ABSTRACT
In 2011, a new virus was isolated from pigs with influenza-like symptoms and subsequently also from cattle, which are the main reservoir of the virus. It is similar to Influenza C virus (ICV), a (predominantly) human pathogen, causing respiratory disease in children. Since the virus is unable to reassort with ICV (and based on several other criteria as discussed in the text) it is now officially named as Influenzavirus D (IDV), a new genus of the Orthomyxoviridae. We summarize the epidemiology, pathology and evolution of IDV and its biological characteristics with emphasis on the only glycoprotein HEF. Based on the limited data available we finally consider whether IDV represent a public health threat.
Introduction
Influenza A virus (IAV) represents a major public health concern, both for its pandemic potential and for the impact of seasonal influenza. The recurrence of IAV infections is due to antigenic drift of its major glycoprotein hemagglutinin (HA),Citation1 i. e. amino acid exchanges that weaken binding of antibodies generated during a previous IAV infection. The main animal reservoir of IAV is waterfowl, which harbor viruses having all possible combinations of 16 HA and 9 neuraminidase (NA) subtypes.Citation2,Citation3 Only 3 HA (H1, H2, and H3) and 2 NA (N1 and N2) subtypes have caused human epidemics,Citation4 defined as sustained, widespread, person-to-person transmission. Influenza epidemics usually occur if an IAV from animals enters the immunologically naive human population. This is often facilitated by antigenic shift, the reassortment of gene segments between 2 different viruses. This may occur in pigs that can be infected by both avian and human viruses.Citation5 In addition, avian influenza viruses often spread to poultry, where multiple subtypes of IAVs currently circulate all over the world, including H7N9 and H5N1 that pose the highest threat to public health.Citation6 Of note, due to the rapidly increasing numbers of infections of humans with the newly emerged highly pathogenic (HP) H7N9 influenza virus, the potential of H7N9 as the causative agent of a new human pandemic is of great concern.Citation7 Furthermore, there have been many reassortment events introducing various neuraminidase (NA) genes into the genetic backbone of the classical Asian HP H5N1. This led to novel H5Nx variants, such as H5N2, H5N5, H5N6 H5N8, H5N9 (among others) that still pose a potential threat to both poultry industry and public health.Citation8,Citation9 Two Influenza A-like viruses were recently also detected in bats, but since their HA and NA lack hemagglutination and neuraminidase activity, the hallmark of IAV, they might probably not easily cross the species barrier.Citation10
In contrast, humans are the primary reservoir of Influenza B virus (IBV).Citation11 The virus infects both the upper and lower respiratory tract and causes symptoms like IAV.Citation12,Citation13 However, IAV and IBV have significant differences in evolution and epidemiology; the limited subtype diversity and gene reassortment may be the reason for the narrower host range of IBV.Citation14 IBV has similar genome structure as IAV, and antigenic drift of HA also occurs, but is more slowly compared with IAV.
Influenza C virus (ICV) causes infection of the upper respiratory tract, especially in children, but clinical symptoms are typically mild.Citation15 Serological studies indicate that ICVhas a worldwide distribution and that most humans develop antibodies against the virus early in life.Citation16 ICV is much more antigenically stable than IAV, and a high degree of cross-reactivity is observed among isolates of ICV.Citation17 The reservoir of influenza C virus are humans, but the virus also infects pigs and some strains could be experimentally transmitted from pig to pig.Citation18 The genome of ICV consists of 7 gene segments and encodes only one glycoprotein (HEF), which combines both the function of HA and NA of IAV or IBV.Citation19 The existing ICV strains are subclassified into 6 genetic and antigenic lineages, but reassortment between viruses of different lineages occurred frequently, and newly emerged reassortant viruses replaced previously circulating ones and became the epidemic strains.Citation20
A novel Influenza C-like virus, recently isolated from clinically-ill pigs and cattle became the founding member of a new genus of the Orthomyxoviridae, Influenzavirus D (IDV, https://talk.ictvonline.org/ictv-reports/ictv_online_report/). Considering the zoonotic potential, the interspecies transmission and the important public health significance of influenza viruses, it is urgently required to understand the characteristic of this novel virus. In this review, we summarize the epidemiology, pathology and molecular biology of IDV and consider its relevance for human and veterinary medicine.
Epidemiological and pathological characteristics of Influenza D virus
In 2011, an influenza C-like virus was isolated from clinically-ill pigs with influenza-like symptoms in the Midwest region of the USA.Citation21 Subsequently a very similar virus was isolated from other regions of the USA, Mexico, Asia (China, Japan) and Europe (France, Italy) in cattle, a species that was never considered to be susceptible to Influenza virus infection.Citation22-27 In addition, antibody for IDV were also detected in small ruminants from USA and Canada.Citation28 To date, cattle are thought to be the primary natural reservoir for IDV according to epidemiological, serological and pathological studies.Citation24,Citation25,Citation29,Citation30 Phylogenetic analysis showed that these strains have only 50% overall amino acid identity to human influenza C viruses, a divergence like that observed between influenza A and B viruses. Accordingly, no cross reactivity was observed between the new virus strains and human ICV antisera. ICV also exhibits a new mechanism for generating the M1 protein and does not reassort with human influenza C virus and generate viable progeny.Citation21,Citation30 Based on these differences to ICV this virus was classified by the International Committee on Taxonomy of Viruses (ICTV) as a new genus of the family Orthomyxoviridae, named influenzavirus D.
Several studies have investigated the epidemiology of IDV in swine, cattle, human and small ruminants (). In pig serum samples the seropositivity rate in hemagglutination inhibition assays is low (9.5%),Citation21 indicating that the virus circulates in pigs, but is not widespread. The prevalence of IDV in cattle is higher, especially in calves. Two studies describe that ∼95% of newborn calves had high levels of maternal antibodies against IDV, which subsequently decreased in the next 6 months, causing increased susceptibility to IDV.Citation25,Citation31It thus seems likely that cattle are most sensitive to IDV by 6 months of age. These susceptible populations of young cattle might create a permissive environment for IDV infection that allows active transmission and generates the virus reservoir. Analyses of archived sera suggested that IDV has been circulating in the US cattle population (Nebraska and Mississippi) since at least 2003.Citation25,Citation31 A nationwide serological survey in Japan reports an average 30% seropositivity rate in cattle, but the positivity rates varied among regions.Citation27,Citation32 Infections of cattle with a very similar virus have been also reported for China and Europe (France and Italy) suggesting that IDV likely circulates widely in the world.Citation22,Citation24,Citation26
Figure 1. Reservoir host mode diagram of IDV. IDV was initially isolated from pigs and subsequently from bovine. Since the seroprevalence in bovine is much higher than in swine, bovine is considered to be the primary natural reservoir for IDV. IDV can be transmitted between cattle and also between pigs as indicated by the solid line. Antibody specific for IDV were also detected in sera form small ruminants (sheep and goat) and from humans, especially in people with cattle exposure, but no virus was isolated (indicated by dotted line), indicating that IDV may be transmitted from cattle to humans (dotted line)
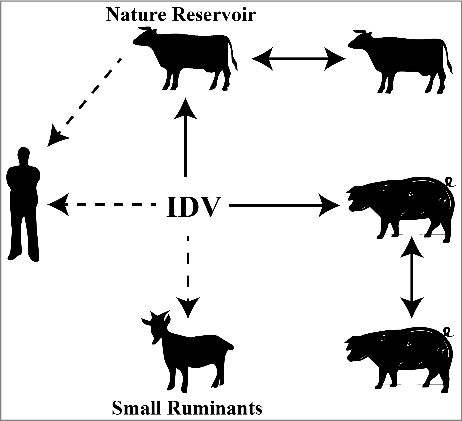
The intercontinental transmission and high prevalence of IDV, especially in cattle highlight its potential threat to other agricultural animals and humans. A serological survey was conducted to investigate the seroprevalence of IDV in small ruminants (sheep and goats) and poultry (chicken and turkey). The results show seropositivity rates of 5.2% for sheep and 8.8% for goats indicating that both are susceptible to IDV, while all tested poultry serum samples were negative for IDV antibodies.Citation28
Several surveys for infections of humans with IDV have also been conducted. Only ICV, but not IDV was identified by RT-PCR in archived human respiratory samples from Scotland.Citation33 However, serological surveys suggest that IDV might infect humans. Screening of serum samples indicate that 1.3% of the general human population have hemagglutination inhibiting antibodies against IDV.Citation21 Another 2 studies analyzed the seroprevalence against IDV in humans with occupational exposure to cattle. Whereas one study reported only 1% seroprevalence among 741 humans,Citation34 a study from Florida reported a very high seropositivity rate (∼95%) among 35 persons working with cattle.Citation35 In the latter study a rather high seroprevalence (18%) was also determined for non-cattle-exposed individuals. However, it was not excluded that the high seropositivity rate is (at least partly) due to antibodies made during an ICV infection since they cross-react with IDV. Clearly, more studies are required to estimate the percentage of humans infected with IDV. Furthermore, it would be interesting to know whether a high prevalence of IDV in humans correlates with an IDV outbreak in cattle in the same area. A newly developed real-Time RT-PCR assay might be a valuable tool for the detection of IDV strains.Citation36 Furthermore, it is unknown whether IDV causes disease in humans and is transmitted from person to person.
The pathology and mode of transmission of IDV was investigated by experimental infection of cattle and swine, but also of guinea pigs and ferrets. In pigs IDV replicates in nasal turbinates and virus shedding was detectable in nasal swabs. The virus was also transmitted to naive animals by direct contact. However, virus was not detected in trachea and lungs, indicating that virus replication was limited to the upper respiratory tract. No clinical symptoms and lesions typical of influenza were observed.Citation21
After experimental infection with IDV in cattle, virus can be detected both in upper and lower respiratory tract and transmitted to contact animals. Highest viral titers were present in nasal turbinates, decreasing in tissue lower in the respiratory tract. Virus infection causes seroconversion both in infected and contact animals, and also cause neutrophil infiltration in infected animals. However, the clinical signs (rhinitis and tracheitis) were rather mild in IDV-infected cattle, but statistically verified in comparison to uninfected controls.Citation30,Citation37,Citation38 The mild symptoms associated with experimental infection conflict with the observation that IDV is associated with a more pronounced disease in the field. This might be due to differences in infection routes and doses or husbandry conditions. However, it was also suggested that IDV alone is not sufficient to cause disease.Citation23 IDV infections might be part of the bovine respiratory disease (BRD) complex, which requires infection by one (or more) respiratory virus that predisposes the host for secondary bacterial colonization of the respiratory tract. Indeed, a recent metagenomic study showed that IDV, bovine adenovirus 3 and bovine rhinitis A virus were the top 3 viruses associated with BRD in dairy calves.Citation23 Previous studies have reported that IDV detection rates are higher in clinically sick cattle than in apparently healthy cattle, and tended to be significantly associated with (BRD).Citation23,Citation30,Citation38 An inactivated vaccine was recently developed, but it only partially protected cattle in challenge experiments.Citation37
The ferret is the best small mammalian model since it recapitulates the hallmarks of human influenza virus infection, such as clinical symptoms and airborne transmission. After experimental infection with IDV, virus can be detected in nasal turbinates, but not in the lower respiratory tract of the ferret. The virus was transmitted to sentinels by direct contact, but not by aerosol. Similar with swine infection, no clinical symptoms and lesions typical of influenza were observed.Citation21 In another study ferrets were exposed to fomites soaked in nasal discharge from infected calves, but they did not seroconvert and did not shed the virus.Citation38
Experimental infections were also conducted with guinea pigs. In contrast to ferrets, IDV replicates in both the upper and lower respiratory tract, including the lung, but no clinical symptoms were observed. IDV could transmit from infected to sentinel animals by direct contact, but not by aerosol.Citation39
Genome structure of Influenza D virus and encoded proteins
IDV has an identical genome structure as ICV, i. e. it consists of 7 gene segments containing negative-sense single-stranded RNA (). This contrasts with the genome of IAV and IBV that consists of 8 gene segments. Each of the 7 segments of ICV contains a noncoding (NC) region both at its 5′ and 3′ end. The first 11 nucleotides (3′-C/UCGUAUUCGUC-5′) at the 3′ end and the last 12 nucleotides (5′-AGCAGUAGCAAG-3′) at the 5′ end are identical between the 7 gene segments, except position 1 at the 3′ terminus, which is either cytosine or uridine.Citation21 The 3′ and 5′ sequences of IDV are also identical to those of ICV, except for the nucleotide at position 5 at the 3′end, which is adenine in IDV, but cytosine in ICV. These conserved regions form “panhandle” structures by partial inverted complementarity between the 5′ and 3′ NC regions. This unique structure serves as the promotor for transcription of mRNAs and plays a pivotal role in genome replication and packaging.Citation40-42 In addition, a uridine-rich region at the 5′ end of each segment in both ICV and IDV serves as a template for the poly A tail present at the 3′ end of each mRNA.Citation40 The 5′ and 3′ NC regions plus terminal coding sequences of each segment also contain the required information for packaging of viral RNPs into virus particles.Citation43 The high similarity of the conserved sequences at the ends of the gene segments suggests the potential for viral segment reassortment between ICV and IDV in nature. However, this was not observed under experimental conditions; IDV strains as well as ICV strains can exchange gene segments only with each other.Citation30,Citation44
Figure 2. Structure of the 7 influenza D virus genome segments. Influenza D virus particles possess 7 segments of minus-sense, single-stranded RNA. The open reading frames (ORFs) for each segment are marked red, the name and the size (number of amino acids) of the encoded proteins are indicated. The PB2, PB1, P3, HEF and NP proteins are translated from an ORF transcript, whereas M1/CM2 and NS1/NS2 are generated by splicing. Each segment contains 10 conserved nucleotides at its 3′ end and 12 conserved nucleotides at its 5′ end, the first nucleotide at 3′ terminus exhibits polymorphism as indicated in red color. A poly-U stretch close to the 5′ end is used to equip each mRNA with a poly-A tail
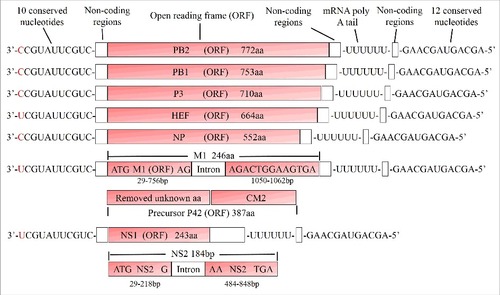
Very little experimental work has been performed on the function of the individual proteins of both ICV and IDV, but they have been extensively studied for IAV.Citation45 The 3 longest segments produce the proteins PB2, PB1 and PA/P3, respectively, which build the heterotrimeric polymerase complex that performs replication and viral mRNA synthesis. PB1 contains the polymerase active site (which is conserved in PB1 of IDV), whereas PB2 and PA/P3 comprise, respectively, cap-binding and endonuclease domains required for initiation of transcription by cap-snatching, a process during which a short nucleotide sequence is cleaved from the 5′ end of cellular mRNAs. The endonuclease active site of PA/P3 is identical between IDV and other influenza viruses. The guanine-binding residues of cap-binding domain are conserved between IDV and IAV, but the phosphate-binding residues are different. The protein encoded by the third segment is named P3 (instead of PA as in the case of IAV and IBV) since P3 of ICV does not exhibit a negative isoelectric point. However, the predicted isoelectric point of P3 of IDV is 6.2, which is between those of the influenza A/B (∼7.2) and influenza C virus (∼5.2).Citation21
The fourth segment encodes the glycoprotein HEF, the sole spike of the viral envelope, which is described in detail below. The fifth segment produces the nucleoprotein NP, which together with the polymerases and the vRNAs constitutes the viral ribonucleoprotein complex (vRNPs).Citation46 The sixth segment encodes 2 proteins, the matrix protein M1, a peripheral membrane protein that covers the viral membrane on its inside, and CM2, a small transmembrane protein that exhibits proton-channel activity.Citation19 In contrast to IAV, the larger protein M1 is translated from a spliced RNA, but details of splicing differ between ICV and IDV. The ICV M segment produces the M1 protein by introducing a termination codon into the mRNA, whereas splicing of the IDV M segment adds an additional 4-amino-acid peptide into the preceding exon. The unspliced mRNA is translated into the precursor protein p42, which contains an internal signal peptide that is subsequently cleaved to generate the integral membrane protein CM2.Citation30,Citation47 The seventh segment produces the 2 non-structural proteins NS1 and NS2 also by splicing. The unspliced mRNA is translated into the NS1 protein that counteracts the cellular interferon response Citation48,Citation59 and the spliced mRNA translates into the shorter NS2 protein, which is also designated nuclear export protein (NEP) since it mediates the nuclear export of RNPs.Citation49
Origin and evolution of Influenza D virus
The amino acid sequence identity between IDV and ICV is 50% for the whole genome and thus much lower in comparison to the 95% identity observed among the 6 lineages of human ICV. PB1, the most conserved viral protein (∼70% identity relative to ICV, but only ∼40% with IAV and IBV), is frequently used to evaluate the evolutionary relationship among influenza viruses.Citation21,Citation50 Here we constructed the maximum-likelihood phylogenetic tree based on nucleotide sequences of PB1 that include influenza A, B, C and D viruses to shed light on the phylogenetic relationship between them (). As expected, IDV clusters most closely with ICV suggesting that both viruses had a common ancestor. Using the PB2, P3, NP, M and NS genes for sequence comparison also indicates that IDV is derived from human ICV.Citation21 In addition, Bayesian analysis was conducted to address the origin and evolutionary history of IDV (). The mean time for the most recent common ancestor (t-MRCA) for ICV (based on the HEF gene sequence) was estimated at 1896.2 of the common era (A.D.), which coincides with a previous study,Citation51 indicating that our analysis is unbiased. The t-MRCA of the HEF gene of ICV and IDV was estimated at 482 (A.D.) (95% HPD, 758 (B.C.) to 1468.8 (A.D.)), about 1534 y ago, which is a little earlier than previously reported.Citation52 Based on the comparison of the nucleotide sequence of the HEF gene, 2 distinct co-circulating lineages represented by D/swine/Oklahoma/1334/2011and D/bovine/Oklahoma/660/2013 have been identified (), which frequently reassort with each other. They show antigenic cross-reactivity, but it is reduced up to 10-fold between both prototype viruses using heterologous compared with homologous antiserum.Citation29 We also estimated that the 2 lineages of IDV share a most recent common ancestor (t-MRCA) just about 44.6 y ago, suggesting that these 2 lineages emerged only recently. Whether another new lineage will emerge needs to be monitored. The mean substitution rate for the HEF gene of IDV was calculated by Bayesian analysis to be 1.54 × 10−3 (95% HPD, 5.4 × 10−4 to 2.7 × 10−3) per site and per year. It is thus faster than estimated for HEF of ICV alone analyzed in a previous study,Citation51 suggesting that the substitution rate for HEF from IDV gene may be faster than HEF from ICV. Thus, continuous monitoring of the evolution of IDV needs to be conducted in the future.
Figure 3. Phylogenetic analysis and evolution history of IDV. (A) Phylogenetic tree of Influenza viruses based on the nucleotide sequences of the PB1 gene. All sequences were aligned with ClustalW and the phylogenetic tree was constructed by maximum likelihood method in combination with 1,000 bootstrap replicates in MEGA 5.0.Citation70 Bootstrap values larger than 95% are shown for the major nodes; scale bars indicate the number of substitutions per site. Influenza A, B, C and D virus cluster independently, indicated in red (IAV), pink (IBV), light green (ICV) and blue (IDV). IDV clusters most closely with influenza C virus. (B) Phylogenetic tree and temporal placement of HEF gene between ICV and IDV. HEF gene sequences of ICV and IDV were used for Bayesian Markov chain Monte Carlo analyses to estimate the rates of nucleotide substitutions (per site, per year) and the time to the most recent common ancestor (TMRCA). The maximum clade credibility (MCC) tree was inferred using the Bayesian evolutionary analysis by sampling trees (BEAST), implemented in the BEAST software v1.8 packageCitation71,Citation72 (http://beast.bio.ed.ac.uk). HKY+I+Г was chosen as the nucleotide substitution model and a relaxed molecular clock with a lognormal distribution was used to model rate variation among branches with a constant size model. Markov chain Monte Carlo (MCMC) algorithm was run for a 10 million step chain and sampled every 1,000 states, and 10% of the chain was removed as burn-in. The time to the most recent common ancestor (TMRCA) was estimated and posterior probability values provided an assessment of the degree of support for the key node of the tree. The different lineages of ICV and IDV are shown in different colors, the representative strains of the 2 lineages of IDV (D/swine/Oklahoma/1334/2011 and D/bovine/Oklahoma/660/2013) are shown in red
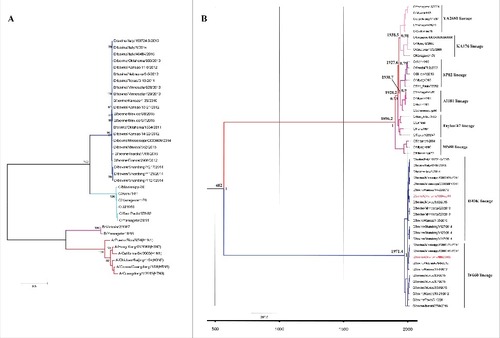
The HEF glycoproteins of ICV and IDV
Comparison of the crystal structures and protein modifications
The hemagglutinating glycoproteins of influenza viruses are typical type I membrane proteins with a cleavable N-terminal signal peptide, a large ectodomain, one transmembrane region and a short cytoplasmic tail (reviewed inCitation19,Citation53). Crystallography revealed that the overall structures as well as folds of individual segments of HA and HEF are quite similar.Citation54 They form a mushroom-shaped trimer consisting of a membrane-near stalk (containing the regions involved in membrane fusion, such as heptad repeats and the fusion peptide) and a globular head domain carrying the receptor-binding site. HEF contains an additional bulge located at the lower part of the globular domain that carries the esterase region not present in HA. Another particular feature of influenza C virus particles not observed for influenza A and B virions is the arrangement of spike proteins in a reticular, mainly hexagonal structure.Citation55,Citation56 The formation of a regular arrangement of HEF trimers on the plasma membrane might induce membrane curvature required for virus budding. Whether HEF of IDV also forms regular arrays in the viral membrane cannot be deduced from the currently available EM micrographs.Citation21
HEF of ICV and IDV have 55% amino acid identity and an almost identical structural fold.Citation57 Accordingly, the locations of the 6 disulphide bonds that stabilize the globular head of HEF and the one that connects HEF1 and HEF2, are completely conserved. Similar are also the parts of HEF not present in the crystalized ectodomain, such as the length of the transmembrane region (26 amino acids) and the very short, positively charged cytoplasmic tail (RTK in ICV and KK in IDV). Both HEFs contain cysteine residues at the end of the transmembrane region, one in ICV and 2 in IDV.Citation21 The cysteine of ICV HEF is stoichiometric acylated with stearic acid, a modification that (modestly) affects virus replication and the membrane fusion activity of HEF.Citation58,Citation59
A large part of the surface of HEF is covered by carbohydrates. The location of 5 glycosylation sites is completely conserved between ICV and IDV HEF, 2 sites are shifted by 10 or 12 residues (marked as gray balls in ). Interestingly, HEF of ICV contains a glycosylation site near the receptor-binding domain that is not present in IDV HEF (, S176). Instead, IDV HEF has one additional glycosylation site near the esterase domain (, N330) and another one in the shorter α-helix of HEF2 (N58).Citation21,Citation52 Furthermore, the consensus sequence compiled from the 27 HEF sequences in the database revealed another glycosylation site at N233 which is located in the 230-helix that confines the upper part of the receptor binding site (marked as red ball in , see also below and ). This glycosylation site is present in 21 HEF sequences, but not in the prototype strain D/swine/Oklahoma used for crystallisation of HEF. This glycosylation site, if used, might restrict access of HEF to its receptor and by its deletion the virus might acquire a broader cell tropism. Interestingly, experimental adaption of the H5N1 IAV to airborne transmission between ferrets led to loss of a glycosylation site located at a very similar position in HA, i. e. above the receptor binding site.Citation60,Citation61 Whether the other carbohydrates affect vital functions of HEF, such as proteolytic processing, accessibility of the receptor binding or esterase domain or might shield antibody epitopes, as demonstrated for HA is also not known.
Figure 4. Structure of the ectodomain of a HEF monomer from IDV. The polypeptide chains of HEF1 and HEF2 subunits are colored in red and blue, respectively. The amino acids of the receptor binding site are marked as green sticks, the catalytic triad of the esterase domain as blue sticks. The location of asparagine residues of N-glycosylation sites (Asn-X-Ser/Thr) that are conserved between ICV and IDV HEF are marked as light gray balls. (One further conserved but unused site is not present in the crystalized ectodomain of HEF). Glycosylation sites not conserved between ICV and IDV HEF are marked as dark gray balls. S176 is a used glycosylation site in ICV HEF which is not present in IDV HEF. N330 and N497 are 2 glycosylation sites present in IDV, but not ICV HEF. The consensus sequence of the 27 HEF sequences in the database revealed another glycosylation site NKT at N233 (marked as red ball), that is changed to NKA in the HEF used for crystallization. Numbering starts with the first amino acid present in the mature protein, assuming signal peptide cleavage after residues 16. The figure was created with PyMol from PDB file 5e64, rendering was done with Blender
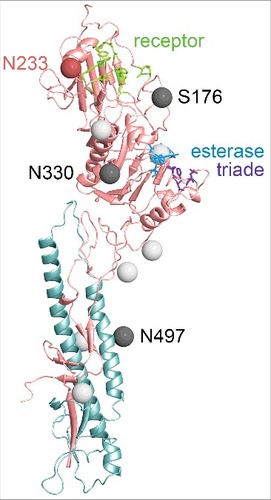
Figure 5. Comparison of the receptor-binding sites of ICV and IDV HEF. Surface presentations of the receptor-binding site of HEF from ICV (A) and IDV (B). The parts of the HEF1 molecule involved in receptor-binding (the 170 loop, the 230 helix, the 270 and 290 loops) are colored yellow. The 5 residues F127 (C HEF: Y127), W185 (C HEF: L184), Y231 (C HEF: Y227), F229 (C HEF: F225) and F297 (C HEF: F293) that form the bottom of the cavity are colored red. Note that K235 (blue) and D269 (red) form a salt bridge in ICV HEF that connects helix 230 with loop 270. At equivalent positions of IDV HEF A273 and T239 (colored red) are present that are not able to form a salt bridge. As a result, the receptor cavity of IDV HEF is more open and thus might allow binding of more 9-O-Ac-Neu5Ac derivatives. The figure was created with PyMol from PDB files 5e64 and1flc and rendering was done with Blender
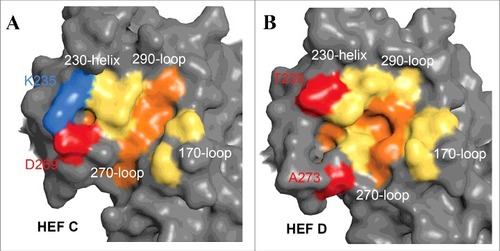
Comparison of the receptor-binding and -destroying sites
HA of IAV uses terminal N-acetylneuraminic acid (Neu5Ac) as receptor determinant and the glycosidic bond of Neu5Ac influence the host specificity. Avian influenza viruses usually bind to Neu5Ac-α2,3-gal while mammalian influenza viruses attach to Neu5Ac-α2,6-gal.Citation62 In contrast, HEF of ICV and IDV requires an acetylated derivative of Neu5Ac, namely N-acetyl-9-O-acetylneuraminic acid (9-O-Ac-Neu5Ac), but HEF binds to its receptor regardless of whether 9-O-Ac-Neu5Ac is attached via an α-2,6 or α-2,3 linkage to the following galactosyl-residue.Citation63 IDV HEF also tolerates small substitutions at the C5 position, such as esterification with glycolic acid instead of acetic acid.Citation57
The receptor-binding site of HEF from both ICV and IDV is located near the top of the globular head in a shallow cavity, surrounded by residues from 4 secondary structure elements: the 170-loop, 190-loop, 230-helix and 270-loop (). The most prominent difference between HEF of ICV and IDV is an open channel in the receptor-binding site of ICV HEF. The negatively-charged residue D269 and the positively-charged residue K235 form a salt bridge, pulling the 270-loop up to attach to the 230-helix. At equivalent positions in IDV HEF the amino acids T239 and A273 are present that cannot form a salt bridge. Thus, helix 230 is not connected with loop 270 and HEF of IVD therefore possesses an open channel (). It has been suggested that the more open receptor binding cavity of IDV HEF is the basis for the broader cell tropism of IDV but definitive proof of this assumption requires a detailed analysis of the ligand binding characteristics of ICV HEF.
Both ICV and IDV HEF bind to trachea sections from human, swine and bovine origin indicating that the relevant receptor determinant is present in all 3 species. However, binding does not necessarily prove that the cells can be infected.Citation57 This requires (at least) attachment to a receptor capable to undergo clathrin-mediated endocytosis and exposure of HEF to acidic pH to trigger its membrane fusion activity.
In agreement with its receptor binding specificity, the receptor-destroying activity of HEF is an esterase that cleaves acetyl from the C9 position of terminal 9-O-Ac-Neu5Ac residues.Citation57,Citation64 The esterase domain is the structurally most conserved part of HEF; the position of the catalytic triad characteristic for serine hydrolases (serine 57, aspartic acid 356 and histidine 359) is identical in ICV and IDV HEF. Interestingly, the HEF esterase of IDV is very active, even at 4°C. Only if the catalytic triad is inactivated HEF can hemagglutinate erythrocytes and binds to MDCK cells or bovine submaxillary mucins, which are enriched in 9-O-Ac-Neu5Ac.Citation57
Comparison of the proteolytic cleavage sites
To catalyze virus entry by membrane fusion HEF must be proteolytically processed into the larger HEF1 and the membrane-bound HEF2 subunits.Citation53 The molecular features of the cleavage site determine which of the many trypsin-like serine proteases recognize the precursor protein and the expression of this protease determines whether a certain cell type produces infectious virus particles. HEF from both ICV and IDV possess a monobasic cleavage site, a single arginine residue at the C-terminus of HEF1. HEF is in this respect similar to HAs from mammalian and low pathogenic avian influenza A viruses. Replication of these viruses is restricted to the site of virus infection, usually the respiratory tract. Spread to other organs or systemic infection, as it happens for highly pathogenic avian influenza virus having a multibasic cleavage site between HA1 and HA2, does not occur.Citation65
Interestingly, the amino acids surrounding the monobasic cleavage site differ between ICV and IDV HEF. HEF from ICV contains 3 closely spaced basic residues at the C-terminus of HEF2 (TVTKPKSR), whereas HEF from IDV contains only 2, widely spaced basic residues (RTLTPATR). In contrast, the N-terminal amino acids of the HEF2 subunit are completely conserved between ICV and IDV HEF (IFGIDDLI), but very different from the respective, strictly conserved amino acids of HA2 (GLFGAIAGFIE). The N-terminal amino acids of HEF2 and HA2 function as the fusion peptide, which is completely buried inside the trimeric structure of HA, but partly surface-exposed in HEF.Citation54,Citation57
The enzymes that activate HEF have not been identified, but in the case of human IAV and IBV, HAs with a monobasic cleavage site are proteolytic activated by members of the TMPRSS (transmembrane protein serine protease) family, mainly by TMPRSS2 and HAT (human airway trypsin-like protease), but a few HA subtypes are cleaved by TMPRSS4 and matriptase. These enzymes are expressed along the exocytic pathway or at the plasma membrane of human bronchial and tracheal epithelial cells. The homologous enzymes in swine have very similar characteristics with respect to cleavage specificity and (sub)cellular expression; information about the respective enzymes of bovine respiratory cells are not available.Citation65 Since the amino acids surrounding the cleavage site are part of the protease recognition motif it will be interesting to identify the enzymes which process HEF of ICV and IDV and analyze their expression in cells of the respiratory epithelium. Since only cells expressing the appropriate protease release infectious virus particles knowledge about the processing enzyme might be instrumental to explain the differences in cell tropism of IAV/IBV and ICV/IDV (upper versus lower respiratory tract) and host range of ICV and IDV (human and swine vs. bovine and swine). However, proteases secreted by certain bacteria might also activate the viral fusion proteins, as has been demonstrated for HA of IAV.Citation66
Temperature sensitivity of HEF
HEF of ICV exhibits intrinsic temperature sensitivity. Folding and trimerization of the molecule, surface expression and membrane fusion is less efficient at 37°C than at 33°C.Citation67 Probably as a consequence, ICV grows to higher titers in cell culture and chicken embryos at 33°C compared with 37°C.Citation68 This temperature sensitivity might be one of the factors that prevent replication of ICV in the lung that has a higher temperature compared with the upper respiratory tract. However, this temperature sensitivity is not preserved in IDV since the virus replicates efficiently at 37°C.Citation21
In summary, the structures of HEF of ICV and IDV are (almost) identical. Notable exceptions are the more open receptor-binding cavity in ICV HEF, the location of certain glycosylation sites and the amino acids surrounding HA cleavage site. Whether these features affect the host range of ICV and IDV needs to be explored.
Does Influenza D virus represent a public health threat?
Using the (very limited) information available on IDV we now assess the public health relevance of this emerging pathogen. The presence of antibodies against IDV in humans, especially with cattle exposure suggests that the virus can infect humans.Citation35 Likewise, ferrets, surrogates for human influenza virus infection, are also susceptible. Is it conceivable that IDV might acquire the ability to also invade the lower respiratory tract possibly creating more severe symptoms? In contrast to ICV, IDV replicates well in cell culture at 37°C,Citation21 the prevailing temperature in the lung. Likewise, the more open receptor binding cavity in HEF of IDV suggests that the virus can infect a wider range of cells compared with ICV.Citation57 Indeed, HEF of IDV binds to human trachea sections and the virus shows a wider tropism in cell culture.Citation57 Thus, there seems to be no inherent blockade that prevents IDV from invading the lower respiratory tract and to do so only a few adaptive genetic changes might be required.
However, very few amino acid exchanges are observed between the proteins of circulating viruses (both in ICV and IDV) suggesting that virus evolution is slow. Furthermore, the only surface glycoprotein HEF is antigenically stable indicating that antibody escape mutants might not occur frequently. Thus, infection with IDV (like infections with ICV) might cause long lasting immunity. The reason for the slow evolutionary change of ICV and IDV proteins is unknown. It might be either a low error rate of the polymerases and/or that viral proteins do not tolerate many amino acid changes without compromising their functionality.
The relevance of IDV for veterinary medicine needs to be further explored, i. e. whether the virus can cause disease on its own or only as part of the bovine respiratory disease (BRD) complex. Since IDV (in contrast to the other Influenza viruses) was isolated only a few years ago one might argue that its pathogenic potential is rather limited. However, one could also imagine that the pathogen may have spread to swine and cattle only recently, possibly from another still unknown natural animal reservoir. In addition, considering the proposed role of swine as the “mixing vessel” for reassortment between avian and human influenza A viruses to generate genetically novel, often pandemic viruses,Citation69 one might envision that pigs play a similar role in IDV ecology. Because swine are also sensitive to both ICV and IDV,Citation18,Citation21 continuous monitoring of pigs for reassortment of IDV is advisable. It is also unknown whether there are more genotypes of influenza C- and influenza D- like viruses circulating in cattle or other hosts. Further epidemiology survey of different animals should be conducted by veterinarians.
In summary, despite its zoonotic potential, the significance of IDV for public health seems to be rather low provided that the pathogenic potential and the antigenic stability of the virus does not change drastically. However, since properties and changes of influenza viruses are hard to predict, closer surveillance of the virus, especially in humans, is needed and efforts should be made to design a prototype vaccine that protects humans in case of an outbreak.
Disclosure of potential conflicts of interest
No potential conflicts of interest were disclosed.
Funding
The work of SS was financially supported by the National Key Research and Development Program of China (2017YFD0500101), the Fundamental Research Funds for the Central Universities (Y0201600147) and the Priority Academic Program Development of Jiangsu Higher Education Institutions. The work of MV on Influenza virus is financed by the German Research Foundation (DFG, SFB 740) and the Human Frontiers Science Program (HFSP). All the authors were financially supported by Funding of a joint project for Sino-German Cooperation on Agricultural Science and Technology (2016–2017), German part funded by the Federal Office for Agriculture and Food is also acknowledged. FXL gratefully acknowledge financial support from China Scholarship Council (CSC, 201708440274).
References
- Sandbulte MR, Westgeest KB, Gao J, Xu X, Klimov AI, Russell CA, Burke DF, Smith DJ, Fouchier RA, Eichelberger MC. Discordant antigenic drift of neuraminidase and hemagglutinin in H1N1 and H3N2 influenza viruses. Proceedings of the National Academy of Sciences of the United States of America. 2011;108:20748-53. doi:https://doi.org/10.1073/pnas.1113801108. PMID:22143798
- Yoon SW, Webby RJ, Webster RG. Evolution and ecology of influenza A viruses. Current topics in microbiology and Immunology. 2014;385:359-75. PMID:24990620
- Olsen B, Munster VJ, Wallensten A, Waldenstrom J, Osterhaus AD, Fouchier RA. Global patterns of influenza a virus in wild birds. Science. 2006;312:384-8. doi:https://doi.org/10.1126/science.1122438. PMID:16627734
- Watanabe T, Watanabe S, Maher EA, Neumann G, Kawaoka Y. Pandemic potential of avian influenza A (H7N9) viruses. Trends in Microbiology. 2014;22:623-31. doi:https://doi.org/10.1016/j.tim.2014.08.008. PMID:25264312
- Lakdawala SS, Jayaraman A, Halpin RA, Lamirande EW, Shih AR, Stockwell TB, Lin X, Simenauer A, Hanson CT, Vogel L, et al. The soft palate is an important site of adaptation for transmissible influenza viruses. Nature. 2015;526:122-5. doi:https://doi.org/10.1038/nature15379. PMID:26416728
- Su S, Bi Y, Wong G, Gray GC, Gao GF, Li S. Epidemiology, Evolution, and Recent Outbreaks of Avian Influenza Virus in China. J Virol. 2015;89:8671-6. doi:https://doi.org/10.1128/JVI.01034-15. PMID:26063419
- Zhang F, Bi Y, Wang J, Wong G, Shi W, Hu F, Yang Y, Yang L, Deng X, Jiang S, et al. Human infections with recently-emerging highly pathogenic H7N9 avian influenza virus in China. The Journal of Infection. 2017;75:71-5. doi:https://doi.org/10.1016/j.jinf.2017.04.001. PMID:28390707
- Zhao K, Gu M, Zhong L, Duan Z, Zhang Y, Zhu Y, Zhao G, Zhao M, Chen Z, Hu S, et al. Characterization of three H5N5 and one H5N8 highly pathogenic avian influenza viruses in China. Vet Microbiol. 2013;163:351-7. doi:https://doi.org/10.1016/j.vetmic.2012.12.025. PMID:23375651
- Gu M, Zhao G, Zhao K, Zhong L, Huang J, Wan H, Liu W, Liu H, Peng D, Liu X. Novel variants of clade 2.3.4 highly pathogenic avian influenza A(H5N1) viruses, China. Emerg Infect Dis. 2013;19:2021-4. doi:https://doi.org/10.3201/eid1912.130340. PMID:24274396
- Brunotte L, Beer M, Horie M, Schwemmle M. Chiropteran influenza viruses: flu from bats or a relic from the past? Current Opinion in Virology. 2016;16:114-9. doi:https://doi.org/10.1016/j.coviro.2016.02.003. PMID:26947779
- Osterhaus AD, Rimmelzwaan GF, Martina BE, Bestebroer TM, Fouchier RA. Influenza B virus in seals. Science. 2000;288:1051-3. doi:https://doi.org/10.1126/science.288.5468.1051. PMID:10807575
- Li WC, Shih SR, Huang YC, Chen GW, Chang SC, Hsiao MJ, Tsao KC, Lin TY. Clinical and genetic characterization of severe influenza B-associated diseases during an outbreak in Taiwan. J Clin Virol. 2008;42:45-51. doi:https://doi.org/10.1016/j.jcv.2007.11.026. PMID:18325832
- Chan PK, Chan MC, Cheung JL, Lee N, Leung TF, Yeung AC, Wong MC, Ngai KL, Nelson EA, Hui DS. Influenza B lineage circulation and hospitalization rates in a subtropical city, Hong Kong, 2000–2010. Clin Infect Dis. 2013;56:677-84. doi:https://doi.org/10.1093/cid/cis885. PMID:23074315
- Huang SS, Banner D, Paquette SG, Leon AJ, Kelvin AA, Kelvin DJ. Pathogenic influenza B virus in the ferret model establishes lower respiratory tract infection. J Gen Virol. 2014;95:2127-39. doi:https://doi.org/10.1099/vir.0.064352-0. PMID:24989173
- Matsuzaki Y, Katsushima N, Nagai Y, Shoji M, Itagaki T, Sakamoto M, Kitaoka S, Mizuta K, Nishimura H. Clinical features of influenza C virus infection in children. The Journal of Infectious Diseases. 2006;193:1229-35. doi:https://doi.org/10.1086/502973. PMID:16586359
- Salez N, Melade J, Pascalis H, Aherfi S, Dellagi K, Charrel RN, Carrat F, de Lamballerie X. Influenza C virus high seroprevalence rates observed in 3 different population groups. The Journal of Infection. 2014;69:182-9. doi:https://doi.org/10.1016/j.jinf.2014.03.016. PMID:24704348
- Matsuzaki Y, Mizuta K, Kimura H, Sugawara K, Tsuchiya E, Suzuki H, Hongo S, Nakamura K. Characterization of antigenically unique influenza C virus strains isolated in Yamagata and Sendai cities, Japan, during 1992–1993. J Gen Virol. 2000;81:1447-52. doi:https://doi.org/10.1099/0022-1317-81-6-1447. PMID:10811928
- Guo YJ, Jin FG, Wang P, Wang M, Zhu JM. Isolation of influenza C virus from pigs and experimental infection of pigs with influenza C virus. J Gen Virol. 1983;64(Pt 1):177-82. PMID:6296296
- Wang M, Veit M. Hemagglutinin-esterase-fusion (HEF) protein of influenza C virus. Protein Cell. 2016;7:28-45. doi:https://doi.org/10.1007/s13238-015-0193-x. PMID:26215728
- Matsuzaki Y, Mizuta K, Sugawara K, Tsuchiya E, Muraki Y, Hongo S, Suzuki H, Nishimura H. Frequent reassortment among influenza C viruses. J Virol. 2003;77:871-81. doi:https://doi.org/10.1128/JVI.77.2.871-881.2003. PMID:12502803
- Hause BM, Ducatez M, Collin EA, Ran Z, Liu R, Sheng Z, Armien A, Kaplan B, Chakravarty S, Hoppe AD, et al. Isolation of a novel swine influenza virus from Oklahoma in 2011 which is distantly related to human influenza C viruses. PLoS Pathogens. 2013;9:e1003176. doi:https://doi.org/10.1371/journal.ppat.1003176. PMID:23408893
- Chiapponi C, Faccini S, De Mattia A, Baioni L, Barbieri I, Rosignoli C, Nigrelli A, Foni E. Detection of Influenza D Virus among Swine and Cattle, Italy. Emerg Infect Dis. 2016;22:352-4. doi:https://doi.org/10.3201/eid2202.151439. PMID:26812282
- Mitra N, Cernicchiaro N, Torres S, Li F, Hause BM. Metagenomic characterization of the virome associated with bovine respiratory disease in feedlot cattle identified novel viruses and suggests an etiologic role for influenza D virus. J Gen Virol. 2016;97:1771-84. doi:https://doi.org/10.1099/jgv.0.000492. PMID:27154756
- Ducatez MF, Pelletier C, Meyer G. Influenza D virus in cattle, France, 2011–2014. Emerg Infect Dis. 2015;21:368-71. doi:https://doi.org/10.3201/eid2102.141449. PMID:25628038
- Ferguson L, Eckard L, Epperson WB, Long LP, Smith D, Huston C, Genova S, Webby R, Wan XF. Influenza D virus infection in Mississippi beef cattle. Virology. 2015;486:28-34. doi:https://doi.org/10.1016/j.virol.2015.08.030. PMID:26386554
- Jiang WM, Wang SC, Peng C, Yu JM, Zhuang QY, Hou GY, Liu S, Li JP, Chen JM. Identification of a potential novel type of influenza virus in Bovine in China. Virus Genes. 2014;49:493-6. doi:https://doi.org/10.1007/s11262-014-1107-3. PMID:25142163
- Murakami S, Endoh M, Kobayashi T, Takenaka-Uema A, Chambers JK, Uchida K, Nishihara M, Hause B, Horimoto T. Influenza D virus infection in Herd of Cattle, Japan. Emerg Infect Dis. 2016;22:1517-9. doi:https://doi.org/10.3201/eid2208.160362. PMID:27434213
- Quast M, Sreenivasan C, Sexton G, Nedland H, Singrey A, Fawcett L, Miller G, Lauer D, Voss S, Pollock S, et al. Serological evidence for the presence of influenza D virus in small ruminants. Vet Microbiol. 2015;180:281-5. PMID:26414999
- Collin EA, Sheng Z, Lang Y, Ma W, Hause BM, Li F. Cocirculation of two distinct genetic and antigenic lineages of proposed influenza D virus in cattle. J Virol. 2015;89:1036-42. PMID:25355894
- Hause BM, Collin EA, Liu R, Huang B, Sheng Z, Lu W, Wang D, Nelson EA, Li F. Characterization of a novel influenza virus in cattle and Swine: proposal for a new genus in the Orthomyxoviridae family. Mbio. 2014;5:e00031-14. PMID:24595369
- Luo J, Ferguson L, Smith DR, Woolums AR, Epperson WB, Wan XF. Serological evidence for high prevalence of Influenza D viruses in cattle, Nebraska, United States, 2003–2004. Virology. 2017;501:88-91. PMID:27888742
- Horimoto T, Hiono T, Mekata H, Odagiri T, Lei Z, Kobayashi T, Norimine J, Inoshima Y, Hikono H, Murakami K, et al. Nationwide distribution of Bovine Influenza D virus infection in Japan. Plos One. 2016;11:e0163828. PMID:27682422
- Smith DB, Gaunt ER, Digard P, Templeton K, Simmonds P. Detection of influenza C virus but not influenza D virus in Scottish respiratory samples. J Clin Virol. 2016;74:50-3. PMID:26655269
- Eckard LE. Assessment of the zoonotic potential of a novel bovine influenza virus [dissertation]. Tennessee (USA): The University of Tennessee. 2016
- White SK, Ma W, McDaniel CJ, Gray GC, Lednicky JA. Serologic evidence of exposure to influenza D virus among persons with occupational contact with cattle. J Clin Virol. 2016;81:31-3. PMID:27294672
- Faccini S, De Mattia A, Chiapponi C, Barbieri I, Boniotti MB, Rosignoli C, Franzini G, Moreno A, Foni E, Nigrelli AD. Development and evaluation of a new Real-Time RT-PCR assay for detection of proposed influenza D virus. J Virol Methods. 2017;243:31-4. PMID:28153610
- Hause BM, Huntimer L, Falkenberg S, Henningson J, Lechtenberg K, Halbur T. An inactivated influenza D virus vaccine partially protects cattle from respiratory disease caused by homologous challenge. Vet Microbiol. 2017;199:47-53. PMID:28110784
- Ferguson L, Olivier AK, Genova S, Epperson WB, Smith DR, Schneider L, Barton K, McCuan K, Webby RJ, Wan XF. Pathogenesis of Influenza D Virus in Cattle. J Virol. 2016;90:5636-42. PMID:27030270
- Sreenivasan C, Thomas M, Sheng Z, Hause BM, Collin EA, Knudsen DE, Pillatzki A, Nelson E, Wang D, Kaushik RS, et al. Replication and transmission of the novel bovine influenza D virus in a guinea pig model. J Virol. 2015;89:11990-2001. PMID:26378161
- Desselberger U, Racaniello VR, Zazra JJ, Palese P. The 3' and 5'-terminal sequences of influenza A, B and C virus RNA segments are highly conserved and show partial inverted complementarity. Gene. 1980;8:315-28. PMID:7358274
- Lee YS, Seong BL. Nucleotides in the panhandle structure of the influenza B virus virion RNA are involved in the specificity between influenza A and B viruses. J Gen Virol. 1998;79 (Pt 4):673-81. PMID:9568960
- Zheng H, Palese P, Garcia-Sastre A. Nonconserved nucleotides at the 3' and 5' ends of an influenza A virus RNA play an important role in viral RNA replication. Virology. 1996;217:242-51. PMID:8599209
- Gao Q, Palese P. Rewiring the RNAs of influenza virus to prevent reassortment. Proceedings of the National Academy of Sciences of the United States of America. 2009;106:15891-6. PMID:19805230
- Peng G, Hongo S, Kimura H, Muraki Y, Sugawara K, Kitame F, Numazaki Y, Suzuki H, Nakamura K. Frequent occurrence of genetic reassortment between influenza C virus strains in nature. J Gen Virol. 1996;77(Pt 7):1489-92. PMID:8757991
- Te Velthuis AJ, Fodor E. Influenza virus RNA polymerase: insights into the mechanisms of viral RNA synthesis. Nat Rev Microbiol. 2016;14:479-93. PMID:27396566
- Noda T, Kawaoka Y. Structure of influenza virus ribonucleoprotein complexes and their packaging into virions. Rev Med Virol. 2010;20:380-91. PMID:20853340
- Pekosz A, Lamb RA. Influenza C virus CM2 integral membrane glycoprotein is produced from a polypeptide precursor by cleavage of an internal signal sequence. Proc Natl Acad Sci U S A. 1998;95:13233-8. PMID:9789071
- Krug RM. Functions of the influenza A virus NS1 protein in antiviral defense. Curr Opin Virol. 2015;12:1-6. PMID:25638592
- Paterson D, Fodor E. Emerging roles for the influenza A virus nuclear export protein (NEP). PLoS Pathog. 2012;8:e1003019. PMID:23236273
- Yamashita M, Krystal M, Palese P. Comparison of the three large polymerase proteins of influenza A, B, and C viruses. Virology. 1989;171:458-66. PMID:2763462
- Gatherer D. Tempo and mode in the molecular evolution of influenza C. PLoS Currents. 2010;2:RRN1199. PMID:21127722
- Sheng Z, Ran Z, Wang D, Hoppe AD, Simonson R, Chakravarty S, Hause BM, Li F. Genomic and evolutionary characterization of a novel influenza-C-like virus from swine. Archives of Virology. 2014;159:249-55. PMID:23942954
- Herrler G, Klenk HD. Structure and function of the HEF glycoprotein of influenza C virus. Adv Virus Res. 1991;40:213-34. PMID:1957719
- Rosenthal PB, Zhang X, Formanowski F, Fitz W, Wong CH, Meier-Ewert H, Skehel JJ, Wiley DC. Structure of the haemagglutinin-esterase-fusion glycoprotein of influenza C virus. Nature. 1998;396:92-6. PMID:9817207
- Flewett TH, Apostolov K. A reticular structure in the wall of influenza C virus. J Gen Virol. 1967;1:297-304. PMID:6082336
- Herrler G, Nagele A, Meier-Ewert H, Bhown AS, Compans RW. Isolation and structural analysis of influenza C virion glycoproteins. Virology. 1981;113:439-51. PMID:7269251
- Song H, Qi J, Khedri Z, Diaz S, Yu H, Chen X, Varki A, Shi Y, Gao GF. An open receptor-binding cavity of Hemagglutinin-Esterase-Fusion glycoprotein from newly-identified influenza D virus: basis for its broad cell tropism. PLoS Pathogens. 2016;12:e1005411. PMID:26816272
- Kordyukova LV, Serebryakova MV, Baratova LA, Veit M. S acylation of thehemagglutinin of influenza viruses: mass spectrometry revealssite-specific attachment of stearic acid to a transmembrane cysteine. J Virol. 2008;82:9288-92. PMID:18596092
- Wang M, Ludwig K, Bottcher C, Veit M. The role of stearate attachment to the hemagglutinin-esterase-fusion glycoprotein HEF of influenza C virus. Cell Microbiol. 2015;18:692-704. PMID:26518983
- Herfst S, Schrauwen EJ, Linster M, Chutinimitkul S, de Wit E, Munster VJ, Sorrell EM, Bestebroer TM, Burke DF, Smith DJ, et al. Airborne transmission of influenza A/H5N1 virus between ferrets. Science. 2012;336:1534-41. PMID:22723413
- Imai M, Watanabe T, Hatta M, Das SC, Ozawa M, Shinya K, Zhong G, Hanson A, Katsura H, Watanabe S, et al. Experimental adaptation of an influenza H5 HA confers respiratory droplet transmission to a reassortant H5 HA/H1N1 virus in ferrets. Nature. 2012;486:420-8. PMID:22722205
- Shi Y, Wu Y, Zhang W, Qi J, Gao GF. Enabling the ‘host jump’: structural determinants of receptor-binding specificity in influenza A viruses. Nat Rev Microbiol. 2014;12:822-31. PMID:25383601
- Rogers GN, Herrler G, Paulson JC, Klenk HD. Influenza C virus uses 9-O-acetyl-N-acetylneuraminic acid as a high affinity receptor determinant for attachment to cells. J Biol Chem. 1986;261:5947-51. PMID:3700379
- Herrler G, Rott R, Klenk HD, Muller HP, Shukla AK, Schauer R. The receptor-destroying enzyme of influenza C virus is neuraminate-O-acetylesterase. EMBO J. 1985;4:1503-6. PMID:2411539
- Bottcher-Friebertshauser E, Klenk HD, Garten W. Activation of influenza viruses by proteases from host cells and bacteria in the human airway epithelium. Pathog Dis. 2013;69:87-100. PMID:23821437
- Tashiro M, Ciborowski P, Klenk HD, Pulverer G, Rott R. Role of Staphylococcus protease in the development of influenza pneumonia. Nature. 1987;325:536-7; PMID:3543690
- Takashita E, Muraki Y, Sugawara K, Asao H, Nishimura H, Suzuki K, Tsuji T, Hongo S, Ohara Y, Kawaoka Y, et al. Intrinsic temperature sensitivity of influenza C virus hemagglutinin-esterase-fusion protein. J Virol. 2012;86:13108-11. PMID:23015703
- O'Callaghan RJ, Loughlin M, Labat DD, Howe C. Properties of influenza C virus grown in cell culture. J Virol. 1977;24:875-82. PMID:73602
- Scholtissek C. Molecular evolution of influenza viruses. Virus Genes. 1995;11:209-15. PMID:8828147
- Tamura K, Peterson D, Peterson N, Stecher G, Nei M, Kumar S. MEGA5: molecular evolutionary genetics analysis using maximum likelihood, evolutionary distance, and maximum parsimony methods. Molecular Biology And Evolution. 2011;28:2731-9. PMID:21546353
- Drummond AJ, Rambaut A. BEAST: Bayesian evolutionary analysis by sampling trees. BMC Evolutionary Biology. 2007;7:214. PMID:17996036
- Drummond AJ, Ho SY, Phillips MJ, Rambaut A. Relaxed phylogenetics and dating with confidence. PLoS Biology. 2006;4:e88. PMID:16683862