ABSTRACT
Anthrax toxins and capsule are the major virulence factors of Bacillus anthracis. They are encoded by genes located on the plasmids pXO1 and pXO2, respectively. The vaccine strain Pasteur II was produced from high temperature subcultures of B. anthracis, which resulted in virulence attenuation through the loss of the plasmid pXO1. However, it is unclear whether the high temperature culture completely abolishes the plasmid DNA or affects the replication of the plasmid pXO1. In this study, we tested 3 B. anthracis vaccine strains, including Pasteur II from France, Qiankefusiji II from Russia, and Rentian II from Japan, which were all generated from subcultures at high temperatures. Surprisingly, we detected the presence of pXO1 plasmid DNA using overlap PCR in all these vaccine strains. DNA sequencing analysis of overlap PCR products further confirmed the presence of pXO1. Moreover, the expression of the protective antigen (PA) encoded on pXO1 was determined by using SDS-PAGE and western blotting. In addition, we mimicked Pasteur's method and exposed the A16R vaccine strain, which lacks the pXO2 plasmid, to high temperature, and identified the pXO1 plasmid in the subcultures at high temperatures. This indicated that the high temperature treatment at 42.5°C was unable to eliminate pXO1 plasmid DNA from B. anthracis. Our results suggest that the attenuation of the Pasteur II vaccine strain is likely due to the impact of high temperature stress on plasmid replication, which in turn limits the copy number of pXO1. Our data provide new insights into the mechanisms of the remaining immunogenicity and toxicity of the vaccine strains.
Introduction
Bacillus anthracis causes anthrax in both animals and humans. Anthrax has 3 clinical manifestations depending on the route of infection, including cutaneous anthrax, pulmonary anthrax, and intestinal anthrax.Citation1 The prevention of B. anthracis transmission among animals plays an important role in controlling anthrax worldwide.
The concept of anthrax vaccine immunization was first raised in 1880.Citation2 A year later, an immunologic trial was successfully conducted by a French scientist, Louis Pasteur.Citation2 After inoculating and incubating B. anthracis in a fluid medium at 42-43°C, Pasteur collected the bacterial cells on the 12th day (Pasteur II) and the 24th day (Pasteur I). The bacterial cells, which were designated as low virulent Pasteur I and Pasteur II strains, were utilized for the immunization of sheep and provided protection following challenge with a highly virulent strain.Citation2 Thus this anthrax vaccine became widely accepted and used for the immunization of livestock in Europe and other countries. Meanwhile, other investigators generated different attenuated anthrax vaccine strains using Pasteur's method and applied them in their own countries, such as the previously reported vaccine Qiankefusiji II (Russia)Citation3 and Rentian II (Japan).Citation4 In 1939, the Sterne vaccine strain replaced the Pasteur vaccine strain due to its lower toxicity and better immunogenicity.Citation5
Despite the success of Pasteur's live vaccine in the prevention of anthrax, the mechanism that produced avirulence was not understood until a century later. It was found that the high temperature treatment of B. anthracis using Pasteur's method at 42.5°C led to the loss of the expression of edema factor (EF) and lethal factor (LF), consequently attenuating virulence. It was then revealed that the Pasteur's standard vaccine strains (Pasteur I and Pasteur II) lacked a 110-Mbp plasmid DNA band in agarose gel after electrophoresis.Citation6 These studies demonstrated that the culturing of virulent B. anthracis in vitro under high temperature caused the loss of the large plasmid encoding the anthrax toxin. It is known that the protective antigen (PA), EF, and LF are encoded by genes located on the pXO1 plasmid of B. anthracis.Citation7-8 Therefore, the attenuation of Pasteur II was attributed to the loss of pXO1 (pXO1−/pXO2+), and likewise for the vaccine strains Qiankefusiji II and Rentian II, which were all produced by similar heat treatment methods. However, residual virulence was observed in these attenuated live spore vaccines.Citation9 After ruling out contamination, some pXO1 elements were detected in the Pasteur II vaccine strain when we conducted PCR analysis, and no natural B. anthracis strain lacking pXO1 was found in the environment in the Chinese surveillance network.Citation10 These facts indicate that the natural curing of pXO1 does not occur in the environment and that there are problems with residual toxicity of the vaccine strains.
It is well established that the virulence of Bacillus anthracis is plasmid determined, with anthrax toxin genes located on pXO1 and capsule genes on a separate pXO2 plasmid. Both plasmids are required for virulence; strains without pXO1 are avirulent because they do not produce the anthrax toxins, whereas strains lacking pXO2, such as the Sterne vaccine strain, are greatly attenuated because they do not have the capsule.Citation11-12 pXO1 plasmid-encoded virulence factors are important in the pathogenesis of diseases caused by Bacillus anthracis. pXO1 has 203 ORFs including the structural genes for all 3 components of the anthrax toxin. These toxin genes are located on a 44.8-kb pathogenicity island.Citation13 The toxic components are EF, a calmodulin-dependent adenylyl cyclase, and LF. Toxin and capsule production, and therefore virulence, is regulated by AtxA, which is encoded by atxA in the pathogenicity island on pXO1. The pXO1 pathogenicity island also encodes an S-layer adhesin, BslA, which is essential for the adherence of B. anthracis cells to human cells.Citation14-18 Apart from its involvement in the pathogenesis of B. anthracis as a toxic component, PA is a major antigen that can induce protective immunity against B. anthracis infection.Citation19
Considering that the virulence and protective functions of B. anthracis are mainly provided by the plasmid pXO1, and the fact that the pXO1-deficient Pasteur II vaccine strain still possesses antigenicity and toxicity, we questioned whether the antigenicity and mild toxicity of Pasteur II was indeed due to the lack of the pXO1, or whether its antigenicity was imparted by the PA produced by pXO1. Consequently, we examined 3 different B. anthracis vaccine strains, including Pasteur II, Qiankefusiji II, and Rentian II, all of which were generated from subcultures at high temperatures. Surprisingly, our investigations demonstrated that the pXO1 plasmid was present in all 3 vaccine strains.
Materials and methods
Bacterial vaccine strains
B. anthracis vaccine strain Pasteur II was originally generated at the Pasteur Institute in France in 1934. It was introduced into the National Institute of Northwest Epidemic Disease Control and Prevention in China, which was renamed as the Institute of Lanzhou Biological Products in 1935, by Professor Shoushen Yang who was a visiting scholar at the Pasteur Institute in 1934. B. anthracis vaccine strain Qiankefusiji II was attenuated through high temperature passage by Qiankefusiji in Russia. It has been used as a livestock vaccine in Russia, and was introduced into the National Institute of Food and Drug Control in China in 1956. The B. anthracis vaccine strain II (Rentian II) was attenuated by Rentian in Japan using high temperature passage and was introduced into the Harbin Veterinary Research Institute, Chinese Academy of Agricultural Sciences. It was used as a livestock vaccine in China. B. anthracis vaccine strains, including Pasteur II, Qiankefusiji II, and Rentian II, were all believed to have lost the pXO1 plasmid. B. anthracis vaccine strain A16R was provided by a B. anthracis research reference laboratory in the Institute of Lanzhou Biological Products. B. anthracis vaccine strain II (Rentian II) was supplied by Xinjiang Tiankang Livestock Biotechnology Co., Ltd. The vaccine strain A16R is a noncapsular B. anthracis, which was generated through ultraviolet irradiation by Shuya Yang in 1958 and contains the pXO1 plasmid. It was used for human vaccination in China. A16R acted as a reference strain for pXO1 presence and pXO2 absence (pXO1+/pXO2−).
Identification of B. anthracis strains
The B. anthracis vaccine strains Pasteur II and Qiankefusiji II were stored in freeze-dried milk in the Institute of Lanzhou Biological Products in 1972. B. anthracis vaccine II (Rentian II) was provided as spore seedlings by a factory. All four vaccine strains were recovered in LB medium and detected using conventional identification methods, including colony morphology, biochemical analysis such as glucose, sucrose, maltose, mannitol, salicin, and arabinose fermentation tests, V-P test, H2S production test (BioMerieux, Leone, France. See Table S1), phage AP631 (gamma phage derivative) susceptibility test (the biological characteristics of phage AP631, the anthrax diagnostic phage used in China, was studied.Citation20), penicillin inhibition test, beaded test, SDS-PAGE electrophoresis, and anthrax precipitin serum gel diffusion.
Pulsed-field gel electrophoresis
The plasmids of Pasteur II, Qiankefusiji II, Rentian II, and A16R were analyzed with pulsed-field gel electrophoresis (PFGE) as previously described.Citation21 Briefly, the bacterial pellet was suspended in 2 ml of TE buffer (50 mM Tris, pH 7.5, 20 mM EDTA) and the concentration was adjusted to 5.0 McIntosh units. In an Eppendorf tube, 300 μl of bacterial suspension was gently mixed with 6 μl of lysozyme (100mg/ml) and incubated at 37°C for 30 min. Then, 15 μl of proteinase K(20mg/ml) was added, followed by immediately adding an equal volume of 1.6% low-melt, preparative-grade agarose (Bio-Rad, Hercules, Calif.). Next, the mixture was distributed into a mold (200 μl/well) and the solidified agarose was incubated in a lysis solution containing lysozyme and proteinase K at 37°C in a water bath with shaking for 5 h. Subsequently, the agarose was washed once and added to a lysis buffer containing ribonuclease S1 at 23°C for 15 min. The agarose embedded with DNAs were loaded into wells and PFGE was performed using a CHEF Mapper apparatus (Bio-Rad) with a 120° field angle and ran for 18 h at 6 V/cm.
Overlap PCR
To detect the presence of plasmid DNA, we performed overlap PCR. The overlap PCR primers were designed and synthesized as previously described.Citation22 Briefly, according to the DNA sequence of pXO1 of the Ames Ancestor strain in GenBank, 3000∼4000 bp of PCR product was designed as an amplified fragment. The amplicons have 300∼400 bp overlap regions between each 2 adjacent fragments. All primers for overlap PCR are listed in . PCR was performed following a standard protocol. After initial denaturation at 95°C for 5 min, the reaction was carried out for 30 cycles, each with denaturation (95°C for 30 sec), annealing (55°C for 30 sec), and extension (72°C for 1 min), and finally extended for 5 min at 72°C. After sequencing all PCR products, the sequences were further analyzed and assembled into a circular plasmid using the software DNAMAN.
Table 1. Primers used in Overlap PCR.
Western blot analysis
To determine whether the vaccine strains possess pXO1, we examined the expression of the protective antigen (PA) encoded in pXO1. The vaccine strains A16R, Pasteur II, Qiankefusiji II, and Rentian II were cultured in 5 ml of LB medium with shaking at 220 rpm at 37°C for 18 h. The supernatants of bacterial cultures were collected after centrifugation. The exported proteins were precipitated by adding an equal volume of 95% ethanol and incubating at 4°C overnight. The proteins were obtained by centrifugation at 8000 rpm for 15 min and resuspended in 50 μl of PBS buffer after natural drying. Then, 20 μl of each sample was subjected to 10% SDS-PAGE. The proteins were then transferred onto a nitrocellulose membrane (Bio-Rad). The membrane was blocked with PBS containing 5% skim milk and then incubated with anti-PA antibody (1:2000, Abcam) overnight at 4°C. After being washed 3 times with PBST (PBS with 0.5% tween-2000), the membrane was incubated with a secondary HRP-conjugated goat anti-mouse antibody (1:4000, Pierce) for 45 min at room temperature. The proteins were detected by using ECL Western Blot Substrate Kit (Pierce) according to the manufacturer's instructions.
DNA isolation and quantitative real-time PCR analysis (qPCR)
Overnight cultures of B. anthracis (Pasteur II, A16R, and A16R II) were incubated in LB medium and grown to the mid-log phase (OD600 = 0.6) at 37°C with shaking. The bacterial cells were harvested by centrifugation, and the total DNA was prepared with the Wizard Genomic DNA Purification Kit (Promega, Madison, WI, USA) according to the manufacturer's instructions. Purified genomic DNA (10 ng) was subjected to quantitative real-time PCR assays, which were conducted in duplicate, using SYBR premix Ex Taq (TaKaRa Ltd, Daliang, China) on CFX96 real time PCR platform (Bio-Rad). After initial denaturation at 95°C for 30 sec, the reaction was carried out for 40 cycles, each with each denaturation at 95°C for 5 sec and annealing at 60°C for 10 sec, followed by a melting curve at the end. The copy number of the target genes on plasmid pXO1 in different strains was compared to the control values. 16s DNA was used as a reference gene for normalization. The primers for target gene amplifications are listed in .
Table 2. Primers used in quantitative real-time PCR.
Results
Isolation of B. anthracis from B. anthracis vaccine stocks
In order to verify whether the 3 vaccine strains from different resources are indeed B. anthracis, we conducted morphological and biochemical analyses. The biochemical reactions of the 3 strains were consistent with those characteristics of the B. anthracis reference stain A16R (Table S1). For example, all these strains could uptake glucose and sucrose, but could not decompose mannitol. The V-P test was negative, and no H2S was produced for all B. anthracis strains. Moreover, the stock strain of the Pasteur II vaccine strain was further confirmed as B. anthracis by using the phage susceptibility test as well as by microscopic observations of capsule formation after the bacteria were cultured in LB medium with 0.9% NaHCO3 under 5% CO2 (Fig. S1). The four vaccine strains showed different morphologies. The bacteria of Pasteur II, Qiankefusiji II, and Rentian II all grew slowly in vitro. Pasteur II grew slower than Qiankefusiji II and Rentian II, displaying a smaller colony phenotype. Our results indicated that the strains tested in this study had different growth rates and that all the vaccine strains were independent isolates of B. anthracis.
Identification of pXO1 in B. anthracis vaccine strains
To pinpoint whether the Pasteur method of high temperature passage eliminates plasmid DNA in B. anthracis vaccine strains, we isolated whole genome DNA and conducted PFGE. Comparing with A16R, which only has the pXO1 plasmid, 2 large bands corresponding to the expected plasmid sizes of pXO1 and pXO2 were observed in all 3 vaccine strains tested: Pasteur II, Qiankefusiji II, and Rentian II (). This suggested that the plasmids pXO1 and pXO2 still existed in these vaccine strains. To further confirm this finding, we employed a more sensitive overlap PCR to detect the presence of plasmid DNA. The pagA gene encoding PA located in pXO1 was amplified by PCR in all 3 B. anthracis vaccine strains (). In addition, entire plasmid DNAs, both pXO1 and pXO2, were obtained by overlap PCR (data not shown). Taken together, these experiments indicated that these vaccine strains still possessed the pXO1 plasmid DNA.
Figure 1. Mobility of the large plasmid from 4 vaccine strains in pulse-field gels after S1 digestion. The upper bands indicate the large pXO1 plasmids with the expected size of 184 kb, the lower bands indicate the pXO2 plasmids with the expected size of 95 kb. The sources of DNA were: H9812 markers digested with XbaI (Lane 1), Pasteur II (Lane 2), Rentian II (Lane 3), Qiankefusiji II (Lane 4), and A16R (pXO1 positive and pXO2 negative, Lane 5).
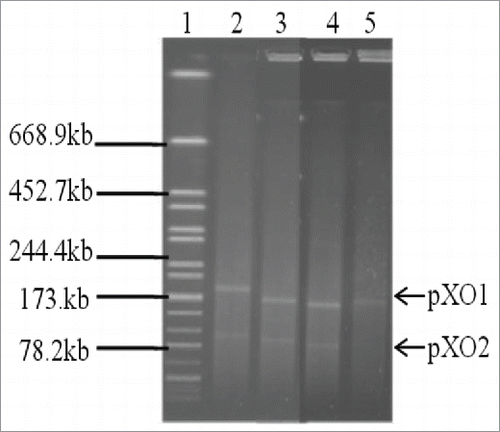
Figure 2. Detection of the pagA gene on the plasmid pXO1 in vaccine strains by PCR amplification. The pagA gene was amplified in all 3 vaccine strains: Pasteur II (Lane 2), Qiankefusiji II (Lane 3), and Rentian II (Lane 4). Lane 1 is a standard DNA marker, Lane 5 is A16R positive control, and Lane 6 is negative control.
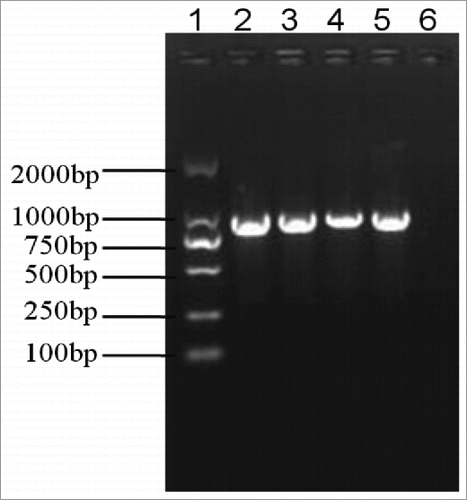
To further confirm whether the PCR products obtained by overlap PCR from these vaccine strains were the plasmid pXO1, we performed pXO1 sequencing analysis. The overlap PCR plasmid DNA was sequenced and blasted against the reference DNA sequences in the NCBI database. Results showed that the whole genome sequence of pXO1 in Pasteur II had 99% similarity with pXO1 of the Ames Ancestor strain. There was no point mutation, insertion, or deletion in the replication loci of the plasmid (InDel). Moreover, the ORFs of plasmid pXO1 in Pasteur II were annotated by using the Glimmer softwareCitation23-24 and compared with those in pXO1 of the Ames Ancestor strain (). The results showed structural similarities between the pXO1 of the Pasteur II strain and the reference Ames strain. The DNA sequencing data were submitted to the NCBI database and designated as BankIt1833550 pXO1_Plasmid KT186230.
Figure 3. Genome map of the plasmid pXO1 from Pasteur II. The order of the 5 rings from the inside is as follows: GCskew of the plasmid, genome structure and genes location of pXO1-Ames Ancestor, genes location and genome structure of Pasteur II. Orange on the genes location ring represents the genes on the sense chain of DNA and blue represents genes on the antisense chain. On the GCskew ring, purple indicates the value of GCskew as plus, green as minus; the black line indicates InDel. I is insertion, D is deletion, and the number that follows is the inserted or deleted nucleotide number. The genes with different functions as categorized by COG are labeled with different colors on the ring of genes location and illustrated on right side.
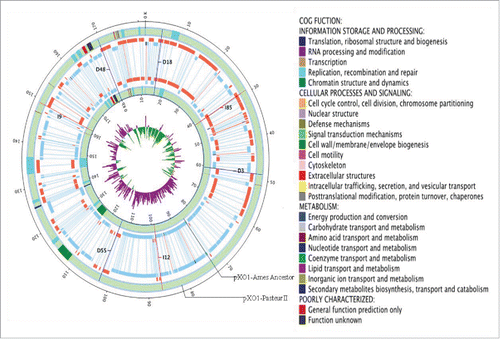
Determining the expression of the protective antigen (PA) encoded by pagA in pXO1 of the vaccine strains
To elucidate whether the protective antigen, encoded on the pXO1 plasmid, is expressed in the vaccine strains, we examined the expression of the PA using protein gel blot analysis. The exported proteins from these vaccine strains were obtained from the supernatants of the cultures and PA was detected in all 3-vaccine strains (). This result further demonstrated the existence of functional pXO1 plasmids in these vaccine strains. Moreover, the expressions of the PA were lower in these 3 vaccine strains compared to A16R ().
Figure 4. Detection of PA in vaccine strains by western blot analysis using monoclonal antibody against PA. The exported protein PA was detected in all 3 vaccine strains including Pasteur II (Lane 3), Qiankefusiji II (Lane 4) and Rentian II (Lane 5). Lane 1 is Protein Molecular Weight Marker and Lane 2 is the PA positive control strain A16R.
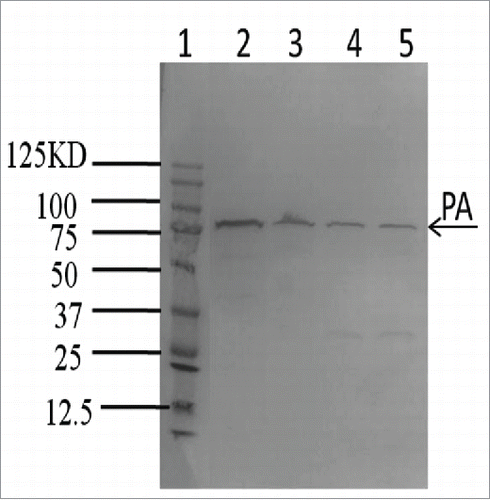
High temperature treatment is unable to eliminate plasmid DNA in B. anthracis
To test whether the exposure of high temperature can eliminate plasmids, we cultivated the A16R vaccine strain in 42.5°C for 36 days and collected the bacterial cells from the culture on Day 12, Day 24, and Day 36. The resultant strains were named as A16R I, A16R II, and A16R III. We then isolated the DNA from the above bacterial cells and performed overlap PCR on pXO1, pagA, and lef genes. Both the pagA and lef gene were detected in these cultures (). These results indicated that the exposure of B. anthracis to high temperatures could not abolish the plasmid DNA.
Figure 5. Detection of the pagA gene and the lef gene on the plasmid pXO1 via PCR amplification shows PCR amplification of the pagA gene in different B. anthracis strains. The pagA gene was positively detected in the untreated A16R strain (Lane 2) and heat-treated strains (A16R I ∼ A16R III). Lane 1 is a Standard DNA marker, Lane 2 is the A16R strain, Lane 3 is the A16R I strain, Lane 4 is the A16R II strain, Lane 5 is the A16R III strain, and Lane 6 is the negative control. shows PCR amplification of the lef gene in different B. anthracis strains. Similar to pagA gene detection, the lef gene was amplified in the untreated A16R strain (Lane 2) and heat-treated strains (A16R I ∼ A16R III). Lane 1 is a Standard DNA marker, Lane 2 is the A16R strain, Lane 3 is the A16R II strain, Lane 4 is the A16R II strain, Lane 5 is the A16R III strain, and Lane 6 is the negative control.
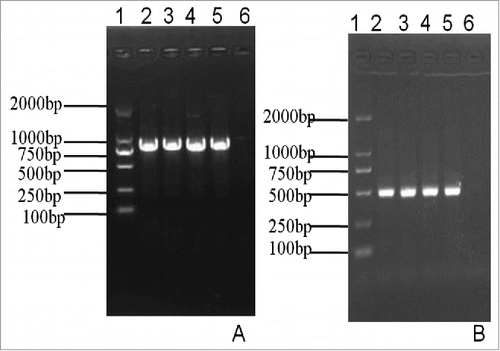
High temperature treatment decreases the copy number of pXO1
Considering that no plasmid could be detected by using traditional plasmid purification and agarose gel detection methods but was detected with the more sensitive PCR method in the vaccine strains, we speculated that the plasmid was present in a low copy number in the vaccine strains, and that the exposure to high temperature is likely to reduce the copy number of plasmid DNA in B. anthracis. To test this possibility, we compared the copy number of the plasmid DNA between high-temperature treated and untreated bacterial cells using a qPCR approach. We purified the whole genomic DNA from mid-log phase to determine whether there are any differences between Pasteur II and A16R strains. Since only one copy of the candidate genes was present on the plasmid pXO1, the copy number of the targeted genes was equal to the copy number of the plasmid. Our results showed that there was a 64-fold increase in the copy numbers of the 8 target genes located in pXO1 in the A16R cells than those in the Pasteur II (), indicating that in the Pasteur II strain, the copy number of the pXO1 plasmid was much lower than that of the A16R strain. In addition, we found the copy numbers of pXO1 in the other 2 vaccine strains. The results showed a 16-fold decrease in the copies of the target genes on pXO1 in Qiankefusiji II and Rentian II compared to that of the A16R strain (data not shown). Moreover, the copy number of pXO1 in A16R cells that were exposed to high temperature for 24 days was 16 fold lower than that of the untreated cells (). All these results indicate that heat treatment can reduce the copy number of pXO1 in B. anthracis.
Table 3. Comparison of gene copies by qPCR analysis between Pasteur II, A16R and A16R II strain.
Discussion
This study is the first to demonstrate that all 3 attenuated B. anthracis vaccine strains from different countries, which were thought to have lost the pXO1 plasmid due to exposure to high temperatures during subculture, still possess the pXO1 plasmid DNA. Our data also showed that the pXO1 plasmid DNA is still functional, as the expression of the protective antigen was detected in these vaccine strains, including B. anthracis vaccine Pasteur II, Qiankefusiji II, and Rentian II. Our results suggest that the attenuation of the Pasteur II vaccine strain is likely due to the impact of high temperature stress on plasmid replication, which in turn limits the copy number of pXO1. Our data may provide new insights into the mechanism of the remaining immunogenicity and toxicity of the vaccine strains. These findings imply potential risks of utilizing these attenuated strains for vaccinations in the field and also indicate that alternative approaches are needed for the construction of safer B. anthracis vaccines.
Our results contradict previous reports that the Pasteur II vaccine strain has lost the pXO1 plasmid,Citation6 which been cited in all relevant Chinese literatures.Citation1 This discrepancy is possibly due to the different sensitivities of the detection methods. In fact, we were also unable to directly detect the presence of pXO1 plasmid DNA from the isolated chromosomal DNA using the traditional plasmid purification and agarose gel detection approaches (data not shown). However, using more sensitive methods, including PFGE, overlap PCR, and DNA sequencing, we were able to detect the presence of pXO1 plasmid DNA from 3 B. anthracis vaccine strains. Using qPCR assay, we found that there are more copies of the pXO1 plasmid in the A16R strain than in the Pasteur II strain. Our data indicate that these vaccine strains still possess at least a low copy number of plasmid DNA after treatment with high temperatures as previously described. Plasmid curing by heating is known to induce chromosomal mutations, such as in the SMC (structural maintenance of chromosomes) homolog, which could reduce plasmid copy number.Citation25 However, no mutations are present in the SMC homolog protein of B. anthracis Pasteur II after DNA sequencing analysis. This suggests that other mechanisms are involved in the regulation of pXO1 copy number in B. anthracis. We are currently in the process of elucidating the mechanisms.
To rule out possible contamination of these stored vaccine strains, we mimicked the Pasteur's method for generating vaccine strains by subculturing the A16R vaccine strain with loss of the pXO2 plasmid and the Pasteur II vaccine strain for 36 days at 42.5°C as described. Consistently, we were able to detect the pXO1 plasmid DNA in these subcultures using overlap PCR. These findings indicate that the high temperature treatment at 42.5°C cannot eliminate the pXO1 plasmid DNA in B. anthracis cells. Surprisingly, we were unable to detect the pXO2 plasmid DNA in Pasteur II subcultures after 24 passages (data not shown). It is possible that pXO1 and pXO2 plasmids have different susceptibilities to heat stress.
In China, a surveillance network on provincial, national, and local levels has been established in the high incidence provinces since 1990, and the cooperation between veterinary and sanitary systems was strengthened. The research areas were mainly focused on epidemiology, ecology, and serology studies, including establishing reference strains and conducting phenotypic, biochemical, and genetic analyses of B. anthracis isolates. Our surveillance studies of anthrax in the field revealed a phenomenon that the pXO1+/pXO2− toxic strains were easily detected in vomitus and feces, whereas strains lacking the pXO1 plasmid have never been detected.Citation10 This observation is consistent with Turnbull's report that the pXO1−/pXO2+ strain has not been found in nature globally.Citation26 We speculate that it would not be difficult to isolate such a wild strain lacking the pXO1 plasmid if the plasmid indeed was absent after 12 passages at 42.5°C. However, it is possible that the high temperature stress induces a gene mutation during the subculture of B. anthracis in vitro, impairs the plasmid replication, and consequently reduces the copy number of the plasmid DNA.Citation27 Recently, it was reported that 3 genes are required for pXO1 plasmid partitioning.Citation28
In conclusion, our data clearly demonstrated the presence of the pXO1 plasmid DNA in B. anthracis vaccine strains, including Pasteur II, Qiankefusiji II, and Rentian II. Our results indicated that the exposure to high temperatures cannot abolish the presence of the pXO1 plasmid in B. anthracis cells. Our findings suggest that it is necessary to confirm the existence of pXO1 plasmid in vaccine strains using more sensitive methods in order to provide safer B. anthracis vaccines.
Disclosure of potential conflicts of interest
No potential conflicts of interest were disclosed.
Author contributions
Conceived and designed the experiments: XL, JZh. Performed the experiments: XL, EZh, XZh, HZh, JW, WeL. Analyzed the data: XL, BWa. Wrote the paper: XL, JZh, SD.
KVIR_S_1164366.docx
Download MS Word (2 MB)Acknowledgments
We thank Dr. Yinduo Ji and Dr. Joe Shaw for suggestions, technical support, and critical reading of the manuscript.
Funding
This work was partially supported by grant 2011SKLID210 from the State Key Laboratory for Infectious Disease Prevention and Control.
References
- Liang, X. Chief Editor, Manual of Anthrax Control and Treatment. Beijing: China Agricultural Press, p28-30, 1995.
- Pasteur L. Del'attenuation des virus et de leur retour a la virulence. 1881. C R Acad Sci Agric Bulg 92:429-435.
- Suo W. Chief Editor, Vaccine-preventable diseases. Moscow: Russia medical Press, p324-325, 1960.
- Commission of Chinese Veterinary Pharmacopoeia. China Veterinary Standard. Beijing: China Agriculture Press, p566-568, 1978.
- Sterne M. The use of anthrax vaccines prepared from a virulent variants of B. anthracis, Ondersteport. J Vet Sci Ind 1939; 13:307-312.
- Mikesell P, Ivins BE, Ristroph JD, Dreier TM. Evidence for plasmid mediated toxin production in B. anthracis. Infect Immun 1983; 39:371-376; PMID:6401695
- Uchida I, Hashimoto K, Terakado N. Virulence and immunogenicity in experimental animals of Bacillus anthracis strains harboring or lacking 110 MDa and 60 MDa plasmids. J Gen Microbiol 1986; 132:557-559; PMID:3086499
- Okinaka RT, Cloud K, Hampton O, Hoffmaster AR, Hill KK, Keim P, Koehler TM, Lamke G, Kumano S, Mahillon J, et al. Sequence and organization of pXO1, the large Bacillus anthracis plasmid harboring the anthrax toxin genes. J Bacteriol 1999; 181:6509-6515; PMID:10515943
- Little SF, Knudson GB. Comparative efficacy of Bacillus anthracis live spore vaccine and protective antigen vaccine against anthrax in the guinea pig. Infect Immun 1986; 52:509-512; PMID:3084385
- Liang X, Li A, Ma F. Plasmid and protein profiles of Bacillus Anthracis strains isolated in China. Salisbury Medical Bulletin 1996; 87:41-43.
- Koehler TM, Dai Z, Kaufman-Yarbray M. Regulation of the Bacillus anthracis protective antigen gene: CO2 and a trans-acting element activate transcription from one of two promoters. J Bacteriol 1994; 176:586-595.
- Hoffmaster AR, Koehler TM. Control of virulence gene expression in Bacillus anthracis. J Appl Microbiol 1999; 87:279-281; PMID:10475965
- Okinaka RT, Cloud K, Hampton O, Hoffmaster AR, Hill KK, Keim P, Koehler TM, Lamke G, Kumano S, Mahillon J, et al. Sequence and organization of pXO1, the large Bacillus anthracis plasmid harboring the anthrax toxin genes. J Bacteriol 1999; 181:6509-6515; PMID:10515943
- Bourgogne A, Drysdale M, Hilsenbeck SG, Peterson SN, Koehler TM. Global effects of virulence gene regulators in a Bacillus anthracis strain with both virulence plasmids. Infect Immun 2003; 71:2736-2743; PMID:12704148
- Mignot T, Mock M, Fouet A. A plasmid-encoded regulator couples the synthesis of toxins and surface structures in Bacillus anthracis. Mol Microbio 2003; l47:917-927; PMID:12581349
- Kern JW, Schneewind O. BslA, a pXO1-encoded adhesin of Bacillus anthracis. Mol Microbiol 2008; 68:504-515; PMID:18366441; http://dx.doi.org/10.1111/j.1365-2958.2008.06169.x
- Singh Y, Klimpel KR, Goel S, Swain PK, Leppla SH. Oligomerization of anthrax toxin protective antigen and binding of lethal factor during endocytic uptake into mammalian cells. Infect Immun 1999; 67:1853-1859; PMID:10085027
- Uchida I, Makino S, Sekizaki T, Terakado N. Cross-talk to the genes for Bacillus anthracis cap-sule synthesis byatxA, the gene encoding the trans-activatorof anthrax toxin synthesis. Mol Microbiol 1997; 23:1229-40
- Woo SJ, Kang SS, Park SM, Yang JS, Song MK, Yun CH, Han SH. Intranasal immunization with protective antigen of Bacillus anthracis induces a long-term immunological memory response. Mol Immunol 2015; 67(2):492-500; PMID:26278659
- Wang B, Zhang S, Yu X, Dong S. Characteristics of Bacillus anthracis bacteripohage AP631. Chinese J Virol 1992; 8:193-194.
- Barton BM, Harding GP, Zuccarelli AJ. A general method for detecting and sizing large plasmids. Anal Biochem 1995; 226:235-240; PMID:7793624
- Ho SN, Hunt HD, Horton RM, Pullen JK, Pease LR. Site-directed mutagenesis by overlap extension using the polymerase chain reaction. Gene 1989; 77:51-59; PMID:2744487
- Kanehisa M, Goto S, Hattori M, Aoki-Kinoshita KF, Itoh M, Kawashima S. From genomics to chemical genomics: New developments in KEGG. Nucleic Acids Res 2006; 34 (Database issue):D354-357; PMID:16381885
- Vargas WA, Martín JM, Rech GE, Rivera LP, Benito EP, Díaz-Mínguez JM, Thon MR, Sukno SA. Plant defense mechanisms are activated during biotrophic and necrotrophic development of Colletotricumgraminicola in maize. Plant Physiol 2012; 158:1342-1358; PMID:22247271
- Panas MW, Jain P, Yang H, Mitra S, Biswas D, Wattam AR, Letvin NL, Jacobs WR Jr. Noncanonical SMC protein in Mycobacterium smegmatis restricts maintenance of Mycobacterium fortuitum plasmids. Proc Natl Acad Sci 2014; 111:13264-13271; PMID:25197070
- Turnbull PC, Hutson RA, Ward MJ, Jones MN, Quinn CP, Finnie NJ, Duggleby CJ, Kramer JM, Melling J. Bacillus anthracis but not always anthrax. J Appl Bacteriol 1992; 72:21-28; PMID:1541596
- Long H, Liu H, Fang M. Study of plasmid curing methods and appraisal of plasmid elimination effect. Chinese J Health Lab Tech 2007; 17:413-415
- Pomerantsev AP, Chang Z, Rappole C, Leppla SH. Identification of three noncontiguous regions on Bacillus anthracis plasmid pXO1 that are important for its maintenance. J Bacteriol 2014; 196:2921-33; PMID:24914182