ABSTRACT
The black yeast Exophiala dermatitidis is an opportunistic pathogen, causing phaeohyphomycosis in immunosuppressed patients, chromoblastomycosis and fatal infections of the central nervous system in otherwise healthy Asian patients. In addition, it is also regularly isolated from respiratory samples from cystic fibrosis patients, with rates varying between 1% and 19%.
Melanin, as part of the cell wall of black yeasts, is one major factor known contributing to the pathogenicity of E. dermatitidis and increased resistance against host defense and anti-infective therapeutics. Further virulence factors, e.g. the capability to adhere to surfaces and to form biofilm were reported. A better understanding of the pathogenicity of E. dermatitidis is essential for the development of novel preventive and therapeutic strategies. In this review, the current knowledge of E. dermatitidis prevalence, clinical importance, diagnosis, microbiological characteristics, virulence attributes, susceptibility, and resistances as well as therapeutically strategies are discussed.
Introduction
The incidence of fungal infections has increased in recent decades, posing new challenges to health care professionals [Citation1]. One group of fungi found as pathogens in clinical specimen are the black yeasts, also known as black yeast-like fungi. These are mainly characterized by their dimorphic character, being able to switch from the yeast-like to the hyphal state. In addition, the dark appearance of colonies, caused by the melanized and thick cell wall is another main characteristic of black yeasts. This melanization represents one virulence attribute. However, not all black yeasts are known to be human pathogens, e.g. Aureobasidium spp. being plant-associated fungi [Citation2]. In a clinical context, black yeasts are most frequently associated with the genus of Exophiala. The most prominent representative and at the same time most isolated melanized fungus is Exophiala (Wangiella) dermatitidis, found as a pathogen in the human host. Next to E. dermatitidis, also other species of the genus known to infect humans, e.g. E. oligosperma, E. jeanselmei and E. xenobiotica [Citation3]. The number of studies dealing with E. dermatitidis as a human pathogen has increased in recent years, highlighting the importance of E. dermatitidis in medical mycology. Among others, the black yeast-like fungus E. dermatitidis is a frequent colonizer of the respiratory tract of cystic fibrosis (CF) patients with varying rates of up to 19% [Citation4–Citation7]. In addition to exacerbation of CF, E. dermatitidis is also known to be a cause of central nervous system infections in otherwise healthy, immunocompetent Asian patients [Citation8–Citation11]. Furthermore, cases of E. dermatitidis infections in immunosuppressive patients have been reported, most often in the form of phaeohyphomycosis [Citation12,Citation13]. The fungus is globally prevalent and case reports on E. dermatitidis infections display the different groups of patients at risk. Various studies focused on virulence factors, e.g. pigmentation, polymorphism, hydrophobicity, adhesion and biofilm formation, production of secondary metabolites and so on, as well as its susceptibility against antifungal agents in both, clinical and environmental isolates. In this review, the current knowledge of E. dermatitidis prevalence, clinical importance, diagnosis, microbiological characteristics, virulence attributes, susceptibility, and resistances as well as therapeutically strategies are discussed.
Figure 1. Prevalence of E. dermatitidis caused infections in immunocompetent (red = 9 cases) and immunodeficient (blue, 9 cases) patients as reported in case reports since 2007.
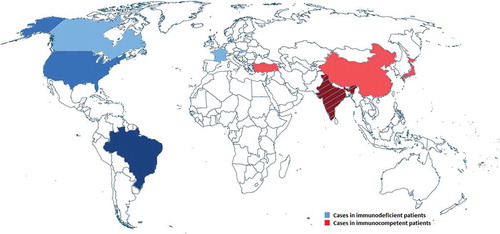
Figure 2. Number of cases of E. dermatitidis infections reported in the literature from 1960 to May 2018. Data until 1999 were retrieved from Taj-Aldeen et al. [Citation12]. Latest reports were recorded by carefully scrutinized for case reports.
![Figure 2. Number of cases of E. dermatitidis infections reported in the literature from 1960 to May 2018. Data until 1999 were retrieved from Taj-Aldeen et al. [Citation12]. Latest reports were recorded by carefully scrutinized for case reports.](/cms/asset/f5fc1e19-7d12-4c7e-96ed-6de12ec66cce/kvir_a_1596504_f0002_b.gif)
Historical and clinical importance and today’s prevalence
E. dermatitidis was first isolated in 1937 by Kano from a lesion on the cheek of a Japanese woman and was classified as Hormiscium dermatitidis [Citation14]. Due to its morphology, the taxonomic classification of E. dermatitidis has varied frequently. In view of the various sporulation patterns and the morphology of conidia and conidiophores, it has been classified in the genera Fonsecaea, Hormodendrum, Phialophora, Rhinogladiella, Exophiala, and Wangiella [Citation14]. Today Exophiala as well as Wangiella are commonly used.
The taxonomy of black yeasts was confusing in the past. After the application of molecular criteria, a huge number of species belonging to the black yeasts was encountered in both, environmental and clinical settings [Citation15]. The order Chaetothyriales with the family Herpotrichiellaceae comprises clinically relevant black yeasts and relatives [Citation15]. One genus within this family is Exophiala. The genus Exophiala, characterized by annellidic conidiogenesis, currently comprises more than 40 species [Citation16,Citation17]. Exophiala spp. belong to the saprophytic fungi which can be isolated from hydrocarbon rich or warm, humid and oligotrophic environments [Citation18]. Some species are solely producing budding cells, some also forming phialidic collarettes, sympodial conidiophores or conidial chains in various ratios [Citation16,Citation17,Citation19]. Among the genus, Exophiala are the most clinically relevant black yeasts with reported mortality rates of 25–80% in systemic and invasive cases, even though fatal systemic cases are relatively rare [Citation20,Citation21]. In contrast, several Exophiala spp. are known to cause cutaneous and superficial infections in human and animals [Citation3,Citation19]. In a study regarding the spectrum of clinically relevant Exophiala species in the US, 188 strains, previously identified as Exophiala ssp., were investigated. In the following, all species identified with rates above 3% are listed: E. xenobiotica (19.7 %), E. oligosperma (18.6%), E. lecanii-corni (6.9 %), E. phaeomuriformis (6.4%), E. jeanselmei (3.7%), E. bergeri (3.7%), and E. mesophila (3.2%) [Citation3]. Systemic infections were mainly caused by E. dermatitidis, which is in addition also the most frequently isolated Exophiala species (29.3%) [Citation3].
E. dermatitidis is a ubiquitous fungus, although rarely isolated from the environment [Citation18]. It is assumed that the origin of this fungus lies in the tropical rainforests, among others in the niche of wild fruits and berries. A possible route of infection by dispersal via feces from frugivorous birds and bats is reported by Sudhadham et al. This group investigated the origin of over 3,000 samples within a period of 3 y. In contrast, the prevalence in soil and plants was almost zero [Citation18]. Little is known about the natural habitat and the transmission routes of E. dermatitidis. A recent review excellently summarizes the ecology of this fungus and reported that it can be mostly found in man-made indoor habitats, connected to water sources [Citation22]. In the man-made environment, the fungus is globally distributed, found in dishwashers, steam baths or sauna facilities, all of those environments are characterized by high temperatures, humidity and pH changes [Citation9,Citation10,Citation22–Citation26]. In subtropical regions, E. dermatitidis was isolated from railway sleepers at a rate of 13% [Citation27]. Due to the high isolation rate of E. dermatitidis in wastewater samples from dishwashers, it is posited that the dishwasher is a possible transmission route between environment and the human host, especially through aerosol inhalation [Citation22]. However, household-acquired infections seem to be rare and either mild [Citation28] or restricted to patient populations with disorders such as CF [Citation22] or immunosuppression [Citation29].
No relevant hints exist that E. dermatitidis would be transmitted via a zoonotic route. Two cases reported invasive E. dermatitidis (intraabdominal and subcutaneous) in dogs [Citation30,Citation31] and in an older study E. dermatitidis was found in the liver of fruit-eating bats, Eidolon helvum [Citation32].
E. dermatitidis in cystic fibrosis (CF)
E. dermatitidis is, among other species, a common colonizer of the respiratory tract of patients with CF. In 1990, Haase et al. [Citation7] reported for the first time E. dermatitidis isolation from the sputum of a 5-year-old CF patient, even after treatment with amphotericin B and 5-fluorcytosine. In 1992, Kusenbach et al. [Citation33] described an E. dermatitidis-associated pneumonia in a 7-year-old patient with CF. The isolation rates of E. dermatitidis varied in numerous studies between 1% and 19% in CF [Citation4–Citation7]. The prevalence of E. dermatitidis in Germany, Sweden, and Belgium is the highest in the world with rates ranging from 4.8% to 17% in contrast to lower rates in other countries like France or the USA [Citation5,Citation34]. The varying rates of E. dermatitidis isolation may be correlated to genetic factors, as described for P. aeruginosa, or to lifestyle variations [Citation34]. Another important factor is the existence of variances in isolation success due to lack of standardization in the mycological analysis of respiratory material [Citation34], as well as problems in the identification of E. dermatitidis, e.g. due to failure in distinguishing between different Exophiala spp. Reliable diagnostic methods for E. dermatitidis identification are mentioned in the section “Diagnosis of infections.”
Lebecque et al. described a prevalence of E. dermatitidis to colonize the respiratory tract of pancreatic insufficient CF patients [Citation6]. Additionally, predisposing factors for E. dermatitidis infections are diabetes mellitus, steroid medication, concurrent bacterial and fungal infections and nutritional deficiencies [Citation14]. In addition, most E. dermatitidis isolates from CF patients occurred in adolescent or adult patients [Citation6]. Grenouillet et al., in 2018 [Citation35], described two cases of mild forms of CF identified in elderly patients following a diagnosis of a respiratory infection or colonization by E. dermatitidis. It was suggested that in patients with chronic respiratory disease and recurrent pulmonary infections, the detection of E. dermatitidis could be a potential marker of atypical CF and should lead clinicians to conduct investigations for CF diagnosis [Citation35].
Traditionally, E. dermatitidis is considered to have low virulence in CF patients [Citation14]. The differentiation between colonization and infection with E. dermatitidis in a CF patient is analog to relevant CF bacteria, e.g. Staphylococcus aureus or Pseudomonas aeruginosa very difficult. However, most CF patients show no clinical symptoms. In patients with pulmonary exacerbation and/or decline in lung function, the clinical significance of E. dermatitidis can be evaluated with the following diagnostic parameters: despite the culture positivity it is possible to analyze specific E. dermatitidis IgG antibodies. A recent work from Sweden reported that 4 of 17 CF patients had a symptomatic E. dermatitidis infection and showed clinical response to antifungal treatment [Citation5]. It was shown that increased IgG serum levels against E. dermatitidis were positively associated with higher white blood cell counts, increased erythrocyte sedimentation rate, pancreatic insufficiency, antibiotic treatment and were negatively associated with respiratory function (FEV1% predicted) [Citation5]. Specific IgG detection is not commercially available so far. The fungal biomarker 1,3-ß-D-glucan can be easily determined in serum. Higher glucan levels were associated with positive serum IgG levels against E. dermatitidis [Citation5]. If no other reasons could be identified for clinical deterioration in CF patients with repeated E. dermatitidis isolation in the airways, the initiation of antifungal treatment might be the last way to evaluate if the patient’s status improves. No larger studies exist that have investigated whether E. dermatitidis contributes to the disease outcome of CF patients. Therefore, it remains elusive whether E. dermatitidis is actively involved in CF lung disease pathologies or whether it rather reflects a dysregulated airway colonization and acts as a microbial bystander [Citation5].
E. dermatitidis causing phaeohyphomycosis in immunosuppressed patients and fatal infections in immunocompetent individuals
In addition to CF patients, also immunosuppressed or elderly patients as well as immunocompetent patients with Asian background are known to be affected by E. dermatitidis. The prevalence of E. dermatitidis infections in humans is global with significantly increased cases of infected immunocompetent patients in Asia. Also, infected immunodeficient patients are globally distributed. Risk of infection in those patients are immune defects like CARD9 mutations, immunodeficiency caused by drug treatment, cancer or transplantation [Citation36–Citation38]. Case reports of E. dermatitidis infections, occurred since 2007, are visualized in a map of the world with infections of immunosufficient patients indicated by blue color and immunocompetent infected patients indicated by red color (). CF case reports were not included.
Immunosuppressed and elderly patients suffer from infections with E. dermatitidis most commonly in the form of phaeohyphomycosis, keratitis or chromoblastomycosis; 54 cases of phaeohyphomycosis have been described between 1934 and 2006 () [Citation12,Citation13]. Literature research on recent published case reports involving E. dermatitidis-caused infections revealed 20 new cases since 2007 in both, immunocompetent and -suppressed patients () [Citation21,Citation36–Citation51]. E. dermatitidis may also be a severe agent of pneumonia [Citation12]. Ex-vivo skin-model evidence of E. dermatitidis penetration suggests that E. dermatitidis may also be responsible for superficial skin infections [Citation52].
Table 1. Summary of E. dermatitidis caused infections case reports since 2007.
Fatal brain infections caused by the neurotropic E. dermatitidis occurred in otherwise healthy individuals in the Asian population [Citation8–Citation11]. Its neurotropic character has been hypothesized to be caused in parts by the capability to assimilate aromatic (alkylbenzene) hydrocarbons [Citation53] and by the capability of cell–cell and cell-surface adherence [Citation19]. Several studies revealed a predilection of E. dermatitidis for the human central nervous system. These infections are chronic but deaths attributed to them are only reported in Asia [Citation10,Citation54]. The restriction to Asian populations of cerebral E. dermatitidis infection cases is currently not sufficiently explained. Hypotheses are, among others the possibility of unequal exposure to the fungus [Citation10] and involvement of different (immunological) host factors [Citation54,Citation55].
Outside Asia, nosocomial-acquired infections in pseudoepidemic situations have been reported, for example from the US. These infections mostly appear after administration of contaminated medication to elderly people [Citation56,Citation57]. Recently, 15 cases of E. dermatitidis bloodstream infections occurred among patients which were treated in a hemato-oncological hospital [Citation58]. A contaminated intravenous flush solution was identified as the source of infection. These infections posed a challenge to clinical management of bloodstream infections with E. dermatitidis, as they are relatively rare and no specific guidelines for treatment of these infections exist [Citation58].
Diagnosis of E. dermatitidis infections
The diagnosis of infections with black yeasts is a challenge in routine diagnostics. Invasive fungal infections diagnosed delayed and appropriate delayed treatment may worsen the patients' outcome [Citation59]. Thus, appropriate methods for species-specific identification are necessary. However, no species-specific standardized diagnostic tools are available.
Identification as E. dermatitidis by cultural methods, microscopy, and ribosomal DNA internal transcribed spacer (ITS) sequencing are commonly used techniques [Citation21]. Morphological identification of E. dermatitidis is restricted by its slow-growing behavior [Citation29] and therefore the risk of bacterial overgrowth, e.g. by P. aeruginosa and Burkholderia cepacia complex organisms, especially in CF patients [Citation60]. Sabouraud agar is the most common used agar for isolation and cultivation of fungal species. However, E. dermatitidis isolation is recommended to be either performed on Burkholderia cepacia- selective agar, potato-dextrose agar with rose-bengal and chloramphenicol, erythritol-chloramphenicol agar (ECA) or Sabouraud gentamicin-chloramphenicol agar (SGCA), all leading to increased isolation rates compared to Sabouraud media by inhibiting bacterial growth [Citation6,Citation29,Citation60–Citation62]. The median time necessary for isolation was ascertained to be at least 6 d using ECA and SGCA [Citation6]. As reported by Horré et al., prior to the use of ECA, E. dermatitidis was never isolated in routine work [Citation61]. However, even when bacterial overgrowth is prevented, microscopic and macroscopic morphological identification on species level of black yeast may not be the optimal method. Often, species-specific morphology is absent/difficult to detect or variable expression of characteristics make it impossible to distinguish between species [Citation63,Citation64]. In addition, patients with invasive fungal infections may nevertheless be culture negative. Thus, alternative diagnostic tools should be considered.
Matrix-assisted laser desorption-ionization time of flight mass spectrometry (MALDI-TOF) has been proven to be an optimal method for rapid identification of pathogenic yeast from cultures [Citation65,Citation66]. Also, the identification of yeast belonging to the genus Exophiala by MALDI-TOF MS showed consistency with ITS sequencing analysis [Citation67]. However, for distinguishing between species of the black yeast, robust reference spectra for every species are necessary [Citation68,Citation69].
Molecular methods are more and more replacing morphology tools for identifying fungi. A species-specific PCR introduced by Nagano et al. in 2008 was the first successful approach for a diagnostic E. dermatitidis PCR, based on rDNA operons and ITS regions[Citation70]. Also, others suggest discriminative ITS data as the method of choice for species identification [Citation63]. The ITS region is a barcode marker for the identification of fungi and ITS analysis has been shown to be a useful tool for distinguishing black yeasts [Citation71,Citation72]. This technique is even used to distinguish between E. dermatitidis subtypes [Citation72]. However, initial studies did not demonstrate an advantage of molecular identification techniques over conventional cultivation techniques using an appropriate medium [Citation73]. A multi-diagnostic approach including two or more methods should be considered for a reliable black yeast diagnostic.
Diversity
E. dermatitidis diversity was analyzed using pheno- and genotypic methods. One phenotypic tool for discrimination and diversity analysis is fatty acid methyl ester (FAME) profile analysis [Citation74]. Genotypic characterization of E. dermatitidis strains was among others performed using ribotyping of the small subunit of rDNA and by random amplification of polymorphic DNA (RAPD) [Citation55,Citation74], as well as by the commonly used analysis of the ITS1. Ribotyping resulted in differentiation of 21 clinical isolates, originating from CF sputum samples and systemic neurotropic infections in Asian patients, into two genotypic groups whereas RAPD analysis resulted in seven genotypes. The interpretation of these RAPD analyses is limited as the number of primers used may skew results and interlaboratory reproducibility of RAPD patterns is low [Citation74].
Three main genotypes of E. dermatitidis have been detected in sequence analysis of the ITS1. These are denoted as groups A, B, and C [Citation75]. Group A mostly corresponds to strains isolated from clinical specimens, showing a high frequency in virulence potential. Genotype B is mostly found in isolates from the natural environment, whereas isolates from the man-made environment occur in both genotypic groups, A and B, with slight predominance in the cluster of genotype A [Citation75,Citation76]. This genotypic cluster distribution is reported worldwide, indicating long-distance dispersal, especially for strains isolated from systemic infections [Citation75]. Genotype A is most frequently identified [Citation24,Citation77]. In contrast, genotype C is rarely found.
Microbiology & characteristics
The black yeast-like fungus E. dermatitidis is dark-pigmented. Its morphology is from dimorphic character, filament and yeast forms are clearly visible in fungus microscopy (). E. dermatitidis is currently accepted to be asexual as no sexual form has been discovered [Citation78]. However, a phylogenetic analysis of various Exophiala species revealed a clade of the genus Exophiala with Caproni mansonii, a telemorph species, thus indicating E. dermatitidis being the anamorph of C. mansonii [Citation17,Citation79]. It is polyextremophilic, metabolically active over a wide temperature (4–40°C) and pH range (pH 2.5–12.5) [Citation9,Citation26,Citation80–Citation82]. E. dermatitidis is a slow-growing organism [Citation29]. It is also known to be strongly hydrophilic [Citation83]. In addition, black yeasts are characterized by the expression of specific gene families, such as alcohol and aldehyde dehydrogenase, membrane transporter proteins and cytochrome P450 [Citation84]. Colonies of E. dermatitidis grow restricted, smooth, waxy and with a dark appearance, often with dark pigments exuded into the growth medium [Citation17].
Figure 3. Light microscopy E. dermatitidis (CBS 149.90) colonies on malt extract agar after 48 h of incubation at 35°C. Scale bar equals 200 µm (Credits: M. Olsowski).
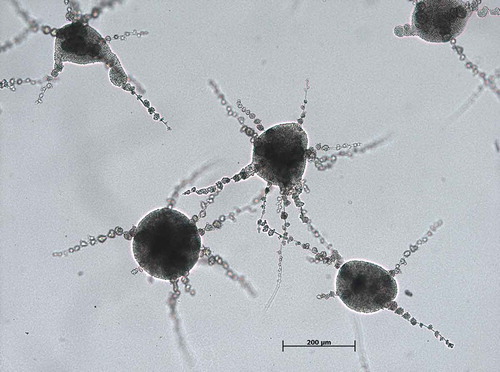
E. dermatitidis was identified to be a producer of exopolysaccharide (EPS), producing irregular EPS with a fibrillary substructure [Citation2]. These EPS are associated with the fungus thermotolerance and thus with its virulence [Citation2]. Possible roles of EPS in, e.g. biofilm formation, drug resistance, and immune evasion have been suggested as described for other fungi [Citation85].
Among other factors, polymorphism is known to contribute to the virulence of certain fungi, as reported for thermally dimorphic species [Citation86]. Also, E. dermatitidis ability to switch from the hyphal to yeast-like growth is associated with pathogenicity [Citation17]. The transcription factor APSES, well known to regulate fungal cellular development and differentiation, is encoded by WdSTUAp. This transcription factor was shown to be a regulator of morphology in the dimorphic E. dermatitidis in both a negative and positive fashion [Citation87].
Budding yeast cells are the predominant morphotype [Citation87]. Conidia with a size of 2.5–4 × 2–3 µm are flask shaped and appear in small groups, free or intercalary [Citation17]. Along with budding yeast cells, also pseudohyphal, moniliform hyphae, true hyphae, and sclerotic forms also appear. Yeast-to-hyphal transition has been monitored using electron microscopy [Citation88]. Budding yeasts in their exponential growth phase were described to have thin cell walls. Aged cells, in contrast, showed altered cell structures with thick cell walls and a considerable amount of stored materials. Only the thick-walled cells were found to be capable of converting to hyphal structures, as the acquisition of “spore-like” characteristics (thick-walled, endogenous substrate reserves) is necessary for transition [Citation88].
The polymorphism of E. dermatitidis was found to be calcium ion-dependent. A low calcium ion concentration results in non-polarized growth leading to multicellular form development in contrast to polarized growth and yeast budding or pseudo/true hyphal growth [Citation89]. Similarly, temperature influences the form of the fungus, it being more likely to propagate in the filamentous form at room temperature, but switching to yeast-like growth at 37°C [Citation90]. Sclerotic morphotypes can further be induced by nutrient-rich media at pH 2.5 [Citation87].
Virulence
E. dermatitidis showed virulence in Galleria mellonella [Citation20,Citation91] as well as in the nematode Caenorhabditis elegans [Citation91]. The virulence of E. dermatitidis is furthermore dependent on the source of isolation as detected in a G. mellonella infection model (). Dimorphism was identified as a key factor for the development of invasive hyphal growth and increased virulence, mostly showed by E. dermatitidis isolated from phaeohyphomycosis in immunocompetent Asian patients [Citation91].
Figure 4. Survival curve of Galleria mellonella infected with E. dermatitidis Environmental isolates (E), CF patients isolates (CF) and Isolates from Asian, immunocompetent patients (PA). Depicted values are means of nine tested strains. Each strain was tested in triplicate. Taken from: Olsowski et al., 2018 [Citation91].
![Figure 4. Survival curve of Galleria mellonella infected with E. dermatitidis Environmental isolates (E), CF patients isolates (CF) and Isolates from Asian, immunocompetent patients (PA). Depicted values are means of nine tested strains. Each strain was tested in triplicate. Taken from: Olsowski et al., 2018 [Citation91].](/cms/asset/6f12a4a9-9016-429a-b76e-78ad52b45f40/kvir_a_1596504_f0004_b.gif)
Additional to the above-described dimorphism of E. dermatitidis other virulence factors exist. The most prominent characteristic contributing to virulence is pigmentation [Citation92–Citation94]. Alongside melanin, carotenoids are also found in cell walls of black yeasts [Citation95].
Capsular material is also a key determinant of virulence as it contributes to adhesion, phagocytose impairment and hindrance to complement-mediated killing [Citation84]. The extracellular polysaccharides (acid mucopolysaccharides) secreted by E. dermatitidis yeast cells have been shown to mask the cells for human phagocytes during the invasion of tissue [Citation2]. Nishimura and Miyaji suggested an additional role of extracellular polysaccharides in the interaction between yeast cells and mononuclear neutrophils in mice [Citation25].
In addition, adherence and biofilm formation have been shown to contribute to virulence. Further virulence attributes of E. dermatitidis are hydrophobicity [Citation96], urease, catalase, proteinase and DNase production [Citation97], chitin synthase [Citation98–Citation100], secondary metabolite production [Citation96,Citation101] and the assimilation of aromatic (alkylbenzene) hydrocarbons [Citation53,Citation102,Citation103]. Latter contributes to neurotropism characteristics of members of Herpotrichiellaceae [Citation53]. The known virulence factors occurring in E. dermatitidis are summarized in .
Table 2. Virulence factors of Exophiala dermatitidis.
Pigmentation
One of the best-studied virulence factors of black yeasts and especially E. dermatitidis is the pigmentation, mostly associated with the pigment melanin [Citation104].
Melanin is ubiquitous and found in all kingdoms of life and therefore also in many microbes (pro- and eukaryotes) as well as animals. The production of melanin in fungi has been documented since the early 1960s [Citation94,Citation105,Citation106]. The melanin group of pigments comprises numerous and diverse substances, sharing a number of properties. They are all negatively charged, hydrophobic and high molecular weight compounds [Citation106]. In the past, they were defined by their dark color, poor solubility and resistance to hot acids, hot and concentrated alkaline solutions and oxidizing acids [Citation107]. Pigmentation of the cell wall of yeast is mostly associated with stability and protection due to the pigments structure, making it an extremely stable molecule. Pigments are able to protect the organism against biotic and abiotic stress factors, e.g. temperatures, osmotic pressures, UV light, radiation, and host immune-cells [Citation108]. Additionally, in E. dermatitidis, the multilayered, melanin carrying cell wall plays a role in cell protection, prevents desiccation, functions as an antioxidant and is able to harvest metabolic energy [Citation84,Citation106,Citation109–Citation111]. The latter is explained by the fact that exposure of ionizing radiation to melanized fungi, e.g. E. dermatitidis showed an increase in growth and biomass by altered electronic properties of the cell wall stored melanin [Citation111]. Dadachova et al. assumed that the capability of melanin to capture electromagnetic radiation and its oxidation-reduction properties contribute to the gain of metabolic energy in melanized fungi [Citation112,Citation113].
The general clinical significance of melanized fungi is considered as relatively low with a detection rate of approximately 10% in fungal isolates in the diagnostic microbiology lab. However, they are significant agents of phaeohyphomycosis developing after traumatic infections [Citation19]. Despite their rarity in clinics, the melanized fungi became significant due to their occurrence and pathogenicity in immunocompromised patients. The rarity of invasive fungal infections in immunocompetent patients might be due to various cells of the host defense. Important cells for the defense against fungal pathogens are, e.g. dendritic cells, neutrophils, macrophages, NK cells, CD4 T cells (Th1, Th2, Th9, Th17, and Treg), and CD8 cells [Citation19,Citation114]. All those cells produce cytokines, reactive oxygen intermediates or antimicrobial peptides, contributing to host protection against fungal pathogens [Citation114]. In contrast, immunosuppressive patients have in one or the other way a lack of those immune cells, leading to a higher risk of infections with invasive fungal pathogens.
Melanin synthesis in fungi is in most cases related to phenol oxidase activity. The phenoloxidase system has been discovered in numerous fungi, e.g. Cryptococcus neoformans and is composed of soluble enzymes with broad substrate specificity [Citation115]. In addition, the substrate-specific pentaketide pathway for melanin synthesis is significant for certain developmental stages [Citation115]. Two prominent melanins, 1,8-dihydroxynapthalene (DHN) melanin and l-3,4-dihydroxyphenylalanine (DOPA) melanin, named after intermediates of the synthesis pathway used, are known to contribute to the pathogenicity of certain fungi [Citation94]. Some fungi are even capable to synthesize melanin in various pathways, e.g. A. fumigatus which is, additionally to DHN melanin production, able to synthesize the so-called pyomelanin in presence of L-tyrosine [Citation116]. The pentaketide pathway for melanin synthesis was detected in E. dermatitidis and melanin produced by E. dermatitidis has been identified as DHN melanin [Citation115]. The pentaketide pathway in E. dermatitidis can be successfully blocked by the addition of the pathway inhibitor tricyclazole [Citation117]. The responsible genes for all three pathways, DHN, DOPA, and L-tyrosine, have been identified in the E. dermatitidis genome [Citation95,Citation118].
The production of DHN melanin has been associated with virulence due to the observations of melanin-deficient strains of E. dermatitidis and C. neoformans exhibiting a decreased virulence [Citation92,Citation93,Citation119]. The considerable effect of melanin on the survival and resistance of the cell is mainly due to its protective role against both environmental stress as described above and the oxidants of host effector cells [Citation104]. Melanin allows the fungus to escape phagocytosis and protects against free radicals by acting as a potent free radical scavenger, protecting the cell against oxidants generated by the immune effector cells of the host organism [Citation19]. It also provides protection from damage by UV-light, temperature and salt extremes, antimicrobial drugs and peptides. Although melanin plays a role in evasion of oxidative stress, this function is mainly carried out by detoxifying enzymes [Citation90,Citation120]. Melanin might also play an immunosuppressive role during fungal infection as it was shown that melanin suppresses the production of proinflammatory cytokines [Citation121,Citation122].
Feng et al. analyzed the virulence of E. dermatitidis wild-type strains against melanin-deficient mutants (wdpks1Δ) in mice and documented a dramatic decrease in mortality when the mice were infected with the melanin-deficient strains carrying wdpks1Δ compared to those infected with wild-type strains [Citation93]. Infections with melanin-deficient strains resulted in a survival rate of 90–100%. In contrast, infections with the wild-type E. dermatitidis strain and the wdpks1Δ complemented strains resulted in a 20% survival rate at day 13 of the experiment [Citation93]. In another study examining the virulence of wild-type E. dermatitidis compared to melanin-deficient mutants in mice, Dixon et al. detected a reduced mortality rate in the Mel3-infected group (0% at 21 d after infection) compared to those infected with wild-type strains (100% at day 6 after infection) [Citation92]. However, the brains of the mice were severely affected by infections with both strains. Whereas the wild-type strain showed invasive hyphal growth, associated with acute and fatal infections, the mutant strain did not show these forms of growth [Citation92]. In contrast, a lack of melanin production does not result in higher susceptibility to anti-infective agents [Citation123].
Carotenoids are, alongside melanin, an important group of pigments synthesized by black-yeasts [Citation95]. In contrast to melanin, carotenoids do not function by the neutralization of harmful oxidants. Instead, they act to shield sensitive molecules or organelles [Citation19,Citation104]. Environmental stress, e.g. osmotic and oxidative stress, leads to increased production of the pigment, thus contributing to cell membrane stability of the carotenogenic yeast [Citation124,Citation125].
In addition to melanin and carotenoids, chitin synthesis also contributes to the virulence of E. dermatitidis [Citation100]. Chitin is part of the fungal cell wall with higher amounts in filamentous than in yeast-like forms [Citation19,Citation126]. Wang et al. deduced that WdCHS2 encodes a class I chitin synthase. It is not essential; however, it is responsible for most of the chitin synthase zymogenic activity as detected in vitro. Mutants with double or triple mutations of the WdCHS2 were shown to be less virulent in in vivo experiments compared to the wild-type. In contrast, strains with one mutation in the WdCHS2 are as virulent as wild-type strains [Citation100]. Chitin synthase class V, also present in E. dermatitidis and encoded by WdChs5p, is a chitin synthase which is known to be responsible for growth at extreme temperatures [Citation98].
Adhesion and biofilm formation
Biofilm-associated infections with fungi are often refractory to targeted treatment due to increased resistance to antifungal drugs, resulting in recurrent and chronic infections [Citation127]. The embedded mode of life in the self-produced extracellular matrix within the biofilm provides the cells further protection against molecules of the host organism and against anti-infective agents [Citation127]. Thus, biofilm formation contributes to the pathogenic potential of fungi.
In response to certain environmental surroundings, the majority of Exophiala spp. are known to exhibit strong morphological plasticity, e.g. the switch from yeast to the filamentous, hydrophobic state [Citation19]. One other example is the cell–cell adherence, forming balls of conidia. These balls of conidia are essential for the initialization of adhesion to target cells, thus may further explain the neurotropic character of E. dermatitidis in parts [Citation19].
Biofilm formation of E. dermatitidis in several environmental settings has been reported, e.g. at water outlet fittings in indoor environments, such as water taps and shower heads [Citation128] or on rubber seals in dishwashers in multispecies consortia with bacteria. In the latter, Zupančič et al. reported also on cross-kingdom synergy of bacteria and E. dermatitidis within biofilms [Citation129].
Next to biofilms formed in the man-made environment, the biofilm formation capabilities of clinical E. dermatitidis isolates have been investigated in two recent studies () [Citation130,Citation131]. Sav et al. identified the biofilm formation capabilities in 15% of environmental (total n = 137) and 29% of clinical isolates (total n = 7) [Citation97]. In a study including 58 E. dermatitidis isolates from both, environmental and clinical sources, the biofilm formation capabilities of the fungus were demonstrated in vitro using a crystal violet stain based assay [Citation130]. Biofilm formation was detected to be significantly higher after a formation period of 48 h compared to 24 h. This might be due to the slow-growing character of black yeasts. Furthermore, a difference in biomass involved in biofilm was detected for the invasive isolates from Asian patients [Citation130].
Figure 5. E. dermatitidis (CBS 116372) biofilm after 48 h of incubation at 35°C, fixation in methanol and staining by acridine orange in a confocal laser scan microscopy. Taken from: Kirchhoff et al., 2017 [Citation130].
![Figure 5. E. dermatitidis (CBS 116372) biofilm after 48 h of incubation at 35°C, fixation in methanol and staining by acridine orange in a confocal laser scan microscopy. Taken from: Kirchhoff et al., 2017 [Citation130].](/cms/asset/d9d1b8e9-a2fd-402a-920b-a2664f40eac3/kvir_a_1596504_f0005_oc.jpg)
Two studies showed that biofilm of E. dermatitidis displays a greater degree of resistance compared to planktonic E. dermatitidis cells as detected by MIC experiments [Citation130,Citation132].
Susceptibility and resistance
Several studies investigated resistance and susceptibility of both environmental and clinical isolates to various anti-infective agents, using planktonic as well as sessile cells.
Currently there are no available standardized broth microdilution methodologies or validated MIC breakpoints for in vitro resistance testing for E. dermatitidis. However, numerous studies dealing with susceptibility patterns of the black yeast-like fungus exist [Citation21,Citation29,Citation41,Citation45,Citation123,Citation130,Citation132–Citation140].
Voriconazole, itraconazole, and posaconazole were shown to be active against E. dermatitidis as reported by Gao et al. [Citation132] (). In a study from Nweze and Ezute from 2010, 16 E. dermatitidis isolates from stool samples in Nigeria were analyzed (CLSI) [Citation133]. Almost all tested strains were susceptible to amphotericin B (MIC = 0.25–2 µg/mL), 5-fluorcytosine (MIC = 0.25–1 µg/mL), itraconazole (MIC = 0.25–8 µg/mL), fluconazole (MIC = 8–64 µg/mL) and voriconazole (MIC = 0.25–1 µg/mL) [Citation133]. Duarte et al. analyzed a set of 43 environmental E. dermatitidis isolates for their susceptibility to itraconazole (MIC50 = 0.06 µg/mL), voriconazole (MIC50 = 0.06 µg(mL), flucytosine (MIC50 = 1 µg(mL), terbinafine (MIC50 = 0.015 µg/mL) and amphotericin B (MIC50 = 1 µg/mL) (CLSI). The MIC ranges were similar to those detected in other studies analyzing clinical isolates () [Citation136]. This is comparable to the findings of Deng et al., stating high in vitro activity of both terbinafine (MIC50 = 0.031–0.5 µg/mL) and amphotericin B (MIC50 = 0.125–4 µg/mL) [Citation135]. Badali et al. tested eight antifungal agents for their activity against both clinical and environmental isolates of E. dermatitidis (CLSI). Posaconazole had the highest activity against the fungus, with a MIC50 of 0.063 µg/mL (CLSI) [Citation134]. In contrast, the echinocandins caspofungin (MIC50 = 4 µg/mL) and anidulafungin (MIC50 = 2 µg/mL) demonstrated weak activity against E. dermatitidis [Citation134]. A MIC of 8 µg/mL micafungin against three different clinical isolates of E. dermatitidis was detected, indicating also a weak activity of micafungin against the fungus (EUCAST) [Citation130]. Supporting this finding, a case report in 2018 described an E. dermatitidis breakthrough infection during prophylactic therapy with micafungin [Citation141]. Conversely, micafungin, when applied in lower dosages, showed antibiofilm activity. This activity was observed both when micafungin was added prophylactically to cultures, as well as when applied to mature biofilm [Citation130]. In general, caspofungin showed a broad range of MICs against E. dermatitidis, varying greatly within and between studies, as summarized in [Citation29,Citation45,Citation134].
Table 3. MIC ranges of several drugs (No. of isolates) against E. dermatitidis isolates from various origins. AMB = amphotericin B, ANI = anidulafungin, CAS = caspofungin, COL = colistin, FLC = fluconazole, ISC = isavuconazole, ITC = itraconazole, MICA = micafungin, POS = posaconazole, TBF = terbinafine, VRC = voriconazole, 5-FC = fluorcytosine.
FK506 (also known as tacrolimus) did not show an inhibitory effect (16 µg/mL) against planktonic E. dermatitidis and had MICs above 64 µg/mL against E. dermatitidis biofilm (CLSI) [Citation134]. However, when analyzed in combination with itraconazole, posaconazole and voriconazole, favorable synergistic effects were demonstrated for the vast majority of a total of 16 tested strains when grown in planktonic cultures [Citation132]. Against E. dermatitidis biofilm cells, the combination of azoles with FK506 also decreased the azole concentration necessary for inhibition [Citation132].
Overall, no significant differences in antifungal activity were observed, regardless of source (environmental or clinical) [Citation135] and type of isolate (invasive or mucocutaneous) [Citation134]. The most promising anti-infective effects against E. dermatitidis were reported from the triazoles voriconazole and posaconazole [Citation142]. Schwarz et al. also highlighted the possibility of treatment with posaconazole for E. dermatitidis infections in CF [Citation143]. Patients suffering from CF have been shown to benefit from a combined therapy of posaconazole with amphotericin B. Recurrent E. dermatitidis infections in CF are recommended to be treated with posaconazole [Citation143]. In vitro, amphotericin B showed additional synergistic activity in combination with terbinafine, and has been recommended as a possible treatment strategy in chromoblastomycosis caused by E. dermatitidis [Citation135].
Novel antifungals agents against E. dermatitidis have also been tested. Isavuconazole showed in vitro activity against two E. dermatitidis reference strains [Citation144]. In contrast, the novel dihydroorotate dehydrogenase inhibitor olorofim (F901318) had no effect (MIC > 4µg/mL) on E. dermatitidis [Citation145]. Evaluating other compounds with antifungal activity against black yeasts was also performed in the last years. Fungicidal activity of N-Chlorotaurine against E. dermatitidis was recently reported when added to both cystic fibrosis sputum medium (simulating the CF lung environment) and standard medium in a concentration of 1–0.1% [Citation146]. Alongside anti-mycotic agents, the success of adjunctive IFN-γ therapy in a CF patient with progressive respiratory morbidity secondary to E. dermatitidis infection was reported [Citation147]. The antibiotic colistin which is also used for inhalation therapy in CF patients exhibited antifungal activity [Citation148] and was able to reduce mature biofilm [Citation130]. Future research in developing novel anti-infective agents for the treatment of E. dermatitidis infection is warranted.
In CNS infections with E. dermatitidis, therapeutic studies suggest the complete excision of the brain abscess. This results in better outcomes compared to partial excision or antifungal therapy [Citation139]. However, if therapy with antifungals is preferred, the azoles voriconazole and posaconazole are recommended in the ESCMID/ECMM joint clinical guidelines for phaeohyphomycosis. Voriconazole is able to penetrate into brain tissue, providing clinical improvements in patients with invasive CNS mycosis. In contrast, therapy with amphotericin B alone is known to have a poor outcome in this patient group. Combination therapy of a triazole with fluorcytosine is a possible first-line therapy when surgery is not feasible [Citation139]. The combination of amphotericin B and fluorcytosine is also a useful treatment regime for fungal infections of the central nervous system (e.g. cryptococcal meningitis). This combination showed a high degree of synergism in vitro against three tested E. dermatitidis isolates [Citation149]. This therapeutic approach with surgical resection was successful in a patient with chronic granulomatous disease and progressive pulmonary and CNS infection with E. dermatitidis [Citation150]. More reports of patient cases or case series are needed to gain more evidence.
Conclusion
E. dermatitidis is an emerging opportunistic pathogen among the ever-increasing numbers of immunocompromised hosts. Several virulence factors like melanization, dimorphism, and biofilm formation have been studied and evaluated in in vitro and animal models. Understanding the mechanisms of transmission, pathogenicity and resistance will be essential for developing new strategies for better diagnosis and treatment of serious E. dermatitidis infections. In addition, it will be important to explore pathogen-specific adaptation mechanisms to the host. Studying the immune response associated with Exophiala dermatitidis infection is also a cornerstone to future research. Furthermore, the development of standardized methods for the detection and identification of E. dermatitidis, as well as the definition of species-specific breakpoints of antifungals are currently under study.
Disclosure statement
No potential conflict of interest was reported by the authors.
References
- Garber G. An overview of fungal infections. Drugs. 2001;61(Suppl 1):1–12.
- Yurlova NA, De Hoog GS. Exopolysaccharides and capsules in human pathogenic Exophiala species. Mycoses. 2002;45:443–448.
- Zeng JS, Sutton DA, Fothergill AW, et al. Spectrum of Clinically Relevant Exophiala Species in the United States. J Clin Microbiol. 2007;45:3713–3720.
- Bakare N, Rickerts V, Bargon J, et al. Prevalence of Aspergillus fumigatus and other fungal species in the sputum of adult patients with cystic fibrosis. Mycoses. 2003;46:19–23.
- Kondori N, Lindblad A, Welinder-Olsson C, et al. Development of IgG antibodies to Exophiala dermatitidis is associated with inflammatory responses in patients with cystic fibrosis. J Cyst Fibros. 2014;13:391–399.
- Lebecque P, Leonard A, Huang D, et al. Exophiala (Wangiella) dermatitidis and cystic fibrosis - Prevalence and risk factors. Med Mycol. 2010;48(Suppl 1):S4–S9.
- Haase G, Skopnik H, Kusenbach G. Exophiala dermatitidis infection in cystic fibrosis. Lancet. 1990;336:188–189.
- Li D-M, Li R-Y, de Hoog GS, et al. Fatal Exophiala infections in China, with a report of seven cases. Mycoses. 2011;54:e136–e142.
- Blasi B, Tafer H, Tesei D, et al. From glacier to sauna: RNA-Seq of the human pathogen black fungus Exophiala dermatitidis under varying temperature conditions exhibits common and novel fungal response. PLoS One. 2015;10:e0127103.
- Matos T, de Hoog GS, de Boer AG, et al. High prevalence of the neurotrope Exophiala dermatitidis and related oligotrophic black yeasts in sauna facilities. Mycoses. 2002;45:373–377.
- Hu B, Li S, Hu H, et al. Central nervous system infection caused by Exophiala dermatitidis in a case and literature review. Zhonghua Er Ke Za Zhi = Chinese J Pediatr. 2014;52:620–624.
- Taj-Aldeen SJ, El Shafie S, Alsoub H, et al. Isolation of Exophiala dermatitidis from endotracheal aspirate of a cancer patient. Mycoses. 2006;49:504–509.
- Matsumoto T, Matsuda T, McGinnis MR, et al. Clinical and mycological spectra of Wangiella dermatitidis infections. Mycoses. 1993;36:145–155.
- Hohl PE, Holley HP, Prevost E, et al. Infections due to Wangiella dermatitidis in humans: report of the first documented case from the United States and a review of the literature. Rev Infect Dis. 1983;5:854–864.
- Hoog GSD, Queiroz-Telles F, Haase G, et al. Black fungi: clinical and pathogenic approaches. Med Mycol. 2000;38:243–250.
- Moussa TAA, Al-Zahrani HS, Kadasa NMS, et al. Nomenclatural notes on Nadsoniella and the human opportunist black yeast genus Exophiala. Mycoses. 2017;60:358–365.
- De Hoog GS, Guarro J. Atlas of clinical fungi. Mycoses. 1995.
- Sudhadham M, Prakitsin S, Sivichai S, et al. The neurotropic black yeast Exophiala dermatitidis has a possible origin in the tropical rain forest. Stud Mycol. 2008;61:145–155.
- Seyedmousavi S, Netea MG, Mouton JW, et al. Black yeasts and their filamentous relatives: principles of pathogenesis and host defense. Clin Microbiol Rev. 2014;27:527–542.
- Song Y, Laureijssen-van de Sande WWJ, Moreno LF, et al. Comparative ecology of capsular Exophiala species causing disseminated infection in humans. Front Microbiol. 2017;8:2514.
- Suzuki K, Nakamura A, Fujieda A, et al. Pulmonary infection caused by Exophiala dermatitidis in a patient with multiple myeloma: A case report and a review of the literature. Med Mycol Case Rep. 2012;1:95–98.
- Babič MN, Zupančič J, Gunde-Cimerman N, et al. Ecology of the human opportunistic black yeast Exophiala dermatitidis indicates preference for human-made habitats. Mycopathologia. 2018;183:201–212.
- Gümral R, Özhak-Baysan B, Tümgör A, et al. Dishwashers provide a selective extreme environment for human-opportunistic yeast-like fungi. Fungal Divers. 2016;76:1–9.
- Zupančič J, Novak Babič M, Zalar P, et al. The black yeast Exophiala dermatitidis and other selected opportunistic human fungal pathogens spread from dishwashers to kitchens. Sturtevant J, editor. PLoS One. 2016;11:e0148166.
- Nishimura K, Miyaji M. Studies on a saprophyte of Exophiala dermatitidis isolated from a humidifier. Mycopathologia. 1982;77:173–181.
- Zalar P, Novak M, de Hoog GS, et al. Dishwashers – A man-made ecological niche accommodating human opportunistic fungal pathogens. Fungal Biol. 2011;115:997–1007.
- Yazdanparast SA, Mohseni S, De Hoog GS, et al. Consistent high prevalence of Exophiala dermatitidis, a neurotropic opportunist, on railway sleepers. J Mycol Med. 2017;27:180–187.
- Saunte DM, Tarazooie B, Arendrup MC, et al. Black yeast-like fungi in skin and nail: it probably matters. Mycoses. 2011;55:161–167.
- Kondori N, Gilljam M, Lindblad A, et al. High rate of Exophiala dermatitidis recovery in the airways of patients with cystic fibrosis is associated with pancreatic insufficiency. J Clin Microbiol. 2011;49:1004–1009.
- Murphy KF, Malik R, Barnes A, et al. Successful treatment of intra-abdominal Exophiala dermatitidis infection in a dog. Vet Rec. 2011;168:217.
- Kano R, Kusuda M, Nakamura Y, et al. First isolation of Exophiala dermatitidis from a dog: identification by molecular analysis. Vet Microbiol. 2000;76:201–205.
- Muotoe-Okafor FA, Gugnani HC. Isolation of Lecythophora mutabilis and Wangiella dermatitidis from the fruit eating bat, Eidolon helvum. Mycopathologia. 1993;122:95–100.
- Kusenbach G, Skopnik H, Haase G, et al. Exophiala dermatitidis pneumonia in cystic fibrosis. Eur J Pediatr. 1992;151:344–346.
- Pihet M, Carrere J, Cimon B, et al. Occurrence and relevance of filamentous fungi in respiratory secretions of patients with cystic fibrosis – a review. Med Mycol. 2009;47:387–397.
- Grenouillet F, Cimon B, Pana-Katatali H, et al. Exophiala dermatitidis revealing cystic fibrosis in adult patients with chronic pulmonary disease. Mycopathologia. 2018;183:71–79.
- Lanternier F, Barbati E, Meinzer U, et al. Inherited CARD9 deficiency in 2 unrelated patients with invasive Exophiala infection. J Infect Dis. 2015;211:1241–1250.
- Silva WC, Gonçalves SS, Santos DWCL, et al. Species diversity, antifungal susceptibility and phenotypic and genotypic characterisation of Exophiala spp. infecting patients in different medical centres in Brazil. Mycoses. 2017;60:328–337.
- Chalkias S, Alonso CD, Levine JD, et al. Emerging pathogen in immunocompromised hosts: Exophiala dermatitidis mycosis in graft-versus-host disease. Transpl Infect Dis. 2014;16:616–620.
- Sood S, Vaid V, Sharma M, et al. Cerebral phaeohyphomycosis by Exophiala dermatitidis. Indian J Med Microbiol. 2014;32:188.
- Chen M, Zhang J, Dong Z, et al. Cutaneous phaeohyphomycosis caused by Exophiala dermatitidis: A case report and literature review. Indian J Dermatology, Venereol Leprol. 2016;82:173.
- Tanuskova D, Horakova J, Buzassyova D, et al. A case of Exophiala dermatitidis infection in a child after allogeneic stem cell transplantation: case report and literature review of paediatric cases. JMM Case Rep. 2017;4:e005102.
- Teixeira MMR, Assuncao CB, Lyon S, et al. A case of subcutaneous phaeohyphomycosis associated with leprosy. Infect Disord - Drug Targets. 2017;17:223–226.
- Gupta AJ, Singh M, Yadav S, et al. Phaeohyphomycosis breast masquerading as fibroadenoma in a young teenage girl. Diagn Cytopathol. 2017;45:939–942.
- Lang R, Minion J, Skinner S, et al. Disseminated Exophiala dermatitidis causing septic arthritis and osteomyelitis. BMC Infect Dis. 2018;18:255.
- Homa M, Manikandan P, Saravanan V, et al. Exophiala dermatitidis Endophthalmitis: case report and literature review. Mycopathologia. 2018;183:603–609.
- Ozawa Y, Suda T, Kaida Y, et al. A case of bronchial infection of Wangiella dermatitidis. Nihon Kokyuki Gakkai Zasshi. 2007;45:907–911.
- Oztas E, Odemis B, Kekilli M, et al. Systemic phaeohyphomycosis resembling primary sclerosing cholangitis caused by Exophiala dermatitidis. J Med Microbiol. 2009;58:1243–1246.
- Chang X, Li R, Yu J, et al. Phaeohyphomycosis of the central nervous system caused by Exophiala dermatitidis in a 3-year-old immunocompetent host. J Child Neurol. 2009;24:342–345.
- Alabaz D, Kibar F, Arikan S, et al. Systemic phaeohyphomycosis due to Exophiala (Wangiella) in an immunocompetent child. Med Mycol. 2009;47:653–657.
- Patel AK, Patel KK, Darji P, et al. Exophiala dermatitidis endocarditis on native aortic valve in a postrenal transplant patient and review of literature on E. dermatitidis infections. Mycoses. 2013;56:365–372.
- Clamp MF, Jumper JM, Ku CW, et al. Chronic exogenous Exophiala dermatitidis endophthalmitis. Retin Cases Brief Rep. 2014;8:265–268.
- Poyntner C, Blasi B, Arcalis E, et al. The transcriptome of Exophiala dermatitidis during Ex-vivo skin model infection. Front Cell Infect Microbiol. 2016;6:136.
- Prenafeta-Boldú FX, Summerbell R, Sybren de Hoog G. Fungi growing on aromatic hydrocarbons: biotechnology’s unexpected encounter with biohazard? FEMS Microbiol Rev. 2006;30:109–130.
- Horré R, de Hoog GS. Primary cerebral infections by melanized fungi: A review. Stud Mycol. 1999;43:176–193.
- Uijthof JM, de Hoog GS, de Cock AW, et al. Pathogenicity of strains of the black yeast Exophiala (Wangiella) dermatitidis: an evaluation based on polymerase chain reaction. Mycoses. 1994;37:235–242.
- Centers for Disease Control and Prevention (CDC). Exophiala infection from contaminated injectable steroids prepared by a compounding pharmacy-United States, July-November 2002. MMWR Morb Mortal Wkly Rep. 2002;51:1109–1112.
- Woollons A, Darley CR, Pandian S, et al. Phaeohyphomycosis caused by Exophiala dermatitidis following intra-articular steroid injection. Br J Dermatol. 1996;135:475–477.
- Vasquez A, Zavasky D, Chow NA, et al. Management of an outbreak of Exophiala dermatitidis bloodstream infections at an outpatient oncology clinic. Clin Infect Dis. 2017;66: 959–962.
- Cañete-Gibas CF, Wiederhold NP. The black yeasts: an update on species identification and diagnosis. Curr Fungal Infect Rep. 2018;12:59–65.
- Raclavsky V, Novotny R. Burkholderia cepacia selective agar can be useful for recovery of Exophiala dermatitidis from sputum samples of cystic fibrosis patients. J Cyst Fibros. 2016;15:e19.
- Horré R, Schaal KP, Siekmeier R, et al. Isolation of fungi, especially Exophiala dermatitidis, in patients suffering from cystic fibrosis. A Prospective Study Respiration. 2004;71:360–366.
- Jayaram M, Nagao H. Potato dextrose agar with rose-bengal and chloramphenicol: A new culture medium to isolate pathogenic Exophiala dermatitidis from the environment. Klimik Dergisi/Klimik J. 2018;11–15.
- Zeng JS, De Hoog GS. Exophiala spinifera and its allies: diagnostics from morphology to DNA barcoding. Med Mycol. 2008;46:193–208.
- Heinrichs G, de Hoog GS, Haase G. Barcode identifiers as a practical tool for reliable species assignment of medically important black yeast species. J Clin Microbiol. 2012;50:3023–3030.
- Fraser M, Brown Z, Houldsworth M, et al. Rapid identification of 6328 isolates of pathogenic yeasts using MALDI-ToF MS and a simplified, rapid extraction procedure that is compatible with the Bruker Biotyper platform and database. Med Mycol. 2015;54:myv085.
- Pinto A, Halliday C, Zahra M, et al. Matrix-assisted laser desorption ionization-time of flight mass spectrometry identification of yeasts is contingent on robust Rreference spectra. Heimesaat MM, editor. PLoS One. 2011;6:e25712.
- Özhak-Baysan B, Öğünç D, Döğen A, et al. MALDI-TOF MS-based identification of black yeasts of the genus Exophiala. Med Mycol. 2015;53:347–352.
- Borman AM, Fraser M, Szekely A, et al. Rapid identification of clinically relevant members of the genus Exophiala by matrix-assisted laser desorption ionization–time of flight mass spectrometry and description of two novel species, Exophiala campbellii and Exophiala lavatrina. Diekema DJ, editor. J Clin Microbiol. 2017;55:1162–1176.
- Ç E, Gök Y, Bayğu Y, et al. ATR-FTIR spectroscopy highlights the problem of distinguishing between Exophiala dermatitidis and E. phaeomuriformis using MALDI-TOF MS. Microb Ecol. 2016;71:339–346.
- Nagano Y, Elborn JS, Millar BC, et al. Development of a novel PCR assay for the identification of the black yeast, Exophiala (Wangiella) dermatitidis from adult patients with cystic fibrosis (CF). J Cyst Fibros. 2008;7:576–580.
- Schoch CL, Seifert KA, Huhndorf S, et al. Nuclear ribosomal internal transcribed spacer (ITS) region as a universal DNA barcode marker for fungi. Proc Natl Acad Sci U S A. 2012;109:6241–6246.
- Suh MK, Lee HC, Kim DM, et al. Molecular phylogenetics of Exophiala species isolated from Korea. Ann Dermatol. 2012;24:287.
- Nagano Y, Elborn JS, Millar BC, et al. Comparison of techniques to examine the diversity of fungi in adult patients with cystic fibrosis. Med Mycol. 2010;48:166–176.
- Rath P-M, Müller K-D, Dermoumi H, et al. A comparison of methods of phenotypic and genotypic fingerprinting of Exophiala dermatitidis isolated from sputum samples of patients with cystic fibrosis. J Med Microbiol. 1997;46:757–762.
- Sudhadham M, Gerrits van Den Ende AHG, Sihanonth P, et al. Elucidation of distribution patterns and possible infection routes of the neurotropic black yeast Exophiala dermatitidis using AFLP. Fungal Biol. 2011;115:1051–1065.
- Machouart M, Gueidan C, Khemisti A, et al. Use of ribosomal introns as new markers of genetic diversity in Exophiala dermatitidis. Fungal Biol. 2011;115:1038–1050.
- Döğen A, Kaplan E, Öksüz Z, et al. Dishwashers are a major source of human opportunistic yeast-like fungi in indoor environments in Mersin, Turkey. Med Mycol. 2013;51:493–498.
- Geiser DM, Gueidan C, Miadlikowska J, et al. Eurotiomycetes: eurotiomycetidae and Chaetothyriomycetidae. Mycologia. 2006;98:1053–1064.
- Haase G, Sonntag L, van de Peer Y, et al. Phylogenetic analysis of ten black yeast species using nuclear small subunit rRNA gene sequences. Antonie Van Leeuwenhoek. 1995;68:19–33.
- Gostinčar C, Lenassi M, Gunde-Cimerman N, et al. Fungal adaptation to extremely high Salt concentrations. Adv Appl Microbiol. 2011;77:71–96.
- Gostinčar C, Muggia L, Grube M. Polyextremotolerant black fungi: oligotrophism, adaptive potential, and a link to lichen symbioses. Front Microbiol. 2012;3:390.
- Padhye AA, McGinnis MR, Ajello L. Thermotolerance of Wangiella dermatitidis. J Clin Microbiol. 1978;8:424–426.
- de Hoog GS, Takeo K, Yoshida S, et al. Pleoanamorphic life cycle of Exophiala (Wangiella) dermatitidis. Antonie Van Leeuwenhoek. 1994;65:143–153.
- Boral H, Metin B, Döğen A, et al. Overview of selected virulence attributes in Aspergillus fumigatus, Candida albicans, Cryptococcus neoformans, Trichophyton rubrum, and Exophiala dermatitidis. Fungal Genet Biol. 2018;111:92–107.
- Sheppard DC, Howell PL. Biofilm exopolysaccharides of pathogenic fungi: lessons from bacteria. J Biol Chem. 2016;291:12529–12537.
- Gauthier GM. Fungal dimorphism and virulence: molecular mechanisms for temperature adaptation, immune evasion, and in vivo survival. Mediators Inflamm. 2017;2017:8491383.
- Wang Q, Szaniszlo PJ. WdStuAp, an APSES transcription factor, is a regulator of yeast-hyphal transitions in Wangiellla (Exophiala) dermatitidis. Eukaryot Cell. 2007;6:1595–1605.
- Oujezdsky KB, Grove SN, Szaniszlo PJ. Morphologica and structural changes during the yeast-to mold conversion of Phialophora dermatitidis. J Bacteriol. 1973;113:468–477.
- Karuppayil SM, Szaniszlo PJ. Importance of calcium to the regulation of polymorphism in Wangiella (Exophiala) dermatitidis. J Med Vet Mycol. 1997;35:379–388.
- Staerck C, Vandeputte P, Gastebois A, et al. Enzymatic mechanisms involved in evasion of fungi to the oxidative stress: focus on Scedosporium apiospermum. Mycopathologia. 2018;183:227–239.
- Olsowski M, Hoffmann F, Hain A, et al. Exophiala dermatitidis isolates from various sources: using alternative invertebrate host organisms (Caenorhabditis elegans and Galleria mellonella) to determine virulence. Sci Rep. 2018;8:12747.
- Dixon DM, Polak A, Szaniszlo PJ. Pathogenicity and virulence of wild-type and melanin-deficient Wangiella dermatitidis. J Med Vet Mycol. 1987;25:97–106.
- Feng B, Wang X, Hauser M, et al. Molecular cloning and characterization of WdPKS1, a gene involved in dihydroxynaphthalene melanin biosynthesis and virulence in Wangiella (Exophiala) dermatitidis. Infect Immun. 2001;69:1781–1794.
- Langfelder K, Streibel M, Jahn B, et al. Biosynthesis of fungal melanins and their importance for human pathogenic fungi. Fungal Genet Biol. 2003;38:143–158.
- Chen Z, Martinez DA, Gujja S, et al. Comparative genomic and transcriptomic analysis of Wangiella dermatitidis, a major cause of phaeohyphomycosis and a model black yeast human pathogen. G3 (Bethesda). 2014;4:561–578.
- de Hoog GS. Evolution of black yeasts: possible adaptation to the human host. Antonie Van Leeuwenhoek. 1993;63:105–109.
- Sav H, Ozakkas F, Altınbas R, et al. Virulence markers of opportunistic black yeast in Exophiala. Mycoses. 2016;59:343–350.
- Abramczyk D, Szaniszlo PJ. Immunoaffinity purification of the class V chitin synthase of Wangiella (Exophiala) dermatitidis. Prep Biochem Biotechnol. 2009;39:277–288.
- Wang Z, Szaniszlo PJ. Characterization of WdChs3p, a class III chitin synthase, of Wangiella (Exophiala) dermatitidis, overexpressed in Saccharomyces cerevisiae. Med Mycol. 2002;40:283–289.
- Wang Z, Zheng L, Liu H, et al. WdChs2p, a class I chitin synthase, together with WdChs3p (class III) contributes to virulence in Wangiella (Exophiala) dermatitidis. Infect Immun. 2001;69:7517–7526.
- Kindler BLJ, H-J K, Nies S, et al. Generation of indole alkaloids in the human-pathogenic fungus Exophiala dermatitidis. European J Org Chem. 2010;2010:2084–2090.
- Isola D, Selbmann L, de Hoog GS, et al. Isolation and screening of black fungi as degraders of volatile aromatic hydrocarbons. Mycopathologia. 2013;175:369–379.
- Moreno LF, Ahmed AAO, Brankovics B, et al. Genomic understanding of an infectious brain disease from the desert. G3 Genes|Genomes|Genetics. 2018;8:909–922.
- Schnitzler N, Peltroche-Llacsahuanga H, Bestier N, et al. Effect of melanin and carotenoids of Exophiala (Wangiella) dermatitidis on phagocytosis, oxidative burst, and killing by human neutrophils. Infect Immun. 1999;67:94–101.
- Plonka PM, Grabacka M. Melanin synthesis in microorganisms - Biotechnological and medical aspects. Acta Biochim Pol. 2006;53:429–443.
- Revankar SG, Sutton DA. Melanized fungi in human disease. Clin Microbiol Rev. 2010;23:884–928.
- Casadevall A, Rosas AL, Nosanchuk JD. Melanin and virulence in Cryptococcus neoformans. Curr Opin Microbiol. 2000;3:354–358.
- Geib E, Gressler M, Viediernikova I, et al. A non-canonical melanin biosynthesis pathway protects Aspergillus terreus conidia from environmental stress. Cell Chem Biol. 2016;23:587–597.
- Eisenman HC, Casadevall A. Synthesis and assembly of fungal melanin. Appl Microbiol Biotechnol. 2012;93:931–940.
- Jacobson ES, Hove E, Emery HS. Antioxidant function of melanin in black fungi. Infect Immun. 1995;63:4944–4945.
- Cordero RJB, Casadevall A. Functions of fungal melanin beyond virulence. Fungal Biol Rev. 2017;31:99–112.
- Dadachova E, Bryan RA, Huang X, et al. Ionizing radiation changes the electronic properties of melanin and enhances the growth of melanized fungi. Rutherford J, editor. PLoS One. 2007;2:e457.
- Dadachova E, Casadevall A. Ionizing radiation: how fungi cope, adapt, and exploit with the help of melanin. Curr Opin Microbiol. 2008;11:525–531.
- Jiang S. Immunity against fungal Infections. Immunol Immunogenet Insights. 2016;8:III.S38707.
- Geis PA, Wheeler MH, Szaniszlo PJ. Pentaketide metabolites of melanin synthesis in the dematiaceous fungus Wangiella dermatitidis. Arch Microbiol. 1984;137:324–328.
- Schmaler-Ripcke J, Sugareva V, Gebhardt P, et al. Production of pyomelanin, a second type of melanin, via the tyrosine degradation pathway in Aspergillus fumigatus. Appl Environ Microbiol. 2009;75:493–503.
- Wheeler MH, Stipanovic RD. Melanin biosynthesis and the metabolism of flaviolin and 2-hydroxyjuglone in Wangiella dermatitidis. Arch Microbiol. 1985;142:234–241.
- Poyntner C, Mirastschijski U, Sterflinger K, et al. Transcriptome study of an Exophiala dermatitidis PKS1 mutant on an ex vivo skin model: is melanin important for infection?. Front Microbiol. 2018;9:1457.
- Wang Y, Aisen P, Casadevall A. Cryptococcus neoformans melanin and virulence: mechanism of action. Infect Immun. 1995;63:3131–3136.
- Robertson KL, Mostaghim A, Cuomo CA, et al. Adaptation of the black yeast Wangiella dermatitidis to ionizing radiation: molecular and cellular mechanisms. Nielsen K, editor. PLoS One. 2012;7:e48674.
- Hamilton AJ, Gomez BL. Melanins in fungal pathogens. J Med Microbiol. 2002;51:189–191.
- Mohagheghpour N, Waleh N, Garger SJ, et al. Synthetic melanin suppresses production of proinflammatory cytokines. Cell Immunol. 2000;199:25–36.
- Polak A, Dixon DM. Loss of melanin in Wangiella dermatitidis does not result in greater susceptibility to antifungal agents. Antimicrob Agents Chemother. 1989;33:1639–1640.
- Marova I, Breierova E, Koci R, et al. Influence of exogenous stress factors on production of carotenoids by some strains of carotenogenic yeasts. Ann Microbiol. 2004;54:73–85.
- Gorbushina AA, Kotlova ER, Sherstneva OA. Cellular responses of microcolonial rock fungi to long-term desiccation and subsequent rehydration. Stud Mycol. 2008;61:91–97.
- de Nobel H, van Den Ende H, Klis FM. Cell wall maintenance in fungi. Trends Microbiol. 2000;8:344–345.
- Lewis K. Persister cells. Annu Rev Microbiol. 2010;64:357–372.
- Heinrichs G, Hübner I, Schmidt CK, et al. Analysis of black fungal biofilms occurring at domestic water taps (II): potential routes of entry. Mycopathologia. 2013;175:399–412.
- Zupančič J, Raghupathi PK, Houf K, et al. Synergistic interactions in microbial biofilms facilitate the establishment of opportunistic pathogenic fungi in household dishwashers. Front Microbiol. 2018;9.
- Kirchhoff L, Olsowski M, Zilmans K, et al. Biofilm formation of the black yeast-like fungus Exophiala dermatitidis and its susceptibility to antiinfective agents. Sci Rep. 2017;7.
- Seneviratne CJ, Fong PH, Wong SS, et al. Antifungal susceptibility and phenotypic characterization of oral isolates of a black fungus from a nasopharyngeal carcinoma patient under radiotherapy. BMC Oral Health. 2015;15:39.
- Gao L, Sun Y, He C, et al. Synergistic effects of tacrolimus and azoles against Exophiala dermatitidis. Antimicrob Agents Chemother. 2017;61:e00948–17.
- Nweze EI, Ezute S. Isolation and antifungal susceptibility of Exophiala dermatitidis isolates from human stool samples in Nigeria. Mycopathologia. 2010;169:201–206.
- Badali H, de Hoog GS, Sudhadham M, et al. Microdilution in vitro antifungal susceptibility of Exophiala dermatitidis, a systemic opportunist. Med Mycol. 2011;49:819–824.
- Deng S, Lei W, de Hoog GS, et al. Combination of amphotericin B and terbinafine against melanized fungi associated with chromoblastomycosis. Antimicrob Agents Chemother. 2018;62:e00270–18.
- Duarte APM, Pagnocca FC, Baron NC, et al. In vitro susceptibility of environmental isolates of Exophiala dermatitidis to five antifungal drugs. Mycopathologia. 2013;175:455–461.
- Gülmez D, Doğan Ö, Boral B, et al. In vitro activities of antifungal drugs against environmental Exophiala isolates and review of the literature. Mycoses. 2018;61:561–569.
- Fothergill AW, Rinaldi MG, Sutton DA. Antifungal susceptibility testing of Exophiala spp.: a head-to-head comparison of amphotericin B, itraconazole, posaconazole and voriconazole. Med Mycol. 2009;47:41–43.
- Chowdhary A, Meis JF, Guarro J, et al. ESCMID and ECMM joint clinical guidelines for the diagnosis and management of systemic phaeohyphomycosis: diseases caused by black fungi. Clin Microbiol Infect. 2014;20:47–75.
- Meletiadis J, Meis JFGM, Hoog GSD, et al. In vitro susceptibilities of 11 clinical isolates of Exophiala species to six antifungal drugs. Mycoses. 2000;43:309–312.
- Watanabe N, Gotoh A, Shirane S, et al. Breakthrough Exophiala dermatitidis infection during prophylactic administration of micafungin during second umbilical cord blood transplantation after graft failure. Transpl Infect Dis. 2018;20:e12833.
- Johnson EM, Szekely A, Warnock DW. In-vitro activity of voriconazole, itraconazole and amphotericin B against filamentous fungi. J Antimicrob Chemother. 1998;42:741–745.
- Schwarz C, Hartl D, Eickmeier O, et al. Progress in definition, prevention and treatment of fungal infections in cystic fibrosis. Mycopathologia. 2018;183:21–32.
- Yamazaki T, Inagaki Y, Fujii T, et al. In vitro activity of isavuconazole against 140 reference fungal strains and 165 clinically isolated yeasts from Japan. Int J Antimicrob Agents. 2010;36:324–331.
- Seufert R, Dittmer S, Heep M, et al. In vitro activity of F901318 against filamentous fungi from cystic fibrosis patients. Mycoses. 2018;61:3–22.
- Gruber M, Moser I, Nagl M, et al. Bactericidal and fungicidal activity of N -chlorotaurine is enhanced in cystic fibrosis sputum medium. Antimicrob Agents Chemother. 2017;61:e02527–16.
- Eades CP, Armstrong-James DPH, Periselneris J, et al. Improvement in Exophiala dermatitidis airway persistence and respiratory decline in response to interferon-gamma therapy in a patient with cystic fibrosis. J Cyst Fibros. 2018;17:e32–e34.
- Schemuth H, Dittmer S, Lackner M, et al. In vitro activity of colistin as single agent and in combination with antifungals against filamentous fungi occurring in patients with cystic fibrosis. Mycoses. 2013;56:297–303.
- Deng S, Pan W, Liao W, et al. Combination of amphotericin B and flucytosine against neurotropic species of melanized fungi causing primary cerebral Pphaeohyphomycosis. Antimicrob Agents Chemother. 2016;60:2346–2351.
- Kenney RT, Kwon-Chung KJ, Waytes AT, et al. Successful treatment of systemic Exophiala dermatitidis infection in a patient with chronic granulomatous disease. Clin Infect Dis. 1992;14:235–242.