, using artificial target sequence); ecological confinement (performing work where the organism cannot live in the wild; reproductive confinement (organisms incompatible with wild genotypes); and barrier confinement (physical laboratory structures and work practices). Akbari and colleagues suggested that all laboratory gene drive research utilize at least two of these measures [Citation21].
For some applications, particularly when the development of an autonomous gene drive is not desired, these recommendations are quite sound. If there is no compelling experimental need to combine all the required components together, it is indeed preferable to implement them separately. Ultimately, the use of molecular, reproductive, and/or ecological confinement only serves to defer or delay the development of a fully functional gene drive, as eventually all the components do need to be combined into a functional unit if it is to be experimentally evaluated. Likewise, such experiments will eventually need to be moved to locations where the climate is suitable for the organism to live, and eventually such experiments will need to involve mating with wild individuals.
Two recent studies using Cas9 to drive transgenes into caged laboratory populations of malaria mosquitoes [Citation9,10] were performed in Southern California and the United Kingdom, locations which are devoid of malaria vectors. A requirement for ecological or reproductive confinement for gene drive experiments would bar scientists in malaria-endemic countries from developing their own gene drive-based technology. Such inequities are theoretically expanded by considering that the nations with the most ecologically diverse territory could therefore perform the widest range of possible gene drive experiments, while less ecologically diverse nations in tropical regions become increasingly excluded, rendering the countries that bear the brunt of vector-borne disease burden unable to pursue solutions to their own problems through modern biotechnology.
Biosafety strategies aimed at maintaining the physical containment of genetically modified organisms correspond to what Akbari et al. [Citation21] described as a single confinement strategy (barrier). However, those with experience and training in handling infectious organisms will quickly recognize that a single confinement strategy is actually an amalgam of engineering controls (physical structures), personal protective equipment, work practices/operating procedures, and training. In essence, the pillars of modern biosafety. So how do gene drive containing organisms compare with the containment of infectious agents, including dangerous human pathogens? Are there lessons that have already been learned by infectious disease researchers that can be applied to gene drive research? Have all of the gaps in oversight and compliance been identified in the context of gene drive research? What areas can be improved further? Finally, what new issues might arise as gene drive research scales up from proof-of-principle laboratory experiments to large-scale contained trials? These questions will be considered over the course of this review, with an emphasis on the development of useful and effective operating procedures and oversight practices. Physical containment measures have been well-described elsewhere [Citation22–24], and will only be mentioned here in the context of work practices specifically related to gene drive experiments.
3. Review and oversight of laboratory gene drive research in arthropods
At institutions receiving funding from the National Institutes of Health (NIH), all gene drive research involving recombinant or synthetic DNA in arthropods must be approved by an Institutional Biosafety Committee (IBC) prior to initiation. However, institutions, organizations or individuals without NIH funding would similarly benefit from such an external review of any proposed gene drive research. IBCs and biosafety officers in turn will consult the Biosafety in Microbiological and Biomedical Laboratories [Citation25] and NIH Guidelines for Research Involving Recombinant or Synthetic Nucleic Acid Molecules [Citation26] for establishing containment levels. However, the current versions of these documents do not address arthropod containment or any type of gene drive system directly. Thus, under the current regulatory conditions, experiments involving gene drive in arthropods could potentially be approved at biosafety level 1 (BL-1) containment. Indeed, this may even be appropriate for some forms of gene drive such as UD, where transgenic individuals are likely to be strongly selected against in the case of accidental release.
For most gene drive research in arthropods, particularly work in disease vector mosquitoes, reviewing entities will likely also make use of the Arthropod Containment Guidelines (ACGs) developed by the American Committee of Medical Entomology, a subcommittee of the American Society for Tropical Medicine and Hygiene [Citation22]. The ACGs specify four containment levels (ACL1–4), and largely approach containment and practices from a vector-borne pathogen-based perspective. While making no mention or distinction for gene drive, the ACL-2 guidelines recommend ACL-2 containment for research involving transgenic arthropods [Citation22]. Other arthropod containment guidelines are used in various countries outside the U.S.A., but do not differ substantially from the ACG [Citation24]. While Benedict et al. [Citation24] recently described additional recommendations to augment ACL-2 practices in the context of gene drive, there remains little guidance available in best practices for developing operating procedures and protocols to meet the performance-based criteria laid out in the ACG. At present, substantial overlap exists between those performing gene drive research in disease vectors and those with long-standing experience in ACL-2 or higher containment practices, but this may not be the case as the technology develops. Given the array of potential applications in agriculture and invasive species biology, research involving gene drive in arthropods is likely to rapidly expand beyond vector biology over the next several years, presenting new challenges to PIs, biosafety personnel, IBC members, and institutions at large.
4. Developing streamlined, useful, safe, and effective SOPs for laboratory gene drive research
Standard operating procedures (SOPs) are required for mandated compliance with Good Laboratory Practice Standards in the pharmaceutical industry [Citation27], and are also used to ensure that clinical study protocols comply with Good Clinical Practice Guidelines [Citation28,29] in accordance with national and international regulatory policies. Similarly established SOPs are also used in hospitals and patient care facilities to ensure quality of care [Citation30,31]. In establishing similar safety guidelines for other relevant areas of industry and research, the National Academies of Sciences, Engineering, and Medicine created Chemical Laboratory Safety and Security: A Guide to Prudent Chemical Management under the auspices of the U.S. Department of State [Citation32]. Recently, this was followed by the Guide to Developing SOPs to assist researchers and laboratory managers in industry and academia in developing their own task-specific SOPs [Citation33]. Years of review and revision have demonstrated the importance of SOPs in maintaining a high level of safety for patients and staff in a wide range of settings [Citation27,31]. Given the uncertainty regarding hazards posed by gene drive research in arthropods [Citation6,11,21,34], it likely should be subjected to stringent standards for SOP development and implementation as well.
In their most basic form, SOPs serve as a written record of the procedures to be performed. SOPs maintain consistency in the work process, regardless of who carries out a procedure, by providing a task-specific set of instructions for workers, and in doing so minimizes systematic error which might otherwise be introduced by variation stemming from differences in the performance of tasks between different workers. An SOP also instills accountability by compelling personnel to follow the designated task-specific instructions exclusively with a document that carries management’s signed, dated approval. By accomplishing these objectives, SOPs ultimately guard the integrity of the containment facility. SOPs are especially relevant with regard to regulatory compliance because the same instructions that ensure consistency in experimental procedures should also make certain that no lapses in safety occur, thereby ensuring the safety of the investigator, study subjects, general public and the environment. In this context, SOPs act as a link connecting risk assessment and research planning with safety and technical training to ensure the scientifically useful outcome of the procedure, as well as regulatory compliance.
In basic research, SOPs ensure that experiments are performed according to a predetermined set of conditions that are designed to ensure both the outcome of the procedure and the safety of those personnel performing it [Citation27]. In the context of hazardous chemicals, SOPs ensure that such substances are handled and disposed of properly to avoid exposing the public or the environment to potentially harmful chemicals [Citation33]. For research involving human or animal pathogens, SOPs must also safeguard the safety of the public and the environment by ensuring that applicable containment measures are not compromised during the course of the experiment [Citation25], and SOPs for research involving arthropod vectors infected with such pathogens must further ensure that measures intended to confine the animal to the research facility are maintained [Citation22].
Experienced investigators will have undoubtedly developed and standardized many containment-related procedures, regardless of whether these procedures have been formalized and documented or remain as an unwritten part of the lab culture. Depending on the institutional culture, biosafety officers and other regulators may want to see far more extensive documentation. As many institutions do not require lab-specific SOP manuals for BL-1 work, these two cultures may not intersect prior to the consideration of a gene drive protocol at ACL-2. We emphasize that both extremes (insufficient documentation, over documentation) are undesirable outcomes. While it is generally intuitive that a failure to effectively document standard practices can result in research staff deviating in practice over time, the utility of SOP manuals diminishes when they become too technical, too repetitive, or too focused on ‘what-if’ scenarios, as they are unlikely to be remembered and become difficult to keep updated [Citation33,35]. Instead, PIs and biosafety oversight personnel should work together to document in a succinct format an SOP manual that can be read and remembered by lab personnel, is amenable to updates as needed, and yet still covers all essential practices. Rather than a compendium of possible scenarios, an SOP manual should document the concepts and reasoning used to maintain containment so that lab personnel can reasonably extrapolate minor variations in a protocol. In the following sections, we provide examples of performance-based criteria specified by the ACG, and some considerations that can aid in the preparation of SOPs to meet these specifications. This list is not meant to be exhaustive, but can be used as a starting point, particularly for those who have not worked in or provided oversight for arthropod rearing facilities operating under stringent ACL-2 containment.
4.1. Access: Restricted to authorized, trained personnel only
As stated by Benedict et al. [Citation24], there are essentially three ways for a contained insect to escape from the laboratory: (1) through the entrance, (2) down a wastepipe, or (3) through a breach in structural barriers (walls, ceiling, etc.). While simple to state, how access is granted and who has access to an ACL-2 insectary containing gene drive organisms is critical to maintaining containment. To start, the definition of access in this case is itself unclear and requires clarification. Does access mean permission? Does it mean the physical ability to enter through some form of key or pin? Practically speaking, it is likely to be a combination of both, as it is doubtful that PIs at university-based labs will be given complete key control of managing access to a laboratory. Depending on any relevant pre-existing departmental and university procedures, this task will likely be controlled centrally, with the PI able to grant permission, but not involved directly in the issuance of actual keys.
For facilities that utilize proximity readers or other forms of electronic access, it is even less likely that a PI will be able to personally control who has the ability to physically enter the insectary. This responsibility is instead often entrusted to institutional personnel that manage key access. One or more local administrators within a department may also maintain access to the insectary based on a master key or master code. Facilities management personnel or their subcontractors, inventory control personnel, and emergency responders may all have independent policies and procedures for obtaining access to a given workspace that may well circumvent the control of the PI or departmental administrators. Maintenance staff might obtain unsupervised access due to lapses in institutional key control, and may even attempt to access these restricted areas outside of normal working hours as part of their job-related duties. In the case of newly constructed or renovated laboratories, institutional facilities management personnel or private subcontractors may continue to have access to the facility after the completion of construction if the severing of their access goes overlooked.
To ensure compliance, PIs overseeing gene drive research studies in arthropods should, therefore, be proactive in working with all relevant agencies within their institution to maintain control over access to the insectary suite. Others who may seek to enter the facility include students, laboratory workers or faculty who may be interested in a shared piece of equipment, vendors and field technicians to service laboratory equipment, senior administrators, members of the press, even family members or friends of members of the lab. Identifying these disparate parties is essential to establishing who is allowed to enter the facility, and under what conditions, if at all.
Unsupervised access to the insectary is thus likely to be restricted to research personnel who have successfully completed SOP training and received permission from the PI, and these SOPs should clearly describe the training required to obtain unsupervised access and the frequency at which refresher training must be completed. All others, including facilities technicians, non-research staff, and untrained research staff should not be allowed to enter the insectary unless accompanied by trained research personnel to ensure that containment measures are not compromised during the course of their activities. Substantial thought should be given to the use of proximity readers or electronic access controls in place of hard keys/pin numbers. While the latter are obviously lower in cost, the combination of ease of sharing and inability to track access to specific individuals makes these forms of access substantially less secure/useful.
Box 1.
Critical questions to be addressed in an Access SOP: What should be the requirements for authorized unsupervised access to the facility? Who will have, or might have the potential to gain physical access? How could these individuals gain access without permission? What types of visitors will be permitted to enter? What information should be provided to visitors? Can access be tracked remotely? Will sign-in be recorded manually? What mechanisms will allow access to be revoked?
Assessing an Access SOP: Periodic audits of electronic and written records can confirm the identities of persons entering the facility. Do sign-in records show that unauthorized persons are entering the facility? Is there evidence that someone has entered the facility without signing in? Do members of the lab confront and/or report unaccompanied individuals they believe are not authorized to enter the facility?
4.2. Entry/Exit: Insectary is separated from corridor via at least two self-closing doors
The requirement for separation is typically, but not always, accomplished by passage through a relatively small double-door vestibule. With this SOP, we emphasize that having the physical structure of a vestibule is meaningless if there are no procedures for how to progress through this area in a manner consistent with why this recommendation exists in the ACG in the first place. That is, no matter whether the barriers are manually operated porch screen doors or interlocking steel fire doors, if lab workers are passing through this zone rapidly without concern for their surroundings, they will not be in a position to identify, and hence stop, an escaped insect. Implementing these procedures and enforcing them can be a challenge, as each researcher may enter/exit the facility a dozen times or more daily depending on their role and responsibilities, while escaped insects in this area are likely to be extraordinarily rare.
In addition to managing access, a manual sign-in present in the vestibule can have the added benefit of slowing fast moving researchers down as they enter/exit the facility. Likewise, entry requirements might include the donning/doffing of insectary-dedicated lab coats or other PPE. While these activities have their own merits, this SOP should clearly spell out the responsibilities of laboratory workers for examining both their surroundings (walls, ceiling, etc.) and their own person (clothes, hair, etc.) for resting, clinging, or flying insects. In additional to visual inspection of the room, flying insects can sometimes be heard, provided background noise from the HVAC systems is low enough. Therefore, adding a listening check to the SOP can help to focus researchers’ attention on escaped insect surveillance in the vestibule.
Some vestibules are so small that a single adult may feel squeezed as they pass through, while others might be spacious enough for large equipment to pass through. As a result, vestibules may also end up being used for storage. The entry/exit SOP should be clear regarding which types of items may be stored, which may not, and why, with the goal of keeping only essential items and minimizing clutter. Where possible, workers’ personal items such as coats and backpacks should also be kept to a minimum. Time spent in the vestibule can also be used to inform guests or other visiting personnel of any hazards present inside the facility, if not done previously, as well the procedures needed in case of an incident during their visit.
Box 2.
Critical questions to be addressed in an Entry/Exit SOP: How should entry/exit be coordinated? If interlocking doors are present, is there a manual override? How long must individuals pause in the vestibule before proceeding into the insectary/corridor? Will a specific list of inspections be performed prior to entrance/exit? What PPE should be donned/doffed? What types of personal items should be allowed to remain in the vestibule? What will constitute proper/improper attire? Should sign-in/out be required?
Assessing an Entry/Exit SOP: Do doors self-close correctly and completely? How long are individuals pausing in the vestibule before proceeding? If decoy arthropods (e.g. plastic model or dead non-gene-drive specimen) are placed in the vestibule, are they noticed/reported? Is PPE being stored and disposed of properly? Does clutter accumulate in the vestibule over time? Laboratory managers and PIs should periodically observe individuals entering/exiting the facility and address potential concerns.
4.3 Facility integrity
Although the ACG recommend a full evaluation of physical containment measures (integrity of walls, screens, traps, etc.) on an annual basis, we have found that additional weekly or daily spot checks of facility integrity are necessary to ensure continuous containment, especially in new facilities where any material flaws might not have been noticed yet. Laboratory staff should regularly inspect likely sources of containment failure, such as screens over HVAC penetrations and caulking around fixtures, such as surface mounted lights. In particular, we have observed that door closures and latches need to be continually adjusted to ensure that doors self-close completely. While these tasks may be done informally, keeping good records can help identify more long term patterns in lapses in physical containment measures by continually documenting the condition of the facility. For example, caulking may be prone to deteriorating more rapidly under certain dry/wet conditions, or screens made from certain materials be more easily compromised over time. PIs may perform many of these spot checks, designate a lab manager, rely on the collective input of all researchers in the lab, or some combination thereof, but the operating procedures should make it clear if this is an expectation.
In addition to regular spot checks, there may be other times when a thorough integrity check is warranted. For example, heavy thunderstorms may result in building leaks that can damage walls or ceilings. Hot spells can challenge building HVAC systems resulting in loss of negative pressure, failure of doors to self-close properly, and/or rising temperatures. Many of these types of events are not predictable during commissioning but become known recurring problems over time and can eventually be anticipated with some regularity.
While it is not possible or practical to predict every type of disaster scenario that may impact containment of gene drive organisms, a few illustrative scenarios included with the SOPs can serve as examples as to how, why, and what decisions need to be made. For example, a compromised screen may be discovered in the vestibule, with gene drive insects sealed in a growth chamber with three doors of separation (the vestibule door, the door to the gene drive manipulation room, and the growth chamber door). Researchers may be instructed to keep the growth chamber sealed until the screen can be replaced, a relatively minor disruption. Similar actions may be taken for small failures in caulking or other seals. By contrast, a broken pipe in a lab located right above the insectary may cause sufficient flooding to result in a partial collapse of the ceiling. This may warrant the implementation of procedures for the immediate devitalization of gene drive insects (perhaps by increasing the temperature in their growth chamber above the thermal death point). As there are gray areas between these scenarios, PIs and biosafety officers will want to work out in advance the criteria to be used that trigger either a suspension of work or the rapid elimination of gene drive insects to prevent escape in the case of a compromise in the physical structure of the insectary.
Annual facility shutdowns are common in high containment labs, and provide a means to thoroughly inspect facility integrity and perform preventative maintenance that otherwise could not be safely done while experiments were ongoing. These shutdowns are facilitated by the fact that the pathogens used in these labs can be safely stored and locked away in freezers. With the exception of aedine mosquitoes, the eggs of which can remain viable after desiccation for months, most insect species must be bred continuously. This complicates the ability to perform a full shutdown, as researchers may require daily access to insects throughout the year without interruption. With this in mind, procedures should be developed to shut down as much work as possible, perhaps finding a window of just 1–2 days where insects can remain sealed in environmental chambers to allow preventative maintenance and a full inspection.
Box 3.
Critical questions to be addressed in a Facility Integrity SOP: How often should different aspects of the facility be inspected? What course of action should be taken if a potential breech of containment is observed? For which types of potential breeches should laboratory work be suspended? When is a breech serious enough to warrant immediate destruction of gene drive containing organisms? What type of preventative maintenance should be performed? Should an annual shutdown be performed to allow preventative maintenance of physical barriers, such as the replacement of screened vent/drain coverings and door sweeps and growth chamber cleaning? Who will serve as emergency contacts in case of immediate facility concerns, such as a wall breech, plumbing leak, or faulty door closure?
Assessing a Facility Integrity SOP: Are cracks or penetrations found during the annual inspection that were not reported previously? If given to suspend activities at any time, were orders to shut down or suspend activities followed by all lab members? How long did it take them to suspend their activities? If lab members are vigilant throughout the year, detailed inspections during an annual shutdown should reveal few defects. Instead, this time can be used for replacing caulk, screens or seals before these items show signs of wear or deterioration.
4.4. Devitalization, waste disposal, and routine decontamination
While there is a general appreciation for the need to ultimately dispose of gene drive containing organisms and associated materials, a waste disposal SOP should spell out exactly how this should be done for the insectary facility. Most obviously, devitalization procedures must ensure that no living arthropods are removed or inadvertently released from the facility. Devitalization procedures should be developed that kill the organisms housed with a high level of certainty at all life stages, and these procedures should be developed before gene drive-related research activities are initiated. We emphasize that different devitalization procedures may be needed for different organisms or life stages, and solid and liquid waste containing the organism may require different devitalization methods. In many cases, boiling or freezing will be effective for killing arthropods, but this should be confirmed empirically as some species or their eggs may be resistant to freezing or require longer periods at these temperature extremes. We stress that, in most cases, devitalization of organisms or associated materials should occur prior to disposal in a waste container, even if such material is destined for the autoclave (an exception being if an autoclave was present within the containment barrier and there was no delay between when the items were placed in solid waste and subsequent sterilization in an autoclave).
A decision must be made regarding whether multiple waste streams will be allowed within a facility. That is, will there be dedicated receptacles for gene drive organisms/materials? Or will they be placed in the same waste stream as other insectary waste? Having a single waste stream simplifies procedures and can prevent mix-ups, and may or may not enforce a higher standard of waste treatment on the rest of the facility. To reduce the burden on waste treatment, it is good practice to avoid bringing extensive packaging, wrappers, or other materials into the insectary. If gene drive research activities are physically segregated from other rearing as recommended [Citation24], then waste collection is simplified as well. Solid waste should be collected within disposable liners in sturdy receptacles with hands-free operated lids, and procedures should specify when these are emptied and who performs this task. In our experience, the result is consistently overflowing waste containers if these duties are not clearly specified.
Some insectary facilities will have dedicated autoclaves for treating solid waste, others will have to safely transport waste to an autoclave elsewhere in the building, or potentially to another building. Procedures should clearly describe how this transport step is performed, and what to do if the autoclave is occupied or out of commission. For example, researchers may place an autoclave tray with solid waste into a large, covered, unbreakable container which is bolted to a transport cart. If the waste cannot be immediately loaded, it is returned into the containment barrier of the insectary, and is not left unsupervised in the shared autoclave area. The autoclave itself should be periodically evaluated by facilities technicians to confirm it is functioning properly in accordance with institutional policy. Procedures should specify how often testing is performed using chemical indicators (every load?) or a biological indicator (weekly, biweekly or monthly?), as well as whether autoclave indicator tape, chemical, or biological indicators are required for load verification.
Liquid waste, particularly for work with disease vector mosquitoes, is likely to be treated quite differently than solid waste. As rearing activities can generate many tens or even hundreds of liters of wastewater per week, it is likely impractical to collect, transport, and autoclave such volumes. Fortunately, the high temperatures and pressures required to kill hardy microbes are far beyond what is necessary to devitalize arthropods. Small to medium volumes can be effectively boiled (hot plate or microwave), and large volumes can be filtered using fine sieves. If specialized liquid collection equipment is available for processing large volumes, instructions for its use and maintenance should be provided.
Box 4.
Critical questions to be addressed by a Waste Disposal SOP: For solid waste, who will be responsible for the timely disposal of containers? What types of material may be placed in these containers? What items or materials should not be disposed of as solid waste? How should solid material be treated prior to being transported to an autoclaving facility? How will waste be transported to an autoclave? How will liquid waste be processed? Will small and large volumes be processed differently? Will specialized equipment be required for devitalizing liquid waste?
Assessing a Waste Disposal SOP: Are containers being emptied regularly? Are waste containers being overfilled? Are items being handled properly during transport to the autoclave? Are chemical and microbiological tests being performed routinely? Have chemical and microbiological tests identified autoclave malfunction in the interim between monthly autoclave inspections? Regular inspections by lab managers and PIs should confirm that workers are adhering to the SOP.
4.5. Escaped arthropod handling, monitoring, and accidental release reporting
In the course of manipulating cages containing hundreds if not thousands of adult mosquitoes, some small scale accidental releases within the containment environment may eventually occur. Following validated good practices will help minimize the number of escapees, but the frequency of such events within the contained environment may never be brought to zero over the long term. When an accidental release within an insectary does occur, the escaped insect automatically becomes the responsibility of everyone in the room. However, it is important to make clear to research personnel that they should complete any tasks in progress prior to assisting with the capture, as hastily dropping an aspirator or neglecting to secure the sleeve of a cage can inadvertently release tens or hundreds more insects. Procedures should clarify how events such as these are recorded/reported, and what to do if the escaped insect cannot be found or the number of escaped insects is not precisely known. Training in this area is particularly critical, as research staff have an inherent conflict of interest that must be managed when it comes to reporting released insects. On the one hand, accurate and timely reporting is essential to adjusting operating procedures or providing additional training so that the work can be performed safely. On the other hand, research staff may have concerns about how reporting (and the events that triggered it) are used in their own performance evaluations. Operating procedures should anticipate this and provide potential solutions, such as a channel for anonymous reporting, reporting to a party outside their chain of command, or limiting/prohibiting working alone.
Unlike releases occurring during active manipulations, the escape of adult flying insects through a damaged or worn cage or an improperly covered larval/pupal pan may do so unobserved. Therefore, there may be times when an escaped arthropod is detected in an insectary room as a result of factors unrelated to activities in which cages are being handled and the insects are being manipulated. We refer to such escaped individuals as ‘free-flying’ insects herein because the exact origin and genotype of such insects is uncertain. Continuous inspection of cages and strict adherence to SOPs for species-specific rearing practices should avoid such releases. However, a variety of factors, such as unknown defects in materials, changes in material providers, and fluctuations in growth chamber temperatures (resulting in an increased rate of development), can contribute to such events. In addition to the capturing, killing, and disposal of free-flying insects, these types of release events must also be tracked and reported to assist in determining the root causes. For example, if the released individuals are all smaller males, perhaps they were able to force their way through the mesh screen due to an increase in rearing density. If free-flying insects are only observed in the early morning, it is likely that adults are emerging from insufficiently covered pans or a compromised screen. If free-flying insects are observed in the middle of the day when work has been ongoing, perhaps they were released unknowingly by another individual. In any case, the assessment and, if necessary, revision of SOPs and training protocols should reduce the frequency and magnitude of similar future events (Figure ).
Figure 1. Monitoring baseline levels of free-flying insects within the contained insectary. The number free-flying insects discovered in the containment facility should be monitored continuously and new SOPs or additional training provided as needed to keep baseline levels in rearing spaces as close to zero as possible.
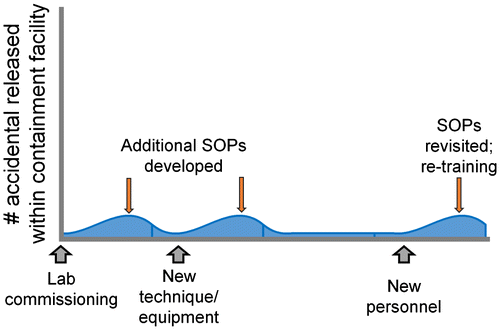
The ACG recommend the use of sticky traps and/or light traps to assist in the monitoring of escaped insects. While these can indeed be useful, we caution against relying on such traps as a containment measure, or even as a sensitive monitoring method. As only a subset of free-flying individuals will be caught in a trap, the presence of captured individuals can be expected to represent only a small portion of the actual escapees. That is, the problem should likely have been identified much earlier by the researchers who have been working in the facility. In addition, if traps are not checked and cleaned regularly (daily, or at most weekly), it becomes almost impossible to link the presence of these insects to any kind of event or work practice that might have occurred and resulted in their release. If traps are present, operating procedures should be clear as to how often they are checked, who is responsible for checking them, and what course of action would be taken if insects are identified.
In the case of an insectary where gene drive insects are present, an added complication to free flying insects is that their genotype is undetermined. As noted previously [Citation24], gene drive insects are likely to share marker genes with other transgenic strains present in the same facility. Thus, operating procedures should spell out when PCR-based diagnostics are used on free-flying insects to confirm or rule out the possibility that it contains a gene drive. One guiding principle may be that the closer the insect got to the containment barrier (corridor door), and hence the greater the potential for a containment breech, the more imperative it may be to know its genotype (Figure ).
Figure 2. Baseline level of escaped insects in an ACL-2 insectary. While the occasional free-flying insect will be discovered within the rearing space, such events should be progressively rarer across each barrier leading finally to the external corridor. *Note that in locations where the same or similar species occur locally, migration of wild insects from outside into the corridor, or from the corridor into the vestibule is possible. Operating procedures for such facilities must anticipate this possibility, and be sufficient to allow the unambiguous determination of changes in the frequency of insect releases.
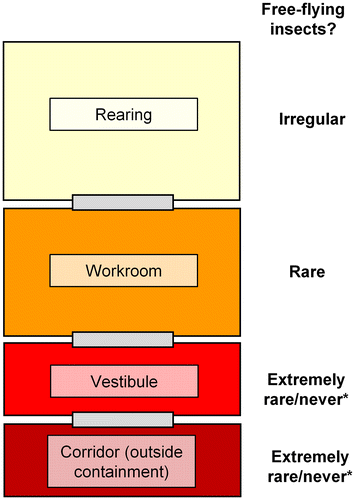
Box 5.
Critical questions to be addressed in an Escaped Arthropod Reporting SOP: The document outlined in the SOP should request information on escaped and accidentally released insects that provides insight into when and where these mishaps might have occurred, and should encourage reporting by staff members. What information should be reported in an Escaped Arthropod Reporting Log? Should a separate log be placed in every room of the insectary facility? Is the purpose of the log clearly stated? How often should the log be reviewed? How should the data from the Escaped Arthropod Reporting Log be analyzed and interpreted?
Assessing an Escaped Arthropod Reporting SOP: Are living or dead insects found in the insectary which have not been reported? Do staff members know the location of the Escaped Arthropod Reporting Log? Do they understand its purpose? Do entries in the log contain all the information requested? Are free-flying insects captured by the surveillance traps and fly paper strips being reported? Have any SOPs been revised as a result of information reported in the log?
4.5. Experimental manipulations
In addition to general procedures that may be common across ACL-2 containment facilities, individual facilities will likely need to document lab-specific experimental procedures. These procedures are expected to vary between facilities based on the organisms studied, research goals, and experimental methods. Some common procedures might include: (a) collecting, storing, or manipulating egg stages; sorting and collection of various larval or pupal stages; (b) adding or removing adult stages from or between cages; (c) setting up experimental crosses between different genotypes; (d) larval/pupal sorting; and (e) screening for phenotypic or fluorescent markers. All of these manipulations have some possibility of an accidental release into the containment area and the contamination of work surfaces or equipment with eggs. Therefore, all can benefit from having standardized, documented procedures.
Ideally, these types of SOPs should be brief, less than one page if possible. The use of images or photographs should substantially reduce the amount of text needed, and is therefore encouraged. For example, in our work with transgenic mosquitoes, we noticed that in densely populated cages adult mosquitoes often rest in or on pupal or oviposition containers in a manner that removing these containers sometimes resulted in their accidental release. Using a combination of covered containers and double-entrance cages, we developed a revised SOP that essentially eliminated the occurrences of accidental releases during this procedure (Figure ).
Figure 3. An SOP to prevent accidental release of adult mosquitoes during small cage manipulation. (A) In high density cages, adult mosquitoes often rest on the interior walls of pupal containers. When the containers are removed, these adults fly away. To prevent this, all pupal containers should be covered with a snap-on lid before removing the container. Adults resting on interior walls will be trapped and devitalized by freezing. (B) Removal of cups/containers using secondary cages. Secondary cages will contain a stocking entrance on two opposite sides, allowing researchers to transfer containers from a main cage directly to the secondary cage. Escapees from the main cage will now be trapped in the secondary cage. With fewer adults present in the secondary cage, the egg cup can be more easily removed while preventing releases. The escapees can then be killed by freezing, or aspirated and returned to the main cage.
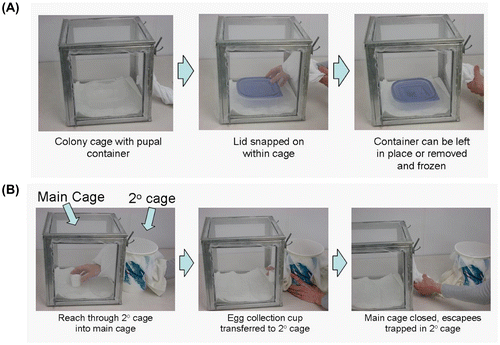
The decision concerning which procedures to document from a biosafety/containment perspective should probably begin with those manipulations that are common, repetitive, and have some chance of resulting in an accidental release. For example, a researcher may remove adult mosquitoes from a cage in order to screen for a marker, dissect midguts, set up genetic crosses, infect with pathogens, and so on. Although different activities will be performed after the insects are transferred to a holding dish, only a single SOP is required to describe the process by which they are removed from the cage and transported to the dish.
Box 6.
Critical questions to be addressed when developing SOPs for experimental manipulation: What experimental procedures will be performed which might result in an accidental release? Which part of each procedure is most likely to directly result in a release? Can the procedure be easily documented for training/reference materials? What equipment is needed? What should be prepared ahead of time? How long is the procedure likely to take? Is more than one staff person required? How can the clarity of SOPs be ensured?
Assessment of methods of SOP development: Upon adoption of a new containment-related SOP, is a decrease in the number of unexplained escapes or accidental releases (occurring during manipulation) observed in the Escaped Arthropod Reporting Log? When inquiries are made, do staff members state that fewer accidental releases occur? Do staff members state that SOPs are written clearly, and are easily understood?
5. Gene drive at scale
Eventually, small scale laboratory gene drive experiments are anticipated to lead to larger scale contained trials if the purpose underlying these experiments is to proceed to eventual field testing. Procedures and work practices developed for the handling and disposal of small hand-held containers of insects must be augmented by new procedures for manipulating vectors in walk-in cages or larger structures. Such large cages, combined with the potentially greater numbers of insects per experiment, present new challenges that must be met to avoid accidental release outside the containment barrier. As mentioned earlier, such large-scale cage studies can be expected to be performed in locations in which the arthropod thrives under the environmental conditions present, and therefore require finely orchestrated efforts in all facets of biosafety to ensure rigorous containment, the safest possible SOPs, detailed and effective training, and vigilant monitoring. While small cages are fairly easy to check for integrity, the inspection of large cages with hundreds of square feet of screening is anticipated to be much more challenging, and will likely require more regular inspections and validation. Scaling up the activities of a single gene drive lab may also impact other established protocols and procedures. An increased workforce means an increased training burden, as well as the potential addition of intermediates between the PI and those responsible for day to day oversight, such as a lab manager, building manager, and/or biosafety specialist.
In addition to the scaling up of a single lab, institutions may find that it is not the scale of any single lab that increases, but rather the number of labs on their campus that begin to conduct gene drive research in what will likely be a diverse array of organisms. An important point that must be considered by each institution is at what scale its gene drive research portfolio warrants combining all of its gene drive labs into a single facility in order to maximize security, efficiency, oversight, and maintenance activities, albeit at significant cost (Figure ). For example, many institutions have centralized the rearing/handling of vertebrate animals as well as high containment labs [Citation36], and these transitions may serve as a valuable precedent for gene drive research. For institutions at which multiple investigators are performing work simultaneously, centralized research facilities might represent the best case scenario for comprehensive, effective containment for gene drive in arthropods. Centralization would avoid potential conflicts of interest between oversight responsibilities and research objectives. In addition, institutions can integrate research, compliance, and facilities support to a level beyond that which can be achieved by individual PIs, and in doing so can provide a research venue for investigators who do not have access to a laboratory that can be made compliant for gene drive research. Likewise, for large-scale studies, the construction, maintenance and regulatory oversight of cages consisting of thousands of square feet of floor space and tens of thousands of square feet of screen walls and ceiling might best be undertaken by whole institutions rather than individual PIs or even teams of investigators. Such an arrangement thus ensures that the efforts of scientists can be focused primarily on research and SOP development, rather than facilities management and regulatory oversight, the latter of which avoids significant potential for conflicts of interests.
Figure 4. Institutional challenges when gene drive research scales up. Management and oversight of gene drive research from an institutional perspective [green, light green, yellow, orange, red represent the progression of difficulty from easiest to hardest].
![Figure 4. Institutional challenges when gene drive research scales up. Management and oversight of gene drive research from an institutional perspective [green, light green, yellow, orange, red represent the progression of difficulty from easiest to hardest].](/cms/asset/71f2f6b7-8b48-4e83-ae73-334c91e817e3/ypgh_a_1424514_f0004_oc.gif)
Box 7.
Critical questions to be addressed in a large-cage manipulation SOP: How will screens/cages be checked for integrity prior to the initiation of experiments? What other objects (foliage, breeding sites, artificial terrain, etc.) will be present in the large cage? How will these objects be treated to devitalize all life stages of the insect? How will objects/insects be added/removed during the course of the experiment? How will the experiment be concluded? If necessary, how will the experiment be terminated prematurely? What treatments will be performed after the completion of experiments to prepare the cage for future use?
Assessing a large-cage manipulation SOP: The quality of screen/barrier integrity inspections, as well as methods to add/remove objects from large cages, will be proportional to the number of arthropod escapes documented. As with small cages, the target here should always be zero. Rapid termination procedures should be validated experimentally with unmodified control insects. The devitalization of cage and objects therein can be evaluated by alternating between two genetically discernible non-gene-drive populations, such that the first genotype should not be subsequently identifiable in the second population.
6. Keeping SOPs alive and well
Once an SOP manual is completed, it is imperative to keep it up to date. This may involve documenting new procedures, pruning outdated ones, and clarifying others. Many procedures may in fact remain unchanged. However, reaffirming these with an updated approval date reinforces a culture of biosafety for lab members, as it can be demoralizing to be asked to read a document that is out of date and bears little resemblance to how the work is actually being performed. Both during the initial generation of SOPs and during annual updates, PIs and laboratory managers should solicit feedback from research staff regarding the clarity of SOPs to ensure adherence and improve future development efforts. A short questionnaire administered following training sessions might be useful for recording such feedback, and the questionnaire can be generalized such that it can be used to elicit feedback about a variety of different SOPs.
7. Conclusions
The biosafety landscape for studies involving gene drive in arthropods is at a critical juncture. Numerous arthropods represent potential target species for gene-drive-based population suppression or replacement, due to the large number of arthropod species that transmit disease agents, damage crops, or act as deleterious invasive species. Recent reviews have discussed containment measures for arthropod research involving gene drive, but none have focused on the development of safe and effective SOPs for these types of experiments [Citation11,21,24,34]. The best guidance currently available, the ACGs, are performance-based. Thus, there may be many alternative pathways to meeting these standards. We have tried to articulate the types of details that should be considered by PIs, biosafety officers, and IBCs as they work together to establish containment conditions appropriate for the organisms under study and the types of gene drive being developed, while maintaining the performance-based approach of the ACG. Well defined, concise SOPs ensure compliance with confinement strategies by acting as a bridge that connects risk assessment efforts and containment measures with safety training and research-specific protocols. Therefore, in the ongoing debate regarding how gene drive research in arthropods should proceed, the importance of SOP development should not go overlooked. We believe that the information provided in this review will serve as a valuable reference for investigators and regulatory personnel not intimately familiar with the ACGs, or the challenges involved in maintaining an ACL-2 insectary.
Funding
This work was supported by the Defense Advanced Research Projects Agency under [grant number HR0011-16-2-0036], as well as by Texas A&M Agrilife Research.
Disclosure statement
ZNA recently served as an external consultant for Target Malaria, a group developing gene drive technology for malaria control.
References
- Champer J, Buchman A, Akbari OS. Cheating evolution: engineering gene drives to manipulate the fate of wild populations. Nat Rev Genet. 2016;17(3):146.10.1038/nrg.2015.34
- National Academies of Sciences, Engineering, and Medicine. Gene drives on the horizon: advancing science, navigating uncertainty, and aligning research with public values. Washington (DC): National Academies Press; 2016.
- Heitman E, Sawyer K, Collins JP. Gene drives on the horizon: issues for biosafety. Appl Biosaf. 2016;21(4):4.
- Webber BL, Raghu S, Edwards OR. Opinion: is CRISPR-based gene drive a biocontrol silver bullet or global conservation threat? Proc Nat Acad Sci. 2015;112(34):10565–10567.10.1073/pnas.1514258112
- Caplan AL, Parent B, Shen M, et al. No time to waste – the ethical challenges created by CRISPR. EMBO Rep. 2015;16(11):1421–1426.10.15252/embr.201541337
- Esvelt K. Precaution: open gene drive research. Science. 2017;355(6325):589.
- DiCarlo JE, Chavez A, Dietz SL, et al. Safeguarding CRISPR-Cas9 gene drives in yeast. Nat Biotechnol. 2015;33(12):1250–1255.10.1038/nbt.3412
- Gantz VM, Bier E. The mutagenic chain reaction: a method for converting heterozygous to homozygous mutations. Science. 2015;348(6233):442.
- Gantz VM, Jasinskiene N, Tatarenkova O, et al. Highly efficient Cas9-mediated gene drive for population modification of the malaria vector mosquito Anopheles stephensi. Proc Nat Acad Sci USA. 2015;112(49):E6736.10.1073/pnas.1521077112
- Hammond A, Galizi R, Kyrou K, et al. A CRISPR-Cas9 gene drive system targeting female reproduction in the malaria mosquito vector Anopheles gambiae. Nat Biotechnol. 2016;34(1):78.
- Esvelt KM, Smidler AL, Catteruccia F, et al. Concerning RNA-guided gene drives for the alteration of wild populations. eLife. 2014;3:e03401.
- Noble C, Min J, Olejarz J, et al. Daisy-chain gene drives for the alteration of local populations. bioRxiv. 2016:057307.
- Marshall JM, Akbari OS. Gene drive strategies for population replacement. In: Adelman ZN, editor. Genetic control of malaria and dengue. Elsevier; 2015. 9, p. 169–200.
- Curtis C. Possible use of translocations to fix desirable genes in insect pest populations. Nature. 1968;218(5139):368–369.10.1038/218368a0
- Davis S, Bax N, Grewe P. Engineered underdominance allows efficient and economical introgression of traits into pest populations. J Theor Biol. 2001;212(1):83–98.10.1006/jtbi.2001.2357
- Akbari OS, Matzen KD, Marshall JM, et al. A synthetic gene drive system for local, reversible modification and suppression of insect populations. Curr Biol. 2013;23(8):671–677.10.1016/j.cub.2013.02.059
- Chen C, Huang H, Ward C, et al. A synthetic maternal-effect selfish genetic element drives population replacement in Drosophila. Science. 2007;316(5824):597.
- Galizi R, Doyle LA, Menichelli M, et al. A synthetic sex ratio distortion system for the control of the human malaria mosquito. Nat Commun. 2014;5:3977.
- Galizi R, Hammond A, Kyrou K, et al. A CRISPR-Cas9 sex-ratio distortion system for genetic control. Sci Rep. 2016;6:31139.
- Adelman ZN, Tu Z. Control of mosquito-borne infectious diseases: sex and gene drive. Trends Parasitol. 2016;32(3):219.10.1016/j.pt.2015.12.003
- Akbari OS, Bellen HJ, Bier E, et al. Safeguarding gene drive experiments in the laboratory. Science. 2015;349(6251):927–929.
- Benedict M, Tabachnick W, Higgs S, et al. Arthropod containment guidelines-(Version 3.1)-A project of the American Committee of Medical Entomology-American Society of Tropical Medicine and Hygiene. Vector Borne Zoonotic Dis. 2003;3(2):75–90.
- Benedict M, D’Abbs P, Dobson S, et al. Guidance for contained field trials of vector mosquitoes engineered to contain a gene drive system: recommendations of a scientific working group. Vector Borne Zoonotic Dis. 2008;8(2):127.10.1089/vbz.2007.0273
- Benedict M, Bier E, Burt A, et al. Recommendations for laboratory containment and management of gene drive systems in arthropods. Vector Borne Zoonotic Dis. 2017; Oct 17. DOI: 10.1089/vbz.2017.2121
- Biosafety in Microbiological and Biomedical Laboratories. 5th ed. Washington (DC): Centers for Disease Control and Prevention, National Institutes of Health, US Department of Health Human Services; 2009.
- National Institutes of Health. NIH guidelines for research involving recombinant or synthetic nucleic acid molecules. Bethesda (MD): US Department of Health Human Services; 2013.
- Isaman V, Thelin R. Standard operating procedures (SOPs): reason for, types of, adequacy, approval, and deviations from and revisions to. Qual Assur. 1995;4(3):167.
- Baber N. International conference on harmonisation of technical requirements for registration of pharmaceuticals for human use (ICH). Br J Clin Pharmacol. 1994;37(5):401.10.1111/j.1365-2125.1994.tb05705.x
- Barton BL. International conference on harmonization-good clinical practices update. Drug Inf J. 1998;32(4):1143–1147.10.1177/009286159803200434
- Lillrank P, Liukko M. Standard, routine and non-routine processes in health care. Int J Health Care Qual Assur. 2004;17(1):39–46.10.1108/09526860410515927
- Bains S, Bhandari M, Hanson B. Standard operating procedures: the devil is in the details. J Long Term Eff Med Implants. 2009;19(3):195.
- Chemical Laboratory Safety and Security. A guide to Prudent chemical management. Washington (DC): National Research Council of the National Academies of Science; 2010.
- Oukem-Boyer OO, Munung NS, Tangwa GB. Small is beautiful: demystifying and simplifying standard operating procedures: a model from the ethics review and consultancy committee of the Cameroon Bioethics Initiative. BMC Med Ethics. 2016;17(1):27.
- Oye K, Esvelt K, Appleton E, et al. Biotechnology. Regulating gene drives. Science. 2014;345(6197):626.
- Barbe B, Verdonck K, Mukendi D, et al. The art of writing and implementing standard operating procedures (SOPs) for laboratories in low-resource settings: review of guidelines and best practices. PLoS Negl Trop Dis. 2016;10(11):e0005053.10.1371/journal.pntd.0005053
- National Research Council. Biosecurity challenges of the global expansion of high-containment biological laboratories: summary of a workshop. Washington (DC): National Academies Press; 2012.