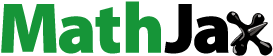
Abstract
The production of polymeric fibers by the electrospinning technique stands out as being simple, accessible, versatile and with potential use of polymer microfibers in many areas of science and technology. In this article, polymeric microfibers of PBAT/PLA and carbon nanotubes were obtained using a Fractional Factorial Design. The statistical results, the optical microscopy and scanning electron microscopy (SEM) showed that the addition of MWCNT’s had an influence in the diameter of the fibers (1.16 ± 0.22 µm), so that its incorporation decreased the value of the diameter, also they were more uniform and homogeneous. Besides, the MWCNT’s provided the fibers a better mechanical resistance, probably being inside the fibers, as well as showed in the analysis of wettability by contact angle.
Graphical Abstract

1. Introduction
The electrospinning process is a simple and versatile technique used in order to produce thin fibers at micro- and nanometric scales using an electrostatic field [Citation1, Citation2]. The pioneering studies on the use of electrostatic forces applied for producing polymeric fibers include patents in the 1930s and 1940s [Citation3–8]. However, only in the last two decades the electrospinning experimented advances in different fields of the science mainly in materials field, due to the excellent characteristics of the micro- and nanomaterials, that, combined with its simplicity and low cost allowed widely application [Citation9, Citation10].
The experimental apparatus to perform electrospinning is based on a high-voltage power supply, a polymeric solution (polymer and solvent) and a metallic collector [Citation9, Citation11–14]. The polymer solution is placed into a syringe with a metal capillary tube (needle) from which the fluid is ejected. In spite the variety of possible arranges, the more frequent is that in which the metallic collector is positioned in front of the needle in horizontal way (high density of randomly oriented fibers) [Citation15]. One of two high-voltage electrodes is connected to the needle tip and the other to the collector that is grounded. Then, a potential difference is established between the needle and collector [Citation14, Citation16].
A syringe pump is used for pushing the polymer solution out of the needle tip in order to form a drop that is, as earlier as it borns, exposed to the electric field and, consequently, subjected to charge-induction. The drop gradually deforms if the electric field is slowly increased and acquires conical aspect (Taylor’s cone). If the intensity of the electric field is further increased, at critical (or threshold) value of electric field the repulsive charge-forces existing in the deformed drop exceed the solution’s surface tension and a charged jet is quickly ejected in direction to the grounded metallic collector. Before the jet to arrive the collector, the polymer molecules are straightened and the solvent is concomitantly vaporized, forming the pre-dried fibers deposited on the collector [Citation12, Citation13].
The characteristics of electrospun fibers are dependent on many parameters related to: i) polymer (example: molecular weight and its distribution); ii) solution (example: concentration, viscosity), iii) process (example: potential difference, flow, distance); and iv) external parameters (example: temperature, humidity) [Citation9].
Despite the wide variety of parameters, it is possible to control the morphology of the fibers, such as the diameter distribution and average value of the fibers, optimizing the key factors that most interfere in the manufacture of fibers through the electrospinning process [Citation9]. Electrospun fibers have unique properties, allowing them to be used in many applications, such as separation membranes, catalysis, controlled drug release, energy storage and storage and sensing. In addition, electrospinning allows the manufacture of composite fibers [Citation12, Citation17–23]. Some studies show that the carbon nanotubes incorporated in the polymeric fibers optimize their electrical properties, resulting in the case of analytical sensors, in an increase in the electron transfer rate between the active sites of the analyte and the sensor electrode, which amplifies the analytical response [Citation24].
As there are many tasks that can be studied in electrospinning (parameters related to the polymer, the solution, the process and external or environmental conditions), the application of design of experiment (DOE) can be an alternative for reducing the time of study, costs of the analysis and also increase productivity and quality the study for optimizing the experimental conditions [Citation25]. This tool allows the adjustment of data by empirical functions (linear or quadratic) with terms of interaction that provide information about the system (trends) [Citation26]. Recent studies on electrospinning achieved success by use this statistical tool for evaluating the process parameters and those of the polymeric solution [Citation27–32].
Fractional factorial designs selectively reduce the size of an experiment because they use known properties of the project. While it is not possible to conduct a comprehensive investigation of all possible combinations of factor levels, critical process information is not lost. This resource proves to be advantageous from an economic point of view, since research requires quick answers, together with careful solutions on reducing the magnitude of the proposed experiments, and which guarantee greater generality of conclusions obtained from the results of the experiments [Citation33].
The present study aims to obtain polymeric fibers based on PBAT/PLA with chemically modified multilayer carbon nanotubes (f-MWCNTs) through Fractional Factorial Design (FFD, that is an important tool of DOE) approach; and to evaluate the possible modifications in the fibers/f-MWCNTs properties, such as morphology and mechanical, caused by the presence of f-MWCNTs on electrospun fibers.
Other works are reported in the literature using polymers with carbon nanotubes in electrically spun fibers for the most diverse applications. However, due to the search carried out in the databases, no studies were found using the polymeric blend PBAT/PLA (biodegradable). Therefore, this article can help other research groups at crucial stages of their work, such as choosing composite fibers for their applications, in addition to understanding how carbon nanotubes act in this polymeric matrix and how the quantities of inserted nanotubes interfere in the electrospinning process and the quality of the fibers obtained. As a result, there is a significant saving of time and resources.
2. Materials and methods
2.1. Materials
The polymer used on the experiment consists of a blend of poly (buthylene adipate-co-terephthalate) (PBAT) and poly (lactic acid) (PLA), commercialized by Basf (Ludwigshafen at Rhein, Germany) as Ecovio® (F Blend C2224) trade name. Beyond this biodegradable blend (PBAT/PLA), multilayer carbon nanotubes (MWCNTs > 90% carbon basis, D × L 110–170 nm × 5–9 μm), acquired from Sigma-Aldrich (Germany), Chloroform (CHCl3) and N,N-dimethylformamide (DMF), both acquired from FMaia (Brazil), were used for preparing the polymeric solution. Nitric acid (HNO3) and sulfuric acid (H2SO4) were acquired respectively from Anidrol (Brazil) and Fmaia (Brazil).
2.2. Methods
In order to functionalize the MWCNT, a treatment was performed by mixing 1.0 g of the carbon nanotubes with 500 mL of a HNO3/H2SO4 (1:3) solution and further stirring during 12 h [Citation34, Citation35]. Then the f-MWCNTs were filtered and washed using distilled water until neutral pH. After, the chemically modified material was dried in a low pressured-oven, at 120 °C.
This process is necessary, since in composite applications there is a need to disperse the MWCNTs homogeneously throughout the polymeric matrix. The acid treatment was used in order to minimize the aggregation of the particles and improve the dispersion behavior, since the MWCNTs have a hydrophobic surface that is prone to aggregation and precipitation [Citation36, Citation37].
The polymeric solutions used in electrospinning were prepared by solubilizing requested amount of PBAT/PLA in CHCl3:DMF, 85:15% (v/v) ratio, and magnetic stirring during two hours. Suspensions were prepared by adding requested amount of f-MWCNTs to polymeric solution. After, the suspension was exposed to an ultrasonic bath for two hours. In order to perform the electrospinning process, the suspension was transferred to a 10 mL glass syringe, with a Hamilton needle (diameter 1.5 mm).
2.2.1. Doe APPROACH
The evaluation of factors that more influenced the electrospinning process was performed in order to obtain fibers at the micrometric scale. Therefore, experiments according to a fractional factorial design (FFD) were performed. In this way, five factors (distance needle-collector, flow rate, applied electric tension, concentrations of PBAT/PLA and f-MWCNTs) were analyzed. Each factor was fixed investigated in three levels (+1), (0) and (-1), being the central point condition [level (0)] performed in triplicate to verify the reproducibility of process. To avoid systematic errors, all the experiments were randomly performed. The evaluated response was the average diameter of the electrospun fibers. The values of used levels for each factor are shown in (as coded) and in (as coded and actual). The statistical analysis of the fractional factorial design were performed using the STATISTICA® 7.
Table 1. Factors and levels used in FFD.
Table 2. Matrix of the fractional factorial design with the coded and actual levels of the variables and the results of the average diameter of the polymeric fibers.
2.2.2. Analysis of the polymeric films
The morphology and the respective diameters of fibers obtained in each run were analyzed through optical microscopy (OM). For this, three optical microscope-slides were randomly attached in the front of metal collector before the electrospinning experiment. After the volume of 60 µL of polymeric solution (conc. given in ) has been electrospun the slides were collected and the mat removed and stored for further OM analysis. An optical microscope Olympus CX31A with magnification of 400x was used for observing the fibers. The software Analysis Get It® was used for obtaining the images. The diameters of the fibers were determined using the software Quantikov Image Analyzer 10.1. For each run, 20 fibers were randomly chosen from the images and the diameters were further measured. In addition, the morphologies of fibers (with and without f-MWCNTs) as well as their uniformity were evaluated using scanning electron microcopy (SEM). For that, the sample was fixed on a support using a carbon double-sided adhesive tape. The sample was then metalized with gold to the thickness of about 5 nm using a Denton Vaccum (Desk V) metallizer.
In order to confirm the presence of f-MWCNTs in the polymer fibers, thermal degradation kinetics (Thermogravimetric Analysis – TGA) of electrospun fibers were evaluated in a Perkin Elmer STA 6000 thermal analyzer with ceramic cell in the range of ca. 25 to 550 °C. For that, heating rate of 10 °C/min in a dynamic atmosphere of 10 mL min−1 nitrogen were used. Once achieved the temperature of 550 °C, this condition was maintained until the polymeric part of material has been complete degraded.
After kinetics of thermal degradation, the f-MWCNTs and the fibers with f-MWCNTs were calcined for 5 h at 550 °C in a EDG10P-S tubular furnace at 550 °C in a dynamic atmosphere of nitrogen with a flow rate of 0.5 L. min−1.The morphologies of the as-thermal treated materials were evaluated by SEM. For the SEM analysis pristine MWCNTs (NF), f-MWCNTs submitted to thermal treatment for 5 h at 550 °C (NQ) and electrospun fibers made with f-MWCNTs and further submitted to thermal treatment for 5 h at 550 °C (NE) were dispersed in ethanol and later deposited in specific supports until the materials were completely dried at room temperature. Before the SEM analysis, the materials were metallized with gold, as previously described.
In order to explore possible modifications of mats-surfaces as well as to evaluate the hydrophilicity/hydrophobic character of mats, their wettabilities were evaluated through measuring of contact angle. Drop water-contact angle (DWCA) were measured in the electrospun fibers (with and without f-MWCNTs) using the sessile drop method and a goniometer (CAM-micro by Tantec). The DWCA measurements were performed by dropping the volume of 10 µL of distilled water in the sample surface, at room temperature and 60% of relative humidity. For each sample, the average value of DWCA was calculated collecting the values of five measures.
The images of the drop water deposited at the surface of the polymeric mat were obtained with an USB microscope connected to a computer with specific software to acquire images. The sample was placed on a fixed support and the USB microscope on a mobile support adequately positioned to take pictures. Beyond the contact angle analysis on the polymer fibers, the hydrophilic character of f-MWCNTs, functionalized in an acid solution of H2SO4 and HNO3 (3:1 v:v), was evaluated through measuring the contact angle of f-MWCNT pastilles and comparing to pastilles of raw MWCNTs [Citation38, Citation39]. For that, samples of approximately 100 mg and applied a pressure of approximately 6.865 MPa for 5 min for obtaining pastilles.
Mechanical properties of mats were evaluated through stress-strain curves standardized by ASTM D882-12 for thin films. The analyzes were performed in triplicates for the mat-samples (with and without f-MWCNTs) using a texturometer (HD Plus by Stable Micro Systems) equipped with a 5 kg load cell. The ratio of axial traction used on the samples was 1 mm·s−1 up to their rupture. The staples were arranged 100 mm apart. The analysis of the stress-strain curve allowed obtaining the Young’s modulus, yield point and stress at fracture point. Although the mechanical analyzes are carried out in triplicate, the results presented correspond to a single representative curve of each polymeric film.
3. Results and discussion
Due to the many factors that may do influences on electrospinning process, a fractional factorial design () was used for systematically evaluating the effects of factors in electrospun fibers. The average diameters of the fibers were measured and used as the response in the factorial design. contains the conditions used for all experiments being the factors given (as coded and actual values) as following: distance needle-collector, flow rate, applied electric potential, concentration of PBAT/PLA and concentration of f-MWCNTs. Also, the values of average diameter of the fibers obtained for each experiment, measured through analysis of SEM micrographs, are also showed. According to , the experiment 14 (average diameter = 1.29 ± 0.32 µm) and the experiment 12 (2.81 ± 0.44 µm) are those that, respectively, resulted on PBAT/PLA/f-MWCNTs fibers with smaller and larger diameter than the others.
The effects of factors and the interactions between the factors on response (average diameter of fibers) are shown in . This table also shows the significance of principal and interaction factor effects on fiber diameters and whether a given effect is statistically relevant or not. The analysis of indicated that the effect of f-MWCNTs concentration factor is significant (p-value < 0.05). It means that the presence of f-MWCNTs caused effective reduction on the diameter of the fibers relative to the neat polymeric fibers. The interaction effect between PBAT/PLA and f-MWCNTs concentrations showed a cooperative action between these factors. The second-order interaction effect between distance and f-MWCNTs concentration factors did not contribute to minimize the average diameter of the fibers, since its effect presented positive value. Nevertheless, the f-MWCNTs concentration was the most important factor due to the higher value of its effect. It is worth to mention the statistically significance of the second-order interaction effects associated to this factor as compared with the others.
Table 3. Values for main and second order effects, standard error and Student t-test for the diameter of the fibers obtained by electrospinning process.
The as-obtained response surfaces are shown the . Cooperative effects between f-MWCNTs concentration versus distance needle-collector factors or PBAT/PLA versus f-MWCNTs concentrations factors are clearly observed. According to the values of interactions effects showed in , the increase of the concentrations of f-MWCNTs and PBAT/PLA provokes reduction in the fibers’ average diameter, as showed in . Such fact is probably due to the increase in the electrical conductivity of the solution subjected to electrospinning, which enhanced the stretching of the fibers and produced homogeneous fibers with smaller average diameters [Citation9, Citation40]. The presence of f-MWCNTs in polymeric solution helps to a huge electrical-charge accumulation in the solution jet, resulting in a strong electrostatic repulsion, which produced fibers with smaller diameters and greater uniformity that match with data of literature [Citation10, Citation41–44]. The OM images (), demonstrated the way that changes in PBAT/PLA and MWCNTs concentrations factors interfered in the fibers’ homogeneity and continuity. The formation of beads, small balls along the fibers, has been observed in some electrospun fibers [Citation9]. The formation of beads depends on many factors such as the applied electrical tension, the viscoelasticity and the surface tension of solution, the charge density [Citation9], etc. Considerable number of beads and discontinuities were observed in the fibers whose the micrographs (runs 01, 08, 13, 14, see ). The micrographs of fibers made in the other runs showed continuous and homogeneous morphologies. In this case, the predominant factor is the viscosity of the solution subjected to electrospinning process, which is directly related to the concentration of the polymer [Citation9]. The higher concentration of both PBAT/PLA and f-MWCNTs hampered the jet elongation resulting in instability of the flow due to the high cohesively of the solution and, consequently, to the increase of surface tension, producing fibers with discontinuities and several beads [Citation45–47]. Decrease in PBAT/PLA concentration enhances the elongation of fiber-jet and continuous and homogeneous fibers are produced. So, suitable extent of entanglements of the polymer chains (due to increase of polymer concentration) contributed for homogeneous and continuous fibers, even with the elevated concentration of f-MWCNTs as also observed by Rodrigues et al. (2016) by increasing the viscosity of the polymeric solution [Citation9, Citation48] subjected to electrospinning.
Figure 1. Response surface for the average diameter of the polymeric fibers according to (a) distance (cm) versus concentration of f-MWCNTs (%); and (b) concentration of PBAT/PLA (%) versus concentration of f-MWCNTs (%).
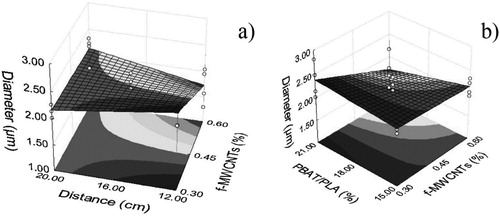
Figure 2. Optical microscopys of fibers with ↓ MWCNT’s and ↓ ecovio® (Exp. 07, 09, 10, 16); ↓ [MWCNT’s] and ↑ [ecovio®] (Exp. 02, 04, 11, 12); ↑ [MWCNT’S] and ↑ [ecovio®] (Exp. 01, 08, 13, 14); ↑ [MWCNT’S] and ↓ ecovio® (Exp. 03, 05, 09, 10).
![Figure 2. Optical microscopys of fibers with ↓ MWCNT’s and ↓ ecovio® (Exp. 07, 09, 10, 16); ↓ [MWCNT’s] and ↑ [ecovio®] (Exp. 02, 04, 11, 12); ↑ [MWCNT’S] and ↑ [ecovio®] (Exp. 01, 08, 13, 14); ↑ [MWCNT’S] and ↓ ecovio® (Exp. 03, 05, 09, 10).](/cms/asset/4ac815b9-8660-42d5-9d47-ef9436ac24ff/yadm_a_1779438_f0002_b.jpg)
In order to obtain fibers with high amount of f-MWCNTs and looking for more homogeneous fibers, the concentration of f-MWCNTs was fixed at 0.60% and the concentration of PBAT/PLA was reduced to 15% (w/v). It is worth to notice that the diameters of the fibers were not influenced by the process parameters, as shown in . Considering the efficiency of the microfibers production, central point values (level 0) for the factors applied electric tension (18 kV), distance needle-collector (16 cm) and the flow of 1.80 mL h−1 were used.
shows the SEM images in which the morphologies of the fibers (with and without f-MWCNTs) being the other factors fixed at central point. Without f-MWCNTs the average diameter of fibers fall on the range of 3.13 1.27 µm beyond significant roughness and heterogeneity were observed. Whether f-MWCNTs nanoparticles are present on polymeric suspension subjected to electrospinning, the average diameter of the fibers decreased to 1.16
0.22 µm, a reduction of approximately 62%. In addition, the fibers showed less roughness as compared to fibers without f-MWCNTs, indicating a possible interaction between the polymer PBAT/PLA and the f-MWCNTs. The higher heterogeneity in fibers without f-MWCNTs can be visualized by SEM images and also be evaluated comparing the standard errors in average diameters of the samples without (1.27 µm) and with f-MWCNTs (0.22 µm).
Figure 3. Images by SEM for the PBAT/PLA fibers without (a) and with f-MWCNTs (b). Conditions: needle-to-collector distance of 16 cm, flow rate of 1.80 mL·h−1 and electrical potential applied of 18 kV. Magnification: 5,000×.
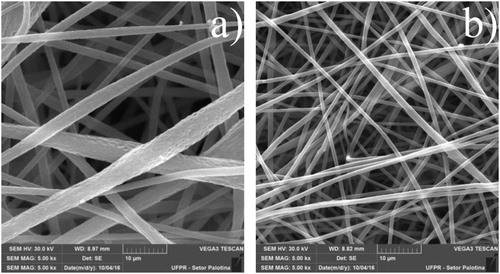
Other works obtained similar diameters when using the PBAT/PLA polymer blend, however they added other additives to their materials and also other solvents were used during the solubilization process [Citation37, Citation49].
Both treatments demonstrate that the fibers are randomly arranged, this is due to the type of collector used (static) and the instability of the electrospinning jet, which is composed of two movement regimes emitted by the Taylor cone. The first consists of a stable jet in a straight line for a short distance (sometimes insignificant) followed by the whipping movement of the fibers [Citation15, Citation50].
The study of degradation kinetics of fibers with f-MWCNTs through TGA curves evidenced that this nano material is located inside of polymeric fibers. The mass of 0.1583 mg (polymeric yarns + f-MWCNTs) was submitted to degradation process at constant temperature of 550 °C. After this thermal treatment, the mass of the obtained residue was 0.69% (0.0011 mg) that is very similar to the expected mass of f-MWCNTs (0.0010 mg) considering the concentration of nanoparticles (0.60%) inserted into the suspension submitted to electrospinning technique.
Analysis of SEM images () of pristine MWCNTs (NF), f-MWCNTs submitted to thermal treatment for 5 h at 550 °C (NQ) and electrospun fibers made with f-MWCNTs and further submitted to thermal treatment for 5 h at 550 °C (NE). It can be observed that thermal residues of neat f-MWCNTs (NQ) and f-MWCNTs from electrospun fibers (NE) possess longer and finer morphologies, similar to f-MWCNTs not submitted to thermal treatment, being visible presence of layers or lamellae [Citation51]. These results confirm that the nanoparticles were effectively inserted in the electrospun fibers.
Figure 4. Images by SEM for the f-MWCNTs (a) functionalized f-MWCNTs (NF), (b) functionalized f-MWCNTs with subsequent thermal treatment for 5 h at 550 °C (NQ) and (c) functionalized f-MWCNTs, electrospun and with subsequent thermal treatment for 5 h at 550 °C (NE).
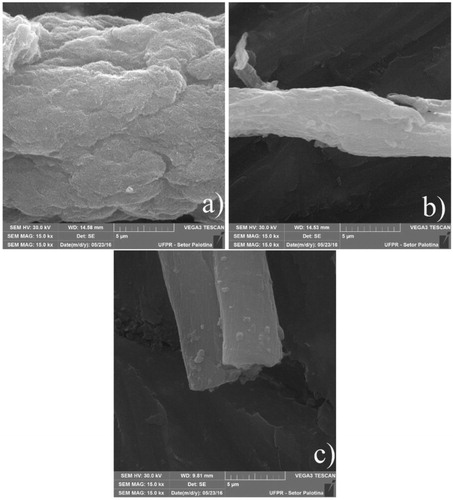
Once the presence of f-MWCNTs in the electrospun PBAT/PLA fibers was confirmed, measurements were performed for evaluating the mechanical (by tensile-stress) and wettability (by DWCA) properties of the materials. The hypothesis that the excellent mechanical properties often observed on carbon nanotubes-based composites is attributed to such nanoparticles [Citation52] may be also observed on PBAT/PLA/f-MWCNTs composite obtained by electrospinning.
According to the stress-strain curves shown in , the presence of f-MWCNTs to the polymeric fibers of PBAT/PLA enhanced the mechanical properties of the material. The values of Young modulus (E), yield point and stress to fracture (σ) are shown in . Significant decrease (180%) in the Young modulus was observed on electrospun fiber due to the presence of f-MWCNTs. This demonstrates be true the hypothesis that PBAT/PLA/f-MWCNTs composite electrospun fibers have low stiffness and higher elasticity. Although decrease in the material rigidity, as observed in others studies [Citation53–55], the reduction of Young modulus on such composites suggested the occurrence of interactions among f-MWCNTs particles and the polymer chains. Such possible interactions allowed alignment of this composite-material caused by orientation of nanoparticles in the fibers surface [Citation56]. Analogous effect was not observed when there is no alignment of f-MWCNTs in the fibers [Citation57, Citation58]. In fact, in such studies the values of Young modulus increased. The authors observed presence of defects and concluded that the presence of defects lead the fibers become fragile [Citation57, Citation58].
Table 4. Elasticity modulus, yield point and stress to fracture of the electrospun PBAT/PLA fibers with and without f-MWCNTs.
Other parameters associated to mechanical properties, such as yield strength and tensile strength increased 163% and 107%, respectively, due to the presence of f-MWCNTs on PBAT/PLA/f-MWCNTs composite fibers. This result showed better mechanical performance of the fibers containing f-MWCNTs. Ye et al. (2004) explained that MWCNTs hampered the tensile strength due to the strengthening effect, which is directly influenced by its distribution in the polymeric matrix and by the interfacial adhesion between the polymer and the nanoparticles.
In view the existence PBAT/PLA/f-MWCNTs interactions, as proposed by the previous discussion, the influence of the f-MWCNTs on polymeric fibers surface properties was evaluated by the analysis of wettability by drop water contact angle (DWCA) measures. The DWCA values for the polymeric fibers (with and without the f-MWCNTs) are shown in . The images of the drops of water deposited on the polymeric fibers (with and without the MWCNTs) are shown in . The results showed in indicated that the polymeric fibers (with and without the f-MWCNTs) have similar contact angle values and the hydrophobic characteristic remained due to the values remaining between 90° < θ < 180° [Citation59]. These results corroborated with the previous analysis and suggested possible interactions between the f-MWCNTs and the PBAT/PLA. So, the addition of f-MWCNTs (that possess hydrophilic character [Citation38, Citation39]) did not change the hydrophobic characteristic of the as-produced fibers. So, the nanomaterial was possibly inside the polymeric fibers. This fact could had occurred due to the acid functionalizing, which promoted the insertion of carboxylic groups and facilitates covalent bonds with countless polymers [Citation35, Citation60].
Figure 6. Drop water contact angle (DWCA) formed between drop of distilled water and the polymeric fiber surface without (a) and with (b) f-MWCNTs.
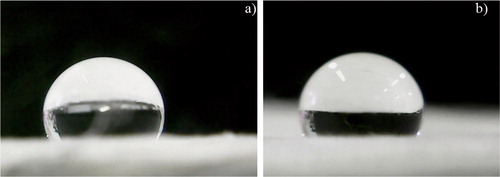
Table 5. Contact angle values for the polymeric films with and without the MWCNT’s.
The microfibers obtained in this study stands out since they can be used in many areas of science and technology, as sensors [Citation61], batteries [Citation62], hydrogen storage [Citation63], enzymes mobilization [Citation64], biomaterials [Citation65], controlled release of drugs [Citation66], catalysis [Citation64] and in solar cells [Citation67].
An expressive improvement in the mechanical properties of the PBAT/PLA fibers was observed as f-MWCNTs are present. This, per si, emphasizes the use of such composite fibers in many applications.
4. Conclusions
Through the application of fractional factorial design (FFD) methodology combined with the microscopy (OM and SEM) analysis of materials from electrospinning experiments, it was possible to obtain microfibers of PBAT/PLA with f-MWCNTs particles with interesting properties. The PBAT/PLA/f-MWCNTs composite fibers were homogeneous and without irregularities as compared to neat fibers (without f-MWCNTs). The presence of f-MWCNTs was the parameter that most influenced and interfered in the average diameter and morphology of fibers. Besides the nanoparticles are located mostly inside of the polymer fibers (due to the no change of drop water wettability of composite and compared to neat fibers). The presence of f-MWCNTs allowed the fiber be more mechanical resistant and potentializes their utilization in many technological applications. Therefore, this material can serve as a basis for future research in several areas, such as: sensors, batteries, hydrogen storage, mobilization of enzymes, biomaterials, controlled release of drugs, catalysis, and solar cells. Thus allowing information capable of contributing to the saving of time and resources in scientific research.
Disclosure statement
No potential conflict of interest was reported by the author(s).
Additional information
Funding
References
- Chang W-M, Wang C-C, Chen C-Y. The combination of electrospinning and forcespinning: Effects on a viscoelastic jet and a single nanofiber. Chem Eng J. 2014;244:540–551.
- Khalf A, Madihally SV. Recent advances in multiaxial electrospinning for drug delivery. Eur J Pharm Biopharm. 2017;112:1–17.
- Formhals Anton. Process and apparatus for preparing artificial threads. U.S. Patent n. 1,975,504, 2 out. 1934.
- Formhals Anton. Method of producing artificial fibers, 1937.
- Formhals Anton. Method of producing artificial fibers. U.S. Patent n. 2,158,415, 16 maio 1939.
- Formhals Anton. Production of artificial fibers from fiber forming liquids, 1940.
- Formhals Anton. Production of artificial fibers from fiber forming liquids. U.S. Patent n. 2,323,025, 29 jun. 1943.
- Formhals Anton. Method and apparatus for spinning. U.S. Patent n. 2,349,950, 30 maio 1944.
- Costa RGF, Oliveira JE, de Paula G. d, et al. Eletrofiação de polímeros em solução: parte II: aplicações e perspectivas. Polímeros. 2012;22(2):178–185.
- Sheikh FA, Macossay J, Cantu T, et al. Imaging, spectroscopy, mechanical, alignment and biocompatibility studies of electrospun medical grade polyurethane (Carbothane™ 3575A) nanofibers and composite nanofibers containing multiwalled carbon nanotubes. J Mech Behav Biomed Mater. 2015;41:189–198.
- Reneker DH, Yarin AL. Electrospinning jets and polymer nanofibers. Polymer (Guildf). 2008;49(10):2387–2425.
- Pal DB, Singh P, Mishra PK. Composite ceria nanofiber with different copper loading using electrospinning method. J Alloys Compd. 2017;694:10–16.
- Ren L-F, Xia F, Shao J, et al. Experimental investigation of the effect of electrospinning parameters on properties of superhydrophobic PDMS/PMMA membrane and its application in membrane distillation. Desalination. 2017;404:155–166.
- Li D, Xia Y. Electrospinning of nanofibers: reinventing the wheel? Adv Mater. 2004;16(14):1151–1170.
- Alfaro De Prá MA, Ribeiro-do-Valle RM, Maraschin M, et al. Effect of collector design on the morphological properties of polycaprolactone electrospun fibers. Mater Lett. 2017;193:154–157.
- Garg K, Bowlin GL. Electrospinning jets and nanofibrous structures. Biomicrofluidics. 2011;5(1):13403.
- Asiabi M, Mehdinia A, Jabbari A. Preparation of water stable methyl-modified metal-organic framework-5/polyacrylonitrile composite nanofibers via electrospinning and their application for solid-phase extraction of two estrogenic drugs in urine samples. J Chromatogr A. 2015;1426:24–32.
- Hosseini SR, Ghasemi S, Kamali-Rousta M. Preparation of CuO/NiO composite nanofibers by electrospinning and their application for electro-catalytic oxidation of hydrazine. J Power Sources. 2017;343:467–476.
- Burke L, Mortimer CJ, Curtis DJ, et al. In-situ synthesis of magnetic iron-oxide nanoparticle-nanofibre composites using electrospinning. Mater Sci Eng C Mater Biol Appl. 2017;70(Pt 1):512–519.
- Kitsara M, Agbulut O, Kontziampasis D, et al. Fibers for hearts: a critical review on electrospinning for cardiac tissue engineering. Acta Biomater. 2017;48:20–40.
- Ahmed FE, Lalia BS, Hashaikeh R. A review on electrospinning for membrane fabrication: Challenges and applications. Desalination. 2015;356:15–30.
- Navarro-Pardo F, Martinez-Hernandez AL, Velasco-Santos C. Carbon nanotube and graphene based polyamide electrospun nanocomposites: a review. J Nanomater. 2016;2016:1–16.
- Rezaei B, Ghani M, Shoushtari AM, et al. Electrochemical biosensors based on nanofibres for cardiac biomarker detection: a comprehensive review. Biosens Bioelectron. 2016;78:513–523.
- Rosenberger AG, Dragunski DC, Muniz EC, et al. Electrospinning in the preparation of an electrochemical sensor based on carbon nanotubes. J Mol Liq. 2020;298:112068.
- Da Silva HA, Da Silva MB. Aplicação de um projeto de experimentos (DOE) na soldagem de tubos de zircaloy-4. Revista P&E. 2016;1(1):41.
- Hibbert DB. Experimental design in chromatography: a tutorial review. J Chromatogr B Analyt Technol Biomed Life Sci. 2012;910:2–13.
- Dong Y, Bickford T, Haroosh HJ, et al. Multi-response analysis in the material characterisation of electrospun poly (lactic acid)/halloysite nanotube composite fibres based on Taguchi design of experiments: fibre diameter, non-intercalation and nucleation effects. Appl Phys A. 2013;112(3):747–757.
- Mohammad Khanlou H, Chin Ang B, Talebian S, et al. Electrospinning of polymethyl methacrylate nanofibers: optimization of processing parameters using the Taguchi design of experiments. Text Res J. 2015;85(4):356–368.
- Seyedmahmoud R, Rainer A, Mozetic P, et al. A primer of statistical methods for correlating parameters and properties of electrospun poly(L-lactide) scaffolds for tissue engineering-PART 1: design of experiments. J Biomed Mater Res A. 2015;103(1):91–102.
- Simonoska Crcarevska M, Dimitrovska A, Sibinovska N, et al. Implementation of quality by design principles in the development of microsponges as drug delivery carriers: identification and optimization of critical factors using multivariate statistical analyses and design of experiments studies. Int J Pharm. 2015;489(1-2):58–72.
- Xie G, Chen Z, Ramakrishna S, et al. Orthogonal design preparation of phenolic fiber by melt electrospinning. J Appl Polym Sci. 2015;132(38):42574–42578.
- Borrotti M, Lanzarone E, Manganini F, et al. Defect minimization and feature control in electrospinning through design of experiments. J Appl Polym Sci. 2017;134: 44740–44750.
- Gunst RF, Mason RL. Fractional factorial design. Wiley Interdiscip Rev Comput Stat. 2009;1(2):234–244. doi:10.1002/wics.27.
- Kim B, Sigmund WM. Functionalized multiwall carbon nanotube/gold nanoparticle composites. Langmuir. 2004;20(19):8239–8242.
- Li J, He W-D, Yang L-P, et al. Preparation of multi-walled carbon nanotubes grafted with synthetic poly(l-lysine) through surface-initiated ring-opening polymerization. Polymer (Guildf). 2007;48(15):4352–4360.
- Rahmatpour A, Kaffashi B, Maghami S. Preparation and rheological properties of functionalized multiwalled carbon nanotube/waterborne polyurethane nanocomposites. J Macromol Sci Part B Phys. 2011;50(9):1834–1846.
- Talaei Z, Mahjoub AR, Rashidi A, Morad, et al. The effect of functionalized group concentration on the stability and thermal conductivity of carbon nanotube fluid as heat transfer media. Int Commun Heat Mass Transf. 2011;38(4):513–517.
- Gómez S, Rendtorff NM, Aglietti EF, et al. Intensity of sulfonitric treatment on multiwall carbon nanotubes. Chem Phys Lett. 2017;689:135–141.
- Gómez S, Rendtorff NM, Aglietti EF, et al. Surface modification of multiwall carbon nanotubes by sulfonitric treatment. Appl Surf Sci. 2016;379:264–269.
- Ramaseshan R, Sundarrajan S, Jose R, et al. Nanostructured ceramics by electrospinning. J Appl Phys. 2007;102(11):111101.
- Saligheh O, Arasteh R, Forouharshad M, et al. Poly(butylene terephthalate)/single wall carbon nanotubes composite nanofibers by electrospinning. J Macromol Sci Part B. 2011;50(6):1031–1041.
- Chen H, Liu Z, Cebe P. Chain confinement in electrospun nanofibers of PET with carbon nanotubes. Polymer (Guildf). 2009;50(3):872–880.
- Ra EJ, An KH, Kim KK, et al. Anisotropic electrical conductivity of MWCNT/PAN nanofiber paper. Chem Phys Lett. 2005;413(1-3):188–193.
- Naebe M, Lin T, Tian W, et al. Effects of MWNT nanofillers on structures and properties of PVA electrospun nanofibres. Nanotechnology. 2007;18(22):225605.
- Lee C-R, Chen L-J. Polyvinylbutyral assisted synthesis and characterization of kesterite quaternary semiconductor Cu2ZnSnSe4 nanofibers by electrospinning route. Sol Energy Mater Sol Cells. 2016;151:24–29.
- Liu H, Hsieh Y-L. Ultrafine fibrous cellulose membranes from electrospinning of cellulose acetate. J Polym Sci B Polym Phys. 2002;40(18):2119–2129.
- Rodrigues BVM, Silva AS, Melo GFS, et al. Influence of low contents of superhydrophilic MWCNT on the properties and cell viability of electrospun poly (butylene adipate-co-terephthalate) fibers. Mater Sci Eng C Mater Biol Appl. 2016;59:782–791.
- Bounioux C, Avrahami R, Vasilyev G, et al. Single-step electrospinning of multi walled carbon nanotubes – poly(3-octylthiophene) hybrid nano-fibers. Polymer (Guildf). 2016;86:15–21.
- Schneider R, Mercante LA, Andre RS, et al. Biocompatible electrospun nanofibers containing cloxacillin: antibacterial activity and effect of pH on the release profile. React Funct Polym. 2018;132:26–35.
- Yuan H, Zhou Q, Zhang Y. 2017. Improving fiber alignment during electrospinning. In: Electrospun Nanofibers. China: Elsevier Ltd; p. 125–147.
- Cañete-Rosales P, Ortega V, Álvarez-Lueje A, et al. Influence of size and oxidative treatments of multi-walled carbon nanotubes on their electrocatalytic properties. Electrochim Acta. 2012;62:163–171.
- De Volder MFL, Tawfick SH, Baughman RH, et al. Carbon nanotubes: present and future commercial applications. Science. 2013;339(6119):535–339.
- McCullen SD, Stevens DR, Roberts WA, et al. Morphological, electrical, and mechanical characterization of electrospun nanofiber mats containing multiwalled carbon nanotubes. Macromolecules. 2007;40(4):997–1003.
- Baji A, Mai YW, Abtahi M, et al. Microstructure development in electrospun carbon nanotube reinforced polyvinylidene fluoride fibers and its influence on tensile strength and dielectric permittivity. Compos Sci Technol. 2013;88:1–8.
- Tijing LD, Park C-H, Choi WL, et al. Characterization and mechanical performance comparison of multiwalled carbon nanotube/polyurethane composites fabricated by electrospinning and solution casting. Compos Part B Eng. 2013;44(1):613–619.
- Wang W, Ciselli P, Kuznetsov E, et al. Effective reinforcement in carbon nanotube–polymer composites. Philos Trans R Soc Lond A Math Phys Eng Sci. 2008;366: 1613–1626.
- Wang S-H, Wan Y, Sun B, et al. Mechanical and electrical properties of electrospun PVDF/MWCNT ultrafine fibers using rotating collector. Nanoscale Res Lett. 2014;9(1):522.
- Grabbert N, Wang B, Avnon A, et al. Mechanical properties of individual composite poly(methyl-methacrylate)-multiwalled carbon nanotubes nanofibers. IOP Conf Ser Mater Sci Eng. 2014;64:012005.
- Assis OBG. Alteração do caráter hidrofílico de filmes de quitosana por tratamento de plasma de HMDS. Quím Nova. 2010;33(3):603–606.
- Goyanes S, Rubiolo GR, Salazar A, et al. Carboxylation treatment of multiwalled carbon nanotubes monitored by infrared and ultraviolet spectroscopies and scanning probe microscopy. Diam Relat Mater. 2007;16(2):412–417.
- Manesh KM, Kim HT, Santhosh P, et al. A novel glucose biosensor based on immobilization of glucose oxidase into multiwall carbon nanotubes-polyelectrolyte-loaded electrospun nanofibrous membrane. Biosens Bioelectron. 2008;23(6):771–779.
- Chen Y, Lu Z, Zhou L, et al. Triple-coaxial electrospun amorphous carbon nanotubes with hollow graphitic carbon nanospheres for high-performance Li ion batteries. Energy Environ Sci. 2012;5(7):7898.
- Zussman E, Yarin AL, Bazilevsky AV, et al. Electrospun polyaniline/poly(methyl methacrylate)-derived turbostratic carbon micro-/nanotubes. Adv Mater. 2006;18(3):348–353.
- Wang Z-G, Xu Z-K, Wan L-S, et al. Nanofibrous membranes containing carbon nanotubes: electrospun for redox enzyme immobilization. Macromol Rapid Commun. 2006;27(7):516–521.
- Mei F, Zhong J, Yang X, et al. Improved biological characteristics of poly(L-lactic acid) electrospun membrane by incorporation of multiwalled carbon nanotubes/hydroxyapatite nanoparticles. Biomacromolecules. 2007;8(12):3729–3735.
- Im JS, Bai BC, Lee Y-S. The effect of carbon nanotubes on drug delivery in an electro-sensitive transdermal drug delivery system. Biomaterials. 2010;31(6):1414–1419.
- Yang L, Leung WW-F. Electrospun TiO2 nanorods with carbon nanotubes for efficient electron collection in dye-sensitized solar cells. Adv Mater Weinheim. 2013;25(12):1792–1795.