ABSTRACT
Human-pathogenic Yersinia produce plasmid-encoded Yersinia outer proteins (Yops), which are necessary to down-regulate anti-bacterial responses that constrict bacterial survival in the host. These Yops are effectively translocated directly from the bacterial into the target cell cytosol by the type III secretion system (T3SS). Cell-penetrating peptides (CPPs) in contrast are characterized by their ability to autonomously cross cell membranes and to transport cargo – independent of additional translocation systems. The recent discovery of bacterial cell-penetrating effector proteins (CPEs) – with the prototype being the T3SS effector protein YopM – established a new class of autonomously translocating immunomodulatory proteins. CPEs represent a vast source of potential self-delivering, anti-inflammatory therapeutics. In this review, we give an update on the characteristic features of the plasmid-encoded Yops and, based on recent findings, propose the further development of these proteins for potential therapeutic applications as natural or artificial cell-penetrating forms of Yops might be of value as bacteria-derived biologics.
Introduction
In the course of an infection pathogenic bacteria produce a plethora of virulence factors to modulate and undermine the host's countermeasures for their own survival and proliferation. Particularly, secretion systems such as the intriguing type 3 secretion system (T3SS) have been recognized as fascinating nanomachines which inject fine-tuned effector proteins in a one-step or two-step process into the cytosol of targeted host cells.Citation1 These effector proteins affect nearly all areas of cellular life by modulating, inhibiting or even exploiting essential cellular mechanisms for the benefit of the microbe and in doing so might act in concert.Citation2-4 Furthermore, bacterial effector proteins might target more than one cellular signaling mechanism so that the currently known targets might not be comprehensive. A mechanistic understanding of these interactions will provide important insights into the strategies of bacterial pathogens for survival in and colonization of the host. Due to the importance of secreted and injected effector proteins during bacterial infection and the spreading resistance against current antibiotics, many studies focused on effector proteins as putative targets for combating bacterial infections. However, exploiting these effector proteins for the development of novel biologics might also offer innovative possibilities for therapeutic interventions in sterile inflammation (reviewed in, refs. Citation5, 6).
In essence, many secreted bacterial virulence factors are highly adapted regulators of the human immune system. The so-called “Drugs from Bugs” concept aims at taking advantage of these immunomodulatory effects and combines it with the technology of cell-penetrating peptides or proteins (CPPs), which are able to autonomously cross biological membranes. As a result, one would have a self-delivering therapeutic at one's disposal, e.g. to treat diseases dominated by a dysregulated immune system or other failing cellular functions. Surprisingly, over the past years, quite a few bacterial effector proteins were found to harbor already an intrinsic cell-penetrating ability. Consequently, these factors were designated cell-penetrating effectors or CPEs.Citation5 These include the Escherichia coli effectors Tir,Citation7 TcpC,Citation89 and NleC,Citation10 SspH1 of Salmonella,Citation11 IpaH 9.8 of Shigella (Norkowski et al., personal communication),Citation12 and YopM of Yersinia.Citation13,14 The latter three effectors share high sequence homology in their N-terminal regions, which are dominated by an α-helical structure, followed by leucine rich repeats.Citation11,13,15 Besides, a growing number of biologic or synthetic cell-penetrating peptides is available, which can be fused either genetically or chemically to heterologous cargo-proteins for delivery into target cells and also specific target cell compartments of interest (for a review see refs. Citation16, 17). How these CPEs – or even CPP in general – actually cross eukaryotic membranes is an area of intense research as it appears that translocation mechanisms may also vary according to the particular CPE or the CPP-cargo pair. For CPEs such as YopM endocytic uptake followed by endosomal escape has been shown to be a major uptake route.Citation5,6,13,14 Employing these technologies we will be able to develop bacterial immunomodulators for therapeutic purposes.
The type III secretion system-associated, plasmid-encoded effectors of the human-pathogenic Yersinia species were among the first effector proteins to be molecularly characterized (for reviews see refs. Citation18-25). Plasmid-encoded Yersinia outer proteins (Yops) encompass the following six proteins: YopE, YopM, YopO, YopT, YopP, and YopH. In addition, recent findings indicate that YopQ/K (YopQ in Y. enterocolitica and YopK in Y. pseudotuberculosis and Y. pestis) – a translocated regulator of type-3-secretion – might also have an impact an host cell proteins like Rac1.Citation26,27 Since there is only very little data available on this Yop, it will, however, not be discussed in any detail in this review. Ongoing research efforts generate novel insights and continuously enhance our understanding of intracellular targets and the mechanisms of action for most Yops (summarized in ). Here, we want to give an update of the currently known status of the molecular functions of the individual Yops, their presently known targets, and discuss potential fields of therapeutic applications for recombinant, autonomously cell-penetrating Yersinia effector proteins. As the multifunctional ‘low-calcium response protein LcrV’ shows links to Yop effectors it has also been included in this review.
Figure 1. Intracellular functions of Yersinia outer proteins (Yops). YopE, YopT, and YopO inactivate RhoGTPases, thus mainly inhibiting phagocytosis, together with YopH, which dephosphorylates components of focal adhesion complexes. YopP (YopJ in Y. pestis and Y. pseudotuberculosis) blocks NF-κB- and MAPK-signaling probably by acetylation of important signal transducers. YopM associates with PRK and RSK and regulates pro- and anti-inflammatory gene transcription via a yet unknown mechanism. The figure was generated using Servier Medical Art.
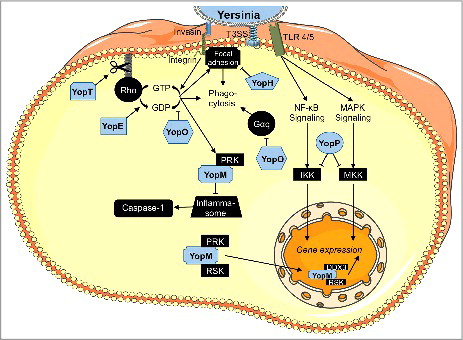
YopM – A cell-penetrating scaffold protein
Structure and function
The Yersinia outer protein M (YopM) is the only known Yop where a catalytic activity appeared to be lacking. In general, YopM belongs to the LPX family of bacterial T3SS-secreted effectors, which is a subgroup of the leucine-rich repeat (LRR) superfamily and includes the Yersinia protein YopM, different IpaH (invasion plasmid antigen H) proteins of Shigella spp. as well as the Salmonella effectors SspH1, SspH2, and SlrP (Salmonella leucine-rich repeat protein).Citation28 These proteins consist of a N-terminal α-helical domain, which is followed by a varying number of LRRs and except for the YopM protein, they all possess a C-terminal NEL domain.Citation28 However, just recently it has been suggested that the N-terminus of YopM harbors a novel E3 ubiquitin ligase that induces necrotic cell death by targeting NLRP3.Citation29 This is quite surprising as an E3 ubiquitin ligase domain has not been found previously in YopM and thus this finding needs to be confirmed.
The horseshoe shaped 13–21 leucine rich repeats (LRRs) of YopM act as a scaffold to bind host cell proteins and thus interfere with interactions in which these proteins are involved.Citation30 In vitro tetrameric complexes of YopM were observed, which form a hollow cylinder of yet unknown function.Citation30 As the number and exact composition of the LRRs varies between Yersinia species and strains,Citation31 YopM proteins vary in size between 41–55 kDa which might also be a reason for the partially contradictory results reported in different studies. Two distinct nuclear localization signals are contained within the highly conserved LRRs 1–3 as well as the C-terminus.Citation31 The N-terminus consists of two anti-parallel α-helices, which contain not only the signal for secretion and translocation via the T3SS,Citation32 but harbor also two distinct synergistically active transduction domains which allow for the autonomous translocation of (recombinant) YopM into a huge variety of eukaryotic host cells in vitro and in vivo.Citation13,14,33,34 Whether an autonomous cell-penetration of effector proteins might have a direct effect during bacterial infections has remained elusive.
Among the few established interaction partners of YopM, mature α-thrombin was the first to be discovered.Citation35 When complexed with YopM, α-thrombin is no longer able to induce platelet aggregation.Citation35 However, this interaction did not contribute to the overall virulence in a mouse infection model of Y. pestis.Citation36 The serine protease inhibitor α1-antitrypsin is also bound by YopM, yet without altering its inhibitory effect.Citation37 Nevertheless, it is remarkable that YopM, which is normally directly translocated into the host cell cytosol by the T3SS, specifically interacts with extracellular host proteins indicating a possible extracellular role of this protein. This is supported by the identification of YopM in culture supernatants of HeLa cells infected with Y. pestis.Citation38
In the cytoplasm, YopM forms a hetero-trimeric complex with the two serine/threonine kinases ribosomal S6 protein kinase (RSK) and protein kinase C-related kinase (PRK, also called PKN), which are subsequently activated.Citation33,39,40 However, formation of the RSK1-YopM-PRK2 trimer is dispensable for the anti-inflammatory effect of (recombinant) YopM and, furthermore, does not even influence physiological functions of the PRK/PKN and RSK kinases such as cell proliferation and migration.Citation33 This is also mirrored in the unaltered phosphorylation of the downstream targets BAD, Jun, CREB or Akt.Citation39,40 On the other hand, interaction with PRK2 seems to be important to dampen pyrin inflammasome activation triggered by YopE- and YopT-induced RhoA inactivation ().Citation41 Consequently, YopM appears to be less important for colonization by YopE/T-negative mutants.Citation41 After trafficking from the cytosol to the nucleus of the cells, T3SS-delivered YopM was recently shown to form yet another heterotrimeric complex, namely with RSK1 and the DEAD Box Helicase DDX3.Citation42 DDX3 mediates nuclear export of YopM, thus controlling YopM activity ().
Furthermore, inhibition of caspase-1 containing inflammasomes by YopM, either through direct binding to caspase-1 or indirectly through blocking the potential caspase-1 activator IQ motif-containing GTPase-activating protein 1 (IQGAP1) has been proposed.Citation43,44
Although the underlying mechanism for the observed effects of YopM has remained elusive, YopM has been clearly shown to be an important virulence factor with anti-inflammatory activities. Depending on the Yersinia strain, the route of infection, and the examined animal model, YopM-dependent colonization of spleen, liver and lungs,Citation45,46 depletion of natural killer cells,Citation47 reduction of pro-inflammatory cytokine secretion (including interleukins 1β, 12, 15, and 18, interferon-γ and tumor necrosis factor-α),Citation13,47 induction of caspase 3-mediated apoptosis,Citation48 or inhibition of apoptosis and migration were observed.Citation49 Additionally, YopM was also suggested to induce elevated levels of the anti-inflammatory cytokine interleukin-10.Citation46
Potential therapeutic uses
As YopM is one of the first identified CPEs, possible therapeutic applications for recombinant YopM are currently under investigation. The most promising therapeutic application to date is a topical administration of YopM for the treatment of the auto-inflammatory skin disease psoriasis, with already two granted patents ().Citation50,51 Like many other inflammatory disorders, psoriasis is characterized by elevated levels of TNF-α, which in turn drives the production of many other cytokines.Citation52 Consequently, today's mostly used therapeutic strategy relies on the systemic application of anti-TNF-α antibodies.Citation53 Clear advantages of a cell-penetrating YopM-based topical treatment would be the lack of systemic distribution of the drug (allowing lower dosage and most probably causing less detrimental side effects), a more convenient administration route (creaming instead of injection), a shift toward an earlier and non-stoichiometric intervention (inhibition of the expression of TNF-α, not of the cytokine itself), and a broader target spectrum (inhibition of TNF-α-independent pro-inflammatory cytokine production, with simultaneous induction of the anti-inflammatory cytokine IL-10). However, although these exciting results are very promising, as details of the molecular mechanism are still under investigation, recombinant YopM as a novel biologic has not reached human patients or the clinics yet.
YopE – A GTPase activating protein
Structure and function
Being the first Yop effector protein to be translocated by the T3SS,Citation54 the 23 kDa YopE plays a major role in the initial bacterial defense against phagocytes. It does so by its GAP (GTPase activating protein) activity targeting the small Rho-GTPases Rac1, RhoG and partially also RhoA, thereby disrupting actin cytoskeleton dynamics (), which is manifested by rounding up of affected cells and their inability to form phagocytic cups.Citation55-58 Furthermore, YopE is able to activate the GTPase domain of Cdc42 in vitro.Citation59
Amino acid sequence similarities of YopE to eukaryotic GAPs can only be found in an arginine finger motif, typical for this class of enzymes. However, YopE shares a striking similarity to its eukaryotic orthologues in structure.Citation60 The first 15 amino acids of YopE contain a secretion and translocation signal, which is necessary and sufficient for translocation into host cells via the T3SS when YopE is bound to its specific chaperone SycE via aa 15–50.Citation61 Amino acid residues 50–77 contain an inhibitory sequence for translocation (reversed by SycE binding)Citation61 which - in the host cell - functions as a membrane localization signal (MLS).Citation62 Depending on the Yersinia serogroup, this MLS harbors two lysine residues which can be ubiquitinated by the host cell, marking YopE for proteasomal degradation.Citation63,64 This represents an interesting mechanism for fine-tuning not only YopE activity but also the entire Yersinia virulence, since YopE also acts as a negative regulator for Yop translocation during infection via a yet unknown mechanism.Citation65,66 Interestingly, some Yersinia strains even secrete a chromosomally-encoded, T3SS-independent A-B toxin, the ‘cytotoxic necrotizing factor of Yersinia’ (CNF-Y), which counteracts YopE-mediated inactivation of RhoA and Rac1 and therefore promotes Yop translocation.Citation67,68
By inhibiting the important Rac1 pathway, YopE not only attenuates phagocytosis and Yop translocation, but also contributes to the general immunomodulatory activities of the Yersinia outer proteins. In epithelial cells, the response to translocon integration is mainly initiated through RhoA signaling.Citation69 Following integrin-mediated signaling, Rac1 can activate the MAPKs p38 and JNK, leading to IL-8 production,Citation70,71 which was found to be inhibited by YopE.Citation72 Furthermore, Rac1 can trigger caspase-1-dependent IL-1β maturation, which is also inhibited by YopE.Citation73 In addition, several Rho-GTPases are involved in the production of reactive oxygen species (ROS)Citation74,75 and by inhibiting these enzymes, YopE was found to reduce ROS levels in macrophages, which appears to be a pre-requisite for splenic colonization by Yersinia.Citation76 Polymorphonuclear neutrophils (PMNs), another important cell type for defense against Yersinia infections,Citation77 are also inactivated by YopE in vivo.Citation78 On the other hand, sustained inactivation of Rho-GTPase function by YopE might be sensed as a danger signal by macrophages, leading to increased killing of intracellular bacteria.Citation79 Further studies showed that chemical or genetic inactivation of RhoA and Rac1 even leads to higher levels of secreted pro-inflammatory cytokines after additional TLR stimulation,Citation80,81 again indicating that a prolonged action of YopE might not be favorable.
Potential therapeutic uses
Based on the molecular mechanism described above, potential areas of medical application for a recombinant, cell-penetrating YopE protein are inflammatory bowel diseases (IBD, ). This conglomerate of several auto-inflammatory disorders of the gastrointestinal tract is among others characterized by massive infiltration and pro-inflammatory activity of neutrophils.Citation82,83 By hitting Rho-GTPases, YopE not only attenuates the production of IL-8 (CXCL8), an important chemoattractant and activator for neutrophils,Citation84 but should also severely affect neutrophil migration, which is largely dependent on Rho-mediated signaling.Citation85 An increasing number of research projects is directed toward an inhibition of neutrophil migration and/or activity as well as modulation of Rho-GTPases for the treatment of IBD or auto-inflammatory disorders in general.Citation85-89
Figure 2. Overview of potential therapeutic uses of Yops. The most promising therapeutic application of YopM is the treatment of the auto-inflammatory diseases such as psoriasis, rheumatoid arthritis (RA), and inflammatory bowel diseases (IBD). Based on the molecular mechanism described before, potential areas of medical application for a recombinant, cell-penetrating YopE protein are IBD. YopT and especially its downstream target Rho-associated protein kinase ROCK are involved in several disease patterns, often within the cardiovascular field e.g., arteriosclerosis but also erectile dysfunction and traumatized neurons might be a target for a cell-penetrating YopT. A cell-penetrating effector YopO might be beneficial for the treatment of diseases associated with hyperactivated Rho-GTPases similar to YopE and YopT, but also for targeting mediators of auto-immune diseases like inflammatory bowel diseases. YopJ and its impact on signaling cascade displays potential therapeutic potential for inflammatory disorders, such as Psoriasis, RA, and IBD, but also for cancer control. RA also appears to be a promising area of application for recombinant YopH. Moreover, cancer progression also relies on signaling pathways tackled by the effector protein. The figure was produced using Servier Medical Art.
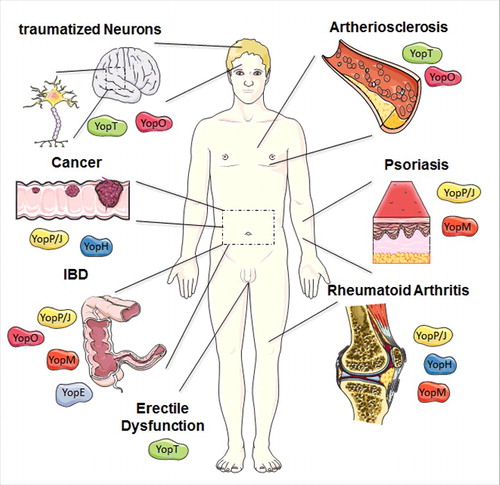
In line with this, YopE – together with YopT – has been proposed as an inhibitor of caspase-1 activation, since chemical inhibitors of Rho-GTPases also had a negative effect on caspase-1. Indeed, transfected YopE or YopT was able to block IL-1β secretion by HEK293T cells through inhibition of the Rac1-LIMK-1-pathway, leading to impaired activation of caspase-1.Citation90 This finding led to a patent for the treatment of caspase-1 related diseases, such as inflammatory bowel disease, Crohn's disease, or rheumatoid arthritis, with YopE and/or YopT.Citation90
YopT – An irreversible inhibitor of Rho-GTPases
Structure and function
In host cells, YopT, a 36 kDa cysteine protease, induces a very similar phenotype as YopE.Citation91 Accordingly, YopE and YopT share the same intracellular targets, however, they have different affinities and follow different mechanisms. In vitro, YopT cleaves the small Rho-GTPases RhoA, RhoG, Rac1 and Cdc42 directly in front of the C-terminal CAAX motif (C = cysteine; A = aliphatic aa; X = any aa), which carries the isoprenyl membrane anchor ().Citation92 In contrast to YopE, YopT therefore leads to an irreversible inhibition of Rho-GTPases by inducing their redistribution from the membrane to the cytosol where they do no longer come into contact with their likewise membrane-associated interaction partners.Citation93 The cleavage occurs regardless of the activation state of the Rho-GTPases,Citation93 but is dependent on preceding endoproteolytic processing of the CAAX motif by Rce1, which removes the AAX residues.Citation94 In vivo, the preferred target of YopT is RhoA.Citation95
YopT is the prototype of a new protein fold subfamily of proteases, which among others encompasses toxin B from E. coli or the avirulent protease AvrPphB of the plant pathogen Pseudomonas syringae.Citation96 This classification was based on predictions and homology analysis, although the actual 3D-structure of YopT has not been resolved yet. However, the crystal structure of the subfamily member AvrPphB revealed a papain-like core.Citation97 Consequently, given the relatedness of YopT to AvrPphB, the in vitro activity of YopT can be blocked by E64, an inhibitor of papain-like proteases.Citation96
Although YopT inactivates roughly the same pathways and leads to a comparable (yet milder) phenotype as YopE, it cannot fully replace YopE's anti-phagocytic and anti-inflammatory effects. Furthermore, when YopE is present, YopT is even dispensable for colonization in vivo.Citation72 In line with these results, some strains of Y. pseudotuberculosis do not even encode a functional copy of YopT.Citation91
Potential therapeutic uses
Hyperactivated RhoA – in macrophages and endothelial cells apparently the main target of YopT −95 and especially its downstream target Rho-associated protein kinase ROCK are involved in several disease patterns, often within the cardiovascular field. A considerable number of studies already investigated small molecule or endogenous inhibitors of RhoA or ROCK for treatment of e.g., arteriosclerosis (). An important signaling axis in this respect is the negative regulation of eNOS (endothelial nitric oxide synthase) synthesis involving RhoA and ROCK. Sildenafil, a standard therapeutic for erectile dysfunction in the context of diabetes mellitus, acts by stabilizing the NO induced second messenger cGMP, resulting in vasodilatation and prolonged penile erection.Citation98 The same effect was observed in rats treated with a ROCK inhibitor.Citation99 In contrast to other indications, where a (unfavorable) systemic treatment with a cell-penetrating variant of YopT would be necessary, treatment of erectile dysfunction holds the possibility of a topical treatment with potentially less systemic side effects than even the established oral therapeutics.
Furthermore, local ROCK inhibition was able to decrease inflammation in inflamed synovial tissues in rheumatoid arthritis.Citation100 Finally, Tan et al. and Lord-Fontaine et al. developed a cell-penetrating RhoA inhibitor based on the C3 toxin from Clostridium botulinum and demonstrated in vitro and in vivo its potential use for regeneration of traumatized neurons, which is strongly retarded by active RhoA ().Citation101,102 Also patients suffering from Alzheimer's disease might eventually profit from a downregulation of the RhoA/ROCK pathway.Citation103
A cell-penetrating variant of YopT might thus be a valuable tool for the treatment of pathologies caused by RhoA hyperactivation. Topical treatment of erectile dysfunction, local treatment of traumatized neurons or inflamed synovial tissues would present interesting options for therapeutic applications here. Still, one has to consider that a more specific inhibition of the ROCK pathway would be superior in most cases.
YopO – A multidomain effector protein
Structure and function
The anti-phagocytic effector YopO (also called Yersinia protein kinase A, YpkA, in Y. pseudotuberculosis and Y. pestis) was the first bacterial kinase to be described as a secreted virulence factor.Citation104 Moreover, it shares striking sequence homologies with catalytic motifs of known eukaryotic Ser/Thr kinases, such as protein kinases A and C (PKA and PKC) or casein kinase 2 (CK2).Citation104
YopO is secreted via the T3SS in an inactive state and is redirected by its N-terminal sequence to the host cell plasma membrane, where binding to an actin monomer leads to auto-phosphorylation and activation of the kinase domain.Citation105-107 To date, the α subunit of a heterotrimeric G protein, Gαq, which controls activation of phospholipase C,Citation108 as well as several regulators of actin polymerization including the vasodilator-stimulated phosphoprotein (VASP),Citation109 the Wiskott-Aldrich Syndrome protein (WASP), the Ena/VASP-like protein (EVL), gelsolin, and the formin diaphanous 1 were identified as direct targets of YopO ().Citation107 By intervening with the regulation of actin polymerization, YopO activity leads to disappearance of stress fibers and rounding of the cells.Citation110 Disruption of the actin cytoskeleton drastically impairs the phagocytosis of bacteria by macrophages – probably the most important function of YopO during infection.Citation106
However, using transfected Henle407 cells as well as yeast cells, it was shown that loss of kinase activity in YopO only attenuates—but not fully abolishes—its ability to disrupt actin polymerization.Citation110,111 This is due to a second functional domain in YopO that resembles eukaryotic GDP dissociation inhibitor (GDI) domains, which associate with GDP-bound small Rho-GTPases, thereby keeping them in an inactive state.Citation112 Indeed, YopO was found to interact directly with RhoA and with slightly higher affinity with Rac1 and Rac2, additional regulators of actin dynamics, and this interaction greatly contributes to the actin destabilizing effect of YopO.Citation112
Thus, YopO comprises three domains (membrane localization, Ser/Thr kinase, and GDI domain) that act synergistically in order to prevent phagocytosis of the invading bacteria by host cells. The importance of this mechanism was shown in animal experiments, where mutants of Yersinia pseudotuberculosis expressing truncated versions of YopO were almost completely attenuated in their virulence.Citation104
Taken together, pathogenic Yersinia manipulate Rho-GTPase signaling via four different mechanisms: acceleration of GTP conversion (YopE), inhibition of GDP dissociation (YopO), release of Rho-GTPases from the membrane (YopT) and deamidation of a catalytic glutamine residue (CNF-Y). This illustrates the importance of RhoGTPases in the defense against invading Yersinia, which includes organization of phagocytosis,Citation113 activation of MAPK-dependent IL-8 production,Citation70,71 caspase-1 dependent IL-1β maturation,Citation73 and the production of reactive oxygen species (ROS) among others.Citation74,75
Potential therapeutic uses
YopO targets both Rho-GTPases as well as Gαq. Examples of diseases associated with hyperactivated Rho-GTPases have been mentioned in the YopE and YopT sections above (). By hitting Gαq in addition, YopO could, however, also have adverse effects. There is growing evidence that Th17 cells are crucial mediators in auto-immune diseases like inflammatory bowel diseases ().Citation114 Gαq activity in turn has lately been linked to reduced differentiation of Th17 cells and disease progression.Citation115 Thus, YopO might have beneficial effects regarding neutrophils in this disease background, but adverse effects regarding Th17 cells. Gαq is also known to play a role in hypertension and the formation of thrombi, and inhibitors of Gαq are already under investigation for these indications.Citation116,117 Being of bacterial origin, a recombinant, cell-penetrating YopO would, however, have to face once more the challenge of systemic application without inducing an acute immune reaction.
YopP – A highly potent anti-inflammatory effector protein
Structure and function
YopJ/P (termed YopJ in Y. pestis and Y. pseudotuberculosis and YopP in Y. enterocolitica) is probably the most effective Yop in terms of suppressing pro-inflammatory signaling pathways in host cells. At the same time, many different isoforms of YopJ/P have been described, which differ in their translocation and/or substrate binding efficiencies and therefore in their contributions to virulence.Citation118-121 In a mouse infection model, Y. pestis EV76 strains which expressed either YopJ or YopP, exhibited remarkable differences in virulence.Citation122 Hence, general statements concerning the actions of YopJ/P have to be taken with caution. Known targets and effects are listed in .
Table 1. Known functions and molecular targets of YopJ/P sorted by host cell types and stimuli. Unless stated otherwise, all listed targets are negatively regulated by YopJ/P. Targets for which direct interaction with YopJ/P was not shown in the respective references, are marked with (?).
Especially in the domains harboring the catalytic center, YopJ/P shares structural (but not sequence) homology to Clan CE cysteine proteases, which encompass many de-ubiquitinating and de-sumoylating enzymes.Citation123 Indeed, recombinant YopJ was shown to be able to cleave ubiquitin chains (but not SUMO modifications) from an artificial substrate in vitro, and cells expressing YopJ contained lower levels of ubiquitinated TRAF2, TRAF6, the inhibitor of κB α (IκBα) as well as the stimulator of interferon genes (STING).Citation124,125 This observation was confirmed in two additional studies for TRAF6 (plus adding TRAF3) in transfected as well as Y. enterocolitica-infected cells.Citation123,126 TRAF2 (downstream of the tumor necrosis factor-α-receptor (TNF-R)), TRAF6 (downstream of the toll like-receptor 4 (TLR-4), T- and B-cell receptor (TCR/BCR) as well as interleukin-1 receptor (IL1-R)), and STING (downstream of receptors for cytosolic DNA) get fully activated by K63-linked ubiquitination.Citation127-129 IκBα, in turn, upon activation is marked for proteasomal degradation by K48-linked ubiquitination, thus unmasking NF-κB, which then consequently can translocate into the nucleus to induce transcription of pro-inflammatory genes ().Citation130 The outcome of TRAF3 ubiquitination is highly dependent on the circumstances and may be pro- as well as anti-inflammatory.Citation131
However, direct de-ubiquitination of cellular proteins by YopJ/P has not been observed. In fact, there is increasing evidence of an intrinsic acetyltransferase activity of YopJ, which is specific for serine, threonine and lysine residues (). Experiments performed in vitro and in cellulo demonstrated acetylation of the activation loop of MAPK kinases 2 and 6 (MEKs 2/6).Citation132,133 In co-transfection experiments, MEKs 4 and 7, transforming growth factor β-activated kinase 1 (TAK1; a MAPK kinase kinase), RICK (a caspase recruitment-domain containing kinase), and IκB kinase (IKK) were also found to be acetylated on critical residues in the presence of catalytically active YopJ.Citation132,134,135 Inhibition of TAK1 was likewise demonstrated for YopP, although it was not able to bind TAK1 in vitro but rather bound to TAB1, a regulatory protein in active TAK1 complexes.Citation136 Since YopJ in addition directly binds MEKs 1/3/5,Citation137 which also contain Ser and Thr residues in their activation loops, these kinases might also be acetylated by YopJ/P. Binding of inositol hexakisphosphate (IP6) to YopJ induces conformational changes resulting in the formation of an acetyl-CoA binding pocket, which greatly boosts acetyltransferase activity in vitro as well as inside the host.Citation138-140
In summary, it is intriguing to see that YopJ/P apparently is able to block activation of central pro-inflammatory pathways at three consecutive levels: (1) TRAF2 and TRAF6, which are among the first proteins to be activated by their respective receptors; (2) TAK1, a MAPKKK which is activated (directly and indirectly) by TRAF2 and TRAF6; (3) the downstream MAPKKs 4, 6, 7, as well as IKK/IκBα, which in turn control the activation of the MAPKs p38 and c-jun amino-terminal kinase (JNK), or NF-κB respectively – all of which can finally elicit a strong inflammatory response (; pathways reviewed inref. 141). Inhibition of MAPKKs by YopJ was also proposed in studies using transfected yeast cells.Citation142 On the other hand, not all of these levels might be targeted by all YopJ isoforms and not in all cell types.Citation143
In line with these findings on intracellular signaling, key pro-inflammatory cytokines are downregulated by YopJ/P. This includes inhibition of IL-8 transcription in human embryonic kidney (HEK) cells by YopP after MAPKKK overexpression or IL-1 stimulation and by YopJ after TLR-2 or TLR-4 activation,Citation123,136 inhibition of IL-6 and IL-8 secretion by human umbilical vein endothelial cells (HUVECs) after YopP translocation,Citation144 as well as reduction of TNF-α secretion by murine macrophages caused by YopP.Citation145 Furthermore, antigen uptake by dendritic cells and expression of intercellular adhesion molecule 1 (ICAM1) in endothelial cells might also be negatively regulated by YopP.Citation144,146
Apart from these strong anti-inflammatory effects of YopJ/P, probably the most captivating feature of YopJ/P is the induction of cell death in macrophages and dendritic cells (YopP only), but not in epithelial or natural killer cells.Citation119,147-153 Inhibition of the TLR-4, MAPK and NF-κB pathways is necessary for this effect,Citation154,155 which involves receptor-interacting protein 1 and 3 (RIP1/3) kinase-dependent activation of caspase-8, thus most probably triggering the extrinsic apoptosis pathway.Citation156,157
Paradoxically, activation of caspase-8 by YopJ/P also promotes activation of caspase-1, which is responsible for the maturation of the pro-inflammatory cytokines IL-1β and IL-18.Citation157 Nevertheless, a medium level of macrophage cytotoxicity was proven to be necessary for full Yersinia virulence in mouse models, whereas strongly pro-apoptotic YopJ/P isoforms or hypersecretion of YopJ/P impair virulence, just as YopJ/P null mutants do.Citation119,122 If it turns out that caspase-1 is indeed partially inactivated by (some isoforms of) YopM, this would be another interesting example for the interplay and fine-tuning of different Yersinia effector proteins.
Potential therapeutic uses
In rheumatoid arthritis (RA) over-activation of macrophages plays a decisive role. Specialized macrophages, termed osteoclasts, are necessary for bone homeostasis by degrading bone tissue, but sterile inflammation can cause a regulatory imbalance leading to excessive bone destruction.Citation158 TNF-α was identified as a central driver of these inflammatory reactions and is thus today the main therapeutic target in RA treatment, especially in the form of neutralizing antibodies.Citation158 In the inflammatory skin disorder psoriasis, macrophages were also suggested to play an important role in maintaining the inflammation status.Citation159,160 Psoriasis is a particularly interesting option for a treatment with bacteria-derived cell-penetrating proteins, since the site of inflammation can be reached easily by topical application, which means that potential detrimental side effects caused by a systemic distribution of such an exogenous protein are circumvented.
In the context of these diseases, intervention with a cell-penetrating rYopP may have several benefits, as it impairs TNF-α-induced signaling as well as NF-κB- and MAPK-driven TNF-α secretion, and most importantly, it triggers apoptosis in activated macrophages, which are the primary source of TNF-α.Citation158 Hence, rYopP would cut inflammation at an earlier stage than e.g., neutralizing antibodies, which might be more efficient and especially more sustaining. Furthermore, one would not need a stoichiometric amount of the therapeutic biologic (one neutralizing antibody may only bind two TNF-α molecules), but could use much less, which in turn might be advantageous further in terms of minimizing side effects.
This high therapeutic potential of YopJ/P had attracted already some attention. About 20 years ago, Pettersson and Wolf-Watz filed a patent for the delivery of YopJ by engineered bacteria for the treatment of inflammatory disorders of the gut with extensive IL-8 expression.Citation161 Wallach and Appel developed a YopP-fusion protein encompassing a TNF-R binding peptide and an endosomal escape sequence for efficient delivery of YopP into activated macrophages, which express high amounts of the TNF-R.Citation162 Another group constructed self-assembling YopJ-nanoparticles which readily translocated into and killed human breast cancer cells.Citation163 Hence, with increasing options for engineering also targeted cell-delivery of YopJ/P, further possible applications will be developed ().
YopH – A versatile phosphotyrosine phosphatase
Structure and function
Phosphorylation of either tyrosine, serine or threonine residues is frequently utilized by eukaryotic cells for signal transduction and thus, pathogenic bacteria have evolved an astonishing arsenal of phosphatases to manipulate these signaling processes in their favor.Citation164 With YopH, Yersinia secrete a highly potent and versatile phosphotyrosine phosphatase.Citation165 It consists of three major domains: an N-terminal domain (aa 1–129) that includes the secretion and translocation signal as well as a chaperone binding region,Citation166,167 a proline-rich repeat (aa 130–192), and a catalytic C-terminal domain (aa 193–468), which comprises all invariant features of eukaryotic phosphatases of the PTPB1 family.Citation168 The catalytic center forms a so-called P-loop (phosphate-binding loop) with the typical HC(X)5R(S/T) motive, which contains the catalytic active residues C403 and R409, which upon ligand binding get in close proximity to D356 to build a catalytic triad.Citation169 D356 is part of a second, highly flexible structure termed ‘WPD loop’.Citation168,170 Mutation of C403 or D356 to either serine or alanine renders YopH inactive.Citation171,172 Residues important for substrate binding were found in the N-terminal as well as the C-terminal domains.Citation173,174
Many – yet most probably not all – direct and indirect targets of YopH in several different cell types have been proposed or identified to date (). As with YopM and YopJ/P, in evaluating these results one has to carefully take into account the particular Yersinia species and experimental set-up (in vitro vs. in vivo, cell types, mouse models, way of YopH delivery, etc.) before reaching more general conclusions. For example, YopH of Y. enterocolitica was reported to dampen phagocytosis in murine dendritic cells,Citation175 while no such effect was observed for YopH of Y. pseudotuberculosis.Citation176 The presumably cell-penetrating construct ANT-YopH, used at a concentration of up to 300 µg/mL by Alonso et al., blocked T-cell activity in vitro, but was later also shown to induce the intrinsic apoptosis pathway independent of YopH activity.Citation177
Table 2. Known functions and molecular targets of YopH sorted by Yersinia species, host cell types and stimuli. Unless stated otherwise, all listed targets are negatively regulated by YopH. Ag = antigen, DC = dendritic cell, hum. = human, mur = murine, ROS = reactive oxygen species, TCR = T-cell receptor.
Notably, all known interaction partners of YopH are associated with contact-dependent signaling via integrins or the T-cell receptor (TCR), which is mainly transduced by non-receptor tyrosine kinases of the src family.Citation178 In particular, most of the target proteins (p130cas, FAK, Fyb, Paxillin, SKAP-HOM, SLP-76, PRAM-1) are part of focal adhesion or focal adhesion-like complexes (), which are an important part of integrin and TCR signaling.Citation179,180 During infection, Yersinia tightly bind to β1-integrins via their outer membrane adhesin invasin.Citation181 Host cells decode this binding as a danger signal that promotes phagocytosis,Citation182 inflammasome activation,Citation183 and secretion of several pro-inflammatory cytokines.Citation184 Therefore, it is of great importance for the invading Yersinia to shut down this signaling axis.
In a murine infection model, enzymatically active YopH was found to be sufficient for successful colonization of the spleen by intravenously injected Y. pseudotuberculosis mutants.Citation185 Intranasally administered Y. pestis lacking functional YopH effectively colonized the lung, but were not able to spread to the spleen and lungs of infected mice or to prevent early cytokine responses.Citation186 This observation was mainly linked to the inactivation of neutrophils by YopH, although YopE could fully complement a loss of YopH in one study.Citation78 A more recent study showed that YopH-deficient Y. enterocolitica mutants were not able to block neutrophil recruitment into Peyer's patches of living mice.Citation187 Currently it is not clear whether an interruption of the T-cell receptor signaling pathway is advantageous for invading Yersinia. In intragastrically infected mice, a virulence plasmid-cured Y. pseudotuberculosis strain readily colonized lymphatic tissues, where it even associated with T- and B-lymphocytes.Citation188 On the other hand, CD8+ T-cells were found to be important for the clearance of repeated Y. pseudotuberculosis infections.Citation189
In times of recurring endemic outbreaks and an increasing awareness of potential bioterroristic attacks, YopH lately became a highly studied target for the treatment of especially Y. pestis infections through small molecule inhibitors of YopH.Citation190-193 Finally, recent data showed that – at least in pathogenic E. coli – bacterial proteins involved in the regulation of virulence, including type III secretion, are also activated by tyrosine phosphorylation – a mechanism that was long believed to be completely absent in bacteria.Citation194 Whether YopH might thus also play a regulatory role within the bacterial cell is an exciting topic for future research.
Potential therapeutic uses
Tyrosine phosphorylation is part of many signaling pathways and thus dysregulation of this mechanism might be involved in a wide range of diseases. In particular, integrin and growth factor receptor signaling rely on tyrosine phosphorylation, which in turn is partly shut down by YopH. Due to the apparent ubiquitous presence of tyrosine phosphorylation in signaling pathways, two selected possible fields of application for a local treatment with a cell-penetrating form of the phosphotyrosine phosphatase YopH are discussed in the following.
First, rheumatoid arthritis (RA) appears to be a promising area of application (). As already mentioned, the gold standard for treatment of RA is simple blockage of the central mediator of inflammation, tumor necrosis factor α (TNF-α), e.g., by antibodies.Citation158 Such a therapy was found to effectively reduce bone erosion, but not the underlying inflammation.Citation195 Therefore, eliminating the source of pro-inflammatory cytokines might be a better approach. In the case of RA, the sources of pro-inflammatory cytokines are mainly hyperactivated monocytes and their differentiated descendants such as macrophages and especially osteoclasts, which in the end cause bone erosion.Citation158 Due to its versatility, YopH could counteract this series of events on several levels:
YopH reduces the activation of the Akt pathway in macrophages, probably by blocking the integrin receptor-mediated activation of the phosphoinositide-3-kinase (PI3K) signaling.Citation196 Recent studies showed improved health when this signaling axis was blocked in murine models of psoriatic arthritis.Citation197
p130cas, probably the best studied direct target of YopH, is essential for actin-remodeling after contact-dependent activation of osteoclasts.Citation198 This remodeling in turn is essential for the bone destructing activity of osteoclasts.Citation199
Finally, contact-dependent activation of T-cells by monocytes plays an important role in the persistence of inflammation in RA – a fact that is already successfully exploited for therapy.Citation158 YopH in turn is very effective in shutting down T-cell responses to antigen stimuli.Citation172,196,200
Blocking just one of the above mentioned pathways would probably not be sufficient to achieve a satisfactory RA treatment. Unlike most small molecule inhibitors, YopH might however be able to block all of them simultaneously, making it a highly potent candidate therapeutic. Especially interesting could be a combination with YopP, which blocks central inflammatory signaling pathways causing macrophage cell death.
Second, cancer progression also relies on signaling pathways described above (). Integrin signaling via focal adhesions promotes cell survival and can protect so-called cancer stem cells from therapeutic intervention.Citation201 Focal adhesion kinase (FAK) is found overexpressed and hyper-phosphorylated in many types of cancers, where it facilitates angiogenesis as well as cell migration and proliferation (and thereby metastasis).Citation202,203 Hyperactivated SKAP-HOM was linked to increased invasion of tumor-associated macrophages, which create a pro-metastatic environment for the tumor cells.Citation204 Finally, p130cas as a central mediator of integrin and growth factor receptor signaling got into the focus of cancer research recently. Especially in HER2-positive breast cancer, there is increasing evidence that over-activated p130cas plays a major role in promoting cell survival, proliferation and spreading.Citation205
As for RA, YopH (targeted by homing sequences or injected directly into the tumor mass) might be able to undermine tumor progression on several levels simultaneously. On the other hand, though, it would also be able to suppress beneficial tumor infiltrating T-cells.Citation206 This versatility might be seen as a general drawback of YopH for a possible role as a novel biologic. In addition, overshooting PTPase activity has also been linked to cancer progression and other disease patterns,Citation207 which illustrates the delicate balance that has to be maintained and/or restored by therapeutic interventions.
LcrV (V-antigen) – An essential multifunctional virulence factor with immunosuppressive properties
Structure and function
Low-calcium response protein V (LcrV or V-antigen, 37–39 kDa) has been identified first in Y. pestis more than 50 years ago.Citation208 Subsequently, this plasmid-encoded secreted protein was also identified in all human pathogenic Yersinia (for recent review see ref. Citation209). Interestingly, homologs of LcrV are also expressed by several other bacteria employing a T3SS such as P. aeruginosa, V. cholerae, Photorhabdus luminescens and Aeromonas spp.Citation209 However, quite surprisingly neither any possible intracellular target(s) nor an enzymatic activity has been associated with LcrV yet. Furthermore, LcrV has been intensely studied as part of candidate vaccines against Yersinia infections and has been shown to confer protection in animal models.Citation210-212
LcrV has been identified as a multifunctional virulence factor exhibiting characteristics of translocator and effector proteins. LcrV is involved in the regulation of Yop production,Citation213 the translocation of virulence proteins by contributing to the needle tip,Citation214,215 and to pore formation in the target cell membrane since it facilitates insertion of YopB and YopD in host cell membranes.Citation216,217 Just recently, LcrV has been described in Y. pseudotuberculosis to be required for the early targeting of YopH in vivo.Citation218 LcrV is secreted by the T3SS and has been detected on the bacterial surface and in the cytosol of target cells.Citation219 Apparently, LcrV enters host cells independently of T3S and the YopB–YopD pore, however, appears to need direct contact of Yersinia with the target cell since extracellularly added LcrV is not able to enter cells.Citation219
Nevertheless, extracellularly added, recombinant LcrV has been found to exert also immunosuppressive properties. Treatment of mice with rLcrV leads to suppression of TNFα and IFNγ via amplification of IL-10 and inhibition of neutrophil chemotaxis.Citation220-223 Further, in murine peritoneal macrophages production of TNFα, IFNγ, IL-12, IL-1β, IL-6, MCP-1, MIP-1α, MIP-1β, and RANTES were inhibited by rLcrV (and rYopB).Citation224 Interactions of rLcrV with CD14 and TLR2 lead to the secretion of IL-10 in stimulated cells such as macrophages and a general hypo-responsiveness of other TLRs. This effect can also be induced by a short conserved N-terminal peptide of LcrV (VLEELVQLVKDKKIDISIK).Citation225 The importance of these findings is further corroborated by the reduced susceptibility for oral Yersinia infection of TLR2-deficient mice, which in contrast to wild-type mice are capable to resolve an infection.Citation225
Potential therapeutic uses
Although unmodified LcrV of Y. pestis has been reported to be an extremely unstable protein,Citation226 it can be produced from Y. enterocolitica as recombinant (e.g., His-tagged) protein in sufficient amounts for therapeutic applications.Citation227 As the effects of LcrV appear to be mainly based on the enhanced production of anti-inflammatory IL-10, possible applications might be directed primarily to the management of infection-associated immunopathology, autoimmunity, or allergy.Citation228 In fact, IL-10 itself has been tested since its discovery in patients suffering from Crohn's disease, rheumatoid arthritis, psoriasis, hepatitis C infection, HIV infection and for the inhibition of therapy-associated cytokine release associated with organ transplantation.Citation229 Although systemic applications showed promising results in early clinical trials – e.g., in Crohn's disease patients – other immune pathologies were not as susceptible to IL-10 treatment, which probably might also be caused by IL-10′s role as a regulatory cytokine which is influenced further by the site of expression and the cell type.Citation230-232 In this respect, topical application of rLcrV might be a suitable strategy to induce targeted, site-specific IL-10 secretion for the treatment of autoimmune disorders. At present, however, studies addressing these potential applications of LcrV have not been reported.
Conclusion
During the long period of coevolution mainly pathogenic bacteria have developed perfectly adapted effector proteins for manipulating cellular responses and the human immune system in their favor. As we are uncovering more and more molecular details of these interactions we might be able to exploit the successful ‘research and development’ of these bacterial pathogens and produce our own ‘biosimilars’. The six plasmid-encoded Yersinia outer proteins and LcrV described in this review target several important regulators in different pathways (e.g., Rho-GTPases, MAPKs, or mediators of integrin signaling; ), which are dysregulated in major human diseases such as inflammatory bowel diseases, rheumatoid arthritis, psoriasis, or cancer ().
Potentially, the addition of further targeting sequences to either autonomously cell-penetrating effectors (CPE) or effectors combined with cell-penetrating peptides could enable the delivery of recombinant Yops and also of LcrV at specific sites and into specific host cells and, eventually, even host cell organelles of interest. Such targeting might make these novel biologics more efficient and less toxic than conventional drugs, which are often less selective and thus have higher EC50. Furthermore, bacterial effector proteins can target intracellular proteins for which no satisfactory chemical inhibitor is available. This would provide a novel, vast pool of innovative candidate therapeutical biologics. Besides, such constructs could be interesting for basic research as well to specifically modulate proteins and pathways of interest. YopH for example has already been suggested as a tool in kinase research.Citation233
However, not every level of interaction between Yops and host proteins has been elucidated to date. This bears the problem of potential unwanted side effects due to modulation of yet unknown intracellular targets by cell-penetrating Yops. Moreover, Yersinia outer proteins are very efficient in silencing anti-bacterial responses of eukaryotic cells, but as they affect many signaling pathways in parallel, their use as a specific therapeutic has to be cautiously explored. On the other hand, as illustrated for the possible role of YopH in the treatment of rheumatoid arthritis, inhibiting more than one pathway can also be an advantage over common standard therapies. Certainly, further thorough and diligent investigations including animal studies are needed to identify and evaluate the severity of probable side effects in relation to the therapeutic benefits of these novel biologics.
Furthermore, bacteria-derived protein therapeutics face similar safety issues as reported for any other drug delivery system.Citation234,235 In this regard, several limitations such as poor serum stability, cytotoxicity, and immunogenicity of conventional CPEs need to be optimized or to be considered in the choice of preferred application routes to expand their usefulness for biomedical applications. Especially the usually pronounced immunogenicity of bacterial CPEs might cause substantial drawbacks for systemic applications and might limit therapeutic options mainly to topically accessible diseases. Concerning serum stability and other safety issues, the field of CPEs can certainly profit from the extensive research on those aspects for other protein therapeutics. For example, Pan et al. developed a strategy to enhance serum stability of a CPP-RNA conjugate by coupling it to diethylene glycol (DEGylation),Citation236 similar to the attachment of polyethylene glycol (PEGylation) to conventional protein therapeutics.
Apart from such obstacles, several patents and ongoing studies on the use of Yops and also other bacterial effector proteins as innovative biologics testify to the appealing nature of this strategy. Further research on the role of Yops during infection will also enhance and strengthen our knowledge base for this translational approach.
Disclosure of potential conflicts of interest
No potential conflicts of interest were disclosed.
Acknowledgments
We like to thank all our coworkers at the Institute of Infectiology - ZMBE for their valuable contributions and helpful discussions. Further, we need to apologize to all our colleagues whose excellent work could not be mentioned or cited due to space limitations.
Funding
Work from our own group has in part been supported by grants from the Deutsche Forschungsgemeinschaft (RU 1884/2-1; RU 1884/3-1; SFB1009 TP B03, Graduiertenkolleg GRK 1409, Cells-in-Motion Cluster of Excellence (EXC 1003 – CiM)) and by a grant from the Interdisciplinary Center for Clinical Research (IZKF, Rüt2/002/16) of the Medical Faculty of the University of Münster.
References
- Akopyan K, Edgren T, Wang-Edgren H, Rosqvist R, Fahlgren A, Wolf-Watz H, Fallman M. Translocation of surface-localized effectors in type III secretion. Proc Natl Acad Sci U S A 2011; 108(4):1639-44; PMID:21220342; http://dx.doi.org/10.1073/pnas.1013888108
- Dean P. Functional domains and motifs of bacterial type III effector proteins and their roles in infection. FEMS Microbiol Rev 2011; 35(6):1100-25; PMID:21517912; http://dx.doi.org/10.1111/j.1574-6976.2011.00271.x
- Portaliou AG, Tsolis KC, Loos MS, Zorzini V, Economou A. Type III secretion: Building and operating a remarkable nanomachine. Trends Biochem Sci 2016; 41(2):175-89; PMID:26520801; http://dx.doi.org/10.1016/j.tibs.2015.09.005
- Santos AS, Finlay BB. Bringing down the host: Enteropathogenic and enterohaemorrhagic Escherichia coli effector-mediated subversion of host innate immune pathways. Cell Microbiol 2015; 17(3):318-32; PMID:25588886; http://dx.doi.org/10.1111/cmi.12412
- Rüter C, Hardwidge PR. “Drugs from Bugs:” Bacterial effector proteins as promising biological (immune-) therapeutics. FEMS Microbiol Lett 2013; 351(2):126-32; PMID:24261744; http://dx.doi.org/10.1111/1574-6968.12333
- Rüter C, Schmidt MA. Cell-penetrating bacterial effector proteins: Better tools than targets. Trends Biotechnol 2017; 35(2):109-20; PMID:27592802; http://dx.doi.org/10.1016/j.tibtech.2016.08.002
- Michgehl S, Heusipp G, Greune L, Rüter C, Schmidt MA. Esp-independent functional integration of the translocated intimin receptor (Tir) of enteropathogenic Escherichia coli (EPEC) into host cell membranes. Cell Microbiol 2006; 8(4):625-33; PMID:16548888; http://dx.doi.org/10.1111/j.1462-5822.2005.00655.x
- Cirl C, Wieser A, Yadav M, Duerr S, Schubert S, Fischer H, Stappert D, Wantia N, Rodriguez N, Wagner H, et al. Subversion of toll-like receptor signaling by a unique family of bacterial Toll/interleukin-1 receptor domain-containing proteins. Nat Med 2008; 14(4):399-406; PMID:18327267; http://dx.doi.org/10.1038/nm1734
- Waldhuber A, Snyder G, Römmler F, Cirl C, Müller T, Xiao T, Svanborg C, Miethke T. A comparative analysis of the mechanism of toll-like receptor-disruption by TIR-containing protein C from uropathogenic Escherichia coli. Pathogens 2016; 5(1):25; PMID:26938564; http://dx.doi.org/10.3390/pathogens5010025
- Stolle A. The recombinant EPEC effector NleC follows uptake strategies similar to short-trip AB toxins and displays immunosuppressive features by cleaving p65 [Dissertation]. Münster, Germany: Westfälische Wilhelms-Universität; 2016
- Lubos M, Norkowski S, Stolle A, Langel Ü, Schmidt MA, Rüter C. Analysis of T3SS-independent autonomous internalization of the bacterial effector protein SspH1 from Salmonella typhimurium. Inflamm Cell Signal 2014; (1):e423; http://dx.doi.org/10.14800/ics.423
- Lubos M, Schmidt MA, Rüter C, inventors; Westfälische Wilhelms-Universität Münster, assignee. Cell-penetrating bacterial E3-ubiquitin-ligases for use in immunotherapy. WO/2015/040215. 2015 Mar 26
- Rüter C, Buss C, Scharnert J, Heusipp G, Schmidt MA. A newly identified bacterial cell-penetrating peptide that reduces the transcription of pro-inflammatory cytokines. J Cell Sci 2010; 123(13):2190-8; PMID:20554895; http://dx.doi.org/10.1242/jcs.063016
- Scharnert J, Greune L, Zeuschner D, Lubos M, Schmidt MA, Rüter C. Autonomous translocation and intracellular trafficking of the cell-penetrating and immune-suppressive effector protein YopM. Cell Mol Life Sci 2013; 70(24):4809-23; PMID:23835836; http://dx.doi.org/10.1007/s00018-013-1413-2
- Rohde JR, Breitkreutz A, Chenal A, Sansonetti PJ, Parsot C. Type III secretion effectors of the IpaH family are E3 ubiquitin ligases. Cell Host Microbe 2007; 1(1):77-83; PMID:18005683; http://dx.doi.org/10.1016/j.chom.2007.02.002
- Dinca A, Chien W, Chin MT. Intracellular delivery of proteins with cell-penetrating peptides for therapeutic uses in human disease. Int J Mol Sci 2016; 17(2):263; PMID:26907261; http://dx.doi.org/10.3390/ijms17020263
- Kurrikof K, Gestin M, Langel Ü. Recent in vivo advances in cell-penetrating peptide-assisted drug delivery. Expert Opin Drug Deliv 2016; 13(3):373-87; PMID: 26634750; http://dx.doi.org/10.1517/17425247.2016.1125879
- Cornelis GR, Biot T, Lambert de Rouvroit C, Michiels T, Mulder B, Sluiters C, Sory MP, van Bouchaute M, Vanooteghem JC. The Yersinia yop regulon. Mol Microbiol 1989; 3(10):1455-9; PMID:2693899; http://dx.doi.org/10.1111/j.1365-2958.1989.tb00129.x
- Cornelis GR, Wolf-Watz H. The Yersinia Yop virulon: A bacterial system for subverting eukaryotic cells. Mol Microbiol 1997; 23(5):861-7; PMID:9076724; http://dx.doi.org/10.1046/j.1365-2958.1997.2731623.x
- Bliska JB. Yop effectors of Yersinia spp. and actin rearrangements. Trends Microbiol 2000; 8(5):205-8; PMID:10785635; http://dx.doi.org/10.1016/S0966-842X(00)01738-8
- Cornelis GR. The Yersinia Ysc-Yop ‘type III’ weaponry. Nat Rev Mol Cell Biol 2002; 3(10):742-52; PMID:12360191; http://dx.doi.org/10.1038/nrm932
- Viboud GI, Bliska JB. Yersinia outer proteins: Role in modulation of host cell signaling responses and pathogenesis. Annu Rev Microbiol 2005; 59:69-89; PMID:15847602; http://dx.doi.org/10.1146/annurev.micro.59.030804.121320
- Ruckdeschel K, Deuretzbacher A, Haase R. Crosstalk of signalling processes of innate immunity with Yersinia Yop effector functions. Immunobiology 2008; 213(3–4):261-9; PMID:18406372; http://dx.doi.org/10.1016/j.imbio.2007.11.001
- Matsumoto H, Young GM. Translocated effectors of Yersinia. Curr Opin Microbiol 2009; 12(1):94-100; PMID:19185531; http://dx.doi.org/10.1016/j.mib.2008.12.005
- Pha K, Navarro L. Yersinia type III effectors perturb host innate immune responses. World J Biol Chem 2016; 7(1):1-13; PMID:26981193; http://dx.doi.org/10.4331/wjbc.v7.i1.1
- Thorslund SE, Edgren T, Pettersson J, Nordfelth R, Sellin ME, Ivanova E, Francis MS, Isaksson EL, Wolf-Watz H, Fallman M. The RACK1 signaling scaffold protein selectively interacts with Yersinia pseudotuberculosis virulence function. PLoS One 2011; 6(2):e16784; PMID:21347310; http://dx.doi.org/10.1371/journal.pone.0016784
- Dewoody R, Merritt PM, Marketon MM. YopK controls both rate and fidelity of Yop translocation. Mol Microbiol 2013; 87(2):301-17; PMID:23205707; http://dx.doi.org/10.1111/mmi.12099
- Miao EA, Scherer CA, Tsolis RM, Kingsley RA, Adams LG, Baumler AJ, Miller SI. Salmonella typhimurium leucine-rich repeat proteins are targeted to the SPI1 and SPI2 type III secretion systems. Mol Microbiol 1999; 34(4):850-64; PMID:10564523; http://dx.doi.org/10.1046/j.1365-2958.1999.01651.x
- Wei C, Wang Y, Du Z, Guan K, Cao Y, Yang H, Zhou P, Wu F, Chen J, Wang P, et al. The Yersinia type III secretion effector YopM is an E3 ubiquitin ligase that induced necrotic cell death by targeting NLRP3. Cell Death Dis 2016; 7(12):e2519; PMID:27929533; http://dx.doi.org/10.1038/cddis.2016.413
- Evdokimov AG, Anderson DE, Routzahn KM, Waugh DS. Unusual molecular architecture of the Yersinia pestis cytotoxin YopM: A leucine-rich repeat protein with the shortest repeating unit. J Mol Biol 2001; 312(4):807-21; PMID:11575934; http://dx.doi.org/10.1006/jmbi.2001.4973
- Benabdillah R, Mota LJ, Lützelschwab S, Demoinet E, Cornelis GR. Identification of a nuclear targeting signal in YopM from Yersinia spp. Microb Pathog 2004; 36(5):247-61; PMID:15043860; http://dx.doi.org/10.1016/j.micpath.2003.12.006
- Boland A, Sory MP, Iriarte M, Kerbourch C, Wattiau P, Cornelis GR. Status of YopM and YopN in the Yersinia Yop virulon: YopM of Y. enterocolitica is internalized inside the cytosol of PU5-1.8 macrophages by the YopB, D, N delivery apparatus. EMBO J 1996; 15(19):5191-5201; PMID:8895564
- Höfling S, Scharnert J, Cromme C, Bertrand J, Pap T, Schmidt MA, Rüter C. Manipulation of pro-inflammatory cytokine production by the bacterial cell-penetrating effector protein YopM is independent of its interaction with host cell kinases RSK1 and PRK2. Virulence 2014; 5(7):761-71; PMID:25513777; http://dx.doi.org/10.4161/viru.29062
- Höfling S, Grabowski B, Norkowski S, Schmidt MA, Rüter C. Current activities of the Yersinia effector protein YopM. Int J Med Microbiol 2015; 305(3):424-32; PMID:25865799; http://dx.doi.org/10.1016/j.ijmm.2015.03.009
- Reisner BS, Straley SC. Yersinia pestis YopM: Thrombin binding and overexpression. Infect Immun 1992; 60(12):5242-52; PMID:1452357
- Hines J, Skrzypek E, Kajava AV, Straley SC. Structure-function analysis of Yersinia pestis YopM's interaction with alpha-thrombin to rule on its significance in systemic plague and to model YopM's mechanism of binding host proteins. Microb Pathog 2001; 30(4):193-209; PMID:11312613; http://dx.doi.org/10.1006/mpat.2000.0424
- Heusipp G, Spekker K, Brast S, Fälker S, Schmidt MA. YopM of Yersinia enterocolitica specifically interacts with alpha 1-antitrypsin without affecting the anti-protease activity. Microbiology 2006; 152(5):1327-35; PMID:16622050; http://dx.doi.org/10.1099/mic.0.28697-0
- Cheng LW, Schneewind O. Yersinia enterocolitica TyeA, an intracellular regulator of the type III machinery, is required for specific targeting of YopE, YopH, YopM, and YopN into the cytosol of eukaryotic cells. J Bacteriol 2000; 182(11):3183-90; PMID:10809698; http://dx.doi.org/10.1128/JB.182.11.3183-3190.2000
- McDonald C, Vacratsis PO, Bliska JB, Dixon JE. The yersinia virulence factor YopM forms a novel protein complex with two cellular kinases. J Biol Chem 2003; 278(20):18514-23; PMID:12626518; http://dx.doi.org/10.1074/jbc.M301226200
- Hentschke M, Berneking L, Belmar Campos C, Buck F, Ruckdeschel K, Aepfelbacher M. Yersinia virulence factor YopM induces sustained RSK activation by interfering with dephosphorylation. PLoS One 2010; 5(10):e13165; PMID:20957203; http://dx.doi.org/10.1371/journal.pone.0013165
- Chung LK, Park YH, Zheng Y, Brodsky IE, Hearing P, Kastner DL, Chae JJ, Bliska JB. The Yersinia virulence factor YopM hijacks host kinases to inhibit type III effector-triggered activation of the pyrin inflammasome. Cell Host Microbe 2016; 20(3):296-306; PMID:27569559; http://dx.doi.org/10.1016/j.chom.2016.07.018
- Berneking L, Schnapp M, Rumm A, Trasak C, Ruckdeschel K, Alawi M, Grundhoff A, Kikhney AG, Koch-Nolte F, Buck F, et al. Immunosuppressive Yersinia effector YopM binds DEAD Box helicase DDX3 to control ribosomal S6 kinase in the nucleus of host cells. PLoS Pathog 2016; 12(6):e1005660; PMID:27300509; http://dx.doi.org/10.1371/journal.ppat.1005660
- Chung LK, Philip NH, Schmidt VA, Koller A, Strowig T, Flavell RA, Brodsky IE, Bliska JB. IQGAP1 is important for activation of caspase-1 in macrophages and is targeted by Yersinia pestis type III effector YopM. mBio 2014; 5(4):e01402-14; PMID:24987096; http://dx.doi.org/10.1128/mBio.01402-14
- LaRock CN, Cookson BT. The Yersinia virulence effector YopM binds caspase-1 to arrest inflammasome assembly and processing. Cell Host Microbe 2012; 12(6):799-805; PMID:23245324; http://dx.doi.org/10.1016/j.chom.2012.10.020
- McCoy MW, Marré ML, Lesser CF, Mecsas J. The C-terminal tail of Yersinia pseudotuberculosis YopM Is critical for interacting with RSK1 and for virulence. Infect Immun 2010; 78(6):2584-98; PMID:20368345; http://dx.doi.org/10.1128/IAI.00141-10
- McPhee JB, Mena P, Bliska JB. Delineation of regions of the Yersinia YopM protein required for interaction with the RSK1 and PRK2 host kinases and their requirement for interleukin-10 production and virulence. Infect Immun 2010; 78(8):3529-39; PMID:20515922; http://dx.doi.org/10.1128/IAI.00269-10
- Kerschen EJ, Cohen DA, Kaplan AM, Straley SC. The plague virulence protein YopM targets the innate immune response by causing a global depletion of NK cells. Infect Immun 2004; 72(8):4589-4602; PMID:15271919; http://dx.doi.org/10.1128/IAI.72.8.4589-4602.2004
- Ye Z, Gorman AA, Uittenbogaard AM, Myers-Morales T, Kaplan AM, Cohen DA, Straley SC. Caspase-3 mediates the pathogenic effect of Yersinia pestis YopM in liver of C57BL/6 mice and contributes to YopM's function in spleen. PLoS One 2014; 9(11):e110956; PMID:25372388; http://dx.doi.org/10.1371/journal.pone.0110956
- Stasulli NM, Eichelberger KR, Price PA, Pechous RD, Montgomery SA, Parker JS, Goldman WE. Spatially distinct neutrophil responses within the inflammatory lesions of pneumonic plague. mBio 2015; 6(5):e01530-15; PMID:26463167; http://dx.doi.org/10.1128/mBio.01530-15
- Rüter C, Schmidt MA, inventors; Westfälische Wilhelms-Universität Münster, assignee. Yersinia Outer Protein M (YopM) in the Treatment of Psoriasis. US20160095898 A1 2014 Jul 30
- Rüter C, Heusipp G, Schmidt MA, inventors; Universitätsklinikum Münster, assignee. YopM as delivery vehicle for cargo molecules and as biological therapeutic for immunomodulation of inflammatory reactions. 2014 US8840901 B2
- Nograles KE, Krueger JG. Anti-cytokine therapies for psoriasis. Exp Cell Res 2011; 317(9):1293-1300; PMID:21300061; http://dx.doi.org/10.1016/j.yexcr.2011.01.024
- Yamauchi PS, Bissonnette R, Teixeira HD, Valdecantos WC. Systematic review of efficacy of anti-tumor necrosis factor (TNF) therapy in patients with psoriasis previously treated with a different anti-TNF agent. J Am Acad Dermatol 2016; 75(3):612-618.e6; PMID:27061047; http://dx.doi.org/10.1016/j.jaad.2016.02.1221
- Mahdavi A, Szychowski J, Ngo JT, Sweredoski MJ, Graham RLJ, Hess S, Schneewind O, Mazmanian SK, Tirrell DA. Identification of secreted bacterial proteins by noncanonical amino acid tagging. Proc Natl Acad Sci U S A 2014; 111(1):433-8; PMID:24347637; http://dx.doi.org/10.1073/pnas.1301740111
- Rosqvist R, Forsberg A, Rimpilainen M, Bergman T, Wolf-Watz H. The cytotoxic protein YopE of Yersinia obstructs the primary host defence. Mol Microbiol 1990; 4(4):657-67; PMID:2191183; http://dx.doi.org/10.1111/j.1365-2958.1990.tb00635.x
- Viboud GI, Bliska JB. A bacterial type III secretion system inhibits actin polymerization to prevent pore formation in host cell membranes. EMBO J 2001; 20(19):5373-82; PMID:11574469; http://dx.doi.org/10.1093/emboj/20.19.5373
- Andor A, Trulzsch K, Essler M, Roggenkamp A, Wiedemann A, Heesemann J, Aepfelbacher M. YopE of Yersinia, a GAP for Rho GTPases, selectively modulates Rac-dependent actin structures in endothelial cells. Cell Microbiol 2001; 3(5):301-10; PMID:11298653; http://dx.doi.org/10.1046/j.1462-5822.2001.00114.x
- Mohammadi S, Isberg RR. Yersinia pseudotuberculosis virulence determinants invasin, YopE, and YopT modulate RhoG activity and localization. Infect Immun 2009; 77(11):4771-82; PMID:19720752; http://dx.doi.org/10.1128/IAI.00850-09
- Black DS, Bliska JB. The RhoGAP activity of the Yersinia pseudotuberculosis cytotoxin YopE is required for antiphagocytic function and virulence. Mol Microbiol 2000; 37(3):515-27; PMID:10931345; http://dx.doi.org/10.1046/j.1365-2958.2000.02021.x
- Evdokimov AG, Tropea JE, Routzahn KM, Waugh DS. Crystal structure of the Yersinia pestis GTPase activator YopE. Protein Sci 2002; 11(2):401-8; PMID:11790850; http://dx.doi.org/10.1110/ps.34102
- Boyd AP, Lambermont I, Cornelis GR. Competition between the Yops of Yersinia enterocolitica for delivery into eukaryotic cells: Role of the SycE chaperone binding domain of YopE. J Bacteriol 2000; 182(17):4811-21; PMID:10940022; http://dx.doi.org/10.1128/JB.182.17.4811-4821.2000
- Krall R, Zhang Y, Barbieri JT. Intracellular membrane localization of Pseudomonas ExoS and Yersinia YopE in mammalian cells. J Biol Chem 2004; 279(4):2747-53; PMID:14597627; http://dx.doi.org/10.1074/jbc.M301963200
- Ruckdeschel K, Pfaffinger G, Trulzsch K, Zenner G, Richter K, Heesemann J, Aepfelbacher M. The proteasome pathway destabilizes Yersinia outer protein E and represses its antihost cell activities. J Immunol 2006; 176(10):6093-6102; PMID:16670318; http://dx.doi.org/10.4049/jimmunol.176.10.6093
- Hentschke M, Trülzsch K, Heesemann J, Aepfelbacher M, Ruckdeschel K. Serogroup-related escape of Yersinia enterocolitica YopE from degradation by the ubiquitin-proteasome pathway. Infect Immun 2007; 75(9):4423-31; PMID:17606597; http://dx.doi.org/10.1128/IAI.00528-07
- Aili M, Isaksson EL, Carlsson SE, Wolf-Watz H, Rosqvist R, Francis MS. Regulation of Yersinia Yop-effector delivery by translocated YopE. Int J Med Microbiol 2008; 298(3–4):183-92; PMID:17597003; http://dx.doi.org/10.1016/j.ijmm.2007.04.007
- Gaus K, Hentschke M, Czymmeck N, Novikova L, Trülzsch K, Valentin-Weigand P, Aepfelbacher M, Ruckdeschel K. Destabilization of YopE by the ubiquitin-proteasome pathway fine-tunes Yop delivery into host cells and facilitates systemic spread of Yersinia enterocolitica in host lymphoid tissue. Infect Immun 2011; 79(3):1166-75; PMID:21149597; http://dx.doi.org/10.1128/IAI.00694-10
- Lockman HA, Gillespie RA, Baker BD, Shakhnovich E. Yersinia pseudotuberculosis produces a cytotoxic necrotizing factor. Infect Immun 2002; 70(5):2708-14; PMID:11953417; http://dx.doi.org/10.1128/IAI.70.5.2708-2714.2002
- Wolters M, Boyle EC, Lardong K, Truelzsch K, Steffen A, Rottner K, Ruckdeschel K, Aepfelbacher M. Cytotoxic necrotizing factor-Y boosts Yersinia effector translocation by activating Rac. J Biol Chem 2013; 288(32):23543-53; PMID:23803609; http://dx.doi.org/10.1074/jbc.M112.448662
- Bliska JB, Wang X, Viboud GI, Brodsky IE. Modulation of innate immune responses by Yersinia type III secretion system translocators and effectors. Cell Microbiol 2013; 15(10):1622-31; PMID:23834311; http://dx.doi.org/10.1111/cmi.12164
- Mainiero F, Soriani A, Strippoli R, Jacobelli J, Gismondi A, Piccoli M, Frati L, Santoni A. RAC1/P38 MAPK signaling pathway controls beta1 integrin-induced interleukin-8 production in human natural killer cells. Immunity 2000; 12(1):7-16; PMID:10661401; http://dx.doi.org/10.1016/S1074-7613(00)80154-5
- Coso OA, Chiariello M, Yu JC, Teramoto H, Crespo P, Xu N, Miki T, Gutkind JS. The small GTP-binding proteins Rac1 and Cdc42 regulate the activity of the JNK/SAPK signaling pathway. Cell 1995; 81(7):1137-46; PMID:7600581; http://dx.doi.org/10.1016/S0092-8674(05)80018-2
- Viboud GI, Mejía E, Bliska JB. Comparison of YopE and YopT activities in counteracting host signalling responses to Yersinia pseudotuberculosis infection. Cell Microbiol 2006; 8(9):1504-15; PMID:16922868; http://dx.doi.org/10.1111/j.1462-5822.2006.00729.x
- Schotte P, Denecker G, van Den Broeke A, Vandenabeele P, Cornelis GR, Beyaert R. Targeting Rac1 by the Yersinia effector protein YopE inhibits caspase-1-mediated maturation and release of interleukin-1beta. J Biol Chem 2004; 279(24):25134-42; PMID:15060067; http://dx.doi.org/10.1074/jbc.M401245200
- Condliffe AM, Webb LMC, Ferguson GJ, Davidson K, Turner M, Vigorito E, Manifava M, Chilvers ER, Stephens LR, Hawkins PT. RhoG regulates the neutrophil NADPH oxidase. J Immunol 2006; 176(9):5314-20; PMID:16621998; http://dx.doi.org/10.4049/jimmunol.176.9.5314
- Hordijk PL. Regulation of NADPH oxidases: The role of Rac proteins. Circ Res 2006; 98(4):453-62; PMID:16514078; http://dx.doi.org/10.1161/01.RES.0000204727.46710.5e
- Songsungthong W, Higgins MC, Rolán HG, Murphy JL, Mecsas J. ROS-inhibitory activity of YopE is required for full virulence of Yersinia in mice. Cell Microbiol 2010; 12(7):988-1001; PMID:20148901; http://dx.doi.org/10.1111/j.1462-5822.2010.01448.x
- Conlan JW. Critical roles of neutrophils in host defense against experimental systemic infections of mice by Listeria monocytogenes, Salmonella typhimurium, and Yersinia enterocolitica. Infect Immun 1997; 65(2):630-5; PMID:9009323
- Westermark L, Fahlgren A, Fällman M. Yersinia pseudotuberculosis efficiently escapes polymorphonuclear neutrophils during early infection. Infect Immun 2014; 82(3):1181-91; PMID:24379291; http://dx.doi.org/10.1128/IAI.01634-13
- Wang X, Parashar K, Sitaram A, Bliska JB. The GAP activity of type III effector YopE triggers killing of Yersinia in macrophages. PLoS Pathog 2014; 10(8):e1004346; PMID:25165815; http://dx.doi.org/10.1371/journal.ppat.1004346
- Schulz A. Influence of Rho GTPases on Toll-Like Receptor (TLR)–induced cytokine production of murine macrophages [Dissertation]. Osnabrück, Germany: Universität Osnabrück; 2014
- Fessler MB, Arndt PG, Just I, Nick JA, Malcolm KC, Scott Worthen G. Dual role for RhoA in suppression and induction of cytokines in the human neutrophil. Blood 2007; 109(3):1248-56; PMID:17018860; http://dx.doi.org/10.1182/blood-2006-03-012898
- Kuhl AA, Kakirman H, Janotta M, Dreher S, Cremer P, Pawlowski NN, Loddenkemper C, Heimesaat MM, Grollich K, Zeitz M, et al. Aggravation of different types of experimental colitis by depletion or adhesion blockade of neutrophils. Gastroenterology 2007; 133(6):1882-92; PMID:18054560; http://dx.doi.org/10.1053/j.gastro.2007.08.073
- Sipponen T. Diagnostics and prognostics of inflammatory bowel disease with fecal neutrophil-derived biomarkers calprotectin and lactoferrin. Dig Dis 2013; 31(3–4):336-44; PMID:24246984; http://dx.doi.org/10.1159/000354689
- Amulic B, Cazalet C, Hayes GL, Metzler KD, Zychlinsky A. Neutrophil function: From mechanisms to disease. Annu Rev Immunol 2012; 30:459-89; PMID:22224774; http://dx.doi.org/10.1146/annurev-immunol-020711-074942
- Biro M, Munoz MA, Weninger W. Targeting Rho-GTPases in immune cell migration and inflammation. Br J Pharmacol 2014; 171(24):5491-5506; PMID:24571448; http://dx.doi.org/10.1111/bph.12658
- Larmonier CB, Midura-Kiela MT, Ramalingam R, Laubitz D, Janikashvili N, Larmonier N, Ghishan FK, Kiela PR. Modulation of neutrophil motility by curcumin. Inflamm Bowel Dis 2011; 17(2):503-15; PMID:20629184; http://dx.doi.org/10.1002/ibd.21391
- Boppana NB, Devarajan A, Gopal K, Barathan M, Bakar SA, Shankar EM, Ebrahim AS, Farooq SM. Blockade of CXCR2 signalling: A potential therapeutic target for preventing neutrophil-mediated inflammatory diseases. Exp Biol Med 2014; 239(5):509-18; PMID:24625439; http://dx.doi.org/10.1177/1535370213520110
- Shioya Y, Katakura K, Ohira H. Neutrophil elastase inhibitor suppresses IL-17 based inflammation of murine experimental colitis. Fukushima J Med Sci 2014; 60(1):14-21; PMID:24670675; http://dx.doi.org/10.5387/fms.2013-2
- Huang Y, Xiao S, Jiang Q. Role of Rho kinase signal pathway in inflammatory bowel disease. Int J Clin Exp Med 2015; 8(3):3089-97; PMID:26064197
- Beyaert R, Schotte P, inventors; Vib Vzw, Universiteit Gent, assignee. The use of yop proteins or rho gtpase inhibitors as caspase-1 inhibitors. WO 2004064713 A2. 2004 Aug 5
- Iriarte M, Cornelis GR. YopT, a new Yersinia Yop effector protein, affects the cytoskeleton of host cells. Mol Microbiol 1998; 29(3):915-29; PMID:9723929; http://dx.doi.org/10.1046/j.1365-2958.1998.00992.x
- Shao F, Vacratsis PO, Bao ZQ, Bowers KE, Fierke CA, Dixon JE. Biochemical characterization of the Yersinia YopT protease: Cleavage site and recognition elements in Rho GTPases. Proc Natl Acad Sci U S A 2003; 100(3):904-9; PMID:12538863; http://dx.doi.org/10.1073/pnas.252770599
- Sorg I, Goehring UM, Aktories K, Schmidt G. Recombinant Yersinia YopT leads to uncoupling of RhoA-effector interaction. Infect Immun 2001; 69(12):7535-43; PMID:11705930; http://dx.doi.org/10.1128/IAI.69.12.7535-7543.2001
- Füller F, Bergo MO, Young SG, Aktories K, Schmidt G. Endoproteolytic processing of RhoA by Rce1 is required for the cleavage of RhoA by Yersinia enterocolitica outer protein T. Infect Immun 2006; 74(3):1712-7; PMID:16495543; http://dx.doi.org/10.1128/IAI.74.3.1712-1717.2006
- Aepfelbacher M, Trasak C, Wilharm G, Wiedemann A, Trulzsch K, Krauss K, Gierschik P, Heesemann J. Characterization of YopT effects on Rho GTPases in Yersinia enterocolitica-infected cells. J Biol Chem 2003; 278(35):33217-23; PMID:12791693; http://dx.doi.org/10.1074/jbc.M303349200
- Shao F, Merritt PM, Bao ZQ, Innes RW, Dixon JE. A Yersinia effector and a Pseudomonas avirulence protein define a family of cysteine proteases functioning in bacterial pathogenesis. Cell 2002; 109(5):575-88; PMID:12062101; http://dx.doi.org/10.1016/S0092-8674(02)00766-3
- Zhu M, Shao F, Innes RW, Dixon JE, Xu Z. The crystal structure of Pseudomonas avirulence protein AvrPphB: A papain-like fold with a distinct substrate-binding site. Proc Natl Acad Sci U S A 2004; 101(1):302-7; PMID:14694194; http://dx.doi.org/10.1073/pnas.2036536100
- Boolell M, Allen MJ, Ballard SA, Gepi-Attee S, Muirhead GJ, Naylor AM, Osterloh IH, Gingell C. Sildenafil: An orally active type 5 cyclic GMP-specific phosphodiesterase inhibitor for the treatment of penile erectile dysfunction. Int J Impot Res 1996; 8(2):47-52; PMID:8858389
- Dai Y, Chitaley K, Webb RC, Lewis RW, Mills TM. Topical application of a Rho-kinase inhibitor in rats causes penile erection. Int J Impot Res 2004; 16(3):294-8; PMID:14961052; http://dx.doi.org/10.1038/sj.ijir.3901171
- He Y, Xu H, Liang L, Zhan Z, Yang X, Yu X, Ye Y, Sun L. Antiinflammatory effect of Rho kinase blockade via inhibition of NF-kappaB activation in rheumatoid arthritis. Arthritis Rheum 2008; 58(11):3366-76; PMID:18975348; http://dx.doi.org/10.1002/art.23986
- Tan EYM, Law JWS, Wang C, Lee AYW. Development of a cell transducible RhoA inhibitor TAT-C3 transferase and its encapsulation in biocompatible microspheres to promote survival and enhance regeneration of severed neurons. Pharm Res 2007; 24(12):2297-2308; PMID:17899323; http://dx.doi.org/10.1007/s11095-007-9454-6
- Lord-Fontaine S, Yang F, Diep Q, Dergham P, Munzer S, Tremblay P, McKerracher L. Local inhibition of Rho signaling by cell-permeable recombinant protein BA-210 prevents secondary damage and promotes functional recovery following acute spinal cord injury. J Neurotrauma 2008; 25(11):1309-22; PMID:19061375; http://dx.doi.org/10.1089/neu.2008.0613
- Huentelman MJ, Stephan DA, Talboom J, Corneveaux JJ, Reiman DM, Gerber JD, Barnes CA, Alexander GE, Reiman EM, Bimonte-Nelson HA. Peripheral delivery of a ROCK inhibitor improves learning and working memory. Behav Neurosci 2009; 123(1):218-23; PMID:19170447; http://dx.doi.org/10.1037/a0014260
- Galyov EE, Håkansson S, Forsberg A, Wolf-Watz H. A secreted protein kinase of Yersinia pseudotuberculosis is an indispensable virulence determinant. Nature 1993; 361(6414):730-2; PMID:8441468; http://dx.doi.org/10.1038/361730a0
- Håkansson S, Galyov EE, Rosqvist R, Wolf-Watz H. The Yersinia YpkA Ser/Thr kinase is translocated and subsequently targeted to the inner surface of the HeLa cell plasma membrane. Mol Microbiol 1996; 20(3):593-603; PMID:8736538; http://dx.doi.org/10.1046/j.1365-2958.1996.5251051.x
- Juris SJ, Rudolph AE, Huddler D, Orth K, Dixon JE. A distinctive role for the Yersinia protein kinase: Actin binding, kinase activation, and cytoskeleton disruption. Proc Natl Acad Sci U S A 2000; 97(17):9431-6; PMID:10920208; http://dx.doi.org/10.1073/pnas.170281997
- Lee WL, Grimes JM, Robinson RC. Yersinia effector YopO uses actin as bait to phosphorylate proteins that regulate actin polymerization. Nat Struct Mol Biol 2015; 22(3):248-55; PMID:25664724; http://dx.doi.org/10.1038/nsmb.2964
- Navarro L, Koller A, Nordfelth R, Wolf-Watz H, Taylor S, Dixon JE. Identification of a molecular target for the Yersinia protein kinase A. Mol Cell 2007; 26(4):465-77; PMID:17531806; http://dx.doi.org/10.1016/j.molcel.2007.04.025
- Yang H, Ke Y, Wang J, Tan Y, Myeni SK, Li D, Shi Q, Yan Y, Chen H, Guo Z, et al. Insight into bacterial virulence mechanisms against host immune response via the Yersinia pestis-human protein-protein interaction network. Infect Immun 2011; 79(11):4413-24; PMID:21911467; http://dx.doi.org/10.1128/IAI.05622-11
- Prehna G, Ivanov MI, Bliska JB, Stebbins CE. Yersinia virulence depends on mimicry of host Rho-family nucleotide dissociation inhibitors. Cell 2006; 126(5):869-80; PMID:16959567; http://dx.doi.org/10.1016/j.cell.2006.06.056
- Nejedlik L, Pierfelice T, Geiser JR. Actin distribution is disrupted upon expression of Yersinia YopO/YpkA in yeast. Yeast 2004; 21(9):759-68; PMID:15282799; http://dx.doi.org/10.1002/yea.1135
- Dukuzumuremyi JM, Rosqvist R, Hallberg B, Akerström B, Wolf-Watz H, Schesser K. The Yersinia protein kinase A is a host factor inducible RhoA/Rac-binding virulence factor. J Biol Chem 2000; 275(45):35281-90; PMID:10950948; http://dx.doi.org/10.1074/jbc.M003009200
- Niedergang F, Chavrier P. Regulation of phagocytosis by Rho GTPases. Curr Top Microbiol Immunol 2005; 291:43-60; PMID:15981459
- Galvez J. Role of Th17 Cells in the pathogenesis of human IBD. ISRN Inflamm 2014; 2014:928461; PMID:25101191; http://dx.doi.org/10.1155/2014/928461
- Liu Y, Wang D, Li F, Shi G. Galphaq controls rheumatoid arthritis via regulation of Th17 differentiation. Immunol Cell Biol 2015; 93(7):616-24; PMID:25732870; http://dx.doi.org/10.1038/icb.2015.13
- Kawasaki T, Taniguchi M, Moritani Y, Hayashi K, Saito T, Takasaki J, Nagai K, Inagaki O, Shikama H. Antithrombotic and thrombolytic efficacy of YM-254890, a G q/11 inhibitor, in a rat model of arterial thrombosis. Thromb Haemost 2003; 90(3):406-13; PMID:12958608; http://dx.doi.org/10.1160/TH03-02-0115
- Zaima K, Deguchi J, Matsuno Y, Kaneda T, Hirasawa Y, Morita H. Vasorelaxant effect of FR900359 from Ardisia crenata on rat aortic artery. J Nat Med 2013; 67(1):196-201; PMID:22388972; http://dx.doi.org/10.1007/s11418-012-0644-0
- Richter K. Charakterisierung der molekularen Wirkungen des Y. enterocolitica Effektorproteins YopP auf die Wirtszelle [Dissertation]. München, Germany: Ludwig Maximilians-Universität; 2004
- Brodsky IE, Medzhitov R. Reduced secretion of YopJ by Yersinia limits in vivo cell death but enhances bacterial virulence. PLoS Pathog 2008; 4(5):e1000067; PMID:18483548; http://dx.doi.org/10.1371/journal.ppat.1000067
- Zheng Y, Lilo S, Brodsky IE, Zhang Y, Medzhitov R, Marcu KB, Bliska JB. A Yersinia effector with enhanced inhibitory activity on the NF-κB pathway activates the NLRP3/ASC/caspase-1 inflammasome in macrophages. PLoS Pathog 2011; 7(4):e1002026; PMID:21533069; http://dx.doi.org/10.1371/journal.ppat.1002026
- Zheng Y, Lilo S, Mena P, Bliska JB. YopJ-induced caspase-1 activation in Yersinia-infected macrophages: Independent of apoptosis, linked to necrosis, dispensable for innate host defense. PLoS One 2012; 7(4):e36019; PMID:22563435; http://dx.doi.org/10.1371/journal.pone.0036019
- Zauberman A, Tidhar A, Levy Y, Bar-Haim E, Halperin G, Flashner Y, Cohen S, Shafferman A, Mamroud E. Yersinia pestis endowed with increased cytotoxicity is avirulent in a bubonic plague model and induces rapid protection against pneumonic plague. PLoS One 2009; 4(6):e5938; PMID:19529770; http://dx.doi.org/10.1371/journal.pone.0005938
- Sweet CR, Conlon J, Golenbock DT, Goguen J, Silverman N. YopJ targets TRAF proteins to inhibit TLR-mediated NF-kappaB, MAPK and IRF3 signal transduction. Cell Microbiol 2007; 9(11):2700-15; PMID:17608743; http://dx.doi.org/10.1111/j.1462-5822.2007.00990.x
- Zhou H, Monack DM, Kayagaki N, Wertz I, Yin J, Wolf B, Dixit VM. Yersinia virulence factor YopJ acts as a deubiquitinase to inhibit NF-kappa B activation. J Exp Med 2005; 202(10):1327-32; PMID:16301742; http://dx.doi.org/10.1084/jem.20051194
- Cao Y, Guan K, He X, Wei C, Zheng Z, Zhang Y, Ma S, Zhong H, Shi W. Yersinia YopJ negatively regulates IRF3-mediated antibacterial response through disruption of STING-mediated cytosolic DNA signaling. Biochim Biochim Biophys Acta 2016; 1863(12):3148-59; PMID:27742471; http://dx.doi.org/10.1016/j.bbamcr.2016.10.004
- Haase R, Richter K, Pfaffinger G, Courtois G, Ruckdeschel K. Yersinia outer protein P suppresses TGF-beta-activated kinase-1 activity to impair innate immune signaling in Yersinia enterocolitica-infected cells. J Immunol 2005; 175(12):8209-17; PMID:16339560; http://dx.doi.org/10.4049/jimmunol.175.12.8209
- Deng L, Wang C, Spencer E, Yang L, Braun A, You J, Slaughter C, Pickart C, Chen ZJ. Activation of the IkappaB kinase complex by TRAF6 requires a dimeric ubiquitin-conjugating enzyme complex and a unique polyubiquitin chain. Cell 2000; 103(2):351-61; PMID:11057907; http://dx.doi.org/10.1016/S0092-8674(00)00126-4
- Shi C, Kehrl JH. Tumor necrosis factor (TNF)-induced germinal center kinase-related (GCKR) and stress-activated protein kinase (SAPK) activation depends upon the E2/E3 complex Ubc13-Uev1A/TNF receptor-associated factor 2 (TRAF2). J Biol Chem 2003; 278(17):15429-34; PMID:12591926; http://dx.doi.org/10.1074/jbc.M211796200
- Zhang J, Hu M, Wang Y, Shu H. TRIM32 protein modulates type I interferon induction and cellular antiviral response by targeting MITA/STING protein for K63-linked ubiquitination. J Biol Chem 2012; 287(34):28646-55; PMID:22745133; http://dx.doi.org/10.1074/jbc.M112.362608
- Chen Z, Hagler J, Palombella VJ, Melandri F, Scherer D, Ballard D, Maniatis T. Signal-induced site-specific phosphorylation targets I kappa B alpha to the ubiquitin-proteasome pathway. Genes Dev 1995; 9(13):1586-97; PMID:7628694; http://dx.doi.org/10.1101/gad.9.13.1586
- Häcker H, Tseng P, Karin M. Expanding TRAF function: TRAF3 as a tri-faced immune regulator. Nat Rev Immunol 2011; 11(7):457-68; PMID:21660053; http://dx.doi.org/10.1038/nri2998
- Mittal R, Peak-Chew S, McMahon HT. Acetylation of MEK2 and I kappa B kinase (IKK) activation loop residues by YopJ inhibits signaling. Proc Natl Acad Sci U S A 2006; 103(49):18574-9; PMID:17116858; http://dx.doi.org/10.1073/pnas.0608995103
- Mukherjee S, Keitany G, Li Y, Wang Y, Ball HL, Goldsmith EJ, Orth K. Yersinia YopJ acetylates and inhibits kinase activation by blocking phosphorylation. Science 2006; 312(5777):1211-4; PMID:16728640; http://dx.doi.org/10.1126/science.1126867
- Meinzer U, Barreau F, Esmiol-Welterlin S, Jung C, Villard C, Léger T, Ben-Mkaddem S, Berrebi D, Dussaillant M, Alnabhani Z, et al. Yersinia pseudotuberculosis effector YopJ subverts the Nod2/RICK/TAK1 pathway and activates caspase-1 to induce intestinal barrier dysfunction. Cell Host Microbe 2012; 11(4):337-51; PMID:22520462; http://dx.doi.org/10.1016/j.chom.2012.02.009
- Paquette N, Conlon J, Sweet C, Rus F, Wilson L, Pereira A, Rosadini CV, Goutagny N, Weber ANR, Lane WS, et al. Serine/threonine acetylation of TGFβ-activated kinase (TAK1) by Yersinia pestis YopJ inhibits innate immune signaling. Proc Natl Acad Sci U S A 2012; 109(31):12710-5; PMID:22802624; http://dx.doi.org/10.1073/pnas.1008203109
- Thiefes A, Wolf A, Doerrie A, A Grassl G, Matsumoto K, Autenrieth IB, Bohn E, Sakurai H, Niedenthal R, Resch K, et al. The Yersinia enterocolitica effector YopP inhibits host cell signalling by inactivating the protein kinase TAK1 in the IL-1 signalling pathway. EMBO Rep 2006; 7(8):838-44; PMID:16845370; http://dx.doi.org/10.1038/sj.embor.7400754
- Orth K, Palmer LE, Bao ZQ, Stewart S, Rudolph AE, Bliska JB, Dixon JE. Inhibition of the mitogen-activated protein kinase kinase superfamily by a Yersinia effector. Science 1999; 285(5435):1920-3; PMID:10489373; http://dx.doi.org/10.1126/science.285.5435.1920
- Pruneda JN, Durkin CH, Geurink PP, Ovaa H, Santhanam B, Holden DW, Komander D. The molecular basis for ubiquitin and ubiquitin-like specificities in bacterial effector proteases. Mol Cell 2016; 63(2):271-6; PMID:27425412; http://dx.doi.org/10.1016/j.molcel.2016.06.015
- Zhang Z, Ma K, Yuan S, Luo Y, Jiang S, Hawara E, Pan S, Ma W, Song J. Structure of a pathogen effector reveals the enzymatic mechanism of a novel acetyltransferase family. Nat Struct Mol Biol 2016; 23(9):847-52; PMID:27525589; http://dx.doi.org/10.1038/nsmb.3279
- Mittal R, Peak-Chew SY, Sade RS, Vallis Y, McMahon HT. The acetyltransferase activity of the bacterial toxin YopJ of Yersinia is activated by eukaryotic host cell inositol hexakisphosphate. J Biol Chem 2010; 285(26):19927-34; PMID:20430892; http://dx.doi.org/10.1074/jbc.M110.126581
- Ajibade AA, Wang HY, Wang R. Cell type-specific function of TAK1 in innate immune signaling. Trends Immunol 2013; 34(7):307-16; PMID:23664135; http://dx.doi.org/10.1016/j.it.2013.03.007
- Yoon S, Liu Z, Eyobo Y, Orth K. Yersinia effector YopJ inhibits yeast MAPK signaling pathways by an evolutionarily conserved mechanism. J Biol Chem 2003; 278(4):2131-5; PMID:12433923; http://dx.doi.org/10.1074/jbc.M209905200
- Rosadini CV, Zanoni I, Odendall C, Green ER, Paczosa MK, Philip NH, Brodsky IE, Mecsas J, Kagan JC. A single bacterial immune evasion strategy dismantles both MyD88 and TRIF signaling pathways downstream of TLR4. Cell Host Microbe 2015; 18(6):682-93; PMID:26651944; http://dx.doi.org/10.1016/j.chom.2015.11.006
- Denecker G, Tötemeyer S, Mota LJ, Troisfontaines P, Lambermont I, Youta C, Stainier I, Ackermann M, Cornelis GR, Denecker G, et al. Effect of low- and high-virulence Yersinia enterocolitica strains on the inflammatory response of human umbilical vein endothelial cells. Infect Immun 2002; 70(7):3510-20; PMID:12065490; http://dx.doi.org/10.1128/IAI.70.7.3510-3520.2002
- Boland AM, Cornelis GR. Role of YopP in suppression of tumor necrosis factor alpha release by macrophages during Yersinia infection. Infect Immun 1998; 66(5):1878-84; PMID:9573064
- Autenrieth SE, Soldanova I, Rösemann R, Gunst D, Zahir N, Kracht M, Ruckdeschel K, Wagner H, Borgmann S, Autenrieth IB. Yersinia enterocolitica YopP inhibits MAP kinase-mediated antigen uptake in dendritic cells. Cell Microbiol 2007; 9(2):425-37; PMID:16953801; http://dx.doi.org/10.1111/j.1462-5822.2006.00800.x
- Mills SD, Boland AM, Sory MP, van der Smissen P, Kerbourch C, Finlay BB, Cornelis GR. Yersinia enterocolitica induces apoptosis in macrophages by a process requiring functional type III secretion and translocation mechanisms and involving YopP, presumably acting as an effector protein. Proc Natl Acad Sci U S A 1997; 94(23):12638-43; PMID:9356502; http://dx.doi.org/10.1073/pnas.94.23.12638
- Monack DM, Mecsas J, Ghori N, Falkow S. Yersinia signals macrophages to undergo apoptosis and YopJ is necessary for this cell death. Proc Natl Acad Sci U S A 1997; 94(19):10385-90; PMID:9294220; http://dx.doi.org/10.1073/pnas.94.19.10385
- Ruckdeschel K, Roggenkamp A, Lafont V, Mangeat P, Heesemann J, Rouot B. Interaction of Yersinia enterocolitica with macrophages leads to macrophage cell death through apoptosis. Infect Immun 1997; 65(11):4813-21; PMID:9353070
- Denecker G, Declercq W, Geuijen CA, Boland AM, Benabdillah R, van Gurp M, Sory MP, Vandenabeele P, Cornelis GR. Yersinia enterocolitica YopP-induced apoptosis of macrophages involves the apoptotic signaling cascade upstream of bid. J Biol Chem 2001; 276(23):19706-14; PMID:11279213; http://dx.doi.org/10.1074/jbc.M101573200
- Erfurth SE, Gröbner S, Kramer U, Gunst DS, Soldanova I, Schaller M, Autenrieth IB, Borgmann S. Yersinia enterocolitica induces apoptosis and inhibits surface molecule expression and cytokine production in murine dendritic cells. Infect Immun 2004; 72(12):7045-54; PMID:15557628; http://dx.doi.org/10.1128/IAI.72.12.7045-7054.2004
- Spinner JL, Seo KS, O'Loughlin JL, Cundiff JA, Minnich SA, Bohach GA, Kobayashi SD. Neutrophils are resistant to Yersinia YopJ/P-induced apoptosis and are protected from ROS-mediated cell death by the type III secretion system. PLoS One 2010; 5(2):e9279; PMID:20174624; http://dx.doi.org/10.1371/journal.pone.0009279
- Koch I, Dach K, Heesemann J, Hoffmann R. Yersinia enterocolitica inactivates NK cells. Int J Med Microbiol 2013; 303(8):433-42; PMID:23810728; http://dx.doi.org/10.1016/j.ijmm.2013.05.004
- Zhang Y, Bliska JB. Role of toll-like receptor signaling in the apoptotic response of macrophages to Yersinia infection. Infect Immun 2003; 71(3):1513-9; PMID:12595470; http://dx.doi.org/10.1128/IAI.71.3.1513-1519.2003
- Zhang Y, Ting AT, Marcu KB, Bliska JB. Inhibition of MAPK and NF-kappa B pathways is necessary for rapid apoptosis in macrophages infected with Yersinia. J Immunol 2005; 174(12):7939-49; PMID:15944300; http://dx.doi.org/10.4049/jimmunol.174.12.7939
- Weng D, Marty-Roix R, Ganesan S, Proulx MK, Vladimer GI, Kaiser WJ, Mocarski ES, Pouliot K, Chan FK, Kelliher MA, et al. Caspase-8 and RIP kinases regulate bacteria-induced innate immune responses and cell death. Proc Natl Acad Sci U S A 2014; 111(20):7391-6; PMID:24799678; http://dx.doi.org/10.1073/pnas.1403477111
- Philip NH, Dillon CP, Snyder AG, Fitzgerald P, Wynosky-Dolfi MA, Zwack EE, Hu B, Fitzgerald L, Mauldin EA, Copenhaver AM, et al. Caspase-8 mediates caspase-1 processing and innate immune defense in response to bacterial blockade of NF-κB and MAPK signaling. Proc Natl Acad Sci U S A 2014; 111(20):7385-90; PMID:24799700; http://dx.doi.org/10.1073/pnas.1403252111
- Davignon J, Hayder M, Baron M, Boyer J, Constantin A, Apparailly F, Poupot R, Cantagrel A. Targeting monocytes/macrophages in the treatment of rheumatoid arthritis. Rheumatology (Oxford) 2013; 52(4):590-8; PMID:23204551; http://dx.doi.org/10.1093/rheumatology/kes304
- Wang H, Peters T, Kess D, Sindrilaru A, Oreshkova T, van Rooijen N, Stratis A, Renkl AC, Sunderkötter C, Wlaschek M, et al. Activated macrophages are essential in a murine model for T cell-mediated chronic psoriasiform skin inflammation. J Clin Invest 2006; 116(8):2105-14; PMID:16886059; http://dx.doi.org/10.1172/JCI27180
- Wang H, Peters T, Sindrilaru A, Scharffetter-Kochanek K. Key role of macrophages in the pathogenesis of CD18 hypomorphic murine model of psoriasis. J Invest Dermatol 2009; 129(5):1100-14; PMID:19242511; http://dx.doi.org/10.1038/jid.2009.43
- Pettersson S, Wolf-Watz H, inventors; Karolinska Innovations Ab, assignee. Composition and methods for the treatment of inflammatory diseases. WO 1998056817 A1. 1998 Dec 17
- Wallach D, Appel E, inventors. Chimeric proteins comprising Yersinia Yop, their preparation and pharmaceutical compositions containing them. WO/2006/109303. 2006 Oct 19
- Herrera Estrada LP, Padmore TJ, Champion JA. Bacterial effector nanoparticles as breast cancer therapeutics. Mol Pharm 2016; 13(3):710-9; PMID:26800341; http://dx.doi.org/10.1021/acs.molpharmaceut.5b00377
- Sajid A, Arora G, Singhal A, Kalia VC, Singh Y. Protein phosphatases of pathogenic bacteria: Role in physiology and virulence. Annu Rev Microbiol 2015; 69:527-47; PMID:26359969; http://dx.doi.org/10.1146/annurev-micro-020415-111342
- Guan KL, Dixon JE. Protein tyrosine phosphatase activity of an essential virulence determinant in Yersinia. Science 1990; 249(4968):553-6; PMID:2166336; http://dx.doi.org/10.1126/science.2166336
- Sory MP, Boland AM, Lambermont I, Cornelis GR. Identification of the YopE and YopH domains required for secretion and internalization into the cytosol of macrophages, using the cyaA gene fusion approach. Proc Natl Acad Sci U S A 1995; 92(26):11998-12002; PMID:8618831; http://dx.doi.org/10.1073/pnas.92.26.11998
- Smith CL, Khandelwal P, Keliikuli K, Zuiderweg ER, Saper MA. Structure of the type III secretion and substrate-binding domain of Yersinia YopH phosphatase. Mol Microbiol 2001; 42(4):967-79; PMID:11737640; http://dx.doi.org/10.1046/j.0950-382x.2001.02711.x
- Zhang ZY, Wang Y, Wu L, Fauman EB, Stuckey JA, Schubert HL, Saper MA, Dixon JE. The Cys(X)5Arg catalytic motif in phosphoester hydrolysis. Biochemistry 1994; 33(51):15266-70; PMID:7803389; http://dx.doi.org/10.1021/bi00255a007
- Stuckey JA, Schubert HL, Fauman EB, Zhang ZY, Dixon JE, Saper MA. Crystal structure of Yersinia protein tyrosine phosphatase at 2.5 A and the complex with tungstate. Nature 1994; 370(6490):571-5; PMID:8052312; http://dx.doi.org/10.1038/370571a0
- Hu X, Stebbins CE. Dynamics of the WPD loop of the Yersinia protein tyrosine phosphatase. Biophys J 2006; 91(3):948-56; PMID:16698773; http://dx.doi.org/10.1529/biophysj.105.080259
- Bliska JB, Clemens JC, Dixon JE, Falkow S. The Yersinia tyrosine phosphatase: Specificity of a bacterial virulence determinant for phosphoproteins in the J774A.1 macrophage. J Exp Med 1992; 176(6):1625-30; PMID:1281213; http://dx.doi.org/10.1084/jem.176.6.1625
- Alonso A, Bottini N, Bruckner S, Rahmouni S, Williams S, Schoenberger SP, Mustelin T. Lck dephosphorylation at Tyr-394 and inhibition of T cell antigen receptor signaling by Yersinia phosphatase YopH. J Biol Chem 2004; 279(6):4922-8; PMID:14623872; http://dx.doi.org/10.1074/jbc.M308978200
- Persson C, Nordfelth R, Andersson K, Forsberg A, Wolf-Watz H, Fällman M. Localization of the Yersinia PTPase to focal complexes is an important virulence mechanism. Mol Microbiol 1999; 33(4):828-38; PMID:10447891; http://dx.doi.org/10.1046/j.1365-2958.1999.01529.x
- Montagna LG, Ivanov MI, Bliska JB. Identification of residues in the N-terminal domain of the Yersinia tyrosine phosphatase that are critical for substrate recognition. J Biol Chem 2001; 276(7):5005-11; PMID:11069923; http://dx.doi.org/10.1074/jbc.M009045200
- Adkins I, Köberle M, Gröbner S, Bohn E, Autenrieth IB, Borgmann S. Yersinia outer proteins E, H, P, and T differentially target the cytoskeleton and inhibit phagocytic capacity of dendritic cells. Int J Med Microbiol 2007; 297(4):235-44; PMID:17462949; http://dx.doi.org/10.1016/j.ijmm.2007.02.005
- Fahlgren A, Westermark L, Akopyan K, Fällman M. Cell type-specific effects of Yersinia pseudotuberculosis virulence effectors. Cell Microbiol 2009; 11(12):1750-67; PMID:19681909; http://dx.doi.org/10.1111/j.1462-5822.2009.01365.x
- Bruckner S, Rhamouni S, Tautz L, Denault J, Alonso A, Becattini B, Salvesen GS, Mustelin T. Yersinia phosphatase induces mitochondrially dependent apoptosis of T cells. J Biol Chem 2005; 280(11):10388-94; PMID:15632192; http://dx.doi.org/10.1074/jbc.M408829200
- Klinghoffer RA, Sachsenmaier C, Cooper JA, Soriano P. Src family kinases are required for integrin but not PDGFR signal transduction. EMBO J 1999; 18(9):2459-71; PMID:10228160; http://dx.doi.org/10.1093/emboj/18.9.2459
- Mitra SK, Schlaepfer DD. Integrin-regulated FAK-Src signaling in normal and cancer cells. Curr Opin Cell Biol 2006; 18(5):516-23; PMID:16919435; http://dx.doi.org/10.1016/j.ceb.2006.08.011
- Ophir MJ, Liu BC, Bunnell SC. The N terminus of SKAP55 enables T cell adhesion to TCR and integrin ligands via distinct mechanisms. J Cell Biol 2013; 203(6):1021-41; PMID:24368808; http://dx.doi.org/10.1083/jcb.201305088
- Isberg RR, Leong JM. Multiple beta 1 chain integrins are receptors for invasin, a protein that promotes bacterial penetration into mammalian cells. Cell 1990; 60(5):861-71; PMID:2311122; http://dx.doi.org/10.1016/0092-8674(90)90099-Z
- Tran Van Nhieu G, Isberg RR. Bacterial internalization mediated by beta 1 chain integrins is determined by ligand affinity and receptor density. EMBO J 1993; 12(5):1887-95; PMID:8491181
- Thinwa J, Segovia JA, Bose S, Dube PH. Integrin-mediated first signal for inflammasome activation in intestinal epithelial cells. J Immunol 2014; 193(3):1373-82; PMID:24965773; http://dx.doi.org/10.4049/jimmunol.1400145
- Kampik D, Schulte R, Autenrieth IB. Yersinia enterocolitica invasin protein triggers differential production of interleukin-1, interleukin-8, monocyte chemoattractant protein 1, granulocyte-macrophage colony-stimulating factor, and tumor necrosis factor alpha in epithelial cells: Implications for understanding the early cytokine network in Yersinia infections. Infect Immun 2000; 68(5):2484-92; PMID:10768935; http://dx.doi.org/10.1128/IAI.68.5.2484-2492.2000
- Bliska JB, Guan KL, Dixon JE, Falkow S. Tyrosine phosphate hydrolysis of host proteins by an essential Yersinia virulence determinant. Proc Natl Acad Sci U S A 1991; 88(4):1187-91; PMID:1705028; http://dx.doi.org/10.1073/pnas.88.4.1187
- Cantwell AM, Bubeck SS, Dube PH. YopH inhibits early pro-inflammatory cytokine responses during plague pneumonia. BMC Immunol 2010; 11:29; PMID:20565713; http://dx.doi.org/10.1186/1471-2172-11-29
- Dave MN, Silva JE, Eliçabe RJ, Jeréz MB, Filippa VP, Gorlino CV, Autenrieth S, Autenrieth IB, Di Genaro MS. Yersinia enterocolitica YopH-deficient strain activates neutrophil recruitment to Peyer's patches promoting clearance of the virulent strain. Infect Immun 2016; 84(11):3172-81; PMID:27550935; http://dx.doi.org/10.1128/IAI.00568-16
- Balada-Llasat J, Mecsas J. Yersinia has a tropism for B and T cell zones of lymph nodes that is independent of the type III secretion system. PLoS Pathog 2006; 2(9):e86; PMID:16948531; http://dx.doi.org/10.1371/journal.ppat.0020086
- Bergman MA, Loomis WP, Mecsas J, Starnbach MN, Isberg RR. CD8(+) T cells restrict Yersinia pseudotuberculosis infection: Bypass of anti-phagocytosis by targeting antigen-presenting cells. PLoS Pathog 2009; 5(9):e1000573; PMID:19730693; http://dx.doi.org/10.1371/journal.ppat.1000573
- Low JL, Chai CLL, Yao SQ. Bidentate inhibitors of protein tyrosine phosphatases. Antioxid Redox Signal 2013; 20(14):2225-50; http://dx.doi.org/10.1089/ars.2013.5710
- Paudyal MP, Wu L, Zhang Z, Spilling CD, Wong CF. A new class of salicylic acid derivatives for inhibiting YopH of Yersinia pestis. Bioorg Med Chem 2014; 22(24):6781-8; PMID:25468042; http://dx.doi.org/10.1016/j.bmc.2014.10.042
- Bottini A, Wu B, Barile E, De SK, Leone M, Pellecchia M. High-throughput screening (HTS) by NMR guided identification of novel agents targeting the protein docking domain of YopH. Chem Med Chem 2015; 11(8):919-27; http://dx.doi.org/10.1002/cmdc.201500441
- Kuban-Jankowska A, Sahu K, Gorska M, Tuszynski J, Wozniak M. Chicoric acid binds to two sites and decreases the activity of the YopH bacterial virulence factor. Oncotarget 2016; 7(3):2229-38; PMID:26735581; http://dx.doi.org/10.18632/oncotarget.6812
- Hansen A, Chaerkady R, Sharma J, Díaz-Mejía JJ, Tyagi N, Renuse S, Jacob HK, Pinto SM, Sahasrabuddhe NA, Kim M, et al. The Escherichia coli phosphotyrosine proteome relates to core pathways and virulence. PLoS Pathog 2013; 9(6):e1003403; PMID:23785281; http://dx.doi.org/10.1371/journal.ppat.1003403
- Binder NB, Puchner A, Niederreiter B, Hayer S, Leiss H, Blüml S, Kreindl R, Smolen JS, Redlich K. Tumor necrosis factor-inhibiting therapy preferentially targets bone destruction but not synovial inflammation in a tumor necrosis factor-driven model of rheumatoid arthritis. Arthritis Rheum 2013; 65(3):608-17; PMID:23280418; http://dx.doi.org/10.1002/art.37797
- Sauvonnet N, Lambermont I, van der Bruggen P, Cornelis GR. YopH prevents monocyte chemoattractant protein 1 expression in macrophages and T-cell proliferation through inactivation of the phosphatidylinositol 3-kinase pathway. Mol Microbiol 2002; 45(3):805-15; PMID:12139625; http://dx.doi.org/10.1046/j.1365-2958.2002.03053.x
- Malemud CJ. The PI3K/Akt/PTEN/mTOR pathway: A fruitful target for inducing cell death in rheumatoid arthritis? Future Med Chem 2015; 7(9):1137-47; PMID:26132523; http://dx.doi.org/10.4155/fmc.15.55
- Nakamura I, Jimi E, Duong LT, Sasaki T, Takahashi N, Rodan GA, Suda T. Tyrosine phosphorylation of p130Cas is involved in actin organization in osteoclasts. J Biol Chem 1998; 273(18):11144-9; PMID:9556601; http://dx.doi.org/10.1074/jbc.273.18.11144
- Goldring SR, Purdue PE, Crotti TN, Shen Z, Flannery MR, Binder NB, Ross FP, McHugh KP. Bone remodelling in inflammatory arthritis. Ann Rheum Dis 2013; 72(Suppl 2):ii52-5; PMID:23253928; http://dx.doi.org/10.1136/annrheumdis-2012-202199
- Gerke C, Falkow S, Chien Y. The adaptor molecules LAT and SLP-76 are specifically targeted by Yersinia to inhibit T cell activation. J Exp Med 2005; 201(3):361-71; PMID:15699071; http://dx.doi.org/10.1084/jem.20041120
- Aoudjit F, Vuori K. Integrin signaling in cancer cell survival and chemoresistance. Chemother Res Pract 2012; 2012:283181; PMID:22567280; http://dx.doi.org/10.1155/2012/283181
- Zhao X, Guan J. Focal adhesion kinase and its signaling pathways in cell migration and angiogenesis. Adv Drug Deliv Rev 2011; 63(8):610-5; PMID:21118706; http://dx.doi.org/10.1016/j.addr.2010.11.001
- Tai Y, Chen L, Shen T. Emerging roles of focal adhesion kinase in cancer. Biomed Res Int 2015; 2015:690690; PMID:25918719; http://dx.doi.org/10.1155/2015/690690
- Tanaka M, Shimamura S, Kuriyama S, Maeda D, Goto A, Aiba N. SKAP2 promotes podosome formation to facilitate tumor-associated macrophage infiltration and metastatic progression. Cancer Res 2015; 76(2):358-69; PMID:26577701; http://dx.doi.org/10.1158/0008-5472.CAN-15-1879
- Barrett A, Pellet-Many C, Zachary IC, Evans IM, Frankel P. p130Cas: A key signalling node in health and disease. Cell Signal 2013; 25(4):766-77; PMID:23277200; http://dx.doi.org/10.1016/j.cellsig.2012.12.019
- Yu P, Fu Y. Tumor-infiltrating T lymphocytes: Friends or foes? Lab Invest 2006; 86(3):231-45; PMID:16446705; http://dx.doi.org/10.1038/labinvest.3700389
- Carr G, Berrue F, Klaiklay S, Pelletier I, Landry M, Kerr RG. Natural products with protein tyrosine phosphatase inhibitory activity. Methods 2013; 65(2):229; PMID:24056213; http://dx.doi.org/10.1016/j.ymeth.2013.09.007
- Burrows TW. An antigen determining virulence in Pasteurella pestis. Nature 1956; 177(4505):426-7; PMID:13309325; http://dx.doi.org/10.1038/177426b0
- Sawa T, Katoh H, Yasumoto H. V-antigen homologs in pathogenic gram-negative bacteria. Microbiol Immunol 2014; 58(5):267-85; PMID:24641673; http://dx.doi.org/10.1111/1348-0421.12147
- Overheim KA, DePaolo RW, DeBord KL, Morrin EM, Anderson DM, Green NM, Brubaker RR, Jabri B, Schneewind O. LcrV plague vaccine with altered immunomodulatory properties. Infect Immun 2005; 73(8):5152-9; PMID:16041032; http://dx.doi.org/10.1128/IAI.73.8.5152-5159.2005
- Quenee LE, Schneewind O. Plague vaccines and the molecular basis of immunity against Yersinia pestis. Hum Vaccin 2009; 5(12):817-23; PMID:19786842; http://dx.doi.org/10.4161/hv.9866
- Ivanov MI, Hill J, Bliska JB. Direct neutralization of type III effector translocation by the variable region of a monoclonal antibody to Yersinia pestis LcrV. Clin Vaccine Immunol 2014; 21(5):667-73; PMID:24599533; http://dx.doi.org/10.1128/CVI.00013-14
- Price SB, Cowan C, Perry RD, Straley SC. The Yersinia pestis V antigen is a regulatory protein necessary for Ca2(+)-dependent growth and maximal expression of low-Ca2+ response virulence genes. J Bacteriol 1991; 173(8):2649-57; PMID:1901573; http://dx.doi.org/10.1128/jb.173.8.2649-2657.1991
- Pettersson J, Holmström A, Hill J, Leary S, Frithz-Lindsten E, von Euler-Matell A, Carlsson E, Titball R, Forsberg A, Wolf-Watz H. The V-antigen of Yersinia is surface exposed before target cell contact and involved in virulence protein translocation. Mol Microbiol 1999; 32(5):961-76; PMID:10361299; http://dx.doi.org/10.1046/j.1365-2958.1999.01408.x
- Mota LJ. Type III secretion gets an LcrV tip. Trends Microbiol 2006; 14(5):197-200; PMID:16564172; http://dx.doi.org/10.1016/j.tim.2006.02.010
- Holmstrom A, Olsson J, Cherepanov P, Maier E, Nordfelth R, Pettersson J, Benz R, Wolf-Watz H, Forsberg A. LcrV is a channel size-determining component of the Yop effector translocon of Yersinia. Mol Microbiol 2001; 39(3):620-32; PMID:11169103; http://dx.doi.org/10.1046/j.1365-2958.2001.02259.x
- Broz P, Mueller CA, Müller SA, Philippsen A, Sorg I, Engel A, Cornelis GR. Function and molecular architecture of the Yersinia injectisome tip complex. Mol Microbiol 2007; 65(5):1311-20; PMID:17697254; http://dx.doi.org/10.1111/j.1365-2958.2007.05871.x
- Ekestubbe S, Broms JE, Edgren T, Fallman M, Francis MS, Forsberg A. The amino-terminal part of the needle-tip translocator LcrV of Yersinia pseudotuberculosis is required for early targeting of YopH and in vivo virulence. Front Cell Inf Microbiol 2016; 6:175; PMID:2799509; http://dx.doi.org/10.3389/fcimb.2016.00175
- Fields KA, Straley SC. LcrV of Yersinia pestis enters infected eukaryotic cells by a virulence plasmid-independent mechanism. Infect Immun 1999; 67(9):4801-13; PMID:10456934
- Motin VL, Nakajima R, Smirnov GB, Brubaker RR. Passive immunity to Yersiniae mediated by anti-recombinant V antigen and protein A-V antigen fusion peptide. Infect Immun 1994; 62(10):4192-4201; PMID:7927675
- Nedialkov YA, Motin VL, Brubaker RR. Resistance to lipopolysaccharide mediated by the Yersinia pestis V antigen-polyhistidine fusion peptide: Amplification of interleukin-10. Infect Immun 1997; 65(4):1196-1203; PMID:9119451
- Sing A, Roggenkamp A, Geiger AM, Heesemann J. Yersinia enterocolitica evasion of the host innate immune response by V antigen-induced IL-10 production of macrophages is abrogated in IL-10-deficient mice. J Immunol 2002; 168(3):1315-21; PMID:11801671; http://dx.doi.org/10.4049/jimmunol.168.3.1315
- Welkos S, Friedlander A, McDowell D, Weeks J, Tobery S. V antigen of Yersinia pestis inhibits neutrophil chemotaxis. Microb Pathog 1998; 24(3):185-96; PMID:9514641; http://dx.doi.org/10.1006/mpat.1997.0188
- Sodhi A, Sharma RK, Batra HV. Yersinia rLcrV and rYopB inhibits the activation of murine peritoneal macrophages in vitro. Immunol Lett 2005; 99(2):146-52; PMID:16009264; http://dx.doi.org/10.1016/j.imlet.2005.02.009
- Sing A, Rost D, Tvardovskaia N, Roggenkamp A, Wiedemann A, Kirschning CJ, Aepfelbacher M, Heesemann J. Yersinia V-antigen exploits toll-like receptor 2 and CD14 for interleukin 10-mediated immunosuppression. J Exp Med 2002; 196(8):1017-24; PMID:12391013; http://dx.doi.org/10.1084/jem.20020908
- Brubaker RR. Interleukin-10 and inhibition of innate immunity to Yersiniae: Roles of Yops and LcrV (V antigen). Infect Immun 2003; 71(7):3673-81; PMID:12819047; http://dx.doi.org/10.1128/IAI.71.7.3673-3681.2003
- Roggenkamp A, Geiger AM, Leitritz L, Kessler A, Heesemann J. Passive immunity to infection with Yersinia spp. mediated by anti-recombinant V antigen is dependent on polymorphism of V antigen. Infect Immun 1997; 65(2):446-51; PMID:9009295
- Saraiva M, O'Garra A. The regulation of IL-10 production by immune cells. Nat Rev Immunol 2010; 10(3):170-81; PMID:20154735; http://dx.doi.org/10.1038/nri2711
- Fioranelli M, Grazia RM. Twenty-five years of studies and trials for the therapeutic application of IL-10 immunomodulating properties. From high doses administration to low dose medicine new paradigm. J Integr Cardiol 2015; 1(1):2-6
- O'Garra A, Barrat FJ, Castro AG, Vicari A, Hawrylowicz C. Strategies for use of IL-10 or its antagonists in human disease. Immunol Rev 2008; 223:114-31; PMID:18613832; http://dx.doi.org/10.1111/j.1600-065X.2008.00635.x
- Tian G, Li J, Wang D, Zhou D. Targeting IL-10 in auto-immune diseases. Cell Biochem Biophys 2014; 70(1):37-49; PMID:24639111; http://dx.doi.org/10.1007/s12013-014-9903-x
- Ma X, Yan W, Zheng H, Du Q, Zhang L, Ban Y, Li N, Wei F. Regulation of IL-10 and IL-12 production and function in macrophages and dendritic cells. F1000Res 2015; 4(F1000 Faculty Rev): 1465; http://dx.doi.org/10.12688/f1000research.6003.2
- Wei P, Wong WW, Park JS, Corcoran EE, Peisajovich SG, Onuffer JJ, Weiss A, Lim WA. Bacterial virulence proteins as tools to rewire kinase pathways in yeast and immune cells. Nature 2012; 488(7411):384-8; PMID:22820255; http://dx.doi.org/10.1038/nature11259
- Uusna J, Langel K, Langel Ü. Toxicity, immunogenicity, uptake, and kinetics methods for CPPs. Methods Mol Biol 2015; 1324:133-48; PMID:26202267; http://dx.doi.org/10.1007/978-1-4939-2806-4_9
- Kim HY, Yum SY, Jang G, Ahn D. Discovery of a non-cationic cell penetrating peptide derived from membrane-interacting human proteins and its potential as a protein delivery carrier. Sci Rep 2015; 5:11719; PMID:26114640; http://dx.doi.org/10.1038/srep11719
- Pan R, Xu W, Jafari M, Chen B, Sheinin T, Chen P. DEGylation enhanced the stability of peptide-siRNA complexes in serum. J Nanosci Nanotechnol 2015; 15(12):9982-90; PMID:26682443; http://dx.doi.org/10.1166/jnn.2015.12074
- Ruckdeschel K, Machold J, Roggenkamp A, Schubert S, Pierre J, Zumbihl R, Liautard JP, Heesemann J, Rouot B. Yersinia enterocolitica promotes deactivation of macrophage mitogen-activated protein kinases extracellular signal-regulated kinase-1/2, p38, and c-Jun NH2-terminal kinase. Correlation with its inhibitory effect on tumor necrosis factor-alpha production. J Biol Chem 1997; 272(25):15920-7; PMID:9188492; http://dx.doi.org/10.1074/jbc.272.25.15920
- Green SP, Hartland EL, Robins-Browne RM, Phillips WA. Role of YopH in the suppression of tyrosine phosphorylation and respiratory burst activity in murine macrophages infected with Yersinia enterocolitica. J Leukoc Biol 1995; 57(6):972-7; PMID:7790780
- Ruckdeschel K, Roggenkamp A, Schubert S, Heesemann J. Differential contribution of Yersinia enterocolitica virulence factors to evasion of microbicidal action of neutrophils. Infect Immun 1996; 64(3):724-33; PMID:8641773
- Visser LG, Seijmonsbergen E, Nibbering PH, van den Broek P J, van Furth R. Yops of Yersinia enterocolitica inhibit receptor-dependent superoxide anion production by human granulocytes. Infect Immun 1999; 67(3):1245-50; PMID:10024567
- Viboud GI, So SSK, Ryndak MB, Bliska JB. Proinflammatory signalling stimulated by the type III translocation factor YopB is counteracted by multiple effectors in epithelial cells infected with Yersinia pseudotuberculosis. Mol Microbiol 2003; 47(5):1305-15; PMID:12603736; http://dx.doi.org/10.1046/j.1365-2958.2003.03350.x
- Black DS, Bliska JB. Identification of p130Cas as a substrate of Yersinia YopH (Yop51), a bacterial protein tyrosine phosphatase that translocates into mammalian cells and targets focal adhesions. EMBO J 1997; 16(10):2730-44; PMID:9184219; http://dx.doi.org/10.1093/emboj/16.10.2730
- Persson C, Carballeira N, Wolf-Watz H, Fällman M. The PTPase YopH inhibits uptake of Yersinia, tyrosine phosphorylation of p130Cas and FAK, and the associated accumulation of these proteins in peripheral focal adhesions. EMBO J 1997; 16(9):2307-18; PMID:9171345; http://dx.doi.org/10.1093/emboj/16.9.2307
- Black DS, Montagna LG, Zitsmann S, Bliska JB. Identification of an amino-terminal substrate-binding domain in the Yersinia tyrosine phosphatase that is required for efficient recognition of focal adhesion targets. Mol Microbiol 1998; 29(5):1263-74; PMID:9767593; http://dx.doi.org/10.1046/j.1365-2958.1998.01014.x
- Mecsas J, Raupach B, Falkow S. The Yersinia Yops inhibit invasion of Listeria, Shigella and Edwardsiella but not Salmonella into epithelial cells. Mol Microbiol 1998; 28(6):1269-81; PMID:9680215; http://dx.doi.org/10.1046/j.1365-2958.1998.00891.x
- Deleuil F, Mogemark L, Francis MS, Wolf-Watz H, Fällman M. Interaction between the Yersinia protein tyrosine phosphatase YopH and eukaryotic Cas/Fyb is an important virulence mechanism. Cell Microbiol 2003; 5(1):53-64; PMID:12542470; http://dx.doi.org/10.1046/j.1462-5822.2003.00236.x
- Hamid N, Gustavsson A, Andersson K, McGee K, Persson C, Rudd CE, Fällman M. YopH dephosphorylates Cas and Fyn-binding protein in macrophages. Microb Pathog 1999; 27(4):231-42; PMID:10502464; http://dx.doi.org/10.1006/mpat.1999.0301
- Black DS, Marie-Cardine A, Schraven B, Bliska JB. The Yersinia tyrosine phosphatase YopH targets a novel adhesion-regulated signalling complex in macrophages. Cell Microbiol 2000; 2(5):401-14; PMID:11207596; http://dx.doi.org/10.1046/j.1462-5822.2000.00061.x
- Yuan M, Deleuil F, Fällman M. Interaction between the Yersinia tyrosine phosphatase YopH and its macrophage substrate, Fyn-binding protein, Fyb. J Mol Microbiol Biotechnol 2005; 9(3–4):214-23; PMID:16415594; http://dx.doi.org/10.1159/000089649
- Bliska JB, Black DS. Inhibition of the Fc receptor-mediated oxidative burst in macrophages by the Yersinia pseudotuberculosis tyrosine phosphatase. Infect Immun 1995; 63(2):681-5; PMID:7822039
- Fällman M, Andersson K, Hakansson S, Magnusson KE, Stendahl O, Wolf-Watz H. Yersinia pseudotuberculosis inhibits Fc receptor-mediated phagocytosis in J774 cells. Infect Immun 1995; 63(8):3117-24; PMID:7622239
- Yao T, Mecsas J, Healy JI, Falkow S, Chien Y. Suppression of T and B lymphocyte activation by a Yersinia pseudotuberculosis virulence factor, yopH. J Exp Med 1999; 190(9):1343-50; PMID:10544205; http://dx.doi.org/10.1084/jem.190.9.1343
- Rolán HG, Durand EA, Mecsas J. Identifying Yersinia YopH-targeted signal transduction pathways that impair neutrophil responses during in vivo murine infection. Cell Host Microbe 2013; 14(3):306-17; PMID:24034616; http://dx.doi.org/10.1016/j.chom.2013.08.013
- La Puerta ML, de, Trinidad AG, del Carmen Rodríguez M, Bogetz J, Sánchez Crespo M, Mustelin T, Alonso A, Bayón Y. Characterization of new substrates targeted by Yersinia tyrosine phosphatase YopH. PLoS One 2009; 4(2):e4431; PMID:19221593; http://dx.doi.org/10.1371/journal.pone.0004431
- Choi HW, Brooking-Dixon R, Neupane S, Lee C, Miao EA, Staats HF, Abraham SN. Salmonella typhimurium impedes innate immunity with a mast-cell-suppressing protein tyrosine phosphatase, SptP. Immunity 2013; 39(6):1108-20; PMID:24332031; http://dx.doi.org/10.1016/j.immuni.2013.11.009