ABSTRACT
Cronobacter sakazakii 505108 was isolated from a sputum specimen of a neonate with severe pneumonia. C. sakazakii 505108 co-harbors 3 resistance plasmids of the IncHI2, IncX3, and IncFIB incomparability groups, respectively. These 3 plasmids have acquired several accessory modules, which carry an extremely large number of resistance genes, especially including those involved in resistance to carbapenems, aminoglycoside, tetracyclines, and phenicols and sulphonamide/trimethoprim. These plasmid-borne antibiotic resistance genes were associated with insertion sequences, integrons, and transposons, indicating that the assembly and mobilization of the corresponding accessory modules with complex chimera structures are facilitated by transposition and/or homologous recombination. This is the first report of fully sequence plasmids in clinical Cronobacter, which provides a deeper insight into plasmid-mediated multi-drug resistance in Cronobacter from hospital settings.
Introduction
Enterobacter sakazakii was initially defined in 1980 and reclassified into a new genus Cronobacter in 2007,Citation1 currently composed of 7 species.Citation2 Cronobacter species are motile, non-sporeforming, peritrichous rods within the Enterobacteriaceae family and ubiquitously distributed in nature. Cronobacter can cause serious infections in neonates and infants, especially those premature or with low birth weight,Citation3-5 and infections in elderly and immunocompromised adults have also been reported.Citation6,7 C. sakazakii, C. malonaticus and C. turicensis are considered as opportunistic human pathogens and account for the majority of clinical isolates of Cronobacter.Citation3-5 Cronobacter-induced neonatal infections manifest as necrotizing enterocolitis, meningitis, septicaemia and severe pneumonia with mortality rates of 40–80%, and in most cases are epidemiologically associated with ingestion of contaminated powdered infant formula.Citation3-5 The number of Cronobacter infection cases is underestimated due to misidentification of Cronobacter as other species such as Enterobacter cloacae.
Cronobacter isolates are generally susceptible to the most commonly clinically used antimicrobial agents, but resistance to one or more old-generation antimicrobials such as cephalothin, streptomycin, gentamicin and tetracycline has developed in a few Cronobacter isolates.Citation8,9 The production of chromosomal AmpC β-lactamases, including CSA-1 and CSA-2 in C. sakazakii, and CMA-1 and CMA-2 in C. malonaticus, confers the resistance exclusively to the first generation cephalosporins (e.g. cephalothin).Citation10 The tetA(B) gene and additional unknown determinants for tetracycline resistance have been reported in an environmental tetracycline-resistant Cronobacter isolate.Citation11 A multi-drug resistant (MDR) C. sakazakii isolate of animal origin co-harbors an IncI2 plasmid pWF-5–19C_mcr-1 (accession number KX505142) carrying mcr-1 (colistin resistance) and an IncB/O plasmid pWF-5–19C_NDM (accession number KX505142) containing fosA3 (fosfomycin resistance) and blaNDM-9 (carbapenem resistance).Citation12 pWF-5–19C_NDM is partially sequenced, while pWF-5–19C_mcr-1 represents the single fully sequenced antibiotic resistance plasmid in Cronobacter.
Co-existence of blaVEB-1 (extended-cephalosporin resistance), qnrA (quinolone resistance) and arr-2 (rifampin resistance) in a plasmid-borne class 1 integron has been identified in a nosocomial MDR Cronobacter isolate.Citation13,14 These examples represent the few reports of plasmid-mediated MDR in clinical Cronobacter isolates, but neither the integron nor the plasmid has been fully sequenced.
This study deals with detailed genetic characterization of 3 resistance plasmids co-existing in a MDR C. sakazakii isolate causing severe neonatal pneumonia. These 3 plasmids carry a total of 22 non-redundant genes or gene loci involved in resistance to antimicrobials and heavy metals. This is the first report of fully sequenced antibiotic resistance plasmids in Cronobacter of clinical origin.
Results and discussion
Case report
On April 28 2016, a female neonate with hyperpyrexia, bradypsychia, hyperspasmia, refusal to feed, recurrent apnea and severe skin jaundice was hospitalized in a public children's hospital in Nanjing City, China, and diagnosed to have bilirubin encephalopathy accompanied with severe pneumonia. Once hospitalized, the patient received a series of symptomatic treatments, especially including nutrition support therapy, exchange transfusion, neonatal phototherapy, mechanical ventilation; in addition, empirical intravenous antimicrobial treatment with latamoxef. Bacterial isolates were repeatedly recovered from the sputum specimens during routine sampling and cultivation from April 30th to May 5th, and one of these isolates was designated 505108. Based on the antimicrobial susceptibility test results, the antibiotic therapy was switched to intravenous administration with erythromycin since May 1st. Her symptoms associated with bilirubin encephalopathy and pneumonia gradually improved.
C. sakazakii co-harboring 3 resistance plasmids
PCR detection, followed by PCR amplicon sequencing, disclosed that the 505108 isolate carried the 2 C. sakazakii signature sequences of cgcA and gyrB. The multilocus sequence typing (MSLT) showed that the 505108 isolate belonged to the C. sakazakii sequence type 1 (ST1), with an allelic profile 1–1–1–1–1–1–1 corresponding to the 7 housekeeping gens atpD, fusA, glnS, gltB, gyrB, infB and pps. PCR screening indicated the presence of blaNDM-1, but none of the other carbapenemase genes were found in C. sakazakii 505108.
The 505108 isolate was found to harbor 3 plasmids, designated p505108-MDR, p505108-NDM and p505108-T6SS, which had circularly closed DNA sequences of 312,880 bp, 53,793 bp and 139,553 bp in length with mean G+C contents of 47.7%, 49.0% and 56.4%, and contained 359, 62 and 126 predicted open reading frames (ORFs), respectively (Figure S1 and ).
Table 1. Major features of plasmids analyzed.
Each plasmid was composed of the backbone regions, together with the accessory modules that were recognized as acquired DNA regions associated with and bordered by mobile elements and inserted at different sites of the backbone (Figure S1). A total of 22 non-redundant genes or gene loci, which were involved in resistance to antimicrobials (β-lactams including carbapenems, quinolons, aminoglycosides, tetracyclines, phenicols, sulphonamides, trimethoprims, rifampicins, bleomycin and acriflavin) and heavy metals (arsenic, copper, mercury, nickel/cobalt and tellurium), were found not only in the accessory modules but also in the backbones of these 3 plasmids ( and ).
Table 2. Drug resistance genes in sequenced plasmids.
p505108-NDM could be transferred into E. coli through conjugation, generating the transconjugant 505108-NDM-EC600 (). Repeated attempts failed to transfer p505108-MDR or p505108-T6SS into E. coli through conjugation and electroporation. Class B carbapenemase activity was observed in both 505108 and 505108-NDM-EC600 (data not shown), which was due to production of NDM enzyme in these 2 strains.
Table 3. Antimicrobial drug susceptibility profiles.
The 505108 isolate was resistant to ampicillin, ceftazidime, meropenem, cefoxitin, aztreonam, amikacin, minocycline, chloramphenicol, trimethoprim and sulfamethoxazole, but remained susceptible to nitrofurantoin, ciprofloxacin, azithromycin, fosfomycin, tigecycline and colistin; as expected, the transconjugant 505108-NDM-EC600 was resistant to ampicillin, ceftazidime, meropenem, cefoxitin and aztreonam, but remained susceptible to all the other drugs tested ().
General features of p505108-MDR
The p505108-MDR backbone had 95% BLAST query coverage and 99% nucleotide identity to the reference IncHI2 plasmid R478,Citation15 and these 2 plasmids shared the core IncHI2 backbone markers including repHI2A and repHI2B for replication initiation, parAB and parMR for partition, and the tra1 and tra2 regions for conjugal transfer (Figure S1 and S2).
Whole genome comparison of p505108-MDR and R478 disclosed 10 different regions (DFRs), designated DFR-1 to DFR-10 (Figure S2). A ΔIS903D element (DFR-1) was inserted between parR and htdA within the tra2 region of p505108-MDR, probably making p505108-MDR non-conjugative. DFR-2 was located between orf564 and orf312 and organized as the hipB to orf411 backbone region, Tn6362, the orf189 to orf258 backbone region in p505108-MDR, but manifested as the Tn1696-Tn6322 region in R478; the acquisition of Tn1696-Tn6322 resulted in the loss of the above 2 small backbone regions from R478. Tn2 (DRF-3) was inserted into orf159 (splitting it into 2 separate parts) in p505108-MDR, which left 5-bp direct repeats (DRs; target site duplication signals of transposition) at both ends of Tn2. DFR-4 existed as the 11.4-kb sil-cop region (conferring resistance to silver and copper) that was inserted between the 2 backbone genes orf159 and orf819 in R478, but as the 25-kb MDR-1 region in p505108-MDR. The 6.9-kb aphA1a region (DRF-5) was observed between int and mucAB in p505108-MDR, and its acquisition led to truncation of mucA and loss of the orf318 to retA region.
In R478, the class C tetracycline resistance transposon Tn10 (DRF-6) was inserted into orf300, while the IS186B element (DRF-7) existed between orf321 and ldrB. DRF-8 was composed of the relE to orf612 region and the ISCfr9-ISCfr15 region (both of which lacked resistance genes) in p505108-MDR, while it existed as an IS150 element flanked by 5-bp DRs in R478. The acquisition of ISCfr9-ISCfr15 by p505108-MDR and that of IS150 by R478 led to truncation of downstream orf606 and loss of the upstream relE to orf612 region, respectively. The 52.4 kb MDR-2 region (DFR-9) was inserted into klaB in p505108-MDR, leading to truncation of klaB as well as deletion of the downstream klaA-orf609 region. A second copy of IS903D (DFR-10) was inserted between orf2385 and orf450, leaving both of them truncated.
DFR-1, DFR-3 to DFR-7 and DFR-10 were entirely composed of accessory modules, while the other DFRs consisted of not only accessory modules but backbone regions; the acquisition of accessory modules induced deletion and/or truncation of surrounding backbone regions (Figure S2). Although p505108-MDR and R478 shared the overwhelming majority of their backbones, these 2 plasmids carried different profiles of accessory modules, most of which were inserted at different sites across the plasmid backbones.
There were in total 5 accessory modules containing resistance genes in p505108-MDR, namely the MDR-1 region (), the MDR-2 region (), Tn6362 (), the aphA1a region () and the blaTEM-1B-carrying Tn2 (Figure S4).
Figure 1. The MDR-1 region from p505108-MDR and comparison with related regions. Genes are denoted by arrows. Genes, mobile elements and other features are colored based on function classification. Shading denotes regions of homology (> 95% nucleotide identity). Numbers in brackets indicate the nucleotide positions within the corresponding plasmids.
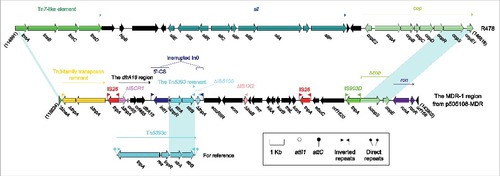
Figure 2. The MDR-2 region from p505108-MDR and comparison with related regions. Genes are denoted by arrows. Genes, mobile elements and other features are colored based on function classification. Shading denotes regions of homology (> 95% nucleotide identity). Numbers in brackets indicate the nucleotide positions within the corresponding plasmids.
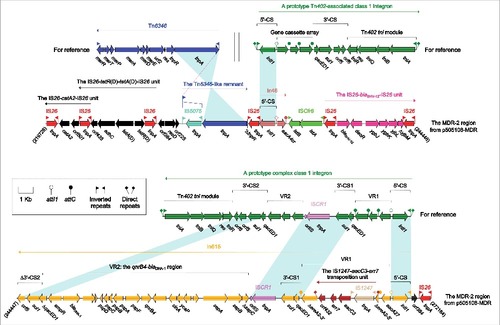
Figure 3. Tn6362 from p505108-MDR and comparison with related regions. Genes are denoted by arrows. Genes, mobile elements and other features are colored based on function classification. Shading denotes regions of homology (> 95% nucleotide identity). Numbers in brackets indicate the nucleotide positions within the corresponding plasmids.
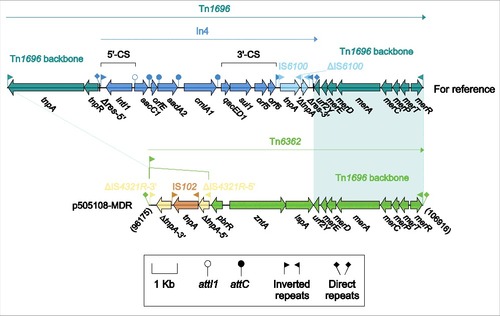
Figure 4. The aphA1a regions from p505108-MDR and p505108-T6SS and comparison with related regions. Genes are denoted by arrows. Genes, mobile elements and other features are colored based on function classification. Shading denotes regions of homology (> 95% nucleotide identity). Numbers in brackets indicate the nucleotide positions within the corresponding plasmids. The arrowheads indicated the location of PCR primers and the expected amplicons. See Figure S6 for the PCR results.
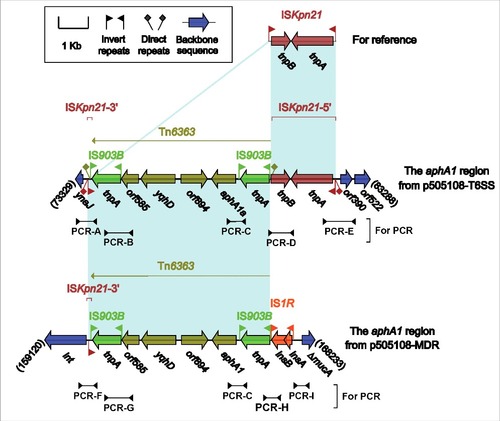
The MDR-1 region from p505108-MDR
The MDR-1 region () was a derivative of, although dramatically genetically differed from, the sil-cop region of R478 because these 2 modules were located at the same site of the IncHI2 backbone and had the same terminal regions. The sil-cop region of R478 carried a Tn7-like core transposition module tnsABCD and the silver (sil) and copper (cop) resistance loci. Various derivatives of the sil-cop region were found in several other IncHI2 plasmids.Citation16
Being dramatically distinct from the sil-cop region of R478, the MDR-1 region lost the entire sil and the most parts of tnsABCD and cop, but instead acquired several intact or residue mobile elements associated with resistance genes, especially including the dfrA18 region and an unusual In0 with paired terminal inverted repeats (TIRs). The dfrA genes were often associated with ISCR1Citation17 as observed in the dfrA18 region of p505108-MDR, in which ISCR1 was truncated due to its connection with upstream IS26. The prototype In0 was an empty class 1 integron, but a 1.9-kb Tn5393 remnant carrying the streptomycin resistance module strAB was integrated at a site downstream of the PcWTGN-10 promoter of In0 in p505108-MDR, interrupting In0 into 2 separate parts.
The MDR-2 region from p505108-MDR
The MDR-2 region () had a complex chimera structure, which carried 5 resistance-conferring substructures, namely the chloramphenicol resistance unit IS26-catA2-IS26, the tetracycline resistance unit IS26-tetR(D)-tetA(D)-IS26 [also designated TntetDCitation18], the extended-spectrum β-lactam resistance unit IS26-blaSHV-12-IS26, In46 and In615. The 3 IS26-flanking units lacked short DR sequences at both ends and were identified as IS26-composite transposon-like mobile elements.Citation19-21 A total of 7 copies of IS26 were found in the MDR-2 region, and the common IS26 component acts as an adaptor to mediate massive recombination and transposition events and thereby plays a pivotal role in assembly of large MDR regions with complex mosaic structures.Citation22,23
In46 from the MDR-2 region contained the 5′-conserved segment (5′-CS) and the sole gene cassette aacA4cr, but lacked the inverted repeat at the integrase end (IRi) and the 3′-terminal region composed of 3′-CS and inverted repeat at the tni end (IRt), which was resulted from connection of In46 with upstream IS26 and downstream ISCfr8. In615 was a complex class 1 integron that contained 2 resistance gene-carrying variable regions (VRs: VR1 and VR2). ISCR1 mobilized the nearby VR1 together with 3′-CS2 from one integron to 3′-CS1 of another integron, facilitating the formation of complex class 1 integrons.Citation17 The primary structure of VR1 from In615 was the gene cassette array aacA27-ereA2, in which ereA2 was interrupted into 2 separate parts by insertion of the IS1247-aacC3-arr7 transposition unit with 4-bp DRs at both ends.Citation24 The qnrB4-blaDHA-1 region (VR2) connected with ISCR1 was found several complex class 1 integrons carried on plasmids, including pCFI-1 (accession number JN215523), pCFI-2 (accession number JN215524), pCFI-3 (accession number JQ356870), pNMDHA (accession number GU943791), pRBDHA (accession number AJ971343) and pMPDHA (accession number AJ971344).Citation25
Tn6362 from p505108-MDR
Tn1696 (), a unit transposon of the Tn21 subgroup of Tn3 family, was assembled from insertion of class 1 integron In4 into the resolution (res) site of a backbone structure IRL (inverted repeat left)-tnpA (transposase)-tnpR (resolvase)-res-mer-IRR (inverted repeat right).Citation26 As a derivative of Tn1696, Tn6362 () retained the mer-IRR region but had 2 major modifications: i) IRL was interrupted by IS4321R (the IS1111 family members IS4321 and IS5075 target and are inserted at a specific position in the 38-bp TIRs of Tn21 subgroup transposons),Citation27 which was further interrupted by IS102; and ii) a pbrR-zntA-lspA region, probably involved in zinc uptake, was acquired instead of the tnpAR-res:In4 region. Tn6362 was bracketed by 5-bp DRs, indicating that mobilization of Tn6362 into p505108-MDR was a transposition process requiring the core transposition determinants (TIRs, tnpAR and res), and that the lesion or loss of these core determinants occurred post transposition.
Comparison of p505108-NDM with closely related pNDM-HN380
pP10159–1 showed >99% BLAST query coverage and >99% nucleotide identity to the first fully sequenced blaNDM-carrying IncX3 plasmid pNDM-HN380.Citation28 These 2 plasmids harbored 2 accessory modules, namely an ISKox3 element and an approximately 18-kb blaNDM-1 region containing 3 resistance genes blaNDM-1, bleMBL and blaSHV-12 (Figure S4). The blaNDM-1 regions of p505108-NDM and pNDM-HN380 might be generated from connection of the prototype blaNDM-1-carrying ISAba125-flanked composite transposon Tn125Citation29 with the upstream IS3000-ΔTn3 region and the downstream composite transposon-like IS26-blaSHV-12-IS26 unit,Citation21 making the truncation of Tn125 itself; moreover, an IS5 element was inserted into ISAba125 at the 5′-flank of Tn125, interrupting ISAba125 into 2 separate parts. These 2 blaNDM-1 regions slightly differed from each other by a 111-bp insertion at adjacent position between IS26-blaSHV-12-IS26 and ΔTn125 and also by a 304-bp deletion within the disrupted ISAba125.
Comparison of p505108-T6SS with closely related pESA3
p505108-T6SS and pESA3Citation30 had almost identical backbones (100% BLAST query coverage and >99% nucleotide identity) and carried a single IncFIB-type replication gene repA, the plasmid partition locus parAB, the toxin-antitoxin system locus hipAB for post-segregational killing, 4 virulence loci [including cpa (plasminogen activator; serum resistance and invasion),Citation31 eit and iuc (iron acquisition), and a type 6 secretion system (T6SS) locus] and 2 putative resistance loci acrAB and ars (Figure S5). The insertion of an 8.3-kb aphA1a region (see below) at a site between ynaJ and orf390 in p505108-T6SS accounted for the major modular difference between p505108-T6SS and pESA3 (Figure S5).
The aphA1a regions from p505108-MDR and p505108-T6SS
The presence of 2 highly similar aphA1a regions () in the 2 co-existent plasmids p505108-MDR and p505108-T6SS were validated, although highly unusual, by a set of PCR amplifications that targeted several key jointing fragments of these 2 aphA1a regions and their surrounding backbone regions, using genomic DNA of the 505108 isolate as template.
The aphA1a region of p505108-MDR was generated from 2 different transposition events: i) insertion of an ISKpn21 element (IRL-tnpAB-IRR) into the p505108-MDR backbone, and ii) that of an IS903B-flanked composite transposon Tn6363 carrying aphA1a at a site between tnpB and IRR of ISKpn21, interrupting ISKpn21 2 separate parts ΔISKpn21–5′ (IRL-tnpA-tnpB) and ΔISKpn21–3′ (IRR). These 2 transposition events left 5-bp and 9-bp DRs bracketing ISKpn21 and Tn6363, respectively.
The prototype aphA1a region (as observed in p505108-MDR) was likely connected with an IS1R element, which resulted from transposition or homologous recombination, generating the aphA1a region of p505108-T6SS with deletion of ΔISKpn21–5′ relative to p505108-MDR. pESA3 and its close derivatives including p505108-T6SS have been widely identified as virulence plasmids in pathogenic C. sakazakii strains.Citation32 Notably, acquisition of the aphA1a region by p505108-T6SS made it to be a carrier of not only virulence determinants but also antibiotic resistance markers.
Concluding remarks
Cronobacter species have the ability to survive in powdered infant formula, and C. sakazakii, C. malonaticus and C. turicensis represent dangerous opportunistic pathogens of neonates.Citation33 Cronobacter species tend to be more sensitive to most antibiotics than other Enterobacteriaceae species. There are few reports describing the MDR in Cronobacter isolates of both environmental and clinical origins, and molecular mechanisms of antimicrobial resistance in Cronobacter are poorly understood. C. sakazakii 505108, causing severe neonatal pneumonia, co-harbors 3 resistance plasmids belonging to the IncHI2, IncX3 and IncFIB incomparability groups, respectively. These 3 plasmids carry an extremely large number of resistance genes, and most of these plasmid-borne resistance genes were associated with insertion sequences, integrons and transposons, constituting various large accessory modules with chimera structures. Mobilization of these accessory resistance modules into plasmid backbones are promoted by transposition and homologous recombination. MDR in Cronobacter isolates leads to limited choice of antibiotics for treatment, resulting in a greater risk of death. Therefore, surveillance of plasmid-mediated MDR in clinical Cronobacter isolates is of paramount importance.
Materials and methods
Bacterial strains and identification
Bacterial species identification was performed using 16S rRNA gene sequencingCitation34 and PCR-detection of a 492-bp cgcA sequenceCitation35 and a 151-bp gyrB sequenceCitation36 specific for C. sakazakii. The MLST scheme for C. sakazakii was derived from the PubMLST database (https://pubmlst.org/cronobacter/).The major plasmid-borne carbapenemase genes were screened for by PCR.Citation37 All the PCR amplicons were sequenced on ABI 3730 Sequencer (LifeTechnologies, CA, USA) with the same primers as used for PCR.
Sequencing and annotation
Genomic DNA was isolated from the 505108 isolate using a Qiagen large construct kit and sequenced from a mate-pair library with average insert size of 5,000 bp, using a MiSeq sequencer (Illumina, CA, USA). DNA contigs were assembled using Newbler 2.6.Citation38 Gaps between contigs were filled using a combination of PCR and Sanger sequencing using an ABI 3730 Sequencer. Open reading frames and pseudogenes were predicted using RAST 2.0Citation39 combined with BLASTP/BLASTNCitation40 searches against the UniProtKB/Swiss-ProtCitation41 and RefSeqCitation42 databases. Annotation of resistance genes, mobile elements and other features was performed using CARD,Citation43 ResFinder,Citation44 ISfinderCitation45 and INTEGRALL.Citation46 Multiple and pairwise sequence comparisons were performed using MUSCLE 3.8.31Citation47 and BLASTN, respectively. Gene organization diagrams were drawn in Inkscape 0.48.1.
Plasmid transfer
Plasmids were transferred in attempt from the 505108 isolate into Escherichia coli TOP10 and EC600 (highly resistant to rifampicin) through electroporation and conjugal transfer, respectively.Citation48 For selection of the electroporant or transconjugant containing the markers repHI2A+strA (p505108-MDR), repB+blaNDM (p505108-NDM) and repA+aphA1a (p505108-T6SS), the antibiotics amikacin (20 μg/ml), imipenem (2 μg/ml) and rifampicin (1000 μg/ml) were used in accordance with specific circumstances.
Phenotypic assays
Activity of Ambler class A/B/D carbapenemases in bacterial cell extracts was determined by a modified CarbaNP test.Citation48 Bacterial antimicrobial susceptibility was tested by the broth dilution method and interpreted as per CLSI guidelines.Citation49
Nucleotide sequence accession numbers
The p505108-MDR, p505108-NDM and p505108-T6SS sequences were submitted to GenBank under accession numbers KY978628, KY978629 and KY978630, respectively.
Disclosure of potential conflicts of interest
No potential conflicts of interest were disclosed.
KVIR_S_1356537.zip
Download Zip (2.6 MB)Funding
This work was supported by the grants AWS15J006 and 2016YFC1202600 from the National Major Research & Development Program of China.
References
- Iversen C, Lehner A, Mullane N, Bidlas E, Cleenwerck I, Marugg J, Fanning S, Stephan R, Joosten H. The taxonomy of Enterobacter sakazakii: Proposal of a new genus Cronobacter gen. nov. and descriptions of Cronobacter sakazakii comb. nov. Cronobacter sakazakii subsp. sakazakii, comb. nov., Cronobacter sakazakii subsp. malonaticus subsp. nov., Cronobacter turicensis sp. nov., Cronobacter muytjensii sp. nov., Cronobacter dublinensis sp. nov. and Cronobacter genomospecies 1. BMC Evol Biol. 2007;7:64. http://doi.org/10.1186/1471-2148-7-64. PMID:17439656
- Stephan R, Grim CJ, Gopinath GR, Mammel MK, Sathyamoorthy V, Trach LH, Chase HR, Fanning S, Tall BD. Re-examination of the taxonomic status of Enterobacter helveticus, Enterobacter pulveris and Enterobacter turicensis as members of the genus Cronobacter and their reclassification in the genera Franconibacter gen. nov. and Siccibacter gen. nov. as Franconibacter helveticus comb. nov., Franconibacter pulveris comb. nov. and Siccibacter turicensis comb. nov., respectively. Int J Syst Evol Microbiol. 2014;64:3402-10. http://doi.org/10.1099/ijs.0.059832-0. PMID:25028159
- Holy O, Forsythe S. Cronobacter spp. as emerging causes of healthcare-associated infection. J Hosp Infect. 2014;86:169-77. http://doi.org/10.1016/j.jhin.2013.09.011. PMID:24332367
- Norberg S, Stanton C, Ross RP, Hill C, Fitzgerald GF, Cotter PD. Cronobacter spp. in powdered infant formula. J Food Prot. 2012;75:607-20. http://doi.org/10.4315/0362-028X.JFP-11-285. PMID:22410240
- Joseph S, Forsythe SJ. Predominance of Cronobacter sakazakii sequence type 4 in neonatal infections. Emerg Infect Dis. 2011;17:1713-5. http://doi.org/10.3201/eid1709.110260. PMID:21888801
- Patrick ME, Mahon BE, Greene SA, Rounds J, Cronquist A, Wymore K, Boothe E, Lathrop S, Palmer A, Bowen A. Incidence of Cronobacter spp. infections, United States, 2003–2009. Emerg Infect Dis. 2014;20:1520-3. http://doi.org/10.3201/eid2009.140545. PMID:25148394
- Holy O, Petrzelova J, Hanulik V, Chroma M, Matouskova I, Forsythe SJ. Epidemiology of Cronobacter spp. isolates from patients admitted to the Olomouc University Hospital (Czech Republic). Epidemiol Mikrobiol Imunol. 2014;63:69-72. PMID:24730997
- Xu X, Li C, Wu Q, Zhang J, Huang J, Yang G. Prevalence, molecular characterization, and antibiotic susceptibility of Cronobacter spp. in Chinese ready-to-eat foods. Int J Food Microbiol. 2015;204:17-23. http://doi.org/10.1016/j.ijfoodmicro.2015.03.003. PMID:25828706
- Li Z, Ge W, Li K, Gan J, Zhang Y, Zhang Q, Luo R, Chen L, Liang Y, Wang Q, et al. Prevalence and characterization of Cronobacter sakazakii in retail milk-based infant and baby foods in Shaanxi, China. Foodborne Pathog Dis. 2016;13:221-7. http://doi.org/10.1089/fpd.2015.2074. PMID:26886843
- Muller A, Hachler H, Stephan R, Lehner A. Presence of AmpC beta-lactamases, CSA-1, CSA-2, CMA-1, and CMA-2 conferring an unusual resistance phenotype in Cronobacter sakazakii and Cronobacter malonaticus. Microb Drug Resist. 2014;20:275-80. http://doi.org/10.1089/mdr.2013.0188. PMID:24568164
- Miranda CD, Kehrenberg C, Ulep C, Schwarz S, Roberts MC. Diversity of tetracycline resistance genes in bacteria from Chilean salmon farms. Antimicrob Agents Chemother. 2003;47:883-8. http://doi.org/10.1128/AAC.47.3.883-888.2003. PMID:12604516
- Liu BT, Song FJ, Zou M, Hao ZH, Shan H. Emergence of colistin resistance gene mcr-1 in Cronobacter sakazakii producing NDM-9 and in Escherichia coli from the same animal. Antimicrob Agents Chemother. 2017;61:e01444-16. http://doi.org/10.1128/AAC.01444-16. PMID:27855074
- Poirel L, Van De Loo M, Mammeri H, Nordmann P. Association of plasmid-mediated quinolone resistance with extended-spectrum β-lactamase VEB-1. Antimicrob Agents Chemother. 2005;49:3091-4. http://doi.org/10.1128/AAC.49.7.3091-3094.2005. PMID:15980408
- Girlich D, Poirel L, Leelaporn A, Karim A, Tribuddharat C, Fennewald M, Nordmann P. Molecular epidemiology of the integron-located VEB-1 extended-spectrum β-lactamase in nosocomial enterobacterial isolates in Bangkok, Thailand. J Clin Microbiol. 2001;39:175-82. http://doi.org/10.1128/JCM.39.1.175-182.2001. PMID:11136767
- Gilmour MW, Thomson NR, Sanders M, Parkhill J, Taylor DE. The complete nucleotide sequence of the resistance plasmid R478: Defining the backbone components of incompatibility group H conjugative plasmids through comparative genomics. Plasmid. 2004;52:182-202. http://doi.org/10.1016/j.plasmid.2004.06.006. PMID:15518875
- Sun F, Zhou D, Sun Q, Luo W, Tong Y, Zhang D, Wang Q, Feng W, Chen W, Fan Y, et al. Genetic characterization of two fully sequenced multi-drug resistant plasmids pP10164-2 and pP10164-3 from Leclercia adecarboxylata. Sci Rep. 2016;6:33982. http://doi.org/10.1038/srep33982. PMID:27658354
- Toleman MA, Bennett PM, Walsh TR. ISCR elements: Novel gene-capturing systems of the 21st century? Microbiol Mol Biol Rev. 2006;70:296-316. http://doi.org/10.1128/MMBR.00048-05. PMID:16760305
- Anantham S, Harmer CJ, Hall RM. p39R861-4, A type 2 A/C2 plasmid carrying a segment from the A/C1 plasmid RA1. Microb Drug Resist. 2015;21:571-6. http://doi.org/10.1089/mdr.2015.0133. PMID:26167918
- Kim MJ, Hirono I, Kurokawa K, Maki T, Hawke J, Kondo H, Santos MD, Aoki T. Complete DNA sequence and analysis of the transferable multiple-drug resistance plasmids (R Plasmids) from Photobacterium damselae subsp. piscicida isolates collected in Japan and the United States. Antimicrob Agents Chemother. 2008;52:606-11. http://doi.org/10.1128/AAC.01216-07. PMID:18070959
- Xiang DR, Li JJ, Sheng ZK, Yu HY, Deng M, Bi S, Hu FS, Chen W, Xue XW, Zhou ZB, et al. Complete sequence of a novel IncR-F33:A-:B- plasmid, pKP1034, harboring fosA3, blaKPC-2, blaCTX-M-65, blaSHV-12, and rmtB from an epidemic Klebsiella pneumoniae sequence type 11 strain in China. Antimicrob Agents Chemother. 2016;60:1343-8. http://doi.org/10.1128/AAC.01488-15
- Ford PJ, Avison MB. Evolutionary mapping of the SHV beta-lactamase and evidence for two separate IS26-dependent blaSHV mobilization events from the Klebsiella pneumoniae chromosome. J Antimicrob Chemother. 2004;54:69-75. http://doi.org/10.1093/jac/dkh251. PMID:15163647
- Harmer CJ, Moran RA, Hall RM. Movement of IS26-associated antibiotic resistance genes occurs via a translocatable unit that includes a single IS26 and preferentially inserts adjacent to another IS26. MBio. 2014;5:e01801-14. http://doi.org/10.1128/mBio.01801-14. PMID:25293759
- Harmer CJ, Hall RM. IS26-mediated formation of transposons carrying antibiotic resistance genes. mSphere. 2016;1:e00038-16. http://doi.org/10.1128/mSphere.00038-16. PMID:27303727
- Verdet C, Benzerara Y, Gautier V, Adam O, Ould-Hocine Z, Arlet G. Emergence of DHA-1-producing Klebsiella spp. in the Parisian region: Genetic organization of the ampC and ampR genes originating from Morganella morganii. Antimicrob Agents Chemother. 2006;50:607-17. http://doi.org/10.1128/AAC.50.2.607-617.2006. PMID:16436717
- Yim G, Kwong W, Davies J, Miao V. Complex integrons containing qnrB4-ampC -blaDHA-1 in plasmids of multidrug-resistant Citrobacter freundii from wastewater. Can J Microbiol. 2013;59:110-6. http://doi.org/10.1139/cjm-2012-0576. PMID:23461518
- Partridge SR, Brown HJ, Stokes HW, Hall RM. Transposons Tn1696 and Tn21 and their integrons In4 and In2 have independent origins. Antimicrob Agents Chemother. 2001;45:1263-70. http://doi.org/10.1128/AAC.45.4.1263-1270.2001. PMID:11257044
- Partridge SR, Hall RM. The IS1111 family members IS4321 and IS5075 have subterminal inverted repeats and target the terminal inverted repeats of Tn21 family transposons. J Bacteriol. 2003;185:6371-84. http://doi.org/10.1128/JB.185.21.6371-6384.2003. PMID:14563872
- Ho P, Li Z, Lo W, Cheung Y, Lin C, Sham P, Cheng V, Ng T, Que T, Chow K. Identification and characterization of a novel incompatibility group X3 plasmid carrying blaNDM-1 in Enterobacteriaceae isolates with epidemiological links to multiple geographical areas in China. Emerging Microbes Infections. 2012;1:e39. http://doi.org/10.1038/emi.2012.37. PMID:26038408
- Poirel L, Bonnin RA, Boulanger A, Schrenzel J, Kaase M, Nordmann P. Tn125-related acquisition of blaNDM-like genes in Acinetobacter baumannii. Antimicrob Agents Chemother. 2012;56:1087-9. http://doi.org/10.1128/AAC.05620-11. PMID:22143526
- Franco AA, Hu L, Grim CJ, Gopinath G, Sathyamoorthy V, Jarvis KG, Lee C, Sadowski J, Kim J, Kothary MH, et al. Characterization of putative virulence genes on the related RepFIB plasmids harbored by Cronobacter spp. Appl Environ Microbiol. 2011;77:3255-67. http://doi.org/10.1128/AEM.03023-10. PMID:21421789
- Franco AA, Kothary MH, Gopinath G, Jarvis KG, Grim CJ, Hu L, Datta AR, McCardell BA, Tall BD. Cpa, the outer membrane protease of Cronobacter sakazakii, activates plasminogen and mediates resistance to serum bactericidal activity. Infect Immun. 2011;79:1578-87. http://doi.org/10.1128/IAI.01165-10. PMID:21245266
- Joseph S, Desai P, Ji Y, Cummings CA, Shih R, Degoricija L, Rico A, Brzoska P, Hamby SE, Masood N, et al. Comparative analysis of genome sequences covering the seven Cronobacter species. PLoS One. 2012;7:e49455. http://doi.org/10.1371/journal.pone.0049455. PMID:23166675
- Feeney A, Kropp KA, O'Connor R, Sleator RD. Cronobacter sakazakii: Stress survival and virulence potential in an opportunistic foodborne pathogen. Gut Microbes. 2014;5:711-8. http://doi.org/10.4161/19490976.2014.983774. PMID:25562731
- Frank JA, Reich CI, Sharma S, Weisbaum JS, Wilson BA, Olsen GJ. Critical evaluation of two primers commonly used for amplification of bacterial 16S rRNA genes. Appl Environ Microbiol. 2008;74:2461-70. http://doi.org/10.1128/AEM.02272-07. PMID:18296538
- Carter L, Lindsey LA, Grim CJ, Sathyamoorthy V, Jarvis KG, Gopinath G, Lee C, Sadowski JA, Trach L, Pava-Ripoll M, et al. Multiplex PCR assay targeting a diguanylate cyclase-encoding gene, cgcA, to differentiate species within the genus Cronobacter. Appl Environ Microbiol. 2013;79:734-7. http://doi.org/10.1128/AEM.02898-12. PMID:23144142
- Huang CH, Chang MT, Huang L. Use of novel species-specific PCR primers targeted to DNA gyrase subunit B (gyrB) gene for species identification of the Cronobacter sakazakii and Cronobacter dublinensis. Mol Cell Probes. 2013;27:15-8. http://doi.org/10.1016/j.mcp.2012.08.004. PMID:22963906
- Chen Z, Li H, Feng J, Li Y, Chen X, Guo X, Chen W, Wang L, Lin L, Yang H, et al. NDM-1 encoded by a pNDM-BJ01-like plasmid p3SP-NDM in clinical Enterobacter aerogenes. Front Microbiol. 2015;6:294. http://doi.org/10.3389/fmicb.2015.00294. PMID:25926823
- Nederbragt AJ. On the middle ground between open source and commercial software - the case of the Newbler program. Genome Biol. 2014;15:113. http://doi.org/10.1186/gb4173. PMID:25180324
- Brettin T, Davis JJ, Disz T, Edwards RA, Gerdes S, Olsen GJ, Olson R, Overbeek R, Parrello B, Pusch GD, et al. RASTtk: A modular and extensible implementation of the RAST algorithm for building custom annotation pipelines and annotating batches of genomes. Sci Rep. 2015;5:8365. http://doi.org/10.1038/srep08365. PMID:25666585
- Boratyn GM, Camacho C, Cooper PS, Coulouris G, Fong A, Ma N, Madden TL, Matten WT, McGinnis SD, Merezhuk Y, et al. BLAST: A more efficient report with usability improvements. Nucleic Acids Res. 2013;41:W29-33. http://doi.org/10.1093/nar/gkt282. PMID:23609542
- Boutet E, Lieberherr D, Tognolli M, Schneider M, Bansal P, Bridge AJ, Poux S, Bougueleret L, Xenarios I. UniProtKB/Swiss-Prot, the manually annotated section of the uniprot knowledgebase: How to use the entry view. Methods Mol Biol. 2016;1374:23-54. http://doi.org/10.1007/978-1-4939-3167-5_2. PMID:26519399
- O'Leary NA, Wright MW, Brister JR, Ciufo S, Haddad D, McVeigh R, Rajput B, Robbertse B, Smith-White B, Ako-Adjei D, et al. Reference sequence (RefSeq) database at NCBI: Current status, taxonomic expansion, and functional annotation. Nucleic Acids Res. 2016;44:D733-45. http://doi.org/10.1093/nar/gkv1189. PMID:26553804
- Jia B, Raphenya AR, Alcock B, Waglechner N, Guo P, Tsang KK, Lago BA, Dave BM, Pereira S, Sharma AN, et al. CARD 2017: Expansion and model-centric curation of the comprehensive antibiotic resistance database. Nucleic Acids Res. 2017;45:D566-73; PMID:27789705; http://doi.org/10.1093/nar/gkw1004.
- Zankari E, Hasman H, Cosentino S, Vestergaard M, Rasmussen S, Lund O, Aarestrup FM, Larsen MV. Identification of acquired antimicrobial resistance genes. J Antimicrob Chemother. 2012;67:2640-4. http://doi.org/10.1093/jac/dks261. PMID:22782487
- Siguier P, Perochon J, Lestrade L, Mahillon J, Chandler M. ISfinder: The reference centre for bacterial insertion sequences. Nucleic Acids Res. 2006;34:D32-6. http://doi.org/10.1093/nar/gkj014. PMID:16381877
- Moura A, Soares M, Pereira C, Leitao N, Henriques I, Correia A. INTEGRALL: A database and search engine for integrons, integrases and gene cassettes. Bioinformatics. 2009;25:1096-8. http://doi.org/10.1093/bioinformatics/btp105. PMID:19228805
- Edgar RC. MUSCLE: Multiple sequence alignment with high accuracy and high throughput. Nucleic Acids Res. 2004;32:1792-7. http://doi.org/10.1093/nar/gkh340. PMID:15034147
- Feng W, Zhou D, Wang Q, Luo W, Zhang D, Sun Q, Tong Y, Chen W, Sun F, Xia P. Dissemination of IMP-4-encoding pIMP-HZ1-related plasmids among Klebsiella pneumoniae and Pseudomonas aeruginosa in a Chinese teaching hospital. Sci Rep. 2016;6:33419. http://doi.org/10.1038/srep33419. PMID:27641711
- CLSI. Performance standards for antimicrobial susceptibility testing: Twenty-fifth informational supplement M100-S25. Wayne, PA, USA: CLSI; 2015