ABSTRACT
The IL-22 signaling pathway is critical for regulating mucosal defense and limiting bacterial dissemination. IL-22 is unusual among interleukins because it does not directly regulate the function of conventional immune cells, but instead targets cells at outer body barriers, such as respiratory epithelial cells. Consequently, IL-22 signaling participates in the maintenance of the lung mucosal barrier by controlling cell proliferation and tissue repair, and enhancing the production of specific chemokines and anti-microbial peptides. Pseudomonas aeruginosa is a major pathogen of ventilator-associated pneumonia and causes considerable lung tissue damage. A feature underlying the pathogenicity of this bacterium is its capacity to persist and develop in the host, particularly in the clinical context of nosocomial lung infections. We aimed to investigate the ability of P. auruginosa to disrupt immune-epithelial cells cross-talk. We found that P. aeruginosa escapes the host mucosal defenses by degrading IL-22, leading to severe inhibition of IL-22-mediated immune responses. We demonstrated in vitro that, protease IV, a type 2 secretion system-dependent serine protease, is responsible for the degradation of IL-22 by P. aeruginosa. Moreover, the major anti-proteases molecules present in the lungs were unable to inhibit protease IV enzymatic activity. In addition, tracheal aspirates of patients infected by P. aeruginosa contain protease IV activity which further results in IL-22 degradation. This so far undescribed cleavage of IL-22 by a bacterial protease is likely to be an immune-evasion strategy that contributes to P. aeruginosa-triggered respiratory infections.
Introduction
Ventilator-associated pneumonia is the most frequent hospital-acquired infection in patients requiring mechanical ventilation and its estimated incidence is 10–20%. In addition to being an independent factor for mortality, ventilator-associated pneumonia is associated with longer intensive care unit and hospital stays, prolonged mechanical ventilation, and higher costs.Citation1,2 Pseudomonas aeruginosa is a major pathogen of nosocomial pneumonia.Citation3 During invasive mechanical ventilation, P. aeruginosa lung infection progresses rapidly, is difficult to eradicate and is life-threatening. Many patients require antibiotic treatment to treat such infections, but this is also a recognized risk factor for the emergence of multi-drug resistance bacteria. Rates of antibiotic resistance by P. aeruginosa are increasing worldwide.Citation4 Another critical feature underlying the pathogenicity of this bacterium is its capacity to evade the host's immune response and thus to persist in the host. Thus, accurately deciphering the mechanisms by which P. aeruginosa circumvents the host immune response is a necessary milestone for innovating therapeutic development.
The respiratory epithelium, a component of the lung immune system, has mechanisms that provide a first line of defense against invading pathogens. The interleukin (IL)-22 was recently shown to be a key mediator of mucosal innate immunity. IL-22 (a member of the IL-10 cytokine) is an important component of immune-epithelial cells cross-talk and plays a critical role in regulating the maintenance of the mucosal barrier.Citation5,6 Consequently, IL-22 is crucial for limiting bacterial replication and dissemination, probably by stimulating epithelial cells at barrier surfaces to produce antimicrobial peptides,Citation5,6 such as β-defensin-2 that plays a pivotal role in the control of P. aeruginosa infection.Citation7,8 IL-22 also directly targets cells at outer-body barriers, such as respiratory epithelial cells, to induce expression of genes involved in tissue homeostasis and control the repair and turnover of lung epithelia cells damaged by infection.
Lung tissue damage is a hallmark of P. aeruginosa–induced lung pathogenesis.Citation9,10 P. aeruginosa produces many virulence factors, including secreted proteases that can degrade natural proteins, enabling bacteria to evade the host immune system and colonize host tissues.Citation11–13 In view of the major role of IL-22 in innate defense mechanisms, we sought to determine the consequences of P. aeruginosa-secreted proteases on IL-22 signaling. We demonstrate that P. aeruginosa-derived protease IV is a new immuno-evasion factor that weakens mucosal innate defenses by proteolytically decreasing the IL-22-dependent response.
Results
Il-22 is degraded by T2SS-dependent products of P. aeruginosa
P. aeruginosa produces numerous virulence factors that target several host cell types or molecular effectors, thus manipulating the host immune response to the bacterium's advantage.Citation12,14 Among these factors is a panel of extracellular proteases.Citation11,12 We tested the integrity of IL-22 in the presence of the P. aeruginosa secretome since IL-22 is a key effector against P. aeruginosa-triggered lung alterations.Citation15
IL-22 was degraded by ∼41%, ∼68%, and ∼79% after one, 2 and 6 hours exposure to P. aeruginosa PAK strain, respectively (). P. aeruginosa releases proteins using 6 different secretion systems (T1SS - T6SS);Citation12 with T2SS and T3SS being the most responsible for fatalities due to P. aeruginosa pneumonia.Citation16 We used the T3SS-deficient P. aeruginosa strain PAK ΔpscF and the double T3SS and T2SS-deficient strain PAK ΔpscF ΔxcpQ to identify the secretion system involved in IL-22 cleavage. IL-22 was strongly degraded after incubation with the PAK ΔpscF secretome (by 70% one hour after challenge), and was barely altered after incubation with the PAK ΔpscF ΔxcpQ secretome (by 20% one hour after challenge) (). We conclude that IL-22 is cleaved essentially by T2SS secretion products.
Figure 1. The degradation of IL-22 Is dependent on P. aeruginosa T2SS and protease IV. (A) IL-22 (50 ng) was incubated with secretomes from a wild-type P. aeruginosa PAK strain, a T3SS-deficient strain (PAKΔpscF), a double T3SS- and T2SS-deficient strain (PAKΔpscFΔxcpQ), or Luria broth as a control. (B) IL-22 samples were incubated with the following purified P. aeruginosa proteases: elastase B (LasB), PA small protease (PASP), protease IV (P-IV), or with alkaline protease (Alk). (C) IL-22 was also incubated with secretomes from PAK ΔpscFΔLasB (a T3SS and elastase B-deficient strain) or PAK ΔpscFΔpiv (a T3SS and protease IV-deficient strain). Proteins were extracted after incubation for various times as indicated in panels (A, C) or after 30 min (B) and analyzed by protein gel blotting using a specific anti-IL-22 antibody. Results are representative of 3 independent experiments.
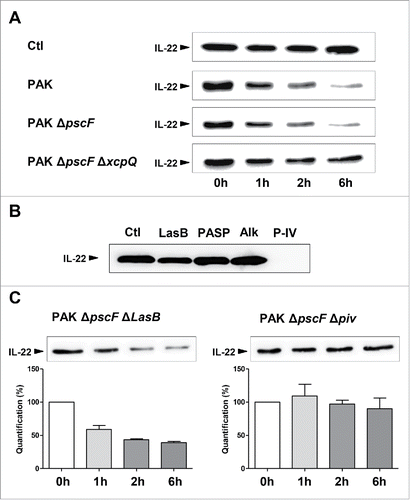
The proteins secreted by the T2SS system include a large number of proteolytic enzymes, such as protease IV, elastase B (LasB), alkaline protease (Alk; also secreted by T1SS),Citation12 and possibly “P. aeruginosa small protease” (PASP). We tested the ability of each of these proteases to cleave IL-22. We initially verified the enzymatic activity of each purified protease by gelatin zymography (data not shown). We found that IL-22 was mostly degraded by protease IV (). We further confirmed this result by comparing the impact on IL-22 integrity of the secretome of a protease IV-deficient P. aeruginosa strain (PAK ΔpscF Δpiv) with that of a LasB-deficient P. aeruginosa strain (PAK ΔpscF ΔLasB); as LasB is a major extracellular protease of P. aeruginosa known to cleave several soluble and matrix proteins.Citation12,17,Citation18 LasB-deficient P. aeruginosa efficiently cleaved IL-22 whereas protease IV-deficient P. aeruginosa was barely active (). Thus, protease IV is responsible for the alteration of IL-22 ().
Degradation of IL-22 by protease IV inhibits lung epithelial-dependent immune responses
The role of protease IV in ocular infections has been extensively studied.Citation19–21 This P. aeruginosa protease also causes considerable damage in keratitis because it destroys host proteins, including fibrinogen and components of the immune system.Citation20 By contrast, little is known regarding its impact on lung mucosa, although Protease IV was found to be expressed by P. aeruginosa lung isolates from chronically infected adult patients.Citation22,23 Approximately 75% of sputa from Pseudomonas aeruginosa-infected lungs were positive for Protease IV.Citation22 The protease IV production of numerous Pseudomonas strains was also found in all ocular isolates.Citation20 To gain insight into the functional impact of protease IV on IL-22-mediated lung immunity, we first observed that as little as 0.1 µM protease IV was enough to degrade IL-22 (), with complete degradation after exposure to 1 µM Protease IV for 10 min. No fragments were observed on the blots (despite the use of a polyclonal antibody). We performed mass spectrometry on IL-22 (0.15 µM) after exposure to protease IV (1 µM) and confirmed that IL-22 was cleaved into several small fragments within 3 minutes of incubation (see Fig S1). We next assessed the biological activity of either intact- or Protease IV-treated IL-22 on human bronchial epithelial cells.
Figure 2. The effect of IL-22 cleavage by protease IV on the lung epithelial immune response. (A) IL-22 (50ng) was incubated with various concentrations of purified P. aeruginosa protease IV (P-IV) or 1µM protease for various times (10 s to 10 min). Proteins were extracted after incubation for 30 min, or at other times as indicated, and analyzed by western blotting using an anti-IL-22 antibody. (B, C) IL-22 was incubated with 1 µM protease IV (or left untreated as a control) for 30 min at 37°C and the reaction stopped by adding excess protease inhibitor cocktail. Human bronchial epithelial BEAS-2B cells were then challenged with or without 20 ng/mL recombinant IL-22. (B) The amount of active, serine-phosphorylated STAT3 transcription factor (p-STAT3) in cell lysates was determined by protein gel blotting. The p-STAT3 signal was normalized to that of the total STAT3 signal. (C) The concentration of β-defensin 2 (BD-2) mRNA was determined by RT-qPCR and normalized to that of Hprt1 mRNA. (D) Bronchial epithelial BEAS-2B cells were infected at multiplicity of infection of 0.1 for 20 hours, with either PAK ΔpscF (a P. aeruginosa mutant strain with deletion only on a critical gene encoding the type III secretion system) and PAK ΔpscF Δpiv (a P. aeruginosa mutant strain with deletions on the gene encoding the type III secretion system as well as on the gene encoding protease IV). We assessed the viability of the cells after incubation with the bacteria by a colorimetric method. Results are expressed as the mean ± SEM of 3 independent experiments. *: P ≤ 0.05; ns: not significant.
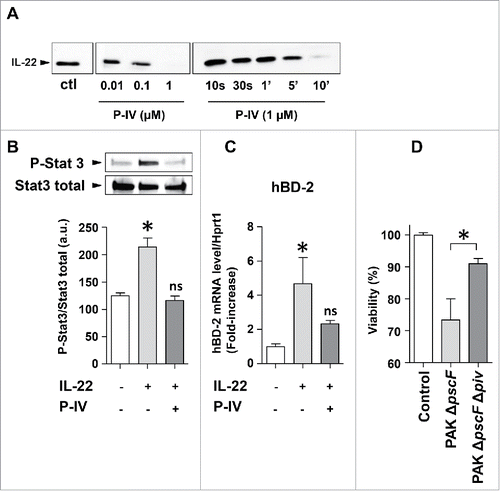
Binding of IL-22 to its receptor induces downstream signaling that requires phosphorylation of the transcription factor STAT3 on Tyr705.Citation24 Phosphorylated STAT3 then mediates the biological effects of IL-22 on epithelial cells, which both facilitate host defenses and limit lung injury by regulating the expression of genes that encode regenerative and mitogenic molecules. Bronchial epithelial cells incubated with intact IL-22 had a higher level of phosphorylated STAT3 than resting cells, as expected. In contrast, bronchial epithelial cells incubated with protease IV-treated IL-22 had a substantially lower level of phosphorylated STAT3 than those incubated with intact IL-22, similar to that of resting cells (). IL-22-induced STAT3 activation regulates the expression of several immunity genes including those encoding antimicrobial peptides such as β-defensin 2, S100A7, S100A12, and lipocalin 2.Citation25–28 Intact IL-22 induced β-defensin 2 expression (as assessed by RT-qPCR), whereas protease IV-treated IL-22 did not (). Furthermore, viability of bronchial epithelial cells exposed to a protease IV-deficient P. aeruginosa strain(PAK ΔpscF Δpiv) was higher than viability of cells exposed to an intact, control P. aeruginosa strain (PAK ΔpscF; ).
Altogether, our data demonstrate that protease IV is a virulent factor that can damage the lung epithelium and can also degrade IL-22, thus weakening tissue repair and the anti-microbial defense against P. aeruginosa in the airway epithelium.
The lungs contain a battery of antiprotease molecules to counterbalance the often harmful effects of pulmonary proteases.Citation29 The major serine proteases inhibitors (the so-called serpins) present in the lungs include α-1 antitrypsin (α1AT), elafin, secretory leukocyte protease inhibitor (SLPI), and α-1-antichymotrypsin (α1ACT).Citation29 We tested whether protease IV enzymatic activity was compatible with the lung environment, rich in protease inhibitors. None of the tested lung antiproteases were able to significantly diminish the proteolytic activity of protease IV (). The absence of an inhibitory effect was not due to a proteolysis of those antiproteases molecules as protease IV cleaved none of them (). Remarkably, protease IV was also still active in the presence of plasma, although it contains several potent serpins such as α-2-macroglobulin, antithrombin III, C1-inhibitor and α 2-antiplasmin in addition to α1AT and α1ACT (). Consistent with a previous report,Citation21 we were only able to inactivate protease IV using AEBSF and TLCK, 2 irreversible pharmacological inhibitors of trypsin and trypsin-like serine proteases.
Figure 3. Assessment of the activity of physiological and synthetic protease inhibitors on protease IV. (A) Protease IV was incubated 30 min with or without the different inhibitors and its proteolytic activity measured using the substrate N-p-Tosyl-Gly-Pro-Lys-pNa. Data (mean ± SEM) are represented as the percentage of inhibition relative to protease alone. (B) SDS-PAGE of α1AT, α1ACT, SLPI and elafin showing the absence of their proteolysis by the protease IV. α1AT (10−7 M), α1ACT (10−7 M), elafin (3.10−7 M) and SLPI (3.10−7 M) were incubated with protease IV (10−7 M) during 30 min at 37°C and then separated on 12% gradient gel and detected by silver staining.
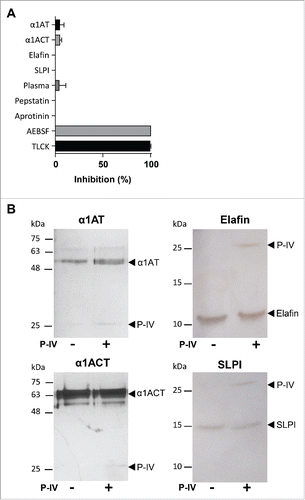
Degradation of IL-22 in human respiratory fluids containing P. aeruginosa protease IV
The airways of mechanically-ventilated patients are at increased risk of colonization by bacterial pathogens. A significant fraction (10–30%) of patients with ventilator-associated trachea-bronchitis progress to ventilator-associated pneumonia.Citation30 The most common microorganism in such infections is P. aeruginosa (30%).Citation31,32 We examined whether respiratory fluids of P. aeruginosa-infected ventilated patients may contain active protease IV in a concentration sufficient to inhibit the IL-22 signaling pathway. We used 2 types of synthetic peptide substrates to, not only quantify, but also distinguish the distinct protease activities present in these respiratory fluids. Such tracheal aspirates may contain not only P. aeruginosa Protease IV but also serine proteases released by polymorphonuclear neutrophils, i.e. elastase, cathepsin G, and protease 3.Citation33 The 2 substrates were Abz-HPVPVYAFSPQ-EDDnp and N-p-Tosyl-Gly-Pro-Lys-pNa. Abz-HPVPVYAFSPQ-EDDnp was cleaved by all neutrophil serine proteases but not protease IV (), whereas N-p-Tosyl-Gly-Pro-Lys-pNa was cleaved only by protease IV (). Thus, the substrate N-p-Tosyl-Gly-Pro-Lys-pNa is specific for protease IV activity, among pseudomonal and neutrophil serine proteases. We tested N-p-Tosyl-Gly-Pro-Lys-pNa with the secretomes produced by the above-mentioned P. aeruginosa mutant strains and confirmed that the T2SS-deficient- or Protease IV-deficient P. aeruginosa strains did not cleave N-p-Tosyl-Gly-Pro-Lys-pNa, whereas the other mutant strains did (data not shown). We collected tracheal aspirates from 2 groups of ventilated patients with tracheo-bronchitis, one positive for P. aeruginosa infection, the other negative (). We first measured the concentration of MPO, a potent inflammatory marker associated with neutrophil recruitment and activation, in the respiratory fluids. We found that MPO levels were not related to the presence or absence of P. aeruginosa in these samples (). In contrast, proteolysis of N-p-Tosyl-Gly-Pro-Lys-pNa was clearly associated with the tracheal aspirates positive for P. aeruginosa, indicating the presence of protease IV only in these fluid samples (). IL-22 was preferentially degraded by the Protease IV-positive fluids when we assessed the stability of IL-22 in the 2 types of tracheal aspirates (). Comparison of Protease IV activity and IL-22 degradation showed a positive and significant correlation (p < 0.05). We thus demonstrate that respiratory fluids of ventilated patients with P. aeruginosa tracheo-bronchitis contain active protease IV which efficiently cleaves IL-22.
Figure 4. Degradation of IL-22 by human respiratory fluids infected by P. aeruginosa. (A) P. aeruginosa and neutrophil proteases were assayed by incubating each protease with specific chromogenic or fluorogenic peptide substrates, i.e., N-p-Tosyl-Gly-Pro-Lys-pNa and Abz-HPVPVYAFSPQ-EDDnp, respectively, at 37°C for 30 min. Cleavage of the peptide substrates was assessed by measuring the increase of either fluorescence emission or optical density. Gelatin zymography was used to verify the enzymatic activities of all P. aeruginosa proteases. The activity of each enzyme was graded on a scale of (−) for “no detectable activity” to (+++) for “maximal activity.” (B) MPO concentration and N-p-Tosyl-Gly-Pro-Lys-pNa cleavage measured in tracheal aspirates from non-infected patients (“PA-,” n = 10) or patients infected by P. aeruginosa (“PA+,” n = 6). (C) IL-22 (10 ng) was incubated with human respiratory fluids for 15 minutes and quantified by ELISA. Results are representative of 2 independent experiments (A) or expressed as the mean ± SEM (B, C). *: P ≤ 0.05; ns: not significant. LasB: elastase B, P-IV: protease IV, PASP: P. aeruginosa small protease, Alk: alkaline protease, SN-PA: supernatant of P. aeruginosa, SN-PMN: supernatant of neutrophils, NE: neutrophil elastase, CG: cathepsin G, PR-3: proteinase 3, ND: not determined.
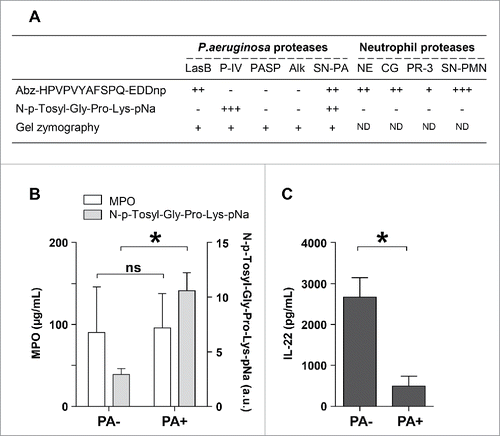
Table 1. Bacterial Strains used in this study were as follows.
Discussion
We demonstrate that the protease IV secreted by P. aeruginosa degrades IL-22 and that none of the major antiproteases present in the lung environment inhibit protease IV activity. IL-22 cleavage by P. aeruginosa leads to the inhibition of IL-22-mediated immunity, markedly altering the host mucosal defense and possibly conferring multiple survival advantages to this opportunistic bacterium. Hence, we have characterized a new P. aeruginosa immuno-evasion mechanism, which may contribute to the deterioration of lung function of patients infected by P. aeruginosa, including mechanically-ventilated individuals.
P. aeruginosa protease IV is known to be a corneal virulence factor, able to degrade several proteins important for innate immunity.Citation20,21 Protease IV in the lungs has been poorly studied. In vitro observations suggest that P. aeruginosa protease IV degrades surfactant proteins, reducing pulmonary surfactant-based host defense and biophysical functions.Citation34 Our findings demonstrate that protease IV not only degrades a final effector of innate immunity, but also inactivates a key component of immune-epithelial cell cross-talk. Indeed, IL-22 is the only known cytokine secreted by immune cells that acts exclusively on non-immune epithelial cells. Consequently, IL-22 signaling is critical for the preservation of the lung mucosal barrier by increasing cell survival, cell proliferation, cell migration, and tissue repair, and by triggering the production of specific chemokines and anti-microbial peptides.Citation5,6,Citation35 A study of lung infection with Klebsiella pneumoniae demonstrated a large, significant increase in mortality in IL22−/− mice due to increased bacterial adhesion and invasion.Citation28 Furthermore, intranasal instillation of recombinant mouse IL-22 before introduction of a sublethal dose of P. aeruginosa increased survival in neutropenic mice.Citation15
Animal models are important for the translation of experimental findings from the bench to bedside. We originally intended to compare the in vivo response of mice infected either by P. aeruginosa or a protease IV-deficient P. aeruginosa strain. A model, by definition, is not a perfect replication of the clinical condition, although it is designed for translation to human disease. The human disease herein is ventilator-associated pneumonia due to P. aeruginosa. In humans, these infections generally develop over several days and steadily progress from initial respiratory tract colonization to tracheobronchitis (defined by fever, increased purulent-appearing endotracheal aspirate, and hyperleukocytosis), and ultimately pneumonia (defined by the previous signs, plus confirmation of lung consolidation by chest X-ray). The biological properties of protease IV may be clinically relevant at early stages of lung infection by P. aeruginosa, to counteract innate defense mechanisms. Animal models poorly mimic this progressive spread of lung infection, but rather model acute lung infection or artificial P. aeruginosa-biofilm with alginate beads. We chose not to study animal models without a clear translational perspective. In contrast, the strength of our work has been the comparison of our in vitro observations with results obtained using respiratory fluids of patients newly infected by P. aeruginosa. Indeed, we confirmed the increased instability of IL-22 in these fluids. Direct visualization of IL-22 cleavage fragments in respiratory fluids would have strengthened the relevance of the study, but the complete degradation of IL-22 in situ made this impossible.
The treatment of the early stages of nosocomial lung infections is an ongoing matter of debate. Antibiotic treatment of patients newly colonized by P. aeruginosa may potentially reduce the incidence of pneumonia, but there is no clear clinical evidence of this.Citation36 Furthermore, not all cases of respiratory tract colonization convert to pneumonia. Treating all patients with respiratory tract colonization would increase the emergence of multidrug-resistant bacteria in these critically ill patients.Citation37 This conundrum highlights the need to improve the therapeutic arsenal against respiratory infections to reduce the need for antibiotherapy. Our findings may lead to the design of new drugs that target host innate immunity to reinstate at least part of the immune defenses altered by pseudomonal proteases. Protease IV appears to be a promising antimicrobial therapeutic target for the protection of IL-22 signaling to restore or prevent P. aeruginosa-triggered alterations of lung mucosal defenses.
In conclusion, protease IV plays an important role in the pathogenesis of P. aeruginosa-induced microbial keratitis, but little is known of its role in lung infection. Our findings suggest that protease IV reduces the innate immunity of the host by disrupting signaling from immune cells to epithelial cells. Altogether, these in vitro and ex vivo observations, combined with previous results,Citation22,34 suggest that P. aeruginosa protease IV may play a significant role in both colonization of the lung and the progression of associated lung tissue damage.
Materials and methods
Materials
Human recombinant IL-22 was obtained from ImmunoTools GmbH and the anti-IL-22 antibody from Abcam. Antibodies against phospho-STAT3 (Tyr705) and STAT3 were purchased from Cell Signaling Technology. The human bronchial epithelial cell line BEAS-2B was supplied by the American Type Cell Collection. Purified Pseudomonas proteases including elastase B, alkaline protease, protease IV, and PA small protease were produced by the group of Dr. Richard O'Callaghan (University of Mississippi Medical Center, Jackson, MS, USA).Citation38 The substrates used to measure protease activities were Abz-HPVPVYAFSPQ-EDDnp obtained from GeneCust Europe and N-p-Tosyl-Gly-Pro-Lys-pNa purchased from Sigma-Aldrich. IL-22 and myeloperoxidase (MPO) concentrations were determined using DuoSet ELISA assay kits (R&D Systems). The materials and methods for RT-qPCR and mass spectrometry have been previously described.Citation39–41 α1AT and ACT were purchased from Preparatis. Pepstatin, Tosyl-L-lysyl-chloromethane hydrochloride, and 4-(2-Aminoethyl) benzenesulfonyl fluoride hydrochloride were obtained from Enzo Life Sciences. Aprotinin and SLPI were purchased from Abcam. Elafin was produced as previously described.Citation42
Bacterial strains
The P. aeruginosa PAK strain used for mutant constructions was grown in Luria broth at 37°C with shaking at 180 rpm or on 1.5% L-agar plates with or without gentamicin (Gm). The plates used for sucrose selection contained 10% sucrose. Gm selection of Escherichia coli was achieved using a final Gm concentration of 15 µg/mL and Gm selection of P. aeruginosa was performed using 50 µg/mL Gm. Deletion mutants of lasB and piv were constructed in the PAKΔpscF strain using the pEX vector and a sucrose-selectable marker.Citation43 Each gene was deleted in-frame by cloning 1-kb DNA fragments upstream and downstream of the region to be deleted into the pEX18Gm vector. The pEX deletion plasmid was verified by sequence analysis and then inserted into the PAKΔpscF mutant strain by electroporation. The gentamicin single crossovers were resolved by late log phase growth in LB and plating on LB agar and sucrose plates. Sucrose-resistant colonies were then screened for gentamicin sensitivity and the double crossover or deletion strains confirmed by PCR using gene-specific primers.
P. aeruginosa proteases were assayed in the supernatants of bacteria grown at 37°C with shaking (250 rpm) in M9 minimal medium (2 mM MgSO4, 0.1 mM CaCl2, 0.4% glucose, 12.8 g/L Na2HPO4.7H2O, 3 g/L KH2PO4, 0.5 g/L NaCl, and 1g/L NH4Cl) until they reached the stationary growth phase (OD600nm ∼1.2). Cultures were centrifuged (40 mL, 30 min, 8000 × g, 4°C) and the resulting supernatants filtered and concentrated 50-fold by ultrafiltration using a 10 kDa cut-off PES membrane (Vivaspin®; Sartorius).
Analysis of proteolytic degradation of IL-22
Recombinant IL-22 (50 ng) was incubated at 37°C with P. aeruginosa supernatants or purified proteases. Reactions were stopped by adding an excess of protease inhibitor cocktail. Proteolytic processing of IL-22 was analyzed by western blotting. The biological activity of IL-22 was determined by measuring phospho-STAT3 in bronchial epithelial BEAS-2B cells treated with an optimal concentration of IL-22 (20 ng/mL).
Analysis of virulence of P. aeruginosa lacking protease IV on human cell culture
We exposed BEAS-2B cells with 2 strains of P. aeruginosa: one which expressed protease IV (PAK ΔpscF) and the other one did not (PAK ΔpscF-Δpiv). Of note is that both strains lacked a functional type III secretion system (T3SS) as the T3SS-dependent virulence factors would drastically trigger a mortality on BEAS-2B epithelial cells, that would not allow to accurately discriminate the impact of protease IV per se. BEAS-2B cells were seeded into 12-well culture plates and incubated 48 hours at 37°C and 5% CO2. Cells were incubated with or without PAK ΔpscF and PAK ΔpscF-Δpiv at multiplicity of infection (MOI) of 0.1 for 20 hours in F-12K medium. The medium with bacteria was then removed and epithelial cells washed with PBS. Medium with gentamycin (25 µg/mL) was further added. We assessed the viability of the cells after incubation with the bacteria by a colorimetric method for determining the number of viable cells in proliferation (CellTiter 96® AQueous One Solution Cell Proliferation Assay, Promega, Madison, USA).
Analysis of substrate susceptibility to proteases
The substrates Abz-HPVPVYAFSPQ-EDDnp and N-p-Tosyl-Gly-Pro-Lys-pNa were used to determine the proteolytic capacities of neutrophil and P. aeruginosa proteases, as previously described.Citation44,45
Analysis of the efficacy of various protease inhibitors on protease IV
Protease IV (5 nM) was incubated 30 min in PBS with or without the different inhibitors: 10−7 M α1AT, α1ACT, or elafin; 10−6 M SLPI or Pepstatin; 10−4 M Aprotinin or 4-(2-Aminoethyl) benzenesulfonyl fluoride hydrochloride (AEBSF); 10−5 M Tosyl-L-lysyl-chloromethane hydrochloride (TLCK); 20% plasma. Protease IV activity was then measured using the substrate N-p-Tosyl-Gly-Pro-Lys-pNa as described.
Human respiratory fluid samples
Tracheal aspirates of ventilated patients were collected prospectively and considered to be purulent when the neutrophil count was greater than 106 cells/g.Citation46 Patients with purulent tracheal secretions were assigned to one of 2 groups: (i) patients whose tracheal secretions were recently positive for P. aeruginosa (≥104 cfu/mL) and (ii) patients with tracheal secretions free of P. aeruginosa (with or without other pathogens). Patients with a new, persistent (>48-h) infiltrate, visible by chest X-ray, were not included. This study was approved by the French bioethics authorities (L'Espace de Réflexion Ethique Région Center) and was conducted in accordance with the ethical standards of the Helsinki Declaration. Informed written consent was obtained from each participant.
Degradation of IL-22 by tracheal secretions
Human respiratory fluids were homogenized in 3 volumes (w/v) of PBS, incubated with 1 mM dithiothreitol for one hour, and then centrifuged. This concentration of dithiothreitol has no impact on the measurement of protease activity or IL-22 detection (data not shown). The human respiratory fluids were incubated for 15 min with human recombinant 10 ng IL-22 cytokine and the IL-22 quantified by ELISA.
Statistical analysis
Results are expressed as means ± SEM for the indicated number of experiments performed independently. Statistical significance between the different values was analyzed by the Man-Whitney or Kruskal-Wallis test (and Dunn's test for post-hoc comparisons) depending on the number of groups to analyze. Relationships between variables were characterized with the Pearson's correlation test. Statistical analyses was performed using GraphPad Prism5®softwre. A p value of <0.05 was considered to be significant.
Disclosure of potential conflicts of interest
No potential conflicts of interest were disclosed.
KVIR_S_1253658.zip
Download Zip (30.4 KB)Acknowledgments
We thank all the patients who volunteered for this study. We also thank Rose Aimar, Veronique Siméon, Christine Mabilat, Aurélie Aubrey, and all the physicians of the Tours Intensive Care department for collecting the human samples. We thank Marie-Louise Zani who has kindly provided us Elafin, and the “Plateforme de Spectrométrie de Masse et Protéomique du Center de Biophysique Moléculaire, Orléans, France” where mass spectrometry was performed.
Funding
This work was supported by a grant from the Association Vaincre la Mucoviscidose (grant ID RF20150501363). AG was funded by an INSERM “poste d'accueil,” EM by an INSERM postdoctoral fellowship. RR was supported by the STUDIUM. These agencies played no role in study design, data collection and analysis, the decision to publish or preparation of the manuscript.
References
- Chastre J, Fagon J-Y. Ventilator-associated pneumonia. Am J Respir Crit Care Med 2002; 165:867-903; PMID:11934711; http://dx.doi.org/10.1164/ajrccm.165.7.2105078
- Bekaert M, Timsit J-F, Vansteelandt S, Depuydt P, Vésin A, Garrouste-Orgeas M, Decruyenaere J, Clec'h C, Azoulay E, Benoit D, et al. Attributable mortality of ventilator-associated pneumonia: a reappraisal using causal analysis. Am J Respir Crit Care Med 2011; 184:1133-9; PMID:21852541; http://dx.doi.org/10.1164/rccm.201105-0867OC
- American Thoracic Society, Infectious Diseases Society of America. Guidelines for the management of adults with hospital-acquired, ventilator-associated, and healthcare-associated pneumonia. Am J Respir Crit Care Med 2005; 171:388-416; PMID:15699079; http://dx.doi.org/10.1164/rccm.200405-644ST
- Nseir S, Di Pompeo C, Diarra M, Brisson H, Tissier S, Boulo M, Durocher A. Relationship between immunosuppression and intensive care unit-acquired multidrug-resistant bacteria: a case-control study. Crit Care Med 2007; 35:1318-23; PMID:17414081; http://dx.doi.org/10.1097/01.CCM.0000261885.50604.20
- Sonnenberg GF, Fouser LA, Artis D. Border patrol: regulation of immunity, inflammation and tissue homeostasis at barrier surfaces by IL-22. Nat Immunol 2011; 12:383-90; PMID:21502992; http://dx.doi.org/10.1038/ni.2025
- Sabat R, Ouyang W, Wolk K. Therapeutic opportunities of the IL-22-IL-22R1 system. Nat Rev Drug Discov 2014; 13:21-38; PMID:24378801; http://dx.doi.org/10.1038/nrd4176
- Shu Q, Shi Z, Zhao Z, Chen Z, Yao H, Chen Q, Hoeft A, Stuber F, Fang X. Protection against Pseudomonas aeruginosa pneumonia and sepsis-induced lung injury by overexpression of beta-defensin-2 in rats. Shock Augusta Ga 2006; 26:365-71; http://dx.doi.org/10.1097/01.shk.0000224722.65929.58
- Kumar A, Zhang J, Yu F-SX. Toll-like receptor 2-mediated expression of beta-defensin-2 in human corneal epithelial cells. Microbes Infect Inst Pasteur 2006; 8:380-9; http://dx.doi.org/10.1016/j.micinf.2005.07.006
- Mijares LA, Wangdi T, Sokol C, Homer R, Medzhitov R, Kazmierczak BI. Airway epithelial MyD88 restores control of Pseudomonas aeruginosa murine infection via an IL-1-dependent pathway. J Immunol Baltim Md 1950 2011; 186:7080-8
- Ramphal R, Balloy V, Huerre M, Si-Tahar M, Chignard M. TLRs 2 and 4 are not involved in hypersusceptibility to acute Pseudomonas aeruginosa lung infections. J Immunol Baltim Md 1950 2005; 175:3927-34
- Sadikot RT, Blackwell TS, Christman JW, Prince AS. Pathogen-host interactions in Pseudomonas aeruginosa pneumonia. Am J Respir Crit Care Med 2005; 171:1209-23; PMID:15695491; http://dx.doi.org/10.1164/rccm.200408-1044SO
- Bleves S, Viarre V, Salacha R, Michel GPF, Filloux A, Voulhoux R. Protein secretion systems in Pseudomonas aeruginosa: A wealth of pathogenic weapons. Int J Med Microbiol IJMM 2010; 300:534-43; PMID:20947426; http://dx.doi.org/10.1016/j.ijmm.2010.08.005
- Beaufort N, Corvazier E, Mlanaoindrou S, de Bentzmann S, Pidard D. Disruption of the endothelial barrier by proteases from the bacterial pathogen Pseudomonas aeruginosa: implication of matrilysis and receptor cleavage. PloS One 2013; 8:e75708; PMID:24069438; http://dx.doi.org/10.1371/journal.pone.0075708
- Pernet E, Guillemot L, Burgel P-R, Martin C, Lambeau G, Sermet-Gaudelus I, Sands D, Leduc D, Morand PC, Jeammet L, et al. Pseudomonas aeruginosa eradicates Staphylococcus aureus by manipulating the host immunity. Nat Commun 2014; 5:5105; PMID:25290234; http://dx.doi.org/10.1038/ncomms6105
- Mear JB, Gosset P, Kipnis E, Faure E, Dessein R, Jawhara S, Fradin C, Faure K, Poulain D, Sendid B, et al. Candida albicans airway exposure primes the lung innate immune response against Pseudomonas aeruginosa infection through innate lymphoid cell recruitment and interleukin-22-associated mucosal response. Infect Immun 2014; 82:306-15; PMID:24166952; http://dx.doi.org/10.1128/IAI.01085-13
- Jyot J, Balloy V, Jouvion G, Verma A, Touqui L, Huerre M, Chignard M, Ramphal R. Type II secretion system of Pseudomonas aeruginosa: in vivo evidence of a significant role in death due to lung infection. J Infect Dis 2011; 203:1369-77; PMID:21502078; http://dx.doi.org/10.1093/infdis/jir045
- Golovkine G, Faudry E, Bouillot S, Voulhoux R, Attrée I, Huber P. VE-cadherin cleavage by LasB protease from Pseudomonas aeruginosa facilitates type III secretion system toxicity in endothelial cells. PLoS Pathog 2014; 10:e1003939; PMID:24626230; http://dx.doi.org/10.1371/journal.ppat.1003939
- Leduc D, Beaufort N, de Bentzmann S, Rousselle J-C, Namane A, Chignard M, Pidard D. The Pseudomonas aeruginosa LasB metalloproteinase regulates the human urokinase-type plasminogen activator receptor through domain-specific endoproteolysis. Infect Immun 2007; 75:3848-58; PMID:17517866; http://dx.doi.org/10.1128/IAI.00015-07
- O'Callaghan RJ, Engel LS, Hobden JA, Callegan MC, Green LC, Hill JM. Pseudomonas keratitis. The role of an uncharacterized exoprotein, protease IV, in corneal virulence. Invest Ophthalmol Vis Sci 1996; 37:534-43; PMID:Can't
- Engel LS, Hill JM, Moreau JM, Green LC, Hobden JA, O'Callaghan RJ. Pseudomonas aeruginosa protease IV produces corneal damage and contributes to bacterial virulence. Invest Ophthalmol Vis Sci 1998; 39:662-5; PMID:9501882
- Engel LS, Hill JM, Caballero AR, Green LC, O'Callaghan RJ. Protease IV, a unique extracellular protease and virulence factor from Pseudomonas aeruginosa. J Biol Chem 1998; 273:16792-7; PMID:9642237; http://dx.doi.org/10.1074/jbc.273.27.16792
- Smith L, Rose B, Tingpej P, Zhu H, Conibear T, Manos J, Bye P, Elkins M, Willcox M, Bell S, et al. Protease IV production in Pseudomonas aeruginosa from the lungs of adults with cystic fibrosis. J Med Microbiol 2006; 55:1641-4; PMID:17108265; http://dx.doi.org/10.1099/jmm.0.46845-0
- Upritchard HG, Cordwell SJ, Lamont IL. Immunoproteomics to examine cystic fibrosis host interactions with extracellular Pseudomonas aeruginosa proteins. Infect Immun 2008; 76:4624-32; PMID:18663005; http://dx.doi.org/10.1128/IAI.01707-07
- Lejeune D, Dumoutier L, Constantinescu S, Kruijer W, Schuringa JJ, Renauld J-C. Interleukin-22 (IL-22) activates the JAK/STAT, ERK, JNK, and p38 MAP kinase pathways in a rat hepatoma cell line. Pathways that are shared with and distinct from IL-10. J Biol Chem 2002; 277:33676-82; PMID:12087100; http://dx.doi.org/10.1074/jbc.M204204200
- Wolk K, Kunz S, Witte E, Friedrich M, Asadullah K, Sabat R. IL-22 increases the innate immunity of tissues. Immunity 2004; 21:241-54; PMID:15308104; http://dx.doi.org/10.1016/j.immuni.2004.07.007
- Wolk K, Witte E, Wallace E, Döcke W-D, Kunz S, Asadullah K, Volk H-D, Sterry W, Sabat R. IL-22 regulates the expression of genes responsible for antimicrobial defense, cellular differentiation, and mobility in keratinocytes: a potential role in psoriasis. Eur J Immunol 2006; 36:1309-23; PMID:16619290; http://dx.doi.org/10.1002/eji.200535503
- Zheng Y, Valdez PA, Danilenko DM, Hu Y, Sa SM, Gong Q, Abbas AR, Modrusan Z, Ghilardi N, de Sauvage FJ, et al. Interleukin-22 mediates early host defense against attaching and effacing bacterial pathogens. Nat Med 2008; 14:282-9; PMID:18264109; http://dx.doi.org/10.1038/nm1720
- Aujla SJ, Chan YR, Zheng M, Fei M, Askew DJ, Pociask DA, Reinhart TA, McAllister F, Edeal J, Gaus K, et al. IL-22 mediates mucosal host defense against Gram-negative bacterial pneumonia. Nat Med 2008; 14:275-81; PMID:18264110; http://dx.doi.org/10.1038/nm1710
- Greene CM, McElvaney NG. Proteases and antiproteases in chronic neutrophilic lung disease – relevance to drug discovery. Br J Pharmacol 2009; 158:1048-58; PMID:19845686; http://dx.doi.org/10.1111/j.1476-5381.2009.00448.x
- Craven DE, Hudcova J, Craven KA, Scopa C, Lei Y. Antibiotic treatment of ventilator-associated tracheobronchitis: to treat or not to treat? Curr Opin Crit Care 2014; 20:532-41; PMID:25051351; http://dx.doi.org/10.1097/MCC.0000000000000130
- Nseir S, Martin-Loeches I, Makris D, Jaillette E, Karvouniaris M, Valles J, Zakynthinos E, Artigas A. Impact of appropriate antimicrobial treatment on transition from ventilator-associated tracheobronchitis to ventilator-associated pneumonia. Crit Care Lond Engl 2014; 18:R129; http://dx.doi.org/10.1186/cc13940
- Craven DE. Ventilator-associated tracheobronchitis (VAT): questions, answers, and a new paradigm? Crit Care Lond Engl 2008; 12:157; http://dx.doi.org/10.1186/cc6912
- Pham CTN. Neutrophil serine proteases: specific regulators of inflammation. Nat Rev Immunol 2006; 6:541-50; PMID:16799473; http://dx.doi.org/10.1038/nri1841
- Malloy JL, Veldhuizen RAW, Thibodeaux BA, O'Callaghan RJ, Wright JR. Pseudomonas aeruginosa protease IV degrades surfactant proteins and inhibits surfactant host defense and biophysical functions. Am J Physiol Lung Cell Mol Physiol 2005; 288:L409-418; PMID:15516485; http://dx.doi.org/10.1152/ajplung.00322.2004
- Rutz S, Eidenschenk C, Ouyang W. IL-22, not simply a Th17 cytokine. Immunol Rev 2013; 252:116-32; PMID:23405899; http://dx.doi.org/10.1111/imr.12027
- Nseir S, Povoa P, Salluh J, Rodriguez A, Martin-Loeches I. Is there a continuum between ventilator-associated tracheobronchitis and ventilator-associated pneumonia? Intensive Care Med 2016; 42:1190-2; PMID:27080532
- Barbier F, Lisboa T, Nseir S. Understanding why resistant bacteria are associated with higher mortality in ICU patients. Intensive Care Med 2015; PMID:26564210 [epub ahead of print]
- Tang A, Marquart ME, Fratkin JD, McCormick CC, Caballero AR, Gatlin HP, O'Callaghan RJ. Properties of PASP: a Pseudomonas protease capable of mediating corneal erosions. Invest Ophthalmol Vis Sci 2009; 50:3794-801; PMID:19255155; http://dx.doi.org/10.1167/iovs.08-3107
- Guillon A, Jouan Y, Brea D, Gueugnon F, Dalloneau E, Baranek T, Henry C, Morello E, Renauld J-C, Pichavant M, et al. Neutrophil proteases alter the interleukin-22-receptor-dependent lung antimicrobial defence. Eur Respir J 2015; 46:771-82; PMID:26250498; http://dx.doi.org/10.1183/09031936.00215114
- Guillon A, Gueugnon F, Mavridis K, Dalloneau E, Jouan Y, Diot P, Heuzé-Vourc'h N, Courty Y, Si-Tahar M. Interleukin-22 receptor is overexpressed in nonsmall cell lung cancer and portends a poor prognosis. Eur Respir J 2016; 47:1277-80; PMID:26846835; http://dx.doi.org/10.1183/13993003.01580-2015
- Guarino C, Legowska M, Epinette C, Kellenberger C, Dallet-Choisy S, Sieńczyk M, Gabant G, Cadene M, Zoidakis J, Vlahou A, et al. New selective peptidyl di(chlorophenyl) phosphonate esters for visualizing and blocking neutrophil proteinase 3 in human diseases. J Biol Chem 2014; 289:31777-91; PMID:25288799; http://dx.doi.org/10.1074/jbc.M114.591339
- Zani M-L, Nobar SM, Lacour SA, Lemoine S, Boudier C, Bieth JG, Moreau T. Kinetics of the inhibition of neutrophil proteinases by recombinant elafin and pre-elafin (trappin-2) expressed in Pichia pastoris. Eur J Biochem FEBS 2004; 271:2370-8; http://dx.doi.org/10.1111/j.1432-1033.2004.04156.x
- Hoang TT, Karkhoff-Schweizer RR, Kutchma AJ, Schweizer HP. A broad-host-range Flp-FRT recombination system for site-specific excision of chromosomally-located DNA sequences: application for isolation of unmarked Pseudomonas aeruginosa mutants. Gene 1998; 212:77-86; PMID:9661666; http://dx.doi.org/10.1016/S0378-1119(98)00130-9
- Caballero AR, Moreau JM, Engel LS, Marquart ME, Hill JM, O'Callaghan RJ. Pseudomonas aeruginosa protease IV enzyme assays and comparison to other Pseudomonas proteases. Anal Biochem 2001; 290:330-7; PMID:11237336; http://dx.doi.org/10.1006/abio.2001.4999
- Kalupov T, Brillard-Bourdet M, Dadé S, Serrano H, Wartelle J, Guyot N, Juliano L, Moreau T, Belaaouaj A, Gauthier F. Structural characterization of mouse neutrophil serine proteases and identification of their substrate specificities: relevance to mouse models of human inflammatory diseases. J Biol Chem 2009; 284:34084-91; PMID:19833730; http://dx.doi.org/10.1074/jbc.M109.042903
- Palmer LB, Smaldone GC, Simon SR, O'Riordan TG, Cuccia A. Aerosolized antibiotics in mechanically ventilated patients: delivery and response. Crit Care Med 1998; 26:31-9; PMID:9428540; http://dx.doi.org/10.1097/00003246-199801000-00013