Abstract
The periodontal pathogen Tannerella forsythia expresses several glycosidases which are linked to specific growth requirements and are involved in the invasion of host tissues. α-l-Fucosyl residues are exposed on various host glycoconjugates and, thus, the α-l-fucosidases predicted in the T. forsythia ATCC 43037 genome could potentially serve roles in host-pathogen interactions. We describe the molecular cloning and characterization of the putative fucosidase TfFuc1 (encoded by the bfo_2737 = Tffuc1 gene), previously reported to be present in an outer membrane preparation. In terms of sequence, this 51-kDa protein is a member of the glycosyl hydrolase family GH29. Using an artificial substrate, p-nitrophenyl-α-fucose (KM 670 μM), the enzyme was determined to have a pH optimum of 9.0 and to be competitively inhibited by fucose and deoxyfuconojirimycin. TfFuc1 was shown here to be a unique α(1,2)-fucosidase that also possesses α(1,6) specificity on small unbranched substrates. It is active on mucin after sialidase-catalyzed removal of terminal sialic acid residues and also removes fucose from blood group H. Following knock-out of the Tffuc1 gene and analyzing biofilm formation and cell invasion/adhesion of the mutant in comparison to the wild-type, it is most likely that the enzyme does not act extracellularly. Biochemically interesting as the first fucosidase in T. forsythia to be characterized, the biological role of TfFuc1 may well be in the metabolism of short oligosaccharides in the periplasm, thereby indirectly contributing to the virulence of this organism. TfFuc1 is the first glycosyl hydrolase in the GH29 family reported to be a specific α(1,2)-fucosidase.
Abbreviations
Amp | = | ampicillin |
BHI | = | brain heart infusion medium |
CBB | = | Coomassie brilliant blue G 250 |
DFJ | = | deoxyfuconojirimycin |
Erm | = | erythromycin |
TfFuc1 | = | T. forsythia ATCC 43037 fucosidase-1 encoded by the bfo_2737 gene, equally Tffuc1 |
FDH | = | fucose dehydrogenase |
HPAEC | = | high-performance anion-exchange chromatography with pulsed amperometric detection |
LC-ESI-MS | = | liquid chromatography-electrospray ionisation-mass spectrometry |
rTfFuc-1 | = | recombinant TfFuc1 enzyme |
NAM | = | N-acetylmuramic acid |
PBS | = | phosphate-buffered saline |
pNP- | = | fucose |
4-nitrophenyl-α-l- | = | fucopyranoside |
SDS-PAGE | = | sodium dodecylsulphate polyacrylamide gel electrophoresis |
T. forsythia | = | Tannerella forsythia ATCC 43037 |
WT | = | wild-type bacterium |
Introduction
Tannerella forsythia is a Gram-negative anaerobic oral pathogen, a member of the so-called “red complex” of bacteria that causes a set of inflammatory diseases named periodontitis, affecting millions of people worldwide.Citation1-3 The effects on the periodontium include loss of the alveolar bone around the teeth, swelling and bleeding of the gum and, in more severe cases, loss of teeth. Periodontitis has also been linked to systemic inflammation and to an increased risk of stroke, heart attacks and atherosclerosis, among others.Citation4
Like other bacteria residing in human hosts, T. forsythia has adapted to better suit its niche with cell surface glycosylation thought to be key to this adaptation.Citation5 As previously described, T. forsythia cells are completely covered by a unique surface (S‑) layer formed by co-assembly of 2 different proteins both of which are highly O‑glycosylated with an equally unique glycan.Citation6-8 Mutant strains lacking either the S‑layer or glycan assembly and maturation genes, display phenotypes involving altered human cell attachment to host cells, biofilm formation, and disease progression.Citation9-11 In addition, the structure of the glycan partially imitates that of host glycoproteins, having a terminal sialic acid-like residue (precisely, a modified pseudaminic acid residue) and a terminal fucose, with the latter shown to be present in substoichiometric amounts and linked to a methylated galactose in an unknown glycosidic linkage.Citation8 The glycobiology of this pathogen, including its repertoire of glycosidases, seems to be key to its physiology and, potentially, its pathogenicity.Citation5,12
Recent evidence suggests that for several periodontal pathogens, but particularly for the “red complex” organism T. forsythia, sialic acid-containing host molecules play an important role in vivo.Citation13 Two different sialidases have been found in T. forsythia, SiaHI and NanH. In the case of SiaHI, its function is unclear. It is not a canonical sialidase (i.e., not in the GH35 family), a siaH1 mutant has no discernible phenotype, and experiments point to it being a periplasmic protein without any role in extracellular interactions.Citation14 These same studies also indicated that mutants lacking the main T. forsythia sialidase NanH had hindered attachment and invasion of human oral epithelial cells. The enzyme was also seen to play an important role in biofilm growth on surfaces coated with salivary glycoproteins.Citation15 The nanH gene is located in a large cluster that contains all the genes required for sialic acid catabolism, which indicates that the cleaved sialic acid can additionally be taken up and utilized.Citation12,13 This gene cluster also contains a β‑hexosaminidase that may cleave sub-terminal residues after sialidase action and may also play a role in vivo.Citation15 Additionally, in a separate study, transcriptome analysis of the oral microbiome also showed up-regulation of the sialidase nanH mRNA in dental plaque.Citation16
α-l-Fucosyl residues are, like sialic acid, frequently located at a terminal position on various host glycoconjugates including blood groups, milk oligosaccharides, gastric and submaxillary mucin, and serum glycoproteins.Citation17,18 Therefore, fucosidases in T. forsythia could potentially play similar roles to sialidases.Citation19 It has generally been shown that terminal fucose residues play important roles in mammalian cell-cell communication and also in their interaction with pathogenic bacteria; for instance, Campylobacter jejuni and Helicobacter pylori are known to bind certain fucosylated blood groups (e.g., 0‑antigen) in order to mediate infection.Citation19-21 In addition, the ability to utilize available fucose provides many bacteria with a nutritional advantage and contributes to survival in a highly competitive ecosystem, such as the human body.Citation22,23
Characterization of α-l-fucosidases in T. forsythia could aid in the elucidation of the structure-function relationship of fucosylated host and bacterial surfaces in the virulence of oral pathogens. The genome of T. forsythia encodes 3 putative α-l-fucosidases, BFO_2737 and BFO_1182, both classified in the CAZy (Carbohydrate Active enZymes; http://www.cazy.org/) glycosyl hydrolase family GH29, and BFO_3101, classified in family GH95. While all 3 enzymes possess a glycosyl hydrolase domain and are classified by CAZy according to their mechanism of action, BFO_1182 and BFO_3101 are not strictly annotated as α-l-fucosidases but as a F5/8 type C domain protein and a putative lipoprotein, respectively.
Here, we describe the molecular cloning and characterization of BFO_2737, which we named TfFuc1. This protein has previously been reported to form part of an outer membrane preparation of T. forsythiaCitation24 and, thus, was a good candidate to be involved in host-pathogen interactions. TfFuc1 is a 446-amino acid protein with a theoretical pI and molecular mass of 6.9 and 50.8 kDa, respectively. It is the first fucosidase in this organism to be characterized to date. The enzyme was shown here to be an α(1,2)‑fucosidase and also possesses an α(1,6) specificity on small unbranched substrates. It is a predicted periplasmic protein, possibly playing a role in the breakdown of small oligosaccharides. It is, to the best of our knowledge, the first glycosyl hydrolase in its family (GH29) reported to be a specific α(1,2)-fucosidase.
Results
Enzymatic characterization of rTfFuc1
The Tffuc1 gene was cloned into pET22-b(+) vector and expressed in E. coli as a C-terminally His6-tagged protein, which enabled purification via nickel affinity chromatography (). The enzymatic activity was then tested using the standard colorimetric α-fucosidase substrate 4-nitrophenyl-α-l-fucopyranoside (pNP-fucose) at 22°C in a range of different pH values and in the presence of MgCl2, KCl and NaCl, in order to establish its pH optimum and cation dependence, respectively. By stopping the reaction with the addition of an alkaline buffer at pH 11.4, it was ensured that all wells where at the same pH for consequent absorbance readings (Fig. 2). The activity of the enzyme was seen to start to plateau at the neutral to alkaline pH range and was considered most active at pH 9.0, assayed in glycine buffer, and not at pH 9.25 where the activity suddenly peaks and then rapidly decreases thereafter. The activity remained largely unaffected by the presence of cations at the 2 concentrations tested (results not shown). The KM and Vmax catalytic constants at 22°C, calculated from the activity of the enzyme at different pNP-fucose concentrations, were 670 μM and 20.4 μmol/min (U) per mg of protein, respectively (). The determined catalytic constants for rTffuc1 are in the range of those reported for other fucosidases/ glycosylhydrolases when tested on their corresponding pNP-substrates.Citation25-27
Table 1. rfFuc1 activity on 4-nitrophenyl-α-l-fucopyranoside (pNP-fucose). Catalytic constants KM and Vmax and the inhibitory effect of DFJ and l-fucose were measured using the colorimetric substrate pNP-fucose within a concentration range from 0.01 to 50 mM at 22°C in glycine buffer at pH 9.0
Figure 1. SDS PAGE (A) and Western immunoblot (B) of total cell extracts from T. forsythia WT (lane 2) and ΔTffuc1 strains (lane 3) and of the His6-tagged rTfFuc1 as purified from E. coli (lane 4), used for activity studies and to raise a polyclonal anti-TfFuc1 antiserum. Western immunoblotting using the anti-TfFuc1 antiserum recognized the protein (∼51 kDa) specifically in the WT strain (lane 2) and indicated absence of the protein in the ΔTffuc1 strain (lane 3), proving that the enzyme was effectively knocked-out. In the preparation of rTfFuc 1 (B, lane 4), the polyclonal antiserum recognizes also minor contaminating E. coli proteins not visible on the SDS-PAGE gel (A, lane 4). Mm; PageRuler Plus prestained protein ladder (Thermo Scientific).
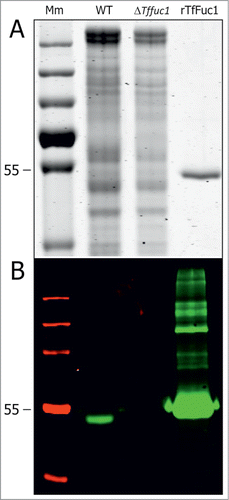
Substrate linkage specificity of rTfFuc1
To determine the enzyme linkage specificity, rTfFuc1 was incubated with a set of different fucosylated substrates of defined structure representing a range of fucose linkages available on host glycoproteins and on oral surfaces (). The reaction products obtained after overnight incubation were analyzed using high-performance anion-exchange chromatography with pulsed amperometric detection (HPAEC) where the release of fucose was confirmed by comparison with the retention time of the standard monosaccharide and of a substrate standard after overnight incubation at 37°C. The enzyme was seen to be active on both α(1,2) fucose containing substrates, 2‑fucosyllactose and H-trisaccharide, and on the α(1,6) fucose disaccharide α-l-Fuc-(1,6)-β-d-GlcNAc, although this latter reaction did not reach completion, indicating weak specificity for this linkage. The α(1,3) and α(1,4) linkages were not cleaved on 3-fucosyllactose and the Lewis A trisaccharide, respectively. The enzyme was also inactive on the substrate α‑l-Fuc-(1,4)-β-d-Gal, added as a second disaccharide control to prove that the α(1,6) activity was not due to differences in substrate length ().
Figure 2. pH profile of rTfFuc1 using 4-nitrophenyl-α-l-fucopyranoside (pNP-fucose) as a substrate. Activity was measured as the increase in Abs405 due to the released 4-nitrophenol product. Citrate/phosphate buffer (0.1 M) was used to assay the pH range from 3–8, 50 mM glycine buffer was used for the pH range from 8.0–10.25.
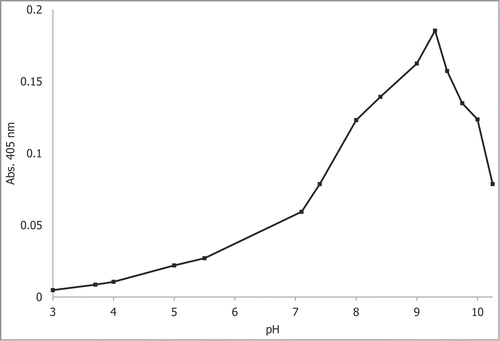
Figure 3. Fucosylated substrates used in this study. The structures are depicted according to the symbolic nomenclature of the Consortium for Functional Glycomics (http://www.functionalglycomics.org/static/consortium/Nomenclatures.html).
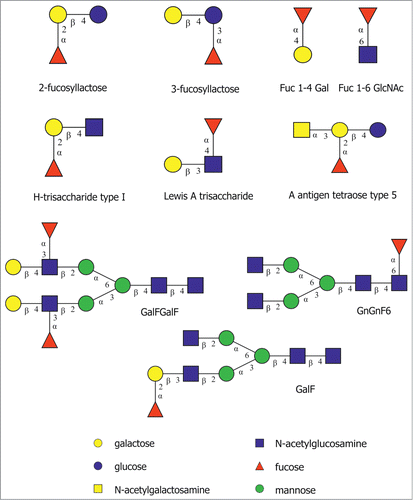
Figure 4. rTfFuc1 activity on standard fucosylated substrates after overnight incubation as determined by HPAEC. Blue lines represent samples which were incubated in absence of rTfFuc1 (substrate standard) and red lines represent samples incubated in the presence of rTfFuc1. Cleavage of the substrates was determined by the appearance of a fucose peak, as determined by the retention time of the standard monosaccharide.
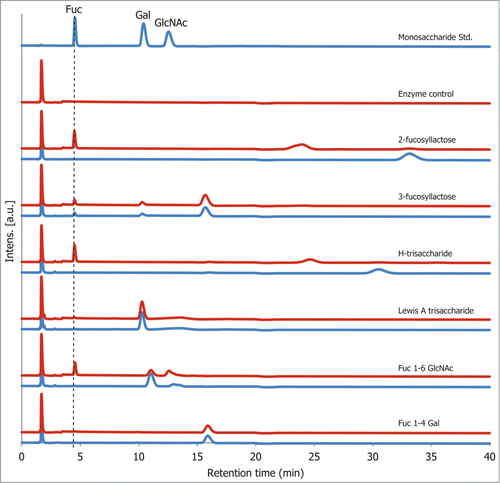
In order to obtain accurate activity values on the cleaved substrates, the K-FUCOSE kit from Megazyme was used, coupled to the enzymatic reaction with rTfFuc1. First, FDH, which also has an alkaline pH optimum, and NADP+ were added to the substrate solution reaction mixture in order to convert any free fucose already present in the sample to l-fucono-1,5-lactone by the reduction of NADP+ to NADPH (ϵ340 = 6.022 mM−1cm−1). rTfFuc1 was then added to the mixture and the reaction was monitored by following the increase in Abs340. The activity was calculated from where the formation of NADPH was linear over time. The enzyme was most active on 2‑fucosyllactose and H-trisaccharide with specific activities of 0.8 U/mg and 0.6 U/mg, respectively. The activity on the α(1,6) disaccharide was significantly lower at 0.35 U/mg ().
Table 2. rTfFuc1 activity on standard fucosylated substrates. Cleavage was determined by HPAEC after overnight incubations with rTfFuc1 (see , with the exception of A antigen tetraose) and specific activities were calculated using the K-FUCOSE kit
The activity of the enzyme on the various substrates could be calculated approximately (as some loss of material occurred during sample preparation) from the HPLC experiments after 1‑h incubation periods (results not shown) and was found to be markedly lower than that observed with the K-FUCOSE kit, indicating that free fucose, which is consumed in the latter, could be inhibiting the enzymatic activity significantly. In order to determine the extent of such an effect, measurement of KM and Vmax values were repeated with pNP-fucose in the presence of either 0.25 mM l-fucose or 0.1 μM deoxyfuconojirimycin (DFJ), which is a strong fucosidase inhibitor.Citation28 The enzyme was competitively inhibited by both fucose and DFJ as the Vmax remained largely unaffected but the KM value increased from 0.67 mM to 16.5 mM and 28.3 mM, respectively ().
Further, the ability of the enzyme to cleave fucose residues off more complex natural glycans and those on branched sugar residues was assayed (compare with ). As expected, the enzyme was unable to cleave the α(1,3) fucose linkage on GalFGalF-pep, included in the assays, as a trace amount of activity could be observed when using 3-fucosyllactose, as measured by the K-FUCOSE kit. The enzyme was also not able to cleave the core α-1,6 fucose linkage on GnGnF6-pep nor the branched α(1,2)-linked fucose on the A antigen. The non-branched α(1,2) fucose linkage present on the Eastern oyster substrate, however, was cleaved off the substrate GalF, seen by the loss of a fucose residue in the MS spectra of the substrate. The major m/z 1703 glycan ([M+H]+) was approximately 50% digested to a defucosylated species of m/z 1557 after overnight incubation with the enzyme (). The enzyme is, therefore, able to cleave off fucose residues which are α(1,2) linked on more complex glycans only when in a terminal unbranched position and is unable to cleave core α(1,6) fucose. This data supports that the enzyme acts as an α(1,2) fucosidase.
Figure 5. Cleavage of natural α(1,2) fucosylated glycans by rTfFuc1. Cleavage of fucose from a large N-glycan substrate was monitored by MALDI-TOF MS spectra after overnight incubation; the conversion of the m/z 1703 glycan (GalF) to one of m/z 1557 (Δm/z 146) is indicative of the loss of fucose. The structures of the substrate and product are depicted according to the symbolic nomenclature of the Consortium for Functional Glycomics.
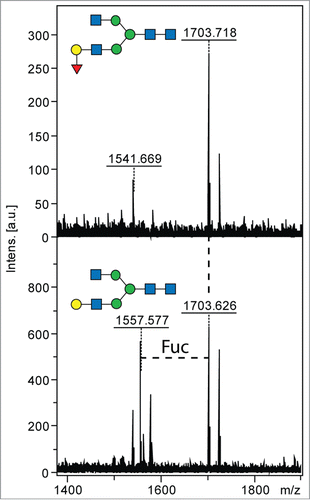
rTfFuc1 activity on bovine submaxillary mucin
rTfFuc1 was incubated with mucin from bovine submaxillary glands and the release of fucose was measured with the K-FUCOSE kit. Incubation was performed also in combination with rNanH from T. forsythia and activities were calculated from the slope of ΔAbs340 where it was linear over time. During the assayed incubation period of 10 min, no activity could be detected when rTfFuc1 was incubated alone with mucin. The ΔAbs340 lead to an irregular data set with a very low r2 value. However, fucose release was detectable when the incubation was performed in conjunction with the rNanH sialidase, presenting a slow but steady increase in the Abs340. The activity was calculated over a period of 300 s where the data points fitted a linear regression with an r2 of 0.98 (). The experiment was repeated several times and yielded an activity of 24 ± 4 mU/mg. rTfFuc1 might cleave fucose off mucin over longer periods of time, but the data shows a significantly higher activity when sialic acid residues are first removed from mucin, indicating that the fucosidase TfFuc1 could work downstream from the sialidase in T. forsythia and presumably cooperate with other glycosidases in the degradation of complex glycans.
Figure 6. rTfFuc1 was incubated with mucin from bovine submaxillary glands and the release of fucose was measured with the K-FUCOSE kit. When incubations were performed in conjunction with the rNanH sialidase, a slow steady increase in the Abs340 was observed. The activity was calculated over a period of 300 s where the data points fitted a linear regression with an r2 of 0.98. No activity could be detected when rTfFuc1 was incubated alone with the mucin. The ΔAbs340 lead to an irregular data set with a very low r2 value of 0.4.
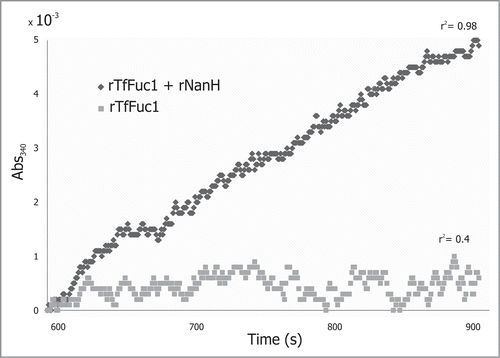
Cellular localization of TfFuc1
TfFuc1 was previously reported to be present in the outer membrane fraction of T. forsythia.Citation24 In an effort to investigate its presence on the surface of T. forsythia cells, TfFuc1-specific polyclonal antiserum was raised against the recombinant enzyme in mice and used for Western immunoblotting of cellular fractions separated by SDS-PAGE. Protein visualization by CBB staining showed good separation between the fractions, as the S‑layer bands were very prominent in the outer membrane fraction but not in the inner-membrane and non-membrane associated fractions. Western immunoblotting showed that all the detectable TfFuc1 fucosidase was found in the non-membrane associated fraction comprising both the cytoplasmic and periplasmic content (), arguing against surface localization of the TfFuc1 enzyme.
Figure 7. Presence of TfFuc1 in cell fractions of T. forsythia WT. (A) SDS-PAGE analysis of the outer membrane fraction (OM) (1), membrane fraction (2) and non-membrane associated fraction (3) showed good separation between the fractions, as the S‑layer bands were very prominent in the OM but not in the membrane and non-membrane associated fractions. Protein loaded was 20 μg of the OM and non-membrane associated fractions and 400 μg of the membrane fraction. Protein visualization was by CBB. (B) Western immunoblot using anti-TfFuc1 antiserum showed the TfFuc1 fucosidase in the non-membrane associated fraction comprising both the cytoplasmic and periplasmic content. Mm; PageRuler Plus prestained protein ladder (Thermo Scientific).
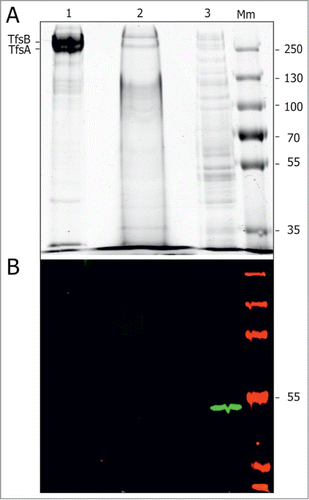
Discussion
Colonization of the periodontal pocket by the pathogenic late colonizer T. forsythia depends largely on pre-existing bacteria that have already tipped the oral balance away from health and toward disease.Citation29 Factors such as a pH shift from neutral to alkaline and slight raises in the temperature due to the host inflammatory response could be contributing factors favoring the process.Citation30 In a situation of oral disease, the number of different bacteria living in the gingival crevice decreases markedly due to putative pathogenic bacteria being more competitive in such an environment.Citation31 It is in these conditions that T. forsythia seems to thrive and becomes one of the key players in severe cases of periodontitis.
The NanH sialidase in T. forsythia has been well established to play important roles in adherence to sialylated glycoprotein-coated surfaces and epithelial cells in addition to triggering biofilm growth and being up-regulated in dental plaque.Citation14-16 As the other important terminal sugar on host glycoproteins is fucose, here, we performed an initial characterization of a putative α-l-fucosidase encoded in the T. forsythia genome, product of the gene Tffuc1, previously reported to be part of the outer membrane proteome.Citation24
By producing the enzyme recombinantly in E. coli we were able to show that the enzyme is active across a broad pH range from 7.0–9.0, having an unusually high pH optimum of 9.0. It presents a unique α(1,2)-linkage specificity on terminal non-branched fucose residues, being also active on small non-branched α(1,6) fucosylated substrates. While both these linkages are cleaved at a considerable rate in the case of small linear substrates, the α(1,6) specificity is not detected on core fucoses on more complex glycopeptides. The α(1,2) linkage specificity was apparent on both small linear substrates, such as 2-fucosyllactose and H-trisaccharide, and on more complex glycans only when fucose occupied a terminal position, but not on a branched substrate where the fucose residue is linked to a fully substituted sugar. The enzyme seems to be, to the best of our knowledge, the first fucosidase in its GH family (GH29) to have a specific α(1,2) activity. The broad, high pH activity profile of this fucosidase ties in with its physiological niche which is known to have a pH that rises as periodontal disease progresses.Citation30 The possession of such enzymes with higher activities in alkaline surroundings could contribute to competiveness and virulence of T. forsythia in a diseased environment.
During the course of this study, it became clear that one of the issues possibly underlining our observations was the enzyme's cellular localization. Even though TfFuc1 was found previously to be present in the outer membrane proteome of the pathogen,Citation24 localization of the enzyme on the surface of T. forsythia cells by fluorescent immunolabelling was not successful (data not shown; see Experimental Procedures in the Supplementary Information) and cell fractionation also showed the detectable protein to be found in the non-membrane associated fraction (). This would be in agreement with bioinformatics interrogation indicating that, while it has a Sec-dependent secretion signal (as predicted by SignalP 4.1 Server), it is not predicted to reside in the outer membrane or be secreted, nor does it contain a T9SS C-terminal secretion signal.Citation32 The difference in location of TfFuc1 between the present study and the outer membrane proteomics experimentCitation24 can be reconciled when considering that in the latter study, cross-contamination of individual proteins between cellular fractions was not investigated.
Incubation of the recombinant fucosidase with bovine submaxillary mucin showed no detectable release of fucose over an incubation period of 10 min. Activity on this complex substrate could only be detected when the incubation was performed in combination with the recombinant NanH sialidase from T. forsythia. It is, therefore, conceivable that TfFuc1 could play an accompanying role to the sialidase in the interaction between T. forsythia and host glycoproteins, but given its periplasmic location, this could merely reflect the need for removal of terminal sialic acid residues for the enzyme to work, either indicating that it most likely acts on internalised fucosyl substrates after sialic acid has already been removed by the action of sialidases or, less likely, that it acts in concert with sialidases externally.
This notion that the T. forsythia fucosidase TfFuc1 plays an internal role was corroborated when we tested the effect of the ΔTffuc1 mutation on the ability of T. forsythia to interact with and invade human oral epithelial cells using an antibiotic protection assay on the oral epithelial cell line H357.Citation33 We found no significant differences in the ability of the ΔTffuc1 to invade these human cells as compared to the WT strain (Fig. S1), indicating that TfFuc1 has no effect on epithelial cell-invasion under the conditions tested. In addition, the mutant did not show hindered biofilm formation when cultured on bovine submaxillary mucin, contrary to the T. forsythia NanH sialidase mutant,Citation15 but showed a slightly increased biofilm formation (Fig. S2).
Our data supports the idea of a periplasmic fucosidase involved in the final breakdown of small substrates that have been internalized, possibly owing to the action of exoglycosidases and endoglycosidases which break-down larger glycans on the outside of the cell.Citation34,35 TfFuc1 would possibly then be able to exert its full potential freeing both α(1,2) and α(1,6) fucoses on small linear substrates. These findings are also in agreement with the hypothesis that the fucosidase acts downstream of the sialidases, which have been shown to act on whole glycoproteins on bacterial and host surfaces.Citation14,15,36 The sialic acid would, therefore, already be missing as smaller parts of the glycan are transported inside the cell.
T. forsythia has no straightforwardly identifiable fucose catabolism locus in its genome, nor does it have the bifunctional l-fucokinase/GDP-fucose pyrophosphorylase required normally for Bacteroidetes to recycle the fucose into its glycans.Citation23 In an effort to see the effect on the latter scenario, the fucose containing S‑layer glycan from both the WT and the ΔTffuc1 strains were compared by LC-ESI-MS with no obvious change under the growth conditions used (Z.A. Megson, L. Neumann, F. Altmann, C. Schäffer, unpublished data). However, the microheterogeneity of the S-layer glycan regarding the terminal fucose residue complicates interpretation of MS data. Therefore, it remains unclear whether the released fucose in the periplasm can be used as a nutrient source or is recycled by the bacteria into its glycosylation pathway, and, thus, is subject of further studies.
Overall, our data suggest that TfFuc1 is a unique α-l-(1,2)-fucosidase which could potentially contribute to fucose utilization in T. forsythia. In order to better elucidate this role and rule out any redundancy in the system, 2 further annotated fucosidases in T. forsythia, BFO_1182 and BFO_3101, together with the annotated fucose permease, BFO_0307, are now being investigated to elucidate the role of fucose in the physiology of T. forsythia.
Experimental Procedures
Bacterial strains, medium and culture conditions
T. forsythia wild-type (WT) strain ATCC 43037 (American Type Culture Collection) and the knockout mutant ΔTffuc1 were grown anaerobically at 37°C for 4–7 d in brain heart infusion (BHI) broth or 0.8% (w/v) BHI agar, supplemented with N‑acetylmuramic acid (NAM), horse serum and gentamycin as described previously.Citation32 Escherichia coli DH5α and BL21 (DE3) (Invitrogen) were cultivated in selective Luria Bertani (LB) medium (agar and broth) supplemented with 100 μg/ml ampicillin (Amp). All strains and plasmids used in the course of this study are summarised in.
Table 3. Bacterial strains and plasmids used in this study
Molecular methods
All enzymes were purchased from Fermentas. Genomic DNA of T. forsythia WT strain ATCC 43037 was isolated from 2 ml of bacterial suspension as described previously and used as the DNA template in all PCRs, unless otherwise specified.Citation37 The GeneJET™ Gel Extraction Kit (Fermentas) was used to purify DNA fragments from agarose gels and to purify digested plasmids and oligonucleotides. Plasmid DNA from transformed cells was isolated with the GeneJET™ Plasmid Miniprep kit (Fermentas). Agarose gel electrophoresis was performed as described elsewhere.Citation38 Primers for PCR and DNA sequencing were purchased from Invitrogen (). PCR was performed using the Phusion®High-Fidelity DNA Polymerase (Fermentas) and a My CyclerTM (Bio-Rad) thermal cycler. Transformation of chemically competent E. coli DH5α and BL21 (DE) cells was performed according to the manufacturer's protocol (Invitrogen). E. coli transformants were screened by PCR using RedTaq ReadyMix PCR mix (Sigma-Aldrich) and recombinant clones were analyzed by restriction mapping. Expression vector and knock-out cassette were sequenced (Microsynth) prior to transformation.
Table 4. Oligonucleotide primers used for PCR amplification reactionsFootnotea
Recombinant production of His6-tagged TfFuc1
The Tffuc1 gene was amplified from the chromosome of T. forsythia ATCC 43037 with a fused C-terminal His6-tag by PCR using primer pair 1/2 (). The His6-tagged amplification product was digested using restriction enzymes NdeI/XhoI and cloned into NdeI/XhoI-linearized pET22‑b (Novagen). The corresponding plasmid was transformed into E. coli BL21 (DE3) cells for protein expression. Freshly transformed cells were grown in 2 400‑ml Erlenmeyer flasks to an OD600 of 0.4–0.5 in the presence of 100 μg/ml of Amp at which point protein expression was induced with a final concentration of 1 mM isopropyl-β-d-thiogalactopyranoside (IPTG) and cultures were shaken (200 rpm) overnight at 18°C. Cells were harvested by centrifugation (6500 g, 20 min, 4°C).
Collected bacterial cells were lysed by sonication in buffer A (50 mM phosphate buffer pH 8, 0.3 M NaCl) containing 5 mM imidazole and cleared lysates after ultracentrifugation at 150000 g for 30 min at 4°C were incubated with 1 ml of Ni-NTA beads (Qiagen) for 1 h at 4°C, shaking slightly. The beads were placed in a chromatography column and the His6-tagged protein was purified using an imidazole gradient in buffer A; 25 mM imidazole (10 ml), 50 mM imidazole (10 ml), followed by 5 elution steps with 500 μl of 250 mM imidazole in buffer A. Eluted fractions containing the purified recombinant protein, rTfFuc1, as determined by SDS-PAGE analysis, were pooled and dialysed overnight at 4°C against 3 l of 10 mM phosphate buffer, pH 8.0. The volume was then reduced 5-fold using a concentration centrifuge yielding a protein concentration of 0.35 mg/ml (as determined by Nanodrop) in 50 mM phosphate buffer.
Construction of a T. forsythia ΔTffuc1 knockout strain
Disruption of the Tffuc1 gene in T. forsythia was performed by gene knockout, as described previously.Citation32 The Tffuc1 gene is not part of an operon, thus, downstream effects due to the chosen mutation strategy are not expected to occur. Briefly, the flanking genomic regions (1000 bp) up-stream and down-stream of Tffuc1 were amplified using primer pairs 3/4 and 5/6, respectively (). The two resulting fragments were joined with the erythromycin resistance gene ermF-ermAM (amplified using primer pair 7/8) by overlap extension PCR and sub-cloned into the blunt-end cloning vector pJET1.2 (Thermo Scientific), resulting in pJET1.2/Tffuc1_ko. Approximately 5 μg of the knockout cassette was transferred by electroporation into 100 μl of competent T. forsythia cells. Cells were regenerated in BHI medium for 24 h before plating on BHI agar plates containing erythromycin (10 μg/ml) as a selection marker. Single colonies were picked and used for inoculation of liquid BHI medium. Genomic DNA of the new ΔTffuc1 mutants were isolated as mentioned above and the absence of the Tffuc1 gene and the correct integration of the erythromycin resistance gene (upstream and downstream) was evaluated by PCR using primer pairs 1/2, 9/10, and 11/12, respectively (). Absence of the enzyme in the ΔTffuc1 strain was also confirmed by Western immunoblotting of the total cell extract separated by SDS-PAGE using TfFuc1-specific polyclonal antiserum ().
General and analytical methods
SDS-PAGE was carried out according to a standard protocol using a Protean II electrophoresis apparatus (Bio-Rad).Citation39 Protein bands were visualized with Coomassie brilliant blue G 250 (CBB) staining reagent. For Western immunoblotting of proteins onto a nitrocellulose membrane (Peqlab), a Mini Trans-Blot Cell (Bio-Rad) was used. Detection of the His6-tag fused to rTfFuc1 and detection of TfFuc1 was done with the Li-Cor Odyssey Infrared Imaging System using an anti-His6 mouse antibody (Roche) or TfFuc1-specific polyclonal antiserum raised in mice (EF-BIO), respectively, both in combination with goat anti-mouse IgGIR Dye 800CW conjugate (Li-Cor).
Enzymatic characterization of rTfFuc1
Enzymatic characterization of rTfFuc1 was performed essentially as described elsewhere.Citation40 0.17 μM of purified, recombinant enzyme was incubated with 5 mM of the colorimetric substrate 4-nitrophenyl-α-l-fucopyranoside (pNP-fucose) (Sigma) at a range of different pH values (3.0–10.25) and cation concentrations in 96-well plates at 22°C in a total volume of 40 μl. The enzymatic reaction was stopped after 3 min by addition of 260 μl of phosphate buffer, pH 11.4. Citrate/phosphate buffer (0.1 M) was used to assay the pH range from 3.0–8.0,Citation41 50 mM glycine buffer was used for a pH range from 8.0–10.0. The effect of MgCl2 (5 mM, 10 mM), KCl (5 mM, 10 mM) and NaCl (50 mM, 150 mM) on the enzyme's activity was assayed in the same way in 50 mM glycine buffer, pH 9.
A 4-nitrophenol standard curve was made by measuring the absorbance at 405 nm (Abs405) of 0, 4, 8, 12, 16, 20 and 24 nmol of 4-nitrophenol per well in 300 μl of phosphate buffer, pH 11.4. The KM and Vmax catalytic constants were calculated at pH 9.0 in 50 mM glycine buffer at 22°C in the presence of 0.01 to 50 mM pNP-fucose. The inhibitory effect of fucose and deoxyfuconojirimycin (DFJ) on the KM and Vmax of the enzyme were assayed in the same way in the presence of 0.25 mM fucose and 0.1 μM DFJ, respectively.Citation28 Readings were performed using an Infinite 200 plate reader (TECAN) and catalytic constants were calculated with the Sigma Plot 12, Systat Software.
Substrate specificity of rTfFuc1
For the determination of enzyme linkage specificity, a set of commercially available fucosylated substrates (2-fucosyllactose and 3-fucosyllactose from Dextra laboratories; H-Trisaccharide, Lewis A trisaccharide, Fuc-α-(1,4)-Gal and Fuc-α-(1,6)-GlcNAc, all from Carbosynth) () were incubated with the enzyme and reaction mixtures were analyzed by HPAEC using an ICS3000 chromatographic system (Dionex, Thermo Fisher) on a CarboPac PA-1 column. Incubations were made overnight at 37°C in a total volume of 100 μl by mixing 0.34 μM of enzyme with 0.5 mM of substrate in 50 mM glycine buffer, pH 9.0. In order to minimize the effect of the buffer, the reaction volume was then diluted with 400 μl of Milli-Q water and the enzyme was removed using an Amicon 3 kDa cut-off spin column (Millipore). Twenty-five microliters of this flow through was then applied to the CarboPacPA-1 column using full-loop injection.
In order to obtain reliable activity values, the K-FUCOSE kit (Megazyme) was adapted to suit requirements. In a total volume of 250 μl of 50 mM glycine buffer at pH 9.0, the substrates 2-fucosyllactose, H-trisaccharide and Fuc-α(1,6)-GlcNAc, were incubated separately at a concentration of 0.5 mM with 1.83 μl of fucose dehydrogenase (FDH) and 9.15 μl of NADP+ (both as supplied) in a cuvette at 37°C for 10 min. When the reaction had reached a constant absorbance at 340 nm (Abs340), rTfFuc1 was added to the mixture at a concentration of 0.34 μM and the formation of NADPH was followed by continuous measurement of the increase in Abs340. The activity of the enzyme on each substrate was calculated according to the supplier's specifications from ΔAbs340/min where the formation of NADPH was linear over time. The experiment was repeated with different enzyme dilutions (1:10; 1:100) to prove the reliability of the method.
The ability of the enzyme to cleave fucose residues off more complex natural glycans and those on branched sugar residues was assayed on the substrates A antigen tetraose type 5 (Carbosynth), GnGnF6-peptide, GalFGalF, and an N‑glycan derived from Crassostrea virginica (Eastern oyster) haemocyte treated with chicken liver α-N-acetylgalactosaminidase and bovine β-galactosidase (both from Sigma) to reveal the underlying H epitope presenting a terminal α(1,2) fucose on an unsubstituted β(1,3)-galactose (i.e., histo blood group antigen H), referred to here as GalFCitation42 (). Activity on the A antigen substrate was assayed using the K-FUCOSE kit as described above. For all other substrates, incubations were performed overnight at 37°C and analyzed by MALDI-TOF MS using an Autoflex Speed instrument (Bruker) in positive ion mode with 6-aza-2-thiothymine (ATT) as matrix. Spectra were processed with the manufacturer's software (Bruker Flexanalysis 3.3.80) using the SNAP algorithm with a signal/noise threshold of 6 for MS (unsmoothed) and then manually interpreted.
rTfFuc1 activity on mucin from bovine submaxillary glands
rTfFuc1 was incubated with bovine submaxillary mucin (Sigma) in combination with the His-tagged recombinant NanH sialidase (rNanH) from T. forsythia.Citation15 Incubations were performed at 37°C in 50 mM glycine buffer, pH 9.0, at a final concentration of 0.1 μM of either enzyme, 0.2 mg/ml of mucin and NADP+ and FDH as described above, in a total volume of 250 μl. rTfFuc1 (and rNanH) was added to the mixture after an initial 5‑min incubation period. The release of fucose (ΔAbs340) was followed over 10 min and calculated according to the supplier's specifications from ΔAbs340/min where the formation of NADPH was linear over time.
Presence of TfFuc1 in T. forsythia membrane, outer membrane and non-membrane preparations
Cells were harvested by centrifugation from a 4‑day‑old 100-ml T. forsythia culture. Separation of cellular fractions was performed as described previously.Citation24 Briefly, cells were washed once in Tris (2-amino-2-hydroxymethy-l-propane-1,3-diol)-buffer, pH 7.5, sonicated, and cell debris were removed by centrifugation. The collected supernatant was ultracentrifuged (100000 g, 4°C, 40 min) to separate the whole membrane fraction (pellet) from the membrane non-associated fraction (cytoplasm and periplasm, supernatant). The pellet was resuspended in 2% (w/v) N‑lauroylsarcosine (Sigma) in Tris buffer and mixed. After incubation (2 h, 25°C), the outer membrane fraction (OM) was collected by centrifugation (100000 g, 4°C, 40 min) and the pellet was resuspended in Tris buffer. The protein content was determined in each fraction by the Bradford method (Bio-Rad).Citation43 A total of 20 μg of protein from the OM and non-membrane associated fractions and 400 μg of the membrane fraction was loaded onto an SDS-PAGE gel and ran as described above. The presence of TfFuc1 in each fraction was determined by Western immunoblotting.
Microtiter assays of Hoechst-stained biofilms
T. forsythia WT and ΔTffuc1 strains were compared in respect to the biofilm formationCitation44 on mucin-coated polystyrene microtiter plates in dependency of the strength of the BHI liquid medium. Biofilm was stained with Hoechst 33258 Fluorescent Stain (Thermo Scientific). Details are described in the Supplementary Information.
Attachment and invasion assays
For both attachment and invasion assays, T. forsythia WT and ΔTffuc1 strains were incubated with the oral epithelial cell line H357 (CCL17; American Type Culture Collection) at a multiplicity of infection of 1:100, as described previously.Citation45 Details are described in the Supplementary Information.
Disclosure and Potential Conflict of Interest
No potential conflicts of interests were disclosed.
1010982_Supplementary_Materials.zip
Download Zip (2.9 MB)Acknowledgments
The authors thank Dr. Bernard Henrissat (CAZy) for discussing classification of the TfFuc1 enzyme and Sonja Zayni for excellent technical assistance with HPAEC sugar analysis.
Funding
This work was supported by the Austrian Science Fund FWF project P24317-B22 (to C. S.) and the Doctoral Program Biomolecular Technology of Proteins (FWF project W1224).
Supplemental Material
Supplemental data for this article can be accessed on the publisher's website.
References
- Vos T, Flaxman AD, Naghavi M, Lozano R, Michaud C, Ezzati M, Shibuya K, Salomon JA, Abdalla S, Aboyans V., et al. Years lived with disability (YLDs) for 1160 sequelae of 289 diseases and injuries 1990-2010: a systematic analysis for the global burden of disease study 2010. Lancet 2012; 380:2163-96; PMID:23245607; http://dx.doi.org/10.1016/S0140-6736(12)61729-2
- Socransky SS, Haffajee AD, Cugini MA, Smith C, Kent RL Jr. Microbial complexes in subgingival plaque. J Clin Periodontol 1998; 25:134-44; PMID:9495612; http://dx.doi.org/10.1111/j.1600-051X.1998.tb02419.x
- Haffajee AD, Socransky SS, Patel MR, Song X. Microbial complexes in supragingival plaque. Oral Microbiol Immunol 2008; 23:196-205; PMID:18402605; http://dx.doi.org/10.1111/j.1399-302X.2007.00411.x
- Cullinan MP, Ford PJ, Seymour GJ. Periodontal disease and systemic health: current status. Aust Dent J 2009; 54:S62-9; PMID:19737269; http://dx.doi.org/10.1111/j.1834-7819.2009.01144.x
- Posch G, Sekot G, Friedrich V, Megson ZA, Koerdt A, Messner P, Schäffer C. Glycobiology aspects of the periodontal pathogen tannerella forsythia. Biomolecules 2012; 2:467-82; PMID:24970146; http://dx.doi.org/10.3390/biom2040467
- Sekot G, Posch G, Oh YJ, Zayni S, Mayer HF, Pum D, Messner P, Hinterdorfer P, Schäffer C. Analysis of the cell surface layer ultrastructure of the oral pathogen tannerella forsythia. Arch Microbiol 2012; 194:525-39; PMID:22273979; http://dx.doi.org/10.1007/s00203-012-0792-3
- Lee SW, Sabet M, Um HS, Yang J, Kim HC, Zhu W. Identification and characterization of the genes encoding a unique surface (S-) layer of tannerella forsythia. Gene 2006; 371:102-11; PMID:16488557; http://dx.doi.org/10.1016/j.gene.2005.11.027
- Posch G, Pabst M, Brecker L, Altmann F, Messner P, Schäffer C. Characterization and scope of S-layer protein O-glycosylation in tannerella forsythia. J Biol Chem 2011; 286:38714-24; PMID:21911490; http://dx.doi.org/10.1074/jbc.M111.284893
- Sabet M, Lee SW, Nauman RK, Sims T, Um HS. The surface (S-) layer is a virulence factor of bacteroides forsythus. Microbiol 2003; 149:3617-27; PMID:14663093; http://dx.doi.org/10.1099/mic.0.26535-0
- Sakakibara J, Nagano K, Murakami Y, Higuchi N, Nakamura H, Shimozato K, Yoshimura F. Loss of adherence ability to human gingival epithelial cells in S-layer protein-deficient mutants of tannerella forsythensis. Microbiol 2007; 153:866-76; PMID:17322207; http://dx.doi.org/10.1099/mic.0.29275-0
- Honma K, Inagaki S, Okuda K, Kuramitsu HK, Sharma A. Role of a tannerella forsythia exopolysaccharide synthesis operon in biofilm development. Microb Pathog 2007; 42:156-66; PMID:17363213; http://dx.doi.org/10.1016/j.micpath.2007.01.003
- Douglas CO, Naylor K, Phansopa C, Frey AM, Farmilo T, Stafford GP. Physiological adaptations of key oral bacteria. Adv Microb Physiol 2014; 65:257-335; PMID:25476768; http://dx.doi.org/10.1002/mbo3.233
- Stafford G, Roy S, Honma K, Sharma A. Sialic acid, periodontal pathogens and Tannerella forsythia: stick around and enjoy the feast! Mol Oral Microbiol 2012; 27:11-22; PMID:22230462; http://dx.doi.org/10.1111/j.2041-1014.2011.00630.x
- Honma K, Mishima E, Sharma A. Role of tannerella forsythia NanH sialidase in epithelial cell attachment. Infect Immun 2011; 79:393-401; PMID:21078857; http://dx.doi.org/10.1128/IAI.00629-10
- Roy S, Honma K, Douglas CW, Sharma A, Stafford GP. Role of sialidase in glycoprotein utilization by tannerella forsythia. Microbiol 2011; 157:31953-202; PMID:21885482; http://dx.doi.org/10.1099/mic.0.052498-0
- Duran-Pinedo AE, Chen T, Teles R, Starr JR, Wang X, Krishnan K, Frias-Lopez J. Community-wide transcriptome of the oral microbiome in subjects with and without periodontitis. ISME J 2014; 8:1659-72; PMID:24599074; http://dx.doi.org/10.1038/ismej.2014.23
- Lühn K, Wild MK. Human deficiencies of fucosylation and sialylation affecting selectin ligands. Semin Immunopathol 2012; 34:383-99; PMID:22461019; http://dx.doi.org/10.1007/s00281-012-0304-1
- Bode L, Jantscher-Krenn E. Structure-function relationships of human milk oligosaccharides. Adv Nutr 2012; 3:383S-91S; PMID:22585916; http://dx.doi.org/10.3945/an.111.001404
- Katayama T, Sakuma A, Kimura T, Makimura Y, Hiratake J, Sakata K, Yamanoi T, Kumagai H, Yamamoto K. Molecular cloning and characterization of bifidobacterium bifidum 1,2-α-L-fucosidase (AfcA), a novel inverting glycosidase (glycoside hydrolase family 95). J Bacteriol 2004; 186:4885-93; PMID:15262925; http://dx.doi.org/10.1128/JB.186.15.4885-4893.2004
- Boren T, Falk P, Roth KA, Larson G, Normark S. Attachment of helicobacter pylori to human gastric epithelium mediated by blood group antigens. Science 1993; 262:1892-5; PMID:8018146; http://dx.doi.org/10.1126/science.8018146
- Ruiz-Palacios GM, Cervantes LE, Ramos P, Chavez-Munguia B, Newburg DS. Campylobacter jejuni binds intestinal H(O) antigen (fuca1, 2Gal b1, 4GlcNAc), and fucosyloligosaccharides of human milk inhibit its binding and infection. J Biol Chem 2003; 278:14112-20; PMID:12562767; http://dx.doi.org/10.1074/jbc.M207744200
- Stahl M, Friis LM, Nothaft H, Liu X, Li J, Szymanski CM, intzi A. L-fucose utilization provides campylobacter jejuni with a competitive advantage. Proc Natl Acad Sci U S A 2011; 108:7194-9; PMID:21482772; http://dx.doi.org/10.1073/pnas.1014125108
- Coyne MJ, Reinap B, Lee MM, Comstock LE. Human symbionts use a host-like pathway for surface fucosylation. Science 2005; 307:1778-81; PMID:15774760; http://dx.doi.org/10.1126/science.1106469
- Veith PD, O'Brien-Simpson NM, Tan Y, Djatmiko DC, Dashper SG, Reynolds EC. Outer membrane proteome and antigens of tannerella forsythia. J Proteome Res 2009; 8:4279-92; PMID:19663511; http://dx.doi.org/10.1021/pr900372c
- Guillotin L, Lafite P, Daniellou R. Unraveling the substrate recognition mechanism and specificity of the unusual glycosyl hydrolase family 29 BT2192 from bacteroides thetaiotaomicron. Biochem 2014; 53:1447-55; PMID:24527659; http://dx.doi.org/10.1021/bi400951q
- Berteau O, McCort I, Goasdoué N, Tissot B, Daniel R. Characterization of a new α-L-fucosidase isolated from the marine mollusk Pecten maximus that catalyzes the hydrolysis of α-L-fucose from algal fucoidan (ascophyllum nodosum). Glycobiol 2002; 12:273-82; PMID:12042250; http://dx.doi.org/10.1093/glycob/12.4.273
- Rodríguez-Diaz J, Monedero V, Yebra MJ. Utilization of natural fucosylated oligosaccharides by three novel α-L-fucosidases from a probiotic lactobacillus casei strain. Appl Environ Microbiol 2011; 77:703-5; PMID:21097595; http://dx.doi.org/10.1128/AEM.01906-10
- Winchester B, Barker C, Baines S, Jacob GS, Namgoong SK, Fleet G. Inhibition of α-L-fucosidase by derivatives of deoxyfuconojirimycin and deoxymannojirimycin. Biochem J 1990; 265:277-82; PMID:2137330
- Hajishengallis G, Lamont RJ. Beyond the red complex and into more complexity: the polymicrobial synergy and dysbiosis (PSD) model of periodontal disease etiology. Mol Oral Microbiol 2012; 27:409-19; PMID:23134607; http://dx.doi.org/10.1111/j.2041-1014.2012.00663.x
- Marsh PD. Are dental diseases examples of ecological catastrophes? Microbiol 2003; 149:279-94; PMID:12624191; http://dx.doi.org/10.1099/mic.0.26082-0
- Marsh PD, Moter A, Devine DA. Dental plaque biofilms: communities, conflict and control. Periodontol 2000 2011; 55:16-35; PMID:21134226; http://dx.doi.org/10.1111/j.1600-0757.2009.00339.x
- Tomek MB, Neumann L, Nimeth I, Koerdt A, Andesner P, Messner P, Mach L, Potempa JS, Schäffer C. The S-layer proteins of tannerella forsythia are secreted via a type IX secretion system that is decoupled from protein O-glycosylation. Mol Oral Microbiol 2014; 29:307-20; PMID:24943676; http://dx.doi.org/10.1111/omi.12062
- Inagaki S, Onishi S, Kuramitsu HK, Sharma A. Porphyromonas gingivalis vesicles enhance attachment, and the leucine-rich repeat BspA protein is required for invasion of epithelial cells by tannerella forsythia. Infect Immun 2006; 74:5023-8; PMID:16926393; http://dx.doi.org/10.1128/IAI.00062-06
- Larsbrink J, Rogers TE, Hemsworth GR, McKee LS, Tauzin AS, Spadiut O, Klinter S, Pudlo NA, Urs K, Koropatkin NM., et al. A discrete genetic locus confers xyloglucan metabolism in select human gut Bacteroidetes. Nature 2014; 506:498-502; PMID:24463512; http://dx.doi.org/10.1038/nature12907
- Martens EC, Koropatkin NM, Smith TJ, Gordon JI. Complex glycan catabolism by the human gut microbiota: the bacteroidetes sus-like paradigm. J Biol Chem 2009; 284:24673-7; PMID:19553672; http://dx.doi.org/10.1074/jbc.R109.022848
- Thompson H, Homer KA, Rao S, Booth V, Hosie AH. An orthologue of bacteroides fragilis NanH is the principal sialidase in tannerella forsythia. J Bacteriol 2009; 191:3623-8; PMID:19304852; http://dx.doi.org/10.1128/JB.01618-08
- Cheng HR, Jiang N. Extremely rapid extraction of DNA from bacteria and yeasts. Biotechnol Lett 2006; 28:55-9; PMID:16369876; http://dx.doi.org/10.1007/s10529-005-4688-z
- Sambrook J, Fritsch EF, Maniatis T. Molecular cloning. New York, Cold Spring Harbor Laboratory Press, Cold Spring Harbor, 1989
- Laemmli UK. Cleavage of structural proteins during the assembly of the head of bacteriophage T4. Nature 1970; 227:680-5; PMID:5432063; http://dx.doi.org/10.1038/227680a0
- Leonard R, Rendic D, Rabouille C, Wilson IB, Preat T, Altmann F. The drosophila fused lobes gene encodes an N-acetylglucosaminidase involved in N-glycan processing. J Biol Chem 2006; 281:4867-75; PMID:16339150; http://dx.doi.org/10.1074/jbc.M511023200
- McIlvaine TA. A buffer solution for colorimetric comparison. J Biol Chem 1921; 49:183-6
- Kurz S, Jin C, Hykollari A, Gregorich D, Giomarelli B, Vasta GR, Wilson IB, Paschinger K. Hemocytes and plasma of the eastern oyster (crassostrea virginica) display a diverse repertoire of sulfated and blood group A-modified N-glycans. J Biol Chem 2013; 288:24410-28; PMID:23824194; http://dx.doi.org/10.1074/jbc.M113.478933
- Bradford MM. A rapid and sensitive method for the quantitation of microgram quantities of protein utilizing the principle of protein-dye binding. Anal Biochem 1976; 72:248-54; PMID:942051; http://dx.doi.org/10.1016/0003-2697(76)90527-3
- Honma K, Mishima E, Inagaki S, Sharma A. The OxyR homologue in tannerella forsythia regulates expression of oxidative stress responses and biofilm formation. Microbiol 2009; 155:1912-22; PMID:19389765; http://dx.doi.org/10.1099/mic.0.027920-0
- Stafford P, Higham J, Pinnock A, Murdoch C, Douglas CW, Stafford GP, Lambert DW. Gingipain-dependent degradation of mammalian target of rapamycin pathway proteins by the periodontal pathogen porphyromonas gingivalis during invasion. Mol Oral Microbiol 2013; 28:366-78; PMID:23714361; http://dx.doi.org/10.1111/omi.12030