Abstract
The environmental opportunistic pathogen Mycobacterium avium subsp hominissuis (MAH), a member of the nontuberculous mycobacteria (NTM) cluster, causes respiratory as well as disseminated disease in patients such as those with chronic respiratory illnesses or AIDS. Currently, there is no effective method to prevent NTM respiratory infections. The formation of mycobacterial microaggregates comprises of phenotypic changes that lead to efficient adherence and invasion of the respiratory mucosa in vitro and in vivo. Microaggregate adhesion to the respiratory epithelium is mediated in part through the mycobacterial protein, MAV_3013 (MBP-1). Through DNA microarray analysis, the small hypothetical gene MAV_0831 (Microaggregate Invasion Protein-1, MIP-1) was identified as being upregulated during microaggregate formation. When MIP-1 was overexpressed in poorly-invasive Mycobacterium smegmatis, it provided the bacterium the ability to bind and enter epithelial cells. In addition, incubating microaggregates with recombinant MIP-1 protein enhanced the ability of microaggregates to invade HEp-2 cells, and exposure to anti-MIP-1 immune serum reduced the invasion of the host epithelium. Through protein-protein interaction assays, MIP-1 was found to bind to the host protein filamin A, a cytoskeletal actin-binding protein integral to the modulation of host cell shape and migration. As visualized by immunofluorescence, filamin A was able to co-localize with microaggregates and to a lesser extent planktonic bacteria. Invasion of HEp-2 cells by microaggregates and planktonic bacteria was also inhibited by the addition of anti-filamin A antibody suggesting that filamin A plays an important role during infection. In addition, at earlier time points binding and invasion assay results suggest that MBP-1 participates significantly during the first interactions with the host cell while MIP-1 becomes important once the bacteria adhere to the host epithelium. In summary, we have unveiled one more step associated with MAH crossing the respiratory mucosa.
Introduction
Mycobacterium avium subspecies hominissuis (MAH) is an opportunistic pathogen commonly found in the environment, such as water and soil and infects various hosts for instance humans and animals such as pigs and birds.Citation1 It has been also identified in hospital water tanks, showerhead biofilms, and hot tubs, which may serve as possible reservoirs of contamination.Citation2-5 MAH commonly infects patients who are immunocompromised, such as those with chronic obstructive pulmonary disease, with AIDS, or individuals with cystic fibrosis. In addition, the frequency of persons with no predisposing illnesses diagnosed with MAH infection is on the rise.Citation1,6 MAH infections are thought to be acquired through the respiratory or gastrointestinal tract often leading to disseminated disease. Nontuberculous mycobacterial (NTM) infections in cystic fibrosis patients are an emerging problem with an overall prevalence ranging from 3.8% to 22.6% of lung infections.Citation6,7 A critical step in the pathogenesis of this infection is the initial adherence and subsequent invasion of the respiratory mucosa.
The formation of bacterial aggregates has been reported to be employed by various pathogens as the initial step to bind and invade the respiratory mucosa.Citation8-14 Aggregation is usually associated with various extracellular bacterial structures such as fimbriae and pili. In addition, other aggregate-inducing proteins have also been described.Citation10-13 Neisseria meningitidis utilizes the protein PilX, a pilus-associated protein to aggregate and attach to the host cell.Citation13 Pseudomonas aeruginosa has been shown to interact with epithelial cells by forming aggregates using the type IV pili.Citation9,15 Conversely, virulent Streptococcus pyogenes microaggregate formation is mediated through homophilic protein-protein interactions between neighboring bacteria and is important for adherence, resistance to phagocytosis and virulence.Citation10 To date, MAH have no known fimbriae or pili that could mediate either adherence or microaggregate formation although pili have been reported in the closely related bacterium Mycobacterium tuberculosis.Citation16
After adhering to the respiratory mucosa, there are several mechanisms in which the bacteria can form microaggregates: bacteria can replicate forming clonal microaggregates or bacteria can secrete extracellular signals to initiate recruitment of bacteria to the site of microaggregate formation.Citation15,17 MAH microaggregates consist of actively recruited planktonic bacteria, although a chemotactic substance or protein responsible for aggregation has not been elucidated.Citation17
Yamazaki et al., Citation2006 and Babrak et alCitation. 2015, examined the initial interaction between MAH and respiratory epithelial cells and identified an invasive phenotype coupled with microaggregate formation when the bacteria were incubated with respiratory BEAS-2B bronchial epithelial cells for 24 hours as well as in vivo in a mouse model.Citation17,18 Subsequently, we genetically characterized the invasive microaggregate phenotype through DNA microarray, identifying highly regulated genes during microaggregate formation.Citation17 The protein, MAV_3013 (MBP-1) was identified as a surface-exposed, small protein, responsible for microaggregate attachment to the host epithelium through the interaction with the host intermediate protein, vimentin. MBP-1 utilizes the host protein vimentin as a surface exposed receptor for microaggregate binding to the epithelial cell wall. Inhibition of MBP-1 significantly inhibited microaggregate binding in vitro and when tested in vivo, the bacterial burden in the mouse lung during infection was significantly reduced.
In this study, we characterized another novel hypothetical gene MAV_0831 that is highly expressed during microaggregate formation. Using an array of molecular and biochemical techniques as well as microscopy, MAV-0831 (microaggregate invasion protein-1) (MIP-1) was shown to be associated with adehesion as well as the invasion of HEp-2 epithelial cells in part through its interaction with the host protein filamin A. In brief, we have gained further insight into bacterial determinants required for efficient invasion of the respiratory mucosa by mycobacterial microaggregates.
Results
In silico characterization of MIP-1
Previously, using DNA microarray, we identified highly regulated genes during microaggregate formation and further characterized the highly expressed, small, hypothetical gene, MBP-1 (MAV_3013).Citation17 In this study, we characterize another highly expressed, small, hypothetical gene, MAV_0831 from that study which we named microaggregate invasion protein-1 (MIP-1).
MIP-1 is a 42 amino acid hypothetical protein that has not been reported in the literature. For the initial analysis of the possible function of the protein, bioinformatic programs were utilized to identify conserved domains or motifs that may suggest a function. Using the Kyoto Encyclopedia of Genes and Genomes (KEGG), a UBA/TS-N domain was identified. The UBA (ubiquitin-associated) domain is novel motif that has some associations with the ubiquitin and ubiquitination pathway while the TS-N domain has been implicated as interacting with the EF-TU protein which is a prokaryotic elongation factor.Citation19,20 This domain encompasses 26/42 amino acids in the central portion of the protein. Currently, there are no paralogs in MAC104 or orthologs in other mycobacteria or other bacteria described.
Functional characterization of MIP-1
In order to determine the function of MIP-1 during microaggregate formation, MIP-1 was overexpressed in Mycobacterium smegmatis mc2155 strain, a fast growing mycobacterium that invades epithelial cells poorly and does not contain MIP-1 in the genome. M. smegmatis containing the plasmid without the protein (Smeg-empty), was used as a control and negates any contribution any endogenous protein would contribute to the assays performed in this study. Although knocking out MIP-1 would be the optimum action to determine the function of the protein, we were unable to construct a knockout due to the technical difficulty of creating a targeted knock out without having polarizing affect on neighboring genes. The ability of M. smegmatis overexpressing MIP-1 (Smeg-0831) to bind and invade epithelial cells was assessed. Smeg-0831 was able to bind significantly better to HEp-2 cells than Smeg-empty (). Likewise, in an invasion assay, Smeg-0831 was able to invade HEp-2 cells significantly better than the Smeg-empty (). To determine which portion of the protein was responsible for the ability of Smeg-0831 to invade, we constructed 4 dominant-negative constructs to assess the various regions of MIP-1, which are depicted in . While all of the dominant-negative proteins reduce some ability of the bacteria to invade epithelial cells, only Smeg-0831Δ1-10 and Smeg-0831Δ12-21 reduce the ability to bind to the epithelial cell at the same level as the control (Smeg-empty) suggesting that the N-terminal portion of this protein is important for invasion ().
Figure 1. Functional characterization of MIP-1 protein (A) Schematic of MIP-1 protein and UBA/TS-N domain (gray). Dashed lines indicate where there has been a deletion in the dominant negative proteins. (B) Mycobacterium smegmatis expressing MAV_0831 (Smeg-0831) was able to bind significantly better than the control. The ability of Smeg-0831 to bind to HEp-2 cells was assessed (n = 3). (C) Smeg-0831Δ1-10 and Smeg-0831Δ12-21 invaded epithelial cells at levels similar to the control. The bacteria were allowed to invade for 3 h at 37°C. MAH microaggregate invasion was used as a positive control (n = 3). (D) Incubation with MIP-1 purified protein significantly increased the ability of MAH microaggregates to invade. MAH microaggregates were incubated with 50 μg of purified MIP-1 for 1 h at 37°C and invasion was assessed. Non-specific protein (Rv3354) and HBSS were used as negative controls. This is one representative with 3 technical replicates of 3 biological replicates. (E) Incubation with anti-MIP-1 immune serum abrogated MAH microaggregate invasion of HEp-2 cells. MAH Microaggregates were incubated with 1:1000 dilution of anti-MIP-1 immune serum for 1 h prior to invasion at 37°C. Pre-immunization serum was used as a negative control. This is one representative with 3 technical replicates of 3 biological replicates. * p < 0.05.
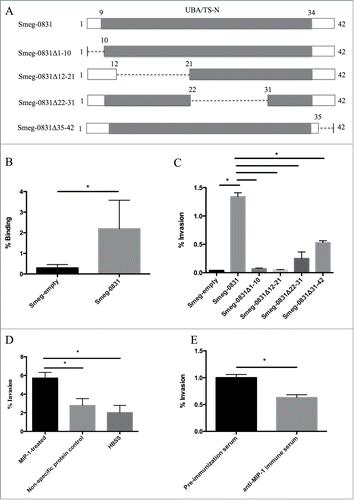
Due to the ability of non-invasive M. smegmatis to gain the ability to enter cells, we hypothesized that MIP-1 may be exported to the surface of the bacterium facilitating invasion of the epithelial cell. To determine whether the expression of MIP-1 on the surface of the bacterium would correlate with the ability to invade, we incubated MAH microaggregates or planktonic bacteria (control) with purified recombinant MIP-1 protein and assessed the ability of the bacteria to invade epithelial cells. Planktonic bacteria consist of MAH incubated in tissue culture media for 24 hours at 37°C in the absence of host cells. After two hours, MIP-1 treated microaggregates were able to invade HEp-2 cells significantly better than microaggregates treated with non-specific protein (Rv3354) or HBSS (). Regardless of their treatment, planktonic bacteria did not show significant differences in the invasion of HEp-2 cells (data not shown). This suggests that MIP-1 is present on the surface of the bacterium during microaggregate formation increasing the ability of microaggregates to invade epithelial cells.
To further investigate the role of MIP-1 during invasion, we produced a specific antibody against MIP-1 protein in mice. We assessed the ability of anti-MIP-1 immune serum to inhibit microaggregate invasion of epithelial cells. Pre-immunization immune serum was used as a control. Microaggregates were incubated with anti-MIP-1 immune serum for 1 h and the invasion of HEp-2 cells was assessed. Incubation with anti-MIP-1 immune serum inhibited approximately 35% of microaggregate invasion of host cells showing the importance of MIP-1 during infection (). To confirm that anti-MIP antibody was specifically inhibiting invasion, total IgGs were purified from the anti-MIP-1 immune serum using protein G affinity chromatography and the concentration was measured. The resulting eluate contained .096 mg of purified antibody and was incubated with microaggregates and invasion was assessed. Purified anti-MIP-1 was also able to significantly inhibit 51% of microaggregate invasion (data not shown).
MIP-1 interacts with host cytoskeletal protein filamin A
Since MIP-1 is involved in the invasion of epithelial cells and may be surface associated, we hypothesized that MIP-1 may directly interact with a surface-associated host protein. To identify MIP-1 host-associated proteins, we utilized 2 methods. Initially, we employed a far-Western blot to identify host binding partners using purified MIP-1 protein, and through mass spectrometry analysis, filamin A was identified as interacting with MIP-1 and the interaction was further confirmed through a pull-down assay ()
Figure 2. MIP-1 interacts with host protein filamin A. (A) Identification of MIP-1 host binding partners by Far-western blot. Lane (1) HEp-1 lysate probed with MIP-1 recombinant protein. Asterisk (*) indicates the position of filamin A on western blot (2) Filamin A recombinant protein (adopted from ) (3) non-specific protein, MAV_3013. *p < 0.05. Lane 2 serves to compare the protein sizes of the far-Western band and filamin A (B) Confirmation of MIP-1-filamin A interaction by pull down assay probed with anti-filamin A antibody. Lane (1) E. coli protein lysate incubated with HEp-2 lysate (negative control) (2) MIP-1 recombinant protein incubated with HEp-2 lysate. Arrow indicates filamin A pull down band (3) HEp-2 lysate (positive control) (C) Anti-filamin A antibody incubation with microaggregates and planktonic bacteria inhibits invasion into HEp-2 cells. Bacteria were incubated with 10 or 50 ug of anti-filamin A antibody or non-specific mouse IgG at the onset of infection. This is one representative with 3 technical replicates of 3 biological replicates. *p < 0.05.
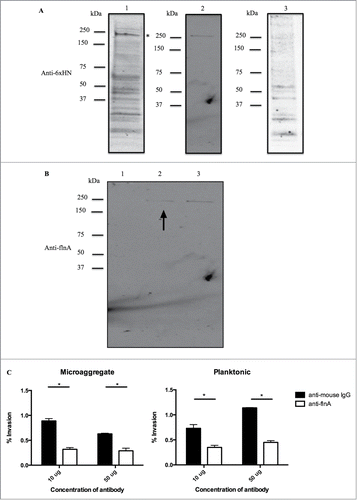
Filamin A has been described as being manipulated for invasion by Ureaplasma parvum during urinary tract infections and by the HIV protein Gag during cell to cell transmission by acting as an adapter protein that links HIV-1 receptors to the actin cytoskeleton remodeling machinery, which facilitates virus infection.Citation21,22 Additionally, filamin A has also been shown to be surface exposed in a variety of host cells such as human neuroblastoma Lan-1 and NMB-7 cell lines and cervical cancer, HeLa cell line and ovarian cancer, SKOV-3 cell line.Citation23 To investigate if filamin A was utilized by microaggregates during infection, we examined if bacterial invasion would be inhibited by blocking surface-associated filamin A. Anti-filamin A antibody was incubated with microaggregates and planktonic bacteria with HEp-2 host cells and invasion was assessed. Incubation with anti-filamin A antibody significantly inhibited invasion of microaggregates and planktonic bacteria, confirming the role of filamin A during invasion (). The data suggest that filamin A plays a non-specific role during invasion or MIP-1 protein may be expressed at lower levels by planktonic bacteria to facilitate invasion. To determine if MIP-1 is expressed during planktonic bacterial infection, real-time PCR was performed. The results indicated that MIP-1 has on average 2.06 × 10ˆ6 mRNA copies/bacterial cell of MIP-1 during planktonic infection explaining why filamin A was able to significantly inhibit the invasion of planktonic bacteria.
To identify if MIP-1 co-localized with filamin A as it has been observed with HIV-Gag protein, by immunofluorescence we examined if MAH microaggregates or planktonic bacteria co-localized with filamin A during infection.Citation22 Filamin A strongly co-localized at the sites of bacterial infection with microaggregates 30% of the time, while planktonic MAH co-localized only 15% of the time further suggesting that filamin A may be used for infection at a lesser extent during planktonic MAH infection ()
Figure 3. Microaggregates and planktonic MAH colocalize with host protein filamin A during infection of Hep-2 cells. Immunofluoresnce microscopy was used to examine the colocalization between filamin A and MAC104 microaggregates and planktonic bacteria. White arrows indicate bacteria (red) and filamin A (green). White bar = 10 μm. (B) Average levels of filamin A colocalization with MAC104 were calculated from the visualization of 10 randomly selected fields for each bacterial phenotype from 2 independent experiments. Percentages shown represent the number of total bacteria associated with filamin A divided by the total number of bacteria.
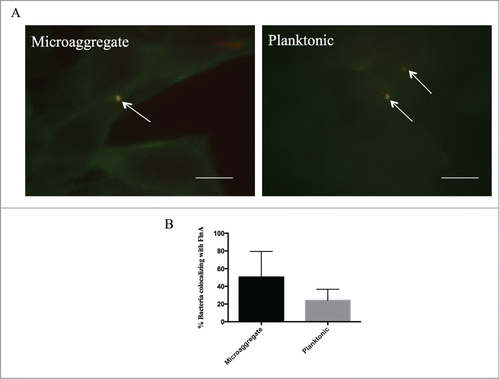
Previously, we identified MBP-1 as overexpressed during microaggregate formation and facilitating microaggregate binding to the host epithelium. In this work, we have characterized MIP-1, which has demonstrated a role during binding and invasion during microaggregate formation. To identify whether MBP-1 and MIP-1 work in conjunction or separately during microaggregate formation, we analyzed binding and invasion efficiencies of M. smegmatis overexpressing these proteins at different time points during infection. M. smegmatis overexpressing MBP-1 (Smeg-3013) was able to bind more efficiently than Smeg-0831 or Smeg-empty at all time points tested. Smeg-3013 was only able to invade more significantly than Smeg-empty and Smeg-0831 at 1 min post-infection (). When compared to results previously published, Smeg-3013 was unable to efficiently invade at 3 h post-infection while Smeg-0831 is able to invade more efficiently at the same time-point suggesting that MBP-1 may play an important early role in microaggregate binding whereas MIP-1 plays a more important role afterwards by facilitating efficient invasion of the respiratory mucosa ().Citation17
Table 1. Early binding and invasion assays for Smeg-3013 and Smeg-0831
Figure 4. Schematic representation of mycobacterial microaggregate formation in the respiratory tract. Mycobacteria are inhaled from aerosolized contaminated material such as water or soil. The bacteria encounter and penetrate past the mucosal layer and where they adhere to the apical surface of the respiratory epithelium, which is facilitated by the MBP-1 protein. Once adherent, the bacteria actively recruit neighboring bacteria and begin to form microaggregates. Facilitated by MIP-1, microaggregates proceed to invade the epithelial cells.
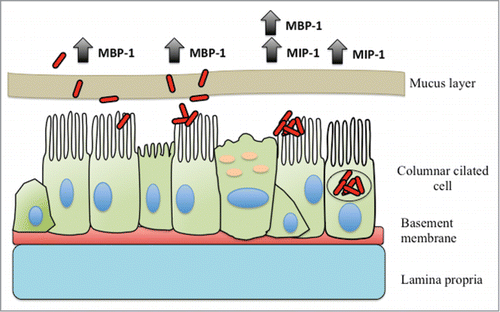
In vivo antibody inhibition assay
The summation of our data suggests that MIP-1 plays a role during microaggregate infection of epithelial cells through the interaction of MIP-1 with the host cell protein filamin A and invasion could be inhibited by anti-MIP-1 antibody immune serum. To test the relevance of MIP-1 in vivo, 2 groups of 10 female C57BL/6 mice were infected with microaggregates that had been incubated with 50 µl of anti-MIP-1 immune serum or pre-immunization serum for 1 h at 37°C. Mice were sacrificed after 24 h and lungs were homogenized and plated to enumerate bacterial colonization. Mice that were infected with microaggregates incubated with anti-MIP-1 immune serum provided significant protection against colonization than microaggregates incubated with pre-immunization serum suggesting that MIP-1 antibody plays an important role during infection in vivo ().
Discussion
As with many pathogenic bacteria, MAH is able to adhere and invade the host respiratory epithelium through an intricate interaction with the host epithelium resulting with efficient invasion and the formation of microaggregates.Citation18 Some evidence suggests that microaggregate formation is a precursor to biofilm formation, which helps explain why NTM respiratory infections have a high rate of reoccurrence and antibiotic resistance. Bacterial aggregates have been found in clinical cases of lung infections.Citation24 In Pseudomonas aeruginosa, bacterial aggregates encased in extracellular matrix have been isolated from the sputum of a cystic fibrosis patient.Citation24 In addition, in previous mycobacterial studies, biofilm mutants were also attenuated in their ability to colonize the respiratory tract in mice illustrating the importance of studying microaggregate formation.Citation18
Our data clearly show that MAH adhere to human epithelial cells, form microaggregates within 24 h and efficiently invade respiratory epithelial cells through the upregulation of MIP-1. The invasive microaggregate phenotype is abrogated by blocking either MIP-1 protein through an anti-MIP-1 immune serum or by inhibiting the MIP-1 host protein receptor, filamin A. Interestingly, there is no complete abrogation of microaggregate invasion indicating that there are other unexplored mechanisms that participate in the invasion by microaggregates. Also, as seen from the far-Western blot, MIP-1 very likely interacts with other host proteins possibly playing other roles during its course of infection perhaps by modulating the ubiquitination pathway. Our studies only focused on the initiation of binding and invasion but it would be worthwhile to investigate other roles MIP-1 plays in microaggregates particularly its' role in the ubiquitination pathway.
While we have shown the importance of MIP-1 expression during microaggregate formation, a knockout mutant of MIP-1 tested in vitro and in vivo would solidly assign a biological significance to these findings. To address this issue, we overexpressed MIP-1 in non-invasive M. smegmatis, which has no known homologs to MIP-1 and assessed for binding and invasion. We also tested the ability of anti-MIP-1 immune serum to inhibit microaggregate invasion during infection in the mouse respiratory infection model. Our data indicate that MIP-1 is a relevant protein expressed in microaggregates that is important during initial colonization of the airways.
The utilization of filamin A by mycobacteria is not unprecedented since it has been reported that MAH utilizes actin polymerization to invade host cells through the activation of Cdc42.Citation25 In addition, there is extensive filopodia-like protrusions, extending from the host cell during invasion resulting from the manipulation of cytoskeletal proteins during microaggregate invasion illustrating the important role cytoskeletal proteins play during microaggregate invasion.Citation17
Microaggregate formation is a complex and multifactorial mechanism that employs many proteins to accomplish the bacterial goal of invasion and survival. We have established a model that characterizes the transition from planktonic to host cell-associated, which is integral to respiratory infection. First, a single bacterium attaches to the surface of the host mediated through the surface-exposed mycobacterial protein, MBP-1 using the host protein vimentin as a adhesion receptor. This is followed by relatively rapid recruitment of planktonic bacteria and resulting in the formation of microaggregates within 24 h. We have preliminary evidence to suggest that this rapid recruitment may be mediated by MAV_1799, which is also highly upregulated during microaggregate formation (unpublished data). Escherichia coli and Samonella typhimurinum have been shown to excrete chemotactic molecules that attract other bacteria leading to the formation of clusters.Citation26,27 Alternatively, this protein may stimulate the host cell to secrete a chemotactic substance that results in the attraction of other bacteria. Once the microaggregate is formed on the surface of the cell, it is able to efficiently invade the host epithelium through MIP-1 by further manipulating the host cytoskeleton through interaction with filamin A.
In conclusion, our data indicate that MAH is able to adhere, form microaggregates, and subsequently invade epithelial cells through the utilization of the MIP-1 protein. We hypothesize that the ability to form microaggregates may also offer MAH an advantage to counteract host immune defenses but further studies are necessary. The characterization of MIP-1 offers an opportunity to uncover novel anti-mycobacterial therapies that can target virulence factors.
Methods and Materials
Bacterial cultures
Mycobacterium avium strain 104 (serovar 1) was isolated from the blood of an AIDS patient. Mycobacterium smegmatis mc2 155 was kindly provided by W. Jacobs, Jr (Albert Einstein School of Medicine, Bronx, NY). Mycobacterial strains were cultured on Middlebrook 7H10 supplemented with 10% oleic acid, albumin, dextrose, and catalase to log-phase at 37°C (Hardy Diagnostics, Santa Maria, CA). As previously described in,Citation17 prior to assays, the bacteria were passed through a 20-gauge needle 5 times to break cell aggregates and the obtained bacterial suspension was allowed to rest for 2 min and only the top 1 ml was ultimately used. An optical density of 0.084 at 600 nm (7 × 10ˆ7 CFU/ml) was used to quantify bacterial concentrations.
Cell culture
Human laryngeal epithelial cell line, HEp-2 cells (CCL-23) were obtained from the American Type Culture Collection (Manassas, VA) and grown in in RPMI-1640 (Lonza, Allendale, NJ) supplemented with 10% fetal bovine serum (FBS), (Gemini Products, Sacramento CA) at 37°C and with 5% CO2. For all of the assays described, epithelial cells were used at 80% confluence. HEp-2 cells are an adequate representation for epithelial cells.
Microscopy
For immunofluorescence microscopy studies, HEp-2 cells were seeded and infected in 8-well chamber slides at an MOI of 10. The infected monolayer was washed and fixed in ice-cold methanol for 15 min at −20°C. Monolayers were then blocked with 2% skim milk powder and incubated with an antibody overnight at 4°C, washed and subsequently incubated with the appropriate secondary antibody for 1 h. A Leica DM4000B microscope was used and all images were acquired with the same optimized parameters for all experiments. For immunofluorescence work, studies were repeated at least 3 times and over 10 fields of view were captured for each condition and >50 bacteria counted.
Formation and isolation of microaggregates
The protocol for the formation and isolation of microaggregates is described in greater detail in.Citation17 Briefly, HEp-2 cells were grown to confluence in T75 flasks (BD Biosciences, San Jose, CA) and then treated with 5 ug/ml of cytochalasin B (Sigma, St. Louis, MO) for 2 h. The monolayer was then infected with a single cell suspension of MAH, as described above, at an MOI of 100 and incubated with the HEp-2 cells for 24 h at 37°C and with 5% CO2. As a planktonic bacterial control, a single cell suspension of MAH was also incubated with cell culture media in the absence of HEp-2 cells. After 24 h, the supernatant was recovered and immediately placed on ice. Additionally, HEp-2 cells were washed 3 times with ice-cold HBSS to obtain the remaining microaggregates. The microaggregate suspension was then observed under a microscope for confirmation of the structure. Differential centrifugation was used to remove any contaminating HEp-2 cells and the resulting bacterial pellet was resuspended in 2 ml of HBSS and the bacterial concentration was determined using optical density. To establish colony forming units (CFU), the bacterial inoculum was serially diluted and plated onto Middlebrook 7H10 agar plates.
Antibodies
We used the following antibodies for the assays in this study: filamin A, clone PM6/317 (MAB1678) (EMD Millepore, Billerica, MA), his-probe antibody (H-15) (Santa Cruz Biotechnology, Dallas, TX), 6XHN polyclonal antibody (Clontech, Mountain View, CA), or non-specific mouse IgG antibody (Santa Crux Biotechnology).
Overexpression & purification of MIP-1
To create the MIP-1 wildtype, and dominant-negative overexpression constructs, genes were amplified using FideliTaq PCR Master Mix (Affymetrix, Santa Clara, CA) with the following primers: Smeg-0831: 5′-TTTTTAAGCTTCCGACGAAGACGAATGGC-3′ and 5′-TTTTTAAGCTTTCACTCATAGCGTGCGTC-3′; Smeg-0831Δ1-10 5′-TTTTAAGCTTTGGCCGACATGTCC-3′ and 5′- GACGCACGCTATGAGTGAGTTAACAAAA-3′; Smeg-0831 Δ12-21: 5′-TTTTAAGCTTTGACCGACGAAGACG-3′, 5′-CCGCGCGGTATTATGCGGCG-3′, 5′-CGTATTCCGTCACCGCGGCG-3′ and 5′-GACGCACGCTATGAGTGAGTTAACAAAA-3′; Smeg-0831Δ22-31: 5′-TTTTAAGCTTTGACCGACGAAGACG-3′,
5′-CCGCGCGGTATTATGCGGCG-3′, 5′-CGCCGCATAATACCGCGCGG-3′ and 5′-GACGCACGCTATGAGTGAGTTAACAAAA-3′; Smeg-0831Δ31-42 5′-TTTTAAGCTTTGGCCGACATGTCC-3′ and 5′-TTTTTAAGCTTTCAGCCGCATAAAC-3′.
The PCR-generated fragments were fused to a red fluorescent protein (RFP) and cloned into Escherichia coli-Mycobacterium shuttle vector pMV261-5HRFP encoding kanamycin resistance.Citation28 Splicing by Overlapping Extension PCR was utilized to create Smeg-0831Δ12-21 and Smeg-0831Δ22-31. The resulting plasmid was propagated in DH10B E. coli and then electroporated into Mycobacterium smegmatis mc2155. Transformants were selected on Middlebrook 7H10 agar plates containing 50 μg/ml of kanamycin and screened by PCR using primers originally used to PCR the fragment.
MIP-1 protein was expressed and purified using pET Express & Purify Kit-His60 (Clontech, Mountain View, CA) and purified using His60 Ni Gravity Column Purification kit (Clontech) as per manufacturers instructions. Overexpression of genes in M. smegmatis and purification of proteins was confirmed by Western blot using the anti-his probe and anti-6XHN antibody, respectively.
Real-time PCR
MIP-1 mRNA copy number was determined using quantitative real-time PCR of the RNA used in the initial microarray performed in,Citation17 with the following primers: 5′ -GTGACCGACGAAGACGAAT- 3′ and 5′-CACTCATAGCGTGCGTCCT-3′. A Biorad iCycler and SYBR green technology was used (Biorad, Hercules, CA), as previously described.Citation28 Briefly, bacterial RNA was transcribed to cDNA using SuperScript III Supermix (Invitrogen, Carlsbad, CA), according to manufacturer protocol. Afterwards, cDNA was denatured for 5 minutes at 95°C, followed by 40 cycles of amplification. Each cycle had a denaturation step of 95°C for 30 seconds, annealing at 60°C for 30 seconds, and primer extension at 68°C for 1 minute. To determine copy number, a standard curve and equation of the line was created using spiked and known amounts of genomic MAC104 DNA and then calculated using N = (Ct− b)/m, where N is copy number, Ct is the threshold cycle, b is the y-intercept, and m is the slope of the standard curve line. Results represent the average of 3 technical replicates and are representative of 3 biological replicates.
Production and purification of MIP-1 antibody
As previously described,Citation17 the production of the MIP-1 antibody was adapted from Thermoscientific Mouse Antibody Production Protocols (http://www.pierce-antibodies.com/custom-antibodies/mouse-antibody-production-protocols.cfm) with the following modifications. Briefly, MIP-1 protein was produced and purified as described above. Ten female C57BL/6 mice were immunized with 3 subcutaneous injections of 0.1 mg MIP-1 protein with incomplete Freund's adjuvant as per manufacturers instructions (Sigma). Boosters were given 21 and 42 days afterwards with one injection of 0.05 µg MIP-1 protein. Blood was collected at 50 days after initial injection. Blood was centrifuged at 1,000 × g for 10 min and the resulting immune serum was collected and used for experiments. Pre-immunized serum was also obtained from mice and used as experimental control. The serum was tested against MIP-1 protein at 1:5,000 dilution by Western blot to verify specificity (data not shown). Pre-immunization immune serum was also examined for reactivity against MIP-1 protein. In addition, mouse anti-MIP-1 antibody and pre-immunization immune serum were purified on Protein G HP Spintrap (Thermoscienfitic). A Bradford protein assay (Biorad) was used to determine the concentration of the purified antibody. This study was performed according to the guidelines of and approved by the institutional animal care and use committee at Oregon State University (Animal Care and Use Proposal 4490).
Invasion assay
The invasion assays were carried out as previously reported.Citation29 Briefly, HEp-2 cells were infected with M. smegmatis overexpressing a protein of interest at an MOI of 100 for 3 h at 37°C and the infection was synchronized by centrifugation for 5 min at 232 × g after the addition of bacteria. Wells were then washed 3 times with HBSS and incubated with 200 μg/ml amikacin in media for 2 h to kill extracellular bacteria and subsequently lysed with 0.1% triton X-100 for 15 min. Cell lysates were plated onto Middlebrook 7H10 agar plates supplemented with 50 μg/ml kanamycin. After growth, CFUs were quantified.
For filamin A antibody or anti-MIP-1 immune serum inhibition invasion assay, microaggregates and planktonic MAH were isolated as described above and respectively, microaggregates and planktonic MAH were incubated with either 50 μg of mouse anti-filamin A antibody or non-specific mouse IgG antibody (control) or 1:1000 dilution of anti-MIP-1 immune serum or pre-immunization serum (control) for 1 h at 37°C. HEp-2 cells were infected at an MOI of 10. The infection proceeded as described in the first invasion assay.
For MIP-1-treated microaggregate invasion assay, microaggregates were isolated as described previously and incubated with 50 μg of purified MIP-1 or non-specific protein for 1 h at room temperature at an MOI of 10. Microaggregates were then washed 3 times with HBSS and the infection proceeded as described in the invasion assay above.
For M. smegmatis overexpression binding and invasion time-series assay, Smeg-3013 and Smeg-0831 were incubated with HEp-2 cells at an MOI of 100 for 1, 5, and 15 min at 4°C for the binding assay, and for the invasion assay, plates were incubated for 1, 5, and 15 min at 37°C (giving the media 10 min to warm up before beginning the invasion time). Also, instead of an amikacin treatment for the invasion assay, wells were washed thoroughly 3 times with HBSS to remove extracellular bacteria as described in Citation30. Smeg-empty was used as a control.
Western blots
For the Far-Western blot, HEp-2 cells were grown in T75 flask and washed twice with HBSS. HEp-2 cells were then lysed with CelLytic M (Sigma) in the presence of protease inhibitor cocktail (Sigma), scraped off the flask, and centrifuged for 15 min at 12,000 × g. HEp-2 cell lysate was run on 4-20% Mini-PROTEAN precast SDS-PAGE protein gel (Biorad, Hercules, CA) under reducing conditions and transferred to a nitrocellulose membrane (Biorad). After 1 h in 5% blocking buffer, the membrane was further incubated with 2 mg/ml of MIP-1 or non-specific protein, MAV_3013 (control) in protein binding buffer (100 mM NaCl, 20 mM Tris (pH 7.6), 0.5 mM EDTA, 10% glycerol, 0.1% tween-20, 2% skim milk powder, 1 mM DTT) overnight at 4°C with gentle shaking. Bound recombinant protein was detected with 6xHN polyclonal antibody (Clontech, Mountain View, CA) followed by the corresponding Li-Cor IR Dye secondary antibody. The host protein that interacted with MIP-1 the strongest was subsequently excised from protein gel using Pierce In-gel Tryptic Digestion kit (Rockford, IL), and identified by mass spectrometry at the Mass Spectrometry Facility at Oregon State University. HEp-2 lysate was run on a SDS-Page gel and probed with anti-filamin A antibody demonstrate that the band in the far-Western blot and filamin A were a similar size.
Pull down assay
MIP-1 protein (bait) was overexpressed and washed extensively using pET Express & Purify Kit-His60 column, isolating only MIP-1 protein on the column. A confluent T75 flask of HEp-2 cells (prey) were washed twice with chilled HBSS and incubated with 100 μl of CelLytic Lysis Buffer (Sigma) with protease inhibitor cocktail (Sigma) and immediately scraped out of the well and put on ice. One mg of HEp-2 lysate was incubated in the Ni column containing MIP-1 protein overnight at 4°C with rotation. After 24 h, the protein mix was passed through the Clontech Ni-resin column. The column was washed with 5 ml of equilibration buffer followed by 5 ml of wash buffer and eluted in 5 ml of elution buffer. The eluate was concentrated to 100 μl using Microsep centrifugal device (Pall, Port Washington, NY). Proteins were run on SDS-page gel and blotted onto a nitrocellulose membrane. The membrane was probed with an anti-filamin A antibody as well as a anti-6xHN antibody. HEp-2 cell lysate incubated with E. coli lysate was used as a negative control.
Mouse study
Female C57BL/6 mice, 6 weeks old, were infected intranasally with 20 µl of 2×1010 cfu per ml of microaggregates incubated with 50 µl anti-MIP-1 immune serum or pre-immunization serum (control) for 1 h at 37°C. After 24 h, the mice were sacrificed and lungs were homogenized and plated onto Middlebrook 7H10 agar plates for determination of colony counts. Ten mice were used in each experimental group. This study was performed according to the guidelines of and approved by the institutional animal care and use committee at Oregon State University (Animal Care and Use Proposal 4396).
Statistical Analysis
Experiments in this paper were repeated at least 2 times and data shown are means of those replicates ± standard error. When comparing 2 groups, the Student's t-test was used and when analyzing more than 2 groups, an ANOVA analysis was carried out. Differences between experimental groups and control were considered significant when p < 0.05. Graph Pad Prism version 5.0 software was used for statistical analysis.
Disclosure of Potential Conflicts of Interest
No potential conflicts of interest were disclosed.
Funding
This work was supported by grant AI 043199 from the National Institutes of Health and from the Microbiology Foundation, of San Francisco, CA.
References
- Falkinham JO. The changing pattern of nontuberculous mycobacterial disease. Can J Infect Dis 2003; 14:281-6; PMID:18159470
- Covert TC, Rodgers MR, Reyes AL, Stelma GN, Jr. Occurrence of nontuberculous mycobacteria in environmental samples. Appl Environ Microbiol 1999; 65:2492-6; PMID:10347032
- Falkinham JO, 3rd, Norton CD, LeChevallier MW. Factors influencing numbers of Mycobacterium avium, Mycobacterium intracellulare, and other Mycobacteria in drinking water distribution systems. Appl Environ Microbiol 2001; 67:1225-31; PMID:11229914; http://dx.doi.org/10.1128/AEM.67.3.1225-1231.2001
- Feazel LM, Baumgartner LK, Peterson KL, Frank DN, Harris JK, Pace NR. Opportunistic pathogens enriched in showerhead biofilms. Proc Natl Acad Sci USA 2009; 106:16393-9; PMID:19805310; http://dx.doi.org/10.1073/pnas.0908446106
- Lumb R, Stapledon R, Scroop A, Bond P, Cunliffe D, Goodwin A, Doyle R, Bastian I. Investigation of spa pools associated with lung disorders caused by Mycobacterium avium complex in immunocompetent adults. Appl Environ Microbiol 2004; 70:4906-10; PMID:15294830; http://dx.doi.org/10.1128/AEM.70.8.4906-4910.2004
- Mirsaeidi M, Farshidpour M, Allen MB, Ebrahimi G, Falkinham JO. Highlight on Advances in Nontuberculous Mycobacterial Disease in North America. Biomed Res Int 2014; 2014:919474; PMID:25574470; http://dx.doi.org/10.1155/2014/919474
- Levy I, Grisaru-Soen G, Lerner-Geva L, Kerem E, Blau H, Bentur L, Aviram M, Rivlin J, Picard E, Lavy A, et al. Multicenter cross-sectional study of nontuberculous mycobacterial infections among cystic fibrosis patients, Israel. Emerg Infect Dis 2008; 14:378-84; PMID:18325250; http://dx.doi.org/10.3201/eid1403.061405
- Chi F, Jong TD, Wang L, Ouyang Y, Wu C, Li W, Huang SH. Vimentin-mediated signalling is required for IbeA+ E. coli K1 invasion of human brain microvascular endothelial cells. Biochem J 2010; 427:79-90; PMID:20088823; http://dx.doi.org/10.1042/BJ20091097
- Lepanto P, Bryant DM, Rossello J, Datta A, Mostov KE, Kierbel A. Pseudomonas aeruginosa interacts with epithelial cells rapidly forming aggregates that are internalized by a Lyn-dependent mechanism. Cell Microbiol 2011; 13:1212-22; PMID:21615664; http://dx.doi.org/10.1111/j.1462-5822.2011.01611.x
- Frick IM, Morgelin M, Bjorck L. Virulent aggregates of Streptococcus pyogenes are generated by homophilic protein-protein interactions. Mol Microbiol 2000; 37:1232-47; PMID:10972839; http://dx.doi.org/10.1046/j.1365-2958.2000.02084.x
- Sherlock O, Schembri MA, Reisner A, Klemm P. Novel roles for the AIDA adhesin from diarrheagenic Escherichia coli: cell aggregation and biofilm formation. J Bacteriol 2004; 186:8058-65; PMID:15547278; http://dx.doi.org/10.1128/JB.186.23.8058-8065.2004
- Menozzi FD, Boucher PE, Riveau G, Gantiez C, Locht C. Surface-associated filamentous hemagglutinin induces autoagglutination of Bordetella pertussis. Infect Immun 1994; 62:4261-9; PMID:7927683
- Helaine S, Carbonnelle E, Prouvensier L, Beretti JL, Nassif X, Pelicic V. PilX, a pilus-associated protein essential for bacterial aggregation, is a key to pilus-facilitated attachment of Neisseria meningitidis to human cells. Mol Microbiol 2005; 55:65-77; PMID:15612917; http://dx.doi.org/10.1111/j.1365-2958.2004.04372.x
- Barnes AM, Ballering KS, Leibman RS, Wells CL, Dunny GM. Enterococcus faecalis produces abundant extracellular structures containing DNA in the absence of cell lysis during early biofilm formation. MBio 2012; 3:e00193-12; PMID:22829679; http://dx.doi.org/10.1128/mBio.00193-12
- Bucior I, Pielage JF, Engel JN. Pseudomonas aeruginosa pili and flagella mediate distinct binding and signaling events at the apical and basolateral surface of airway epithelium. PLoS Pathogens 2012; 8:e1002616; PMID:22496644; http://dx.doi.org/10.1371/journal.ppat.1002616
- Alteri CJ, Xicohtencatl-Cortes J, Hess S, Caballero-Olin G, Giron JA, Friedman RL. Mycobacterium tuberculosis produces pili during human infection. Proc Natl Acad Sci USA 2007; 104:5145-50; PMID:17360408; http://dx.doi.org/10.1073/pnas.0602304104
- Babrak L, Danelishvili L, Rose SJ, Kornberg T, Bermudez LE. The Environment of “Mycobacterium avium subsp hominissuis” Microaggregates Induces Synthesis of Small Proteins Associated with Efficient Infection of Respiratory Epithelial Cells. Infect Immun 2015; 83:625-36; PMID:25422262; http://dx.doi.org/10.1128/IAI.02699-14
- Yamazaki Y, Danelishvili L, Wu M, Hidaka E, Katsuyama T, Stang B, Petrofsky M, Bildfell R, Bermudez LE. The ability to form biofilm influences Mycobacterium avium invasion and translocation of bronchial epithelial cells. Cell Microbiol 2006; 8:806-14; PMID:16611229; http://dx.doi.org/10.1111/j.1462-5822.2005.00667.x
- Wilkinson CR, Seeger M, Hartmann-Petersen R, Stone M, Wallace M, Semple C, Gordon C. Proteins containing the UBA domain are able to bind to multi-ubiquitin chains. Nat Cell Biol 2001; 3:939-43; PMID:11584278; http://dx.doi.org/10.1038/ncb1001-939
- Makarova KS, Aravind L, Galperin MY, Grishin NV, Tatusov RL, Wolf YI, Koonin EV. Comparative genomics of the Archaea (Euryarchaeota): evolution of conserved protein families, the stable core, and the variable shell. Genome Res 1999; 9:608-28; PMID:10413400
- Allam AB, Alvarez S, Brown MB, Reyes L. Ureaplasma parvum infection alters filamin A dynamics in host cells. BMC Infect Dis 2011; 11:101; PMID:21507248; http://dx.doi.org/10.1186/1471-2334-11-101
- Jimenez-Baranda S, Gomez-Mouton C, Rojas A, Martinez-Prats L, Mira E, Ana Lacalle R, Valencia A, Dimitrov DS, Viola A, Delgado R, et al. Filamin-A regulates actin-dependent clustering of HIV receptors. Nat Cell Biol 2007; 9:838-46; PMID:17572668; http://dx.doi.org/10.1038/ncb1610
- Bachmann AS, Howard JP, Vogel CW. Actin-binding protein filamin A is displayed on the surface of human neuroblastoma cells. Cancer Sci 2006; 97:1359-65; PMID:16999820; http://dx.doi.org/10.1111/j.1349-7006.2006.00327.x
- Parsek MR, Singh PK. Bacterial biofilms: an emerging link to disease pathogenesis. Ann Rev Microbiol 2003; 57:677-701; PMID:14527295; http://dx.doi.org/10.1146/annurev.micro.57.030502.090720
- Harriff MJ, Wu M, Kent ML, Bermudez LE. Species of environmental mycobacteria differ in their abilities to grow in human, mouse, and carp macrophages and with regard to the presence of mycobacterial virulence genes, as observed by DNA microarray hybridization. Appl Environ Microbiol 2008; 74:275-85; PMID:17981953; http://dx.doi.org/10.1128/AEM.01480-07
- Budrene EO, Berg HC. Complex patterns formed by motile cells of Escherichia coli. Nat 1991; 349:630-3; PMID:2000137; http://dx.doi.org/10.1038/349630a0
- Budrene EO, Berg HC. Dynamics of formation of symmetrical patterns by chemotactic bacteria. Nat 1995; 376:49-53; PMID:7596432; http://dx.doi.org/10.1038/376049a0
- Danelishvili L, Poort MJ, Bermudez LE. Identification of Mycobacterium avium genes up-regulated in cultured macrophages and in mice. FEMS Microbiol Letters 2004; 239:41-9; PMID:15451099; http://dx.doi.org/10.1016/j.femsle.2004.08.014
- Bermudez LE, Young LS. Factors affecting invasion of HT-29 and HEp-2 epithelial cells by organisms of the Mycobacterium avium complex. Infect Immun 1994; 62:2021-6; PMID:8168968
- Early J, Bermudez LE. Mimicry of the pathogenic mycobacterium vacuole in vitro elicits the bacterial intracellular phenotype, including early-onset macrophage death. Infect Immun 2011; 79:2412-22; PMID:21444666; http://dx.doi.org/10.1128/IAI.01120-10