ABSTRACT
A number of monosaccharides characteristic of plant gums were found in paint layers and preparation layers of samples of Maya mural paintings of 10 archaeological sites located in Campeche and Yucatan regions. This finding opens the question about the deliberate use of these organic polymers as additives for improving workability and mechanical properties in the preparation layer mortar and conferring cohesion to the pigments in the paint layer. The study performed by GC-MS has confirmed the presence, in significant amounts, of a series of monosaccharides, being glucose and mannose between the most abundantly found. Nevertheless, the low amount present in most of the samples hindered the quantification of the relative proportion of monosaccharides necessary for identifying the botanical species of the plant gum. According to the accepted methodology used by Maya artists for preparing painting materials, bark of trees containing plant gums was added to the slaked lime stored in pools and that should be consistent with the notable amounts of glucose, mannose and other monosaccharides forming the skeleton of hemicelluloses and cellulose found in most of the samples. Although organic matter can be present in paint samples exposed to the external environment in Mesoamerican region as result of the microbiological activity, marker compounds characteristic of products resulting from their metabolism were not found in the studied samples.
1. Introduction
1.1. Structure, properties and classification of vegetable gums
From an analytical perspective, vegetable gums are considered extractable matter taken from plants. They are solid vegetable secretions and are usually soluble in water and insoluble in alcohol and organic solvents which have since long ago been used as a binding and adhesive material (Mills and White Citation1987; Matteini and Moles Citation1991; Gettens and Stout Citation1966).
These polysaccharide-type substances are produced by certain plants to lessen the damage produced by external aggressions. Gums, contrary to cellulose, are constituted by macromolecules of a complex structure, both in their monomer variety and in the ramified structure adopted by their macromolecules.
The basic units that conform a polysaccharide are called monosaccharides. They are bound among them by a glycosidic bond. The molecules in the monosaccharide, depending on their number of carbon atoms, are called tetroses (C4) (examples: eritrose, triose), pentoses (C5) (examples: arabinose, ribose, ribulose, xylose, xylulose, lyxose) and hexoses (C6) (examples: alose, altrose, fructose, galactose, glucose, gulose, idose, mannose, sorbose, talose, tagatose). Structurally, they are polyhydroxy aldehydes or cyclic polyhydroxy ketones called hemiacetals and acetals. When these cycles contain six links, they are called pyranoses and when they contain five links they are called furanoses. Uronic acids are composed of a type of aldehyde-polyalcohol in which the primary hydroxyl has oxidized to carboxylic acid. When a high number of monosaccharides and/or uronic acids are combined through glycosydic bonds, the resulting polymeric compound is called polysaccharide ().
Figure 1. Structure of xylopyranose (left) and manofuranose (middle) and structure of uronic acid (right); Structure of disaccharide by linkage of both monosaccharides through glycosidic linkage (down).
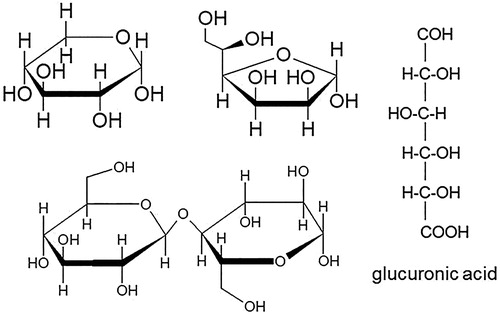
Vegetable gums, since long ago, have been used as adhesives and binding material. shows the main vegetable gums used in Europe and Asia and their monosaccharide and uronic acid compositions (Bonaduce et al. Citation2007). summarizes the vegetable gums proposed as possible additives used in mural paint in Mesoamerica (Magaloni Citation2001).
Table 1. Vegetable gums used in Europe and Asia and their monosaccharide and uronic acids composition.
Table 2. Vegetable gums used in Mesoamerica. Source for Mayan and scientific names on the table: Catálogo de la Flora de la Península de Yucatán.
1.2. Components of cellular wall and extractable material from tree wood and barks
and show the content intervals (% weight) of the three main components of conifer and hardwoods: cellulose, hemicelluloses and lignine. While cellulose and hemicelluloses are polysaccharide substances, lignine is a polyphenolic compound.
Table 3. Interval of contents (% weight) of the three main components of wood in hardwoods and conifers.
Table 4. Average content (% weight) of some botanical wood species.
Of all these components, the ones which are of special interest in the present study are the extractable materials. As recorded during colonial times and according to oral tradition, the procedure to prepare mural paint included the immersion of gluten-rich barks in water deposits where lime underwent a process of slaking (transformation into calcium hydroxide) (Vázquez de Ágredos Citation2006, Citation2010).
The extractable matter (with neutral solvents) and the dry residue obtained when a wood is analysed are formed by substances which do not contribute to the composition of cellular walls. In total, they constitute 4–10% of the total weight of wood in warm climates and can constitute up to 20% of it in tropical climates. These materials include fats, waxes, alcaloids, proteins, simple and complex phenolic compounds, simple sugars, pectins, mucilages, gums, terpenoid resins, terpenes, starches, glycosides, saponins and essential oils. Many of these substances work as intermediate compounds in the tree’s metabolism, reserve materials or are part of the tree’s chemical defence mechanisms against microorganisms. These substances are important to the colour, smell and deterioration of wood (Sjostrom Citation1993; Petterson Citation1984).
The dry residue is the amount of matter that results after the high-temperature combustion of wood. Usually, it is less than 1% in warm climate woods and slightly higher in tropical climate woods.
1.3. Methods of analysis for polysaccharides used in artwork
There are several papers reporting gas chromatography methods for the identification of vegetable gums used in artwork and archaeological objects as binders, adhesives or mortar additives. These can be found in the specialized bibliography. Two techniques have been applied: gas chromatography-mass spectrometry (GC-MS) and pyrolysis-gas chromatography-mass spectrometry (Py-GC-MS).
1.3.1. GC-MS
The analysis of vegetable gums and polysaccharides in general demands the breakage of glycosydic bonds and the release of the monosaccharide/uronic acid units and their derivatization to confer them enough volatility to go through the chromatographic column. Since the monomeric units integrating the gums are practically the same, their discrimination and exact identification is only possible after determining the quantitative contents of each of these components. For this reason, the complete analytical process includes some times includes principal component analysis that improves the discrimination between plan gum. shows the steps included in the most common work procedures: de-polymerization, derivatization and integration of peak areas for their quantitative treatment.
Hydrolysis is one of the most widely used methods of releasing monosaccharides integrating vegetable gums. It is carried out with mineral acids (Pancella, Bart, and Furlan Citation1989) and preferably with trifluoroacetic acid (TFA) (Kharbade and Joshi Citation1995; Vallance et al. Citation1998; Ormsby et al. Citation2005; Pitthard et al. Citation2006a; Pitthard, Griesser, and Stanek Citation2006b). Metanolysis has also been proposed as an alternative method to release monomeric units which integrate the vegetable gum (Bleton et al. Citation1996). This method has an advantage over hydrolysis: it significantly reduces the number of isomers and improves the performance of uronic acids.
Derivatization of the polysaccharide can be carried out in different ways. The most common ones are:
Trimetylsilation with hexamethyldisilazane (HMDS) and trimethylchlorosilane (TMCS) (Bersani et al. Citation2004; Marinach, Papillon, and Pepe Citation2004) o HMDS y TFA (Pitthard and Finch Citation2001; Bonaduce et al. Citation2007; Lluveras-Tenorio, Mazurek, Restivo, Colombini, and Bonaduce Citation2012). These two methods include a previous step of mercaptalation of aldoses with ethanethiol and TFA which, after derivatization, produces the diethyldithioacetalics and diethyldithioacetalic trimethylsilylated lactones. This procedure significantly reduces the number of isomers, which is one of the main problems when analysing polysaccharides through GC-MS. This procedure also avoids the formation of peracetals and improves the detection of uronic acids. However, it has the inconvenience of forming certain unwanted secondary products.
Formation of alditol acetates through anhydride acetic and methylimidazole (Pancella, Bart, and Furlan Citation1989).
Formation of acetate of methyl oximes through methoxyamine hydroxide which allows the formation of oximes, which are made react with acetic anhydride to form the methyl oxime (Pitthard et al. Citation2006a; Pitthard, Griesser, and Stanek Citation2006b; Lluveras-Tenorio, Mazurek, Restivo, Colombini, Bonaduce, and Neves Citation2012).
1.3.2. Py-GC-MS
One of the advantages of this method over GC-MS is the reduction of the previous manipulation of the sample, since the derivatization is performed “on line”. On the other hand, this technique improves the sensibility of the analysis, which in turn allows the identification of gums in samples in micrograms. The main disadvantage is the higher complexity of the obtained pyrograms, which include a high number of peaks associated with each monomer and their products of dehydration and isomerization.
Tetramethylamine hydroxide (TMAH) (Chiavari, Fabbri, and Prati Citation2001; Chiantore, Riedo, and Scalarone Citation2009) and HMDS have been suggested as derivatization reagents.
The Py-GC-MS techniques have been applied to the characterization of gums in watercolour (Chiantore, Riedo, and Scalarone Citation2009), and of gums used as paint binders (Scalarone, Chiantore, and Riedo Citation2008; Chiantore, Riedo, and Scalarone Citation2009; Riedo, Scalarone, and Chiantore Citation2013). In the context of Mesoamerican mural painting, this technique has been applied to identify indigoid and polysaccharide compounds in Mayan blue samples, Doménech-Carbó et al. (Citation2014).
1.4. Identification of vegetable gums in Maya mural painting
In general terms, vegetation in the Yucatan Peninsula is tropical. Most of the peninsula is covered by jungles such as the low deciduous forest, medium subdeciduous forests and medium semideciduous forests. Conversely, there are semidecidous and evergreen forests that take up a reduced area. More specifically, in the state of Yucatán, low deciduous forests, low thorny deciduous forests, and medium subdeciduous forests are typical. They are formed by unique vegetable communities. For its part, in the state of Campeche, vegetation is formed by medium subdecidous forest, semideciduous forest, high evergreen forest, low flooding forests, savannah and swamps. Finally, in the state of Quintana Roo middle semideciduous forests are swamps that are the most common. Moreover, it must be noted that in the three states there exists coastal dune vegetation and hydrophyte groups (Pennington and Sarakhán Citation2005).
Taking into account that the area of study for this paper is focused on the Northern Mayan Lowlands, the superposition of the forest distribution in the Yucatan Peninsula indicates that the characteristic forests with their corresponding vegetation in the area of study are (Pennington and Sarakhán Citation2005):
Medium subdeciduous forest: it is found in the central part, the east and north east of the Yucatan state and north if the Campeche state. It is commonplace in altitudes between 8 and 60 m.; typical plats for this type of vegetation are yaa-axnik (Vitex gaumeri), dzalam (Lysiloma bahamensis), kitin-ché (Caesalpinea gaumeri), among others.
Low deciduous forest: it lies in the north, northeast and central parts of the Yucatan state and northeast of the Campeche state. It is characterized by vegetation that is 6–15 m in height and most species are woody: chucum (Havardia albicans), chimay (Acaccia milleriara), catzín (Acaccia gaumeri), kitanché (Caesalpinea gaumeri), dzidzilche (Gymnospodium antigonoides), dzalam (Lysiloma bahamensis), chaka’ (Bursera simaruba), ha’bin (Piscidia piscipula) huaxim (Leucaena glauca), pixoy (Guazuma ulmifolia), subim (Acaccia cornígera), among others.
Medium evergreen forest: it extends homogeneously to the south of the Campeche pass, where it forms an undulated line and extends to Quintana Roo, almost following the Yucatán state border. The most common species in this forest are la Brosímum alicastrum, Manilkara zapota, Pimenta dioca, Aphananthe monoica, chaka’ (Bursera simaruba), Dendropanax arboreus, Sideroxylon capiri spp. Tempisque, Carpodiptera ameliae, palo de campana (Hernandia sonora), hoja blanca (Guarea glabra), Protium copal, among others, depending on the local conditions.
These vegetable compositions are and were of great economic importance for Mayas since they had many purposes, such as nutritional, medicinal, fodder, firewood, fertilizer, wood, colouring, biologic control, ritual, weather indicator, toxic, honey-producing, detergent, ornamental, handcrafting, coal, tools, alive closings, gums and resins, construction and colouring fixers, among others. Ethnobotanical studies aim to obtain information about the uses the Maya communities gave to plants around them (Flores and Vermont Citation1996; Flores Citation2002).
The first mention of the possible technique of manufacturing of stucco linings in Mayan architecture was made by Fray Diego de Landa in the sixteenth century. He stated that “the higher part [of the building] was made of sand, strongly whitewashed with some sort of water coming from tree bark” (Vázquez de Ágredos Citation2010). With this statement, the chronicler referred to the Pre-Hispanic practice of submerging gluten-rich tree barks in water deposits where calcium oxide was turned into calcium hydroxide Ca(OH)2 which can still be seen in some rural Mayan communities in the Yucatan Peninsula (Mexico). Nineteenth century architects and artist, especially painters, such as Viollet le Duc (Citation1838, 40–46) and Frederick Catherwood (Catherwood Citation1844, spp) respectively, did not say anything about this technique when they analysed and carefully observed these surfaces before drawing them. In the obtained results, there was no reference to the possible use of gluten-rich barks by Mayas, as mentioned by Fray Diego de Landa. Should rest of organic substances had appeared, they would have been recognized (Vázquez de Ágredos Citation2010).
It was in 1931 when archaeology offered the first information about organic additives that were used to prepare lime destined for architecture and its stuccoes. On those dates, the Carnegie Institution of Washington was conducting archaeological and restoration work on the site of Chichén Itzá (Yucatan). On those dates, Carnegie researchers were able to verify that local workers introduced barks rich in sap in deposits where lime was to hydrate. According to these local workers, this way of preparing lime dates back to Pre-Hispanic times had been perpetuated from generation to generation to obtain “better stucco”. The barks rich in organic additive that in 1931 the indigenous inhabitants added to water together with calcium oxide (CaO) to reinforce it came from chukum (Havardia albicans) (Morris Citation1931, 347–384). It was on the grounds of ethnography that the statement by Fray Diego de Landa made over 300 years ago, which described the use of binding saps such as the chukum during the hydration of lime, could be confirmed. Finally, Edwin Littman (Littman Citation1960) performed, during the 60s, a series of researches that led him to confirm Fray Diego de Landa’s thesis and the validity of the ethnographic study carried out by Ann Axtell Morris in 1931. At the same time, these analyses allowed to establish that Mayas used the binding saps present in trees from ha'bin (Piscidia piscipula), chak te’ (Caesalpinia mollis), chaka’ (Bursera simaruba) and chukum (Havardia albicans) (Vázquez de Ágredos Citation2010).
A few years later, David Hyman (Hyman Citation1970) distinguished through X-ray diffraction the use of these organic binding saps in the stucco linings found in the ancient site of Palenque (Chiapas, Mexico). Finally, these results were confirmed in 1978 in the research conducted by Elsa María Dubois (Vázquez de Ágredos Citation2010).
More recently, the thorough research by Magaloni (Citation1996, Citation1998, Citation2001) and others investigators (Sefcu et al. Citation2018; Rampazzi et al. Citation2016; Brecoulaki et al. Citation2012) and investigations by Doménech-Carbó et al. (Citation2014) and Straulino et al. (Citation2016) as well as the study performed by Guasch-Ferré et al. (Citation2013) and Guasch-Ferré (Citation2016) have confirmed these hypotheses.
2. Materials and methods
2.1. Reagents and reference materials
Glucose, Mannose, Galactose, Xylose, Fucose, Rhamnose, Arabinose, Glucuronic acid, Galacturonic acid, Inositol (Sigma-Aldrich) were used as reference standards for monosaccharides and uronic acids.
HuizacheFootnote1 gum (Acacia pennatula (Schltdl. & Cham.) Benth.), Mezquite gum (Prosopis glandulosa), Nopal (Opuntia vulgaris) of the Mercado de Sonora (Mexico, D.F) have been used as reference materials.
Trimethylsilylimidazole (TMSI), trimethylchlorosilane (TMCS), chloroform, acetyl chloride and methanol (Sigma) were used in the methanolysis-trimethylsilylation process.
2.2. Samples of Maya mural paintings
The stuccos considered in this paper belong to mural paintings dated back to in the Maya Classic period (ca. 300-950/1000 AD) in three different subregions of the Mayan Northern Lowlands in the Yucatan Peninsula (Mexico), as detailed below ():
Figure 3. Map of the region of the Maya Northern Lowlands with the geographic location of the archaeological sites. Acronyms: MU: Mulchic; DL: D’zula; KU: Kulubá; AC: Acanceh; CH: Chacmultún; EB: Ek’Balam; CI: Chichén Itzá; SR: Sta. Rosa Xtampak; ET: El Tabasqueño; DZ: Dzibilnocac.
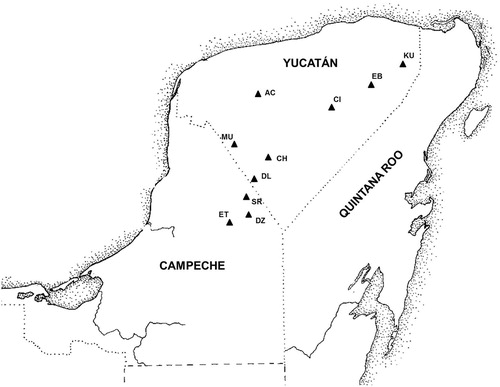
• Subregion of the Puuc (Yucatan state): mural painting of the Structure A from Mulchich Footnote2 (MU) (Late Classic; ca. 600–800 AD); mural painting of room 10 in Edificio de las Pinturas de Chacmultún (CH) (Late Classic; ca. 600–800 AD); mural painting of the Palace Structure in D’zula (DL) (Late Classic; ca. 600–800 AD).
• North-eastern subregion of Yucatan (Yucatan state): mural painting of the Palace Structure of Pinturas de Acanceh Footnote3 (AC) (Early Classic; ca. 300–600 AD); mural painting of room 64 in Ek’Balam Acropolys (EB) (between the end of 8th century and beginning of 9th century, but always under the reign of Ukit Kan Lek’Tok, who dies in 825 AD) (transition between Late Classic and Terminal Classic in the Northern Mayan Lowlands); polychromatic façade of the substructureFootnote4 of the Chichen Itzá Initial Series (CI) (Terminal Classic; 878 AD); polychromatic façade of the palace structure of the B group in Kulubá Paintings (KU) (Classic Terminal; ca. 800-950/1000 AD).
• Chenes Subregion (Campeche state): mural painting of the Structure I of El Tabasqueño (ET) (Late Classic; ca. 600–800 AD); mural painting of the Structure I of Dzibilnocac (DZ) (Late Classic; ca. 600–800 AD); polychromaticFootnote5 façade of the Palace Boca de Serpiente de Santa Rosa Xtampak (SR) (transition between Late Classical and Terminal Classic; ca. 800-950/1000 AD).
2.3. Instrumentation
2.3.1. Gas Chromatography/Mass Spectrometry (GC/MS)
An Agilent 5973N mass spectrometer coupled to an Agilent 6890N gas chromatograph (Agilent Instruments, USA) was used for identifying the organic materials present in some of the samples. Agilent Chemstation software (MSD) was used for the integration of peaks and for the mass spectra evaluation. GC separation was achieved in a chemically bonded fused-silica capillary column HP-5-MS (Agilent, USA), (stationary phase 5% phenyl 95% methylpolysiloxane, 30 m × 0.25 mm i.d., 0.25 mm film thickness). The chromatographic conditions were: initial temperature, 100°C for 2 min. The temperature has been programmed with a gradient of 6°C min−1 up to 300°C held for 5 min. Helium has been used as the carrier gas with a flow division ratio of 1:20. The electronic pressure control was set in the constant flow mode with vacuum compensation. The ions were generated by ionization of electrons (70 eV) in the ionization chamber. The mass spectrometer was scanned from m/z 20–800, with a cycle time of 1 s.
2.4. Pretreatment of the samples
The new method proposed for the analysis of vegetable gums and other matter extractable from wood and tree bark is based on the method suggested by Bleton et al. (Citation1996) for the analysis of polysaccharides. Such method combines an initial treatment with methanolysis, which is performed with acetyl chloride and methanol with the second process of derivatization (trymethilation) (). For this reason, a combination of trimethylsilylimidazole (TMSI) and dimethylchlorosilane (TMCS) has been chosen instead of HMDS suggested by Bleton et al. (Citation1996) given the higher efficiency as a derivatisation reagent for hydroxyl groups (Knapp Citation1979).
Figure 4. Scheme of the preparation procedure of samples: (1). Methanolysis. Rupture of the glycosidic bond and incorporation of the methyl group; (2). Trimethylsilication of –OH groups.
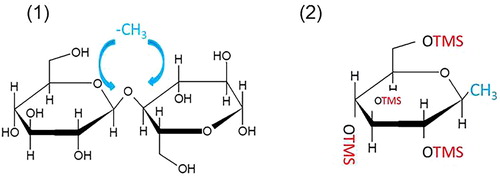
Methanolysis allows the release of monosaccharides and uronic acids which integrate the macromolecule of the gum by the rupture of glycosidic bonds and the formation of the corresponding methylated glycosides. It is worth noting the different processes depending on the monomeric unit which integrates the gum macromolecule (Mejanelle et al. Citation2002):
Reducing monosaccharides react with methanol in an acid catalysis process through their anomeric hydroxyl groups to produce metylglicosides. As a result of this process only four possible isomers are obtained for each monosaccharide, which differ in the ring size (furanoside or pyraoside) and in the stereochemistry of the anomeric carbon atom (α or β anomer). For instance for glucose, α-glucofuranose (α-glu-f), α-glucopyranose (α-glu-p), β-glucofuranose (β-glu-f), β-glucopyranose (β-glu-p). Quantitation is made by measuring the peak area of the more intense peak (isomer) corresponding to each monosaccharide. Normalized value is obtained as the ratio of its peak area value and the summation of peak areas of the more intense isomer for all the monosaccharides identified in the sample.
The, 1,6-anhydro derivatives are formed through an intramolecular reaction which implies the elimination of water between C6 and the anomeric hydroxyl of the β-aldohexopyranoses in the formation of chair 1C.
The methanolysis of uronic acids implies two simultaneous reactions: the acetylation of the anomeric hydroxyl which produces the methylglyoxal and the esterification of the carboxyl group.
shows the treatment applied to the samples.
Table 5. Steps of the pretreatment of samples.
3. Results and discussion
3.1. Characterization of reference materials
With the previously described method, three vegetable gums have been extracted: on the one hand, from two autochthonous plants from Mexico in the Mesoamerican part of the Mayan culture (huizache and mezquite gums); on the other, from a plant native to cultures such as the teotihuacana, tolteca and zapoteca (nopal), which have been previously suggested in the specialized bibliography as possible additives in the making of the preparation layers and paint layers for mural decoration, as previously described. – show the obtained chromatrograms.
Figure 5. Nopal chromatogram: p (pyranose) and f (furanose) indicate the different cyclic isomers; a (alpha) and b (beta) indicate the different anomers. Acronyms. Ara: Arabinose, Rha: Rhamnose, Fuc: Fucose, Xyl: Xylose, Man: Mannose, Gal: Galactose, Glu: Glucose, GaIU: Galacturonic Acid.
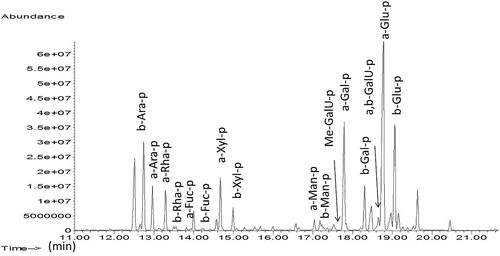
summarizes the chemical composition of autochthonous vegetable gums considered for their possible use as additives in Maya mural paintings.
Table 6. Composition of autochthonous vegetable gums considered possible additives of Maya mural paintings.
It is observed that all gums possess significant amounts of galactose and arabinose except for jonote, which is particularly rich in rhamnose. Furthermore, nopal has high glucose content. Only nopal and plum tree contain xylose. The gums jonote, jobo, cedar and nopal contain some fucose. These differences can facilitate the identification of a gum within the series of analysed Maya mural paintings.
shows the contents of arabinose and galactose expressed as a percentage of area for the chromatographic peak of these monosaccharides for four of the gums and vegetable extracts selected as reference materials for this research. These two monosaccharides have been chosen as a reference compound since they are major components in most vegetable gums. The diagram shows a good discrimination between nopal, mezquite against both plum tree and huizache gums, which show a more similar composition in these monosaccharides.
3.2. Characterization of stuccos in Maya mural paintings
3.2.1. The paint layer binder
The values of relative percentage area of chromatographic peak experimentally obtained are shown in . and show the chromatograms obtained in samples of the paint layer extracted from stuccos in Sta. Rosa Xtampak and in Chichén Itzá sites as an example. The rest of chromatograms are presented in (rest of archaeological sites in the state of Campeche) and in (rest of the archaeological sites in the state of Yucatan).
Figure 11. Chromatograms of the rest of samples of paint layer from archaeological sites in the state of Campeche: Dzibilnocac (DZ) and El Tabasqueño (ET). Acronyms as in .
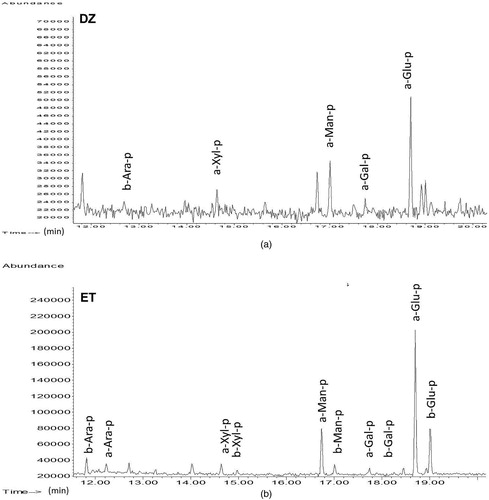
Figure 12. Chromatograms of the rest of samples of preparation layer from archaeological sites in the state of Yucatan: Mulchic (MU); Chacmutún (CH); D’zula (DL); Kulubá (KU) and Ek’Balam (EB). Acronyms as in .
Table 7. Relative percentage values of chromatographic peak area experimentally obtained in the paint layer from analysed samples.
It is worth mentioning the high contents of glucose and mannose exhibited by all samples, as well as the moderate amounts of xylose. Conversely, the contents of galactose and arabinose never surpass 12%. The high content of these monosaccharides which are present in lower proportion in the gums suggested as possible binders of mural paintings and the low contents of arabinose and galactose, which are major components in most gums, suggest that polysaccharides present in these paint layers may be complex mixes of gums and other extractable materials originating from tree bark. Other possible causes of this variation of expected results of monosaccharides in vegetable gums could be the metabolic action of living beings, particularly microorganisms, the addition of starch to the plaster or the highly alkaline environment of lime water where these paints were prepared.
The diagram shown in shows the relationship between the galactose content and the arabinose content found in the samples of Maya paint layers next to the reference vegetable gums. It is observed that the samples of Maya mural paintings are arranged (except in the case of Chacmultún) in low values for both monosaccharides, as previously stated. There seems to be no correlation associated with the region, which results in sample values being randomly distributed independently of their origin. The deviation in the case of Chacmultún is associated with the fact that the samples were taken after the restoration of these paintings. In Chacmultún is well documented that the restoration consisted of a consolidation treatment of the wall paintings with an acrylic emulsion, which is a microdispersion of an acrylic polymer in water stabilized with a number of additives such as surfactants, etc. This aqueous medium could dissolve partially the gums or polysaccharides present in the wall paintings or the oligomers formed as a result of the action of the natural alteration environmental agents thus modifying substantially the original composition of the mural paints in that concerning polysaccharides.
3.2.2. The additive in the preparation layer
shows the relative percentage values of chromatographic peak area experimentally obtained in the preparation layers in the analysed samples. and show the chromatograms obtained in the samples of preparation layers extracted from El Tabasqueño and D’zula sites, as an example. The rest of chromatograms are presented in and .
Figure 16. Chromatograms of the rest of the samples of preparation layer from archaeological sites in the state of Campeche: Sta. Rosa Xtampak (SR) and Dzibilnocac (DZ). Acronyms as in .
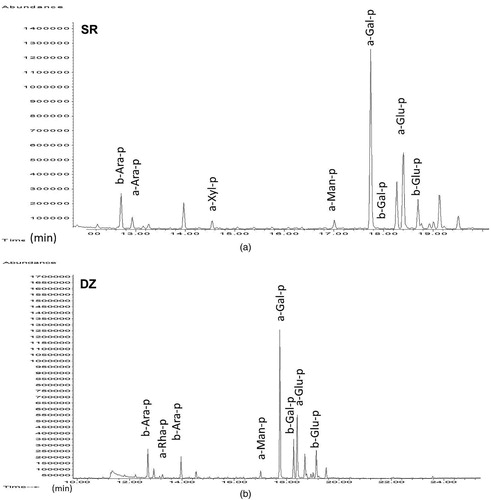
Figure 17. Chromatograms of the rest of the samples of preparation layer from archaeological sites in the state of Yucatán: Mulchic (MU); Chacmutún (CH); Kulubá (KU); Acanceh (AC); Ek’Balam (EB) and Chichén Itzá (CI). Acronyms as in .
Table 8. Percentages relative to the area of chromatographic peak experimentally obtained in the preparation layer from the analysed samples.
The obtained results are similar to those already shown for paint layers. Especially worthy of mention are the high contents in glucose and mannose. However, the galactose content, in this case, is higher than in the previous case, reaching values of 71.5% and 41.9% in Sta. Rosa Xtampak and Acanceh respectively. The content in arabinose has also increased slightly, in general, when compared to that obtained in paint layers in the same archaeological site, reaching maximum values of 22.2% and 19.0% in Chacmultún and Mulchic, both sites located in the state of Yucatan.
shows the existing relationship between the galactose and arabinose contents in the analysed samples of preparation layer samples. It seems the samples coming from the state of Yucatan show a higher content in arabinose, while those coming from the state of Campeche have a higher galactose proportion, even if both are quite far apart in the diagram. It is also observed that, in general, the amount of samples show values similar to those of nopal. However, the high mannose and glucose content exhibited by the preparation layer samples, which do not correspond to the typical vegetable gum composition, seem to indicate that the polysaccharide materials present in this stratum constitute, rather, a mix of gums and other extractable materials coming from tree bark.
3.2.3. Effects of alkaline medium in gum composition
A significant number of researches can be found in the reference material section describing the effect alkaline mediums produce in polysaccharides which can mainly produce:
Partial hydrolysis with elimination of monomeric units or “peeling”,
Processes of isomerization: (i.e.: glucose ↔ mannose).
With the aim to establish the influence of the highly alkaline medium (to which vegetable gums were added) in the final composition of the samples, an experiment with a selection of reference vegetable gums has been designed. In this experiment, the vegetable gums were dissolved to 1% in a solution of calcium hydroxide at pH=12 and they were exposed to this alkaline medium for 72 h. The resulting solution was analysed following the same analytical protocol as in real samples. The obtained results are showed in . and show the chromatograms obtained for gum mezquite and nopal.
Table 9. Variation in the composition of a vegetable gum once exposed to a strongly alkaline medium.
As observed in , gum mezquite shows high chromatographic signals while nopal () shows a drastic reduction of chromatographic signals having been exposed to the same strongly alkaline medium. This indicates a greater degree of degradation in this polymer under alkaline action. In gum mezquite, the most intense signal corresponds to arabinose, where a significant reduction of the content in the rest of monosaccharides present in this polysaccharide was observed. These results seem to suggest that the effect of highly alkaline mediums, such as lime water, is variable in vegetable gums depending on their composition (types of branching and monosaccharides components). Arabinose, therefore, seems to be the most alkaline resistant monosaccharide.
On the other hand, the results of this experiment suggest that in general, vegetable gums submitted to an alkaline medium should show higher arabinose contents and lower contents in galactose and other monosaccharides. However, the experimental results obtained both in the paint layer and the preparation layer of the samples extracted from Maya archaeological sites show the opposite ( and ), that is, lower percentage of chromatographic peak areas for arabinose in comparison with those of galactose.
4. Conclusions
The presence of monosaccharides components of vegetal gums allows confirming the use of vegetal extracts in both, the paint layer and preparation layer of the Maya mural painting. However, the restrictions in both sample amount and sensitivity of the analytical technique prevent the identification of the vegetal gum used in each case. The identification is further complicated if it is assumed that the stucco samples from mural paintings are complex mixtures of polysaccharide materials from bark and various parts of plants, which easily can be extracted in water.
The Maya’s preparation technique for binders and additives was based on the extraction in aqueous medium of polysaccharide materials from the barks of certain trees. The high content of glucose and mannose in the samples seems to confirm that, along with the vegetal gums, significant amounts of other materials soluble in water and in strongly alkaline medium were also extracted. Partial hydrolysis of non-extractable materials components of wood and vegetable tissues whose cellular walls are cellulose-rich (i.e. hemicelluloses, pectins, among others) could also took place. The partial extraction/hydrolysis of all these substances should be the evidence of the significant amounts of glucose, formed from the hydrolysis of cellulose, as well as mannose and xylose formed from the hydrolysis of hemicelluloses of glucuronoxylano and glucomannan type. These processes should be favoured by the strongly alkaline medium of limewater.
Acknowledgements
The analytical study carried out in this research has been funded through the Projects CTQ2014-53736-C3-1-P and CTQ2014-53736-C3-2-P, which are supported with Ministerio de Economía, Industria y Competitividad (MINECO) and Fondo Europeo de Desarrollo Regional (ERDF) funds, as well as project CTQ2017-85317-C2-1-P supported with funds from, MINECO, ERDF and Agencia Estatal de Investigación (AEI) are gratefully acknowledged. R+D Project BIA2014-53887-C2-2-P: Arte y Arquitectura maya. Nuevas tecnologías para su estudio y conservación, supported by Ministerio de Economía y Competitividad. The authors wish to thank Manuel Planes and Dr. José Luís Moya, responsible technicians in Servei de Microscopia Electrònica de la Universitat Politècnica de València for their collaboration. On the other hand, the authors wish to thank the Instituto Nacional de Antropología e Historia of Yucatan and Campeche to extend the official permits that made possible the transfer of the archaeological samples to Spain for their physical–chemical analysis.
Disclosure statement
No potential conflict of interest was reported by the author(s).
Notes on contributors
Núria Guasch-Ferré is D. Phil. in Science and Restoration of Historic-Artistic Heritage (Universitat Politècnica de València). Degree in Geology (Universitat Autònoma de Barcelona). In a research career spanning fourteen years: since 2004 researcher specializing in Conservation Science of Archaeological and Monumental Heritage, since 2010 researcher specializing in Archaeometric studies and from 2010 to 2012 she was a research technician in the Universitat Politècnica de València. Currently, she is a professor in Science of Conservation, Universitat de Barcelona. She has published some papers in her specialty and has made over 35 presentations of her research work at international seminars and conferences.
José Luis Prada Pérez is D.Phil. in Geological Sciences, since 1995 researcher specialized in conservation of archaeological and monumental heritage. Professor at the Superior School of Conservation and Restoration of Cultural Assets – Generalitat de Catalunya. He has published more than 50 specialized publications and participated as a consultant for the Unesco World Heritage Committee in Costa Rica. Pre-Columbian stone spheres.
Ma. Luisa Vázquez de Ágredos Pascual is D. Phil. in Geography and History (Universitat de València 2006) and D. Phil. in History of Art (Universitat Politècnica de València 2007). Her research focuses on archaeometric and cultural studies of Maya mural painting. Recently, in order to understand their compositions and cultural significances, she has concentrated on physical–chemical analysis of the colours, aromatics and drugs in Pre-Columbian Mesoamerica and other ancient cultures. She is author of numerous articles in specialized journals, such as Archaeometry, Journal of Archaeological Science, Journal of Cultural Heritage, Analytical Chemistry, book chapters and books in prestigious publishing, such as Universidad Nacional Autónoma de México, the British Archaeological Reports, Cambridge University Press, New Mexico University Press, among others.
Laura Osete-Cortina is D. Phil. in Chemistry. Since 2009 is technician in the Universitat Politècnica de València. In a research career spanning eighteen years, she has published over forty papers on chemical and physical methods of analysis of artworks and has made over fifty presentations of her research work at international seminars and conferences.
María Teresa Doménech-Carbó, born in Valencia (1958), B.Sc., D. Phil. in Chemistry (1989, University of Valencia), since 1999 professor in Science of Conservation, Universitat Politécnica de València, since 2005 until 2016 director of the IRP of the UPV. In a research career spanning 27 years, she has published over 180 papers on chemical and physical methods of analysis of artworks and has made over 120 presentations of her research work at international seminars and conferences. She has supervised 19 research students successfully for the degree of Ph.D. She has directed more than 10 regional, national and European research projects.
Additional information
Funding
Notes
1 The common name of the Huizache in Mexico varies depending on the region of origin Huizache, Guizache (Rep. Mex.); Güizache yóndiro (Mich., Gro.); Aromo, Aroma (Yuc., Tab., Chis.); Bihi (l. zapoteca, Oax.); Coo-ca, Cucá (L. guarigia, Son.); Espino, Espino blanco (Oax.); Fiñisache (Gto.); Flor de niño, Quisache (Chis.); Gabia, Gavia (Dgo.); Iai-do-no (l. cuicatleca, Oax.); Kánkilis-ché, Kántilis, X-kántilis, Xkantiris, Zubín, Zubínché (Yuc.); Minza (l. otomí, Hgo.); Tsurúmbini, Tsurímbini (l. tarasca, Mich.); Vinorama (Son., B.C., Sin.); Xcantiris (Mich.); Thujánum (l. huasteca, S.L.P.);Cucca (l. mayo, Son.). The botanical name. The botanical name of the Huizache is: Acacia Farnesiana (L.) Will.
2 Extraction of these mural paintings. Today found in Museo Regional de la Ciudad de Mérida.
3 Mural painting named Estucos de Breton (Vázquez de Ágredos Citation2006) that has disappeared. Nowadays it is stored inside boxes and the location of the original site is currently unknown and under search. Eduard Seler and Adela Breton documented it at the end of the 19th century.
4 Originally an exterior temple. The samples correspond to the stucco façade in this temple.
5 Stucco of the corner in the interior part of the ledge in the exterior façade of the building.
References
- Bersani, D., Pier Paolo Lottici, Gianni Antonioli, Elisa Campani, Antonella Casoli, and Carla Violante. 2004. “Pigments and Binders in the Wall Paintings of Santa Maria della Steccata in Parma (Italy): the Ultimate Technique of Parmigianino.” Journal of Raman Spectroscopy 35: 694–703. doi: 10.1002/jrs.1156
- Bleton, J., P. Mejanelle, J. Sansoulet, S. Goursaud, and A. Tchapla. 1996. “Characterization of Neutral Sugars and Uronic Acids After Methanolysis and Trimethylsilylation for Recognition of Plant Gums.” Journal of Chromatography A 720: 27–49. doi: 10.1016/0021-9673(95)00308-8
- Bonaduce, I., Hariclia Brecoulaki, Maria Perla Colombini, Anna Lluveras, Vincenzo Restivo, and Erika Ribechini. 2007. “Gas Chromatographic–Mass Spectrometric Characterisation of Plant Gums in Samples from Painted Works of Art.” Journal of Chromatography A 1175: 275–282. doi: 10.1016/j.chroma.2007.10.056
- Brecoulaki, H., A. Andreotti, I. Bonaduce, M. P. Colombini, and A. Lluveras. 2012. “Characterization of Organic Media in the Wall-Paintings of the “Palace of Nestor” at Pylos, Greece: Evidence for a Secco Painting Techniques in the Bronze Age.” Journal of Archaeological Science 39: 2866–2876. doi: 10.1016/j.jas.2012.04.018
- Catherwood, F. 1844. These Views of Ancient Monuments in Central America, Chiapas and Yucatan. New York: Donald A. Heald Rare Books (ABAA).
- Chiantore, O., C. Riedo, and D. Scalarone. 2009. “Gas Chromatography–Mass Spectrometric Analysis of Products from on-Line Pyrolysis/Silylation of Plant Gums Used as Binding Media.” International Journal of Mass Spectrometry 284: 35–41. doi: 10.1016/j.ijms.2008.07.031
- Chiavari, G., D. Fabbri, and S. Prati. 2001. “Analytical Pyrolysis of Carbohydrates in the Presence of Hexamethyldisilazane.” Analytica Chimica Acta 449: 271–280. doi: 10.1016/S0003-2670(01)01359-9
- Doménech-Carbó, M. T., L. Osete-Cortina, A. Doménech-Carbó, M. L. Vázquez de Agredos-Pascual, and C. Vidal-Lorenzo. 2014. “Identification of Indigoid Compounds Present in Archaeological Maya Blue by Pyrolysis-Silylation-gas Chromatography-Mass Spectrometry.” Journal of Analytical and Applied Pyrolysis 105: 352–362. doi: 10.1016/j.jaap.2013.11.025
- Flores, J. S. 2002. “Diferentes usos de las leguminosas en la Península de Yucatán.” Sociedades Rurales, Producción y Medio Ambiente 3 (1): 59–63.
- Flores, J. S., and R. Vermont. 1996. “The Secretions and Exudates of Plants Used in Mayan Tradicional Medicine.” Journal of Herbs, Spices & Medicinal Plants 4 (1): 53–59. doi: 10.1300/J044v04n01_07
- Gettens, R. J., and G. L. Stout. 1966. Painting Materials. A Short Encyclopedia. New York: Dover Pub.
- Guasch-Ferré, N. 2016. “Optimització de mètodes multitècnica per a la caracterització de components orgànics i morters de calç tradicionals de l'antiguitat.” Desenvolupament de metodologies experimentals per a la seva conservació i restauració. Aplicació a un cas d'estudi: els estucs de la pintura mural de la cultura maia (Antiga Mesoamèrica). Tesis Doctoral. València: Universitat Politècnica de València.
- Guasch-Ferré, N., M. L. Vázquez de Ágredos-Pascual, M. T. Doménech-Carbó, L. Osete-Cortina, and A. Doménech-Carbó. 2013. “Caracterización de materiales constitutivos en la pintura mural maya de la Península de Yucatán, México.” I Workshop Internacional de estudios arqueométricos en el arte prehispánico, València, October 3–5.
- Hyman, D. 1970. Pre-Colombian Cements. Tesis de maestría. Baltimore, MD: John Hopkins.
- Kharbade, B. V., and G. P. Joshi. 1995. “Thin-layer Chromatography and Hydrolysis Methods for the Identification of Plant Gums in art Objects.” Studies in Conservation 40: 93–102.
- Knapp, D. R. 1979. Handbook of Analytical Derivatization Reactions. 551. New York: J. Wiley and sons.
- Littman, E. R. 1960. “Ancient Mesoamerican Mortars, Plasters and Stuccos. The Use of Bark Extracts in Lime Plasters.” American Antiquity 25 (4): 593–597. doi: 10.2307/276642
- Lluveras-Tenorio, A., Joy Mazurek, Annalaura Restivo, Maria Perla Colombini, and Ilaria Bonaduce. 2012. “Analysis of Plant Gums and Saccharide Materials in Paint Samples: Comparison of GC-MS Analytical Procedures and Databases.” Chemistry Central Journal 6: 115. doi: 10.1186/1752-153X-6-115
- Lluveras-Tenorio, A., Joy Mazurek, Annalaura Restivo, Maria Perla Colombini, Ilaria Bonaduce, and Nuno M. Neves. 2012. “The Development of a New Analytical Model for the Identification of Saccharides Binders in Paint Samples.” Plos One 7: e49383. doi: 10.1371/journal.pone.0049383
- Magaloni, D. 1996. “ Materiales y Técnicas de la pintura mural maya.” Tesis de Maestría. Facultad de Filosofía y Letras, Universidad Nacional Autónoma de México, México.
- Magaloni, D. 1998. “El arte en el hacer: técnicas de pintura mural.” In Fragmentos del pasado: Murales Prehispánicos, edited by M. T. Uriarte Castañeda, 88–109. México DF: Instituto de Investigaciones Estéticas, Universidad Nacional Autónoma de México-Artes de México.
- Magaloni, D. 2001. “Materiales y técnicas de la pintura mural maya.” In La pintura Mural Prehispánica en México II. Área maya. Tomo III, edited by L. Staines, 155–198. México: UNAM-IIE.
- Marinach, C., M. C. Papillon, and C. Pepe. 2004. “Identification of Binding Media in Works of Art by Gas Chromatography–Mass Spectrometry.” Journal of Cultural Heritage 5: 231–240. doi: 10.1016/j.culher.2003.12.002
- Matteini, M., and A. Moles. 1991. La Quimica del Restauro. I materiali dell'arte pittorica. Florencia: Nardini.
- Mejanelle, P., J. Bleton, A. Tchapla, and S. Goursaud. 2002. “Gas Chromatography-Mass Spectrometry of Monosaccharides After Methanolysis and Trimethylsllylation. Potential for the Characterization of Substances of Vegetal Origin: Application to the Study of Museum Objects, Carbohydrate Analysis by Modern Chromatography and Electrophoresis.” Journal of Chromatofraphy Library 66: 845–902. doi: 10.1016/S0301-4770(02)80049-5
- Mills, J. S., and R. White. 1987. The Organic Chemistry of Museum Objects. London: Butterworths.
- Morris, A. A. 1931. “Murals From the Temple of the Warriors and Adjacent Structures.” In The Temple of the Warriors of Chichen Itza, edited by H. E. Morris, A. A. Morris, and J. Charlot, 347–384. Washington, DC: Carnegie Institution of Washington. Publication 406.
- Ormsby, B. A., Joyce H. Townsend, Brian W. Singer, and John R. Dean. 2005. “British Watercolour Cakes from the Eighteenth to the Early Twentieth Century.” Studies in Conservation 50: 45–66. doi: 10.1179/sic.2005.50.1.45
- Pancella, R., R. Bart, and V. Furlan. 1989. “Application de la chromatographie en phase gazeuse à l’identification des matières organiques dans les couches picturales.” In Méthodes de conservation des biens culturels, edited by F. Schweizer and V. Villiger, 39–44. Bern: Haupt.
- Pauk, V., Tomáš Pluháček, Vladimír Havlíček, and Karel Lemr. 2017. “Ultra-High Performance Supercritical Fluid Chromatography-Mass Spectrometry Procedure for Analysis of Monosaccharides from Plant gum Binders.” Analytical Chimica Acta 989: 112–120. doi: 10.1016/j.aca.2017.07.036
- Pennington, T. D., and J. Sarakhán. 2005. Árboles tropicales en México. Manual para identificación de las principales especies. México: Ediciones Científicas Universitarias de la Universidad Nacional Autónoma de México.
- Petterson, R. C. 1984. “The Chemical Composition of Wood (Chapter 2).” In The Chemistry of Solid Wood. Advances in Chemistry Series 207, edited by R. M. Rowell. Washington, DC: American Chemical Society.
- Pitthard, V., and P. Finch. 2001. “GC-MS Analysis of Monosaccharide Mixtures as Their Diethyldithioacetal Derivatives: Application to Plant Gums Used in Art Works.” Chromatographia 53: S317–S321. doi: 10.1007/BF02490349
- Pitthard, V., M. Griesser, and S. Stanek. 2006b. “Methodology and Application of GC-MS to Study Altered Organic Binding Media from Objects of the Kunsthistorisches Museum, Vienna.” Annali di Chimica 96: 561–573. doi: 10.1002/adic.200690058
- Pitthard, V., Martina Griesser, Sabine Stanek, and Tatjana Bayerova. 2006a. “Study of Complex Organic Binding Media Systems on Artworks Applying GC-MS Analysis: Selected Examples from the Kunsthistorisches Museum.” Vienna Macromolecular Sympossium 238, 37–45. Vienna: Vienna University of Technology.
- Rampazzi, L., M. P. Colombini, C. Conti, C. Corti, A. Lluveras-Tenorio, A. Sansonetti, and M. Zanaboni. 2016. “Technology of Medieval Mortars: an Investigation Into the Use of Organic Additives.” Archaeometry 58 (1): 115–130. doi: 10.1111/arcm.12155
- Riedo, C., Dominique Scalarone, and Oscar Chiantore. 2013. “Multivariate Analysis of Pyrolysis-GC/MS Data for Identification of Polysaccharide Binding Media.” Analytical Methods 5 (16): 4060–4067. doi: 10.1039/c3ay40474a
- Scalarone, D., O. Chiantore, and C. Riedo. 2008. “Gas Chromatographic/Mass Spectrometric Analysis of On-Line Pyrolysis–Silylation Products of Monosaccharides.” Journal of Analytical and Applied Pyrolysis 83: 157–164. doi: 10.1016/j.jaap.2008.07.006
- Sefcu, R., Václav Pitthard, Helena Dáňová, and Anna Třeštíková. 2018. “An Analytical Investigation of a Unique Medieval Wood Sculpture and its Monochrome Surface Layer.” Wood Science and Technology 52: 541–554. doi: 10.1007/s00226-017-0965-y
- Sjostrom, E. 1993. Wood Chemistry. Fundamentals and Applications. 2nd ed., p. 292. San Diego, CA: Academic Press.
- Straulino Mainou, L., S. Sedov, A. M. Soler Arrechalde, T. Pi Puig, G. Villa, S. Balanzario Granados, M. T. Doménech-Carbó, L. Osete-Cortina, and D. Leonard. 2016. “Maya Lime Mortars-Relationship Between Archaeomagnetic Dating, Manufacturing Technique, and Architectural Function - The Dzibanché Case.” Geosciences 6 (49): 1–27.
- Vallance, S. L., B. W. Singer, S. M. Hitchen, and J. H. Townsend. 1998. “The Development and Initial Application of a Gas Chromatographic Method for the Characterization of Gum Media.” Journal of the American Institute for Conservation 37: 294–311. doi: 10.1179/019713698806082750
- Vázquez de Ágredos, M. L. 2006. “Recursos materiales y técnicas pictóricas en los murales de las tierras bajas mayas.” Tesis Doctoral inédita (Dir. Cristina Vidal). València: Universitat de València.
- Vázquez de Ágredos, M. L. 2010. La pintura mural maya. Materiales y técnicas. México: Universidad Nacional Autónoma de México.
- Viollet le Duc, E. 1838. Le voyage d’Italie 1836-1837. Adresseées à sa famille. Paris: L. Laget.