ABSTRACT
Staphylococcus aureus is a major pathogen causing intramammary infection and mastitis in dairy cows. S. aureus genotypes (GT) can differ significantly in their ability to diffuse and persist in the herd; while the association of virulence gene carriage with epidemiological behavior remains unclear, a role for secreted proteins has been postulated. We characterized the secretome of six S. aureus strains belonging to two genotypes with opposite within-herd prevalence, GTB (high) and GTS (low), corresponding to sequence types (ST) 8 and 398, by high-resolution tandem mass spectrometry and differential analysis with Proteome Discoverer. Data are available via ProteomeXchange with identifier PXD029571. Out of 720 identified proteins, 98 were unique or more abundant in GTB/ST8 and 68 in GTS/ST398. GTB/ST8 released more immunoglobulin-binding proteins, complement and antimicrobial peptide inhibitors, enterotoxins, and metabolic enzymes, while GTS/ST398 released more leukocidins, hemolysins, lipases, and peptidases. Furthermore, GTB/ST8 released the von Willebrand factor protein, staphylokinase, and clumping factor B, while GTS released the staphylococcal coagulase and clumping factor A. Hence, GTB/ST8 secretomes indicated a higher propensity for immune evasion and chronicity and GTS/ST398 secretomes for cellular damage and inflammation, consistent with their epidemiological characteristics. Accordingly, GTS/ST398 secretions were significantly more cytotoxic against bovine PBMCs in vitro. Our findings confirm the crucial role of extracellular virulence factors in S. aureus pathogenesis and highlight the need to investigate their differential release adding to gene carriage for a better understanding of the relationship of S. aureus genotypes with epidemiological behavior and, possibly, disease severity.
Introduction
Staphylococcus aureus is still a relevant cause of bovine mastitis worldwide, despite efforts to control its presence and diffusion in dairy herds. A multitude of factors associated with the phenotypic and genotypic characteristics of the infecting strain influence its ability to spread and persist in the herd, as well as the outcome of disease. S. aureus strains isolated from milk samples of cows with mastitis are genetically heterogeneous and can harbor an extensive array of virulence-associated genes. However, only a few genotypes have been linked to a high within-herd prevalence of intramammary infection (IMI) [Citation1–3]. Previous European studies used Ribosomal Spacer PCR (RS-PCR) to classify S. aureus strains in genotypes. These demonstrated that S. aureus belonging to genotype B (GTB) are associated with a high diffusion within the herd and are frequently isolated from dairy farms in Central Europe and in Italy [Citation4]. Genotype B generally corresponds to Sequence Type 8 (ST8), a bovine-adapted genotype originated from a human-to-cow host jump [Citation5]. The highly contagious S. aureus GTB/ST8 strains are characterized by the presence of specific genes coding for enterotoxins (sea, sed, and sej) [Citation1–3,Citation6,Citation7]. On the other hand, S. aureus belonging to genotype S (GTS), which generally corresponds to ST398, are more likely associated with sporadic IMI [Citation2–4,Citation6]. S. aureus ST398 have a broad host range and represent a threat to public health for their ability to affect livestock, especially swine, and humans [Citation8], and to acquire multidrug resistance; methicillin-resistant S. aureus strains frequently belong to GTS/ST398 [Citation6]. In 2017, Capra and coworkers [Citation9] performed a detailed genomic and transcriptomic investigation of three S. aureus GTB/ST8 and three GTS/ST398 strains to shed further light on their differential characteristics. Their work revealed relevant differences in several genes associated with virulence factors, with some of them being exclusive of one genotype.
S. aureus can produce diverse secreted and surface-associated virulence factors that contribute collectively to colonization and invasion of host cells and tissues, as well as evasion of immune responses [Citation10]. Wall-bound virulence factors include Microbial Surface Components Recognizing Adhesive Matrix Molecules (MSCRAMMs) that mediate adherence to different substrates of the host [Citation10,Citation11], as well as Secreted Expanded Repertoire Adhesive Molecules [SERAMs). Among other findings, Capra and coworkers [Citation9] observed a high polymorphism in the fnbB gene between S. aureus GTB/ST8 and GTS/ST398, resulting in a truncated form of the protein in the latter strains and possibly affecting S. aureus colonization and infection efficiency [Citation9, Citation12]. In addition, the staphylococcal complement inhibitor (SCIN), which helps the bacteria to survive in the host by preventing chemotaxis and phagocytosis [Citation13], was over-expressed in GTB/ST8 and down-regulated in GTS/ST398 [Citation9]. GTB/ST8 strains also showed higher expression of signal transduction Target of RNAIII Activating Protein (TRAP), which activates RNAIII synthesis increasing the pathogenic potential of the bacteria [Citation14,Citation15]. Adding to differential secretion or release, major differences in the composition of S. aureus secretome are related to differences in transcriptional regulation by the agr system, resulting in the expression of diverse secreted virulence factors [Citation16,Citation17].
Genomic and transcriptomic investigations, however, may not reflect the actual composition of secreted virulence factors [Citation18] which can be better understood with the analysis of the extracellular proteome (secretome) [Citation16]. Previous studies found that S. aureus may differ considerably in the composition and abundance of secreted proteins [Citation16,Citation18]. Importantly, these are also thought to represent the main reservoir of virulence factors, and grouping of clinical isolates based on their secretome profile can be related to virulence [Citation19]. Investigating the bacterial secretome by high-performance shotgun proteomics is therefore a powerful approach for exploring staphylococcal pathogenicity and developing novel strategies for S. aureus detection and control, including vaccine design [Citation17,Citation20].
Here, we report the detailed characterization of the secretome of the three S. aureus GTB/ST8 and three GTS/ST398 strains previously investigated by genomics and transcriptomics [Citation9] and associated with high within-herd vs low within-herd prevalence, respectively. Some relevant phenotypic traits related to the secretome differences are also presented.
Materials and methods
S. aureus strains, culture conditions, and growth analysis
This study was carried out on 6 S. aureus strains. These had been isolated from the milk of cows with subclinical mastitis belonging to herds with different IMI prevalence and characterized by Ribosomal Spacer polymerase chain reaction (RS-PCR) and Multilocus sequence typing (MLST) [Citation6,Citation9]. Three strains, identified as GTS/ST398, had been isolated from herds with low IMI prevalence (range: 2–4%), and three strains, identified as GTB/ST8, had been isolated from herds with high IMI prevalence (range: 49–62%) [Citation6]. For this study, each S. aureus strain was thawed and revitalized in Brain Heart Infusion broth (BHI, Oxoid, Rodano, IT) overnight at 37°C. After incubation, the optical density of each suspension was measured at 620 nm (OD620) using a SpectraMax 340PC spectrophotometer (Molecular Devices, LLC, CA), diluted to an OD620 value of 0.08–0.1 in BHI broth and incubated overnight at 37°C. For whey preparation, fresh milk from 10 single quarters of as many cows with SCC ≤7,000/ml was ultracentrifuged twice for 30 min at 45,000 g at 4°C. The supernatant was transferred to a sterile bottle and then sterilized through a 0.22 μm Millipore filter. Revitalized cultures were used for inoculating milk whey with the same dilutions calculated for BHI and incubated overnight at 37°C. On the following day, overnight culture suspensions obtained in both media were diluted 1:100 in the corresponding medium (BHI or milk whey, respectively) and incubated with agitation at 37°C for 7 hours. Bacterial growth was evaluated by plating each suspension on Blood agar plates (Oxoid) in triplicate at different time points during liquid culture for evaluating the colony forming units (CFU) per mL. Statistical analysis was carried out on the growth curves with GraphPad Prism 9 (San Diego, CA) using the paired t-test for means.
Preparation of secreted proteins
S. aureus suspensions for proteomic analysis and cell viability assays were prepared in BHI broth by seeding with the overnight culture suspensions obtained as described above and incubating at 37°C with agitation for 3.5 h. Then, bacterial cultures were centrifuged at 9,300 g for 5 minutes and supernatants were transferred to new sterile Eppendorf tubes. For SDS-PAGE and mass spectrometry analysis, bacterial culture supernatants were concentrated 10x in Amicon Ultra-0.5 centrifugal filter units with Ultracel-10 membrane (Millipore, Billerica, MA, USA). Protein concentration was evaluated with the Pierce™ 660 nm Protein Assay Kit (Thermo Scientific, San Jose, CA, USA).
SDS-PAGE analysis
SDS-PAGE separation of proteins was carried out on a Tetra Cell™ with AnykD™ precast gels (Bio-Rad Laboratories, Hercules, CA, USA) according to the user manual. The concentrated BHI supernatants were mixed with loading buffer, reduced and denatured, loaded into the wells, and subjected to electrophoretic separation as described previously [Citation21]. After the run, the gels were stained with Coomassie SafeStain (Bio-Rad) for protein visualization.
Protein digestion and peptide quantitation
For shotgun proteomic analysis, the concentrated supernatants were processed by filter-aided sample preparation (FASP) [Citation22]. Briefly, samples were subjected to reduction, alkylation, and trypsin digestion within Amicon Ultra-0.5 centrifugal filter units with a 3 kDa cutoff membrane. Peptide concentrations were measured by absorbance at 280 nm using a NanoDrop 2000 spectrophotometer (Thermo Scientific, San Jose, CA, USA) using MassPREP E. coli Digest Standard (Waters, Milford, MA, USA) for calibration.
Tandem mass spectrometry analysis of peptides
Peptide mixtures were subjected to tandem mass spectrometry (MS/MS) analysis on a Q-Exactive coupled with an UltiMate 3000 RSLCnanoLC system (Thermo Scientific, San Jose, CA, USA). Peptide mixtures (4 μg) were concentrated and washed in a trapping precolumn (Acclaim PepMap C18, 75 μm × 2 cm nanoViper, 3 μm, 100 Å, Thermo Scientific) and then fractionated on a C18 RP column (Acclaim PepMap RSLC C18, 75 μm × 50 cm nanoViper, 2 μm, 100 Å, Thermo Scientific) at flow rate of 250 nL/min. The linear gradient lasted 245 minutes from 5 to 37.5% eluent B (0.1% formic acid in 80% acetonitrile) in eluent A (0.1% formic acid). Fragmentation was done by Higher Energy Collisional Dissociation (HCD) with nitrogen as the collision gas. Each sample was obtained in one growth experiment and was processed in duplicate MS/MS runs.
Proteomic data analysis
Protein identification was carried out with Proteome Discoverer (version 2.4; Thermo Scientific) and Sequest-HT as search engine. Analysis of MS/MS spectra was carried out with the following settings. Database: Staphylococcus aureus (137,957 sequences retrieved from UniProt Knowledgebase (UniprotKb), release 2021_02); enzyme: trypsin, with two missed cleavages allowed; precursor mass tolerance: 10 ppm; MS/MS tolerance: 0.02 Da; charge states: +2, +3, and +4; cysteine carbamidomethylation as static modification and methionine oxidation and acetylation (Acetyl), loss of Methionine (Met-loss) and loss of Methionine-loss+Acetylation (Met-loss+Acetyl) on N-Terminal as dynamic modifications. Protein significance and peptide validation (false discovery rate, FDR, <1%) were defined with the percolator algorithm. Peptide and protein grouping were allowed according to the Proteome Discoverer algorithm by applying the strict maximum parsimony principle. A Consensus step was performed by creating two experimental groups represented by three samples for each group, processed in duplicate MS/MS runs. A standard consensus workflow was set on Proteome Discoverer 2.4 to evaluate label-free (LFQ) and precursor ion quantification. Precursor ion abundances were calculated using intensity as abundance parameter, normalized for evaluating the abundance ratio significance among the proteins identified in the two different experimental groups. The fold ratio was calculated by the pair wise ratio-based method and the maximum allowed fold ratio was set to 100. The abundance ratio (AR) was log-transformed (Log2) and differential proteins were predicted using the t-test (background based) and adjusting the p-value by Benjamini-Hochberg correction. Proteins were considered as significantly different if they had an abundance ratio of less than or equal to −1.5 and greater than or equal to +1.5 (−1.5≤ AR≥+1.5) with an adjusted p-value ≤0.05. Principal component analysis (PCA) was performed using the normalized abundance of all identified proteins with Proteome Discoverer 2.4. Biological processes and molecular functions of the differential proteins were retrieved from their UniProtKB entry pages. Normalized protein abundance values were calculated with Proteome Discoverer. The differential protein abundance heatmaps were prepared using Microsoft ExcelTM.
Isolation of PBMCs
Whole blood from 6 clinically healthy pluriparous dairy cows was collected in sterile tubes with EDTA as an anticoagulant during routine slaughtering procedures. The peripheral blood mononuclear cells (PBMCs) were purified as previously reported from 60 mL of whole blood [Citation23]. Briefly, the tubes were centrifuged at 1,260 g for 30 min at 18°C without brake, and the buffy coat was collected and diluted in cold PBS + 2 mM EDTA (1:2 dilution). The diluted buffy coat (10 mL) was layered on 3 mL of Ficoll-Paque Plus (1.077 g/mL) and centrifuged at 1,700 g for 30 min at 4°C without brake. The PBMC ring was collected, and the cells were counted with an automatic cell counter (TC20TM, BioRad) in Trypan blue. The cells were then resuspended in complete medium (RPMI-1640 + 25 mM Hepes, 10% heat-inactivated FBS, 1% penicillin/streptomycin, 1% non-essential amino acids).
Determination of cell viability
Cell viability was determined using the Cell Proliferation Kit I (MTT, Roche), following the manufacturer’s instructions. To determine the cytotoxicity of GTB/ST8 and GTS/ST398 S. aureus secretomes, 1 × 105 cells/well were seeded in 96-well plates and incubated for 18 hours with increasing concentrations (0.5%, 1%, 2.5% and 10%) of the 1X secreted protein preparation obtained as described above but using RPMI-1640 as the growth medium. Cells incubated with complete medium only were used as control. After the incubation period, 10 μl of MTT reagent was added to each well and incubated for 4 hours; 100 μL of solubilization buffer was added and the cells were incubated overnight. Absorbance was subsequently measured with a LabSystems Multiskan plate reader spectrophotometer (LabX, Midland, Canada) at a test wavelength of 550 nm. Data were expressed as fold change compared to the control. Six technical replicates (six replicate wells) were performed for each measurement. Statistical analysis was carried out using GraphPad Prism 9 and the normal distribution of the dataset was assessed using the Shapiro Wilk test. Kruskal-Wallis and Dunn’s multiple comparisons test were used. Statistical significance was accepted at P ≤ 0.05.
Results
Phenotypic characteristics
All the strains had similar morphology when plated on blood agar; the colonies were pale yellow, round, smooth, and surrounded by a halo of hemolysis. All the GTS/ST398 strain produced a β-hemolysis versus only one GTB/ST8 (GTB3) strain. When grown in milk whey, all GTB/ST8 strains yielded a visible, large white clot, while the GTS/ST398 did not induce protein coagulation. All strains reached the logarithmic phase of growth at 3.5 h of liquid culture in both BHI and milk whey. While GTB strains grew significantly slower than GTS strains in milk whey (p < 0.01, data not shown), no statistically significant differences were observed in BHI, the growth medium used for proteomic analysis. The growth curves observed in BHI for the six analyzed strains are illustrated in .
Figure 1. Growth curves in brain-heart infusion broth (BHI) medium of the six Staphylococcus aureus GTB/ST8 (shades of blue) and GTS/ST398 (shades of Orange) strains used in this study. The curves report the bacterial colony forming units (Log10 CFU)/mL as a function of time. Each point represents the mean (symbol) and standard deviation (bars) of three replicate CFU measurements. The X axis indicates the sampling times.
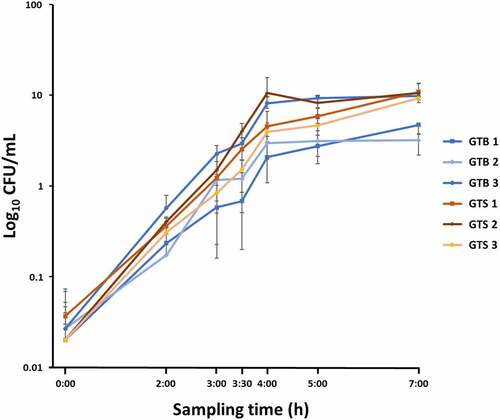
SDS-PAGE analysis of cellular and extracellular proteins
Secreted bacterial proteins were analyzed at 3.5 h of growth in BHI. The secreted proteins of the six investigated strains were first analyzed by SDS-PAGE for a visual comparison. As shown in , secreted protein profiles showed evident differences between strains of the two genotypes. This prompted us to further investigate the secretome by high-performance differential shotgun proteomics to shed light on these differences.
Differential shotgun proteomics; general results
By applying high-performance tandem mass spectrometry and Proteome Discoverer analysis for protein identification, we identified a total of 720 unique proteins in the six secreted protein samples. Principal component analysis (PCA) carried out on the protein normalized abundance data clustered the six strains according to the GT/ST by the first component (48.9%) ()). Upon differential proteomic analysis with Proteome Discoverer, 166 proteins showed a significantly different abundance between the two genotypes: 98 were more abundant in GTB/ST8 and 68 in GTS/ST398 ()).
Figure 3. General results of the differential shotgun proteomics of the Staphylococcus aureus secretome obtained in brain-heart infusion (BHI) broth. (a) Principal Component Analysis based on the normalized protein abundances. (b) Venn diagram illustrating the distribution of the 720 proteins identified in the secretomes of the two GT (ST), showing shared proteins and differential proteins identified for each sample group.
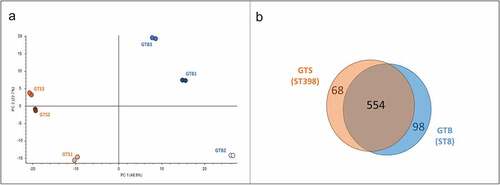
Functional analysis of the differential proteins
The differential secreted proteins identified in the GTB/ST8 and GTS/ST398 strains are reported in , respectively. reports the distribution of the protein functions based on the categories reported in . Most of the unique/differential proteins in GTS/ST398 had a well-recognized role in staphylococcal pathogenesis (37%) against 26% in GTB/ST8. In GTS/ST398, 48% mediated eukaryotic cell lysis, while in GTB/ST8 36% were primarily involved in immune evasion. Conversely, a large part of the differential proteins in GTB/ST8 (26%) were metabolic enzymes, mainly of the carbohydrate metabolism. Differential metabolic enzymes were only 16% of the GTS/ST398 secretome and 60% them belonged to the lipid metabolism. Phage-encoded proteins were 12% in GTS/ST398 and only 1% in GTB/ST8. Of note, 6% of all GTB/ST8 differential proteins were ribosomal proteins, found only in this genotype.
Table 1. Lists of the 98 proteins found exclusively (log2 + 6.64) or at significantly higher levels (log2 > +1.50) in the secretome of GTB/ST8 strains grown in brain-heart infusion (BHI) broth. The information reported in the first three columns (accession number, protein name, and abundance ratio – AR – expressed as the log2 value) was obtained by Proteome Discoverer Analysis. The last column reports biological process and molecular function information as found in the UniProtKB. The definition “other” indicates that the protein belongs to metabolic pathways different than amino acid, carbohydrate, lipid, or nucleotide metabolism
Table 2. Lists of the 68 proteins found exclusively (log2 − 6.64) or at significantly higher levels (log2 > −1.50) in the secretome of GTS/ST398 strains grown in brain-heart infusion (BHI) broth. The information reported in the first three columns (accession number, protein name, and abundance ratio – AR – expressed as the log2 value) was obtained by Proteome Discoverer Analysis. The last column reports biological process and molecular function information as found in the UniProtKB. The definition “other” indicates that the protein belongs to metabolic pathways different than amino acid, carbohydrate, lipid, or nucleotide metabolism
Figure 4. Distribution of the differential functions of the proteins secreted in brain-heart infusion (BHI) broth by the six Staphylococcus aureus strains evaluated in this study, classified according to the respective GT/ST. The smaller graphs illustrate the relative composition of the categories “Pathogenesis” (Orange) and “Metabolic enzyme” (blue). Abbreviations: Reg: regulation of gene expression; MDR: multidrug resistance; Amino: aminoacid metabolism; Carbo: carbohydrate metabolism; Lipid: lipid metabolism; Nucleo: nucleotide metabolism.
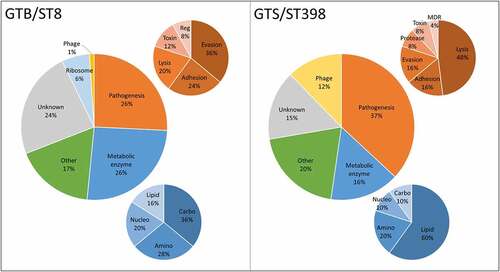
The differential extracellular virulence factors (those reporting the term “pathogenesis” in their UniProtKB entry page as reported in ) and metabolic enzymes (also according to UniProtKB as reported in ) were arranged in heatmaps for the six investigated strains according to the genotype and to the normalized protein abundance value. The virulence factor heatmap is reported in and the extracellular metabolic enzyme heatmap is reported in .
Figure 5. Heatmap of the extracellular virulence factors showing significant differences between the two GT/ST, reported in order of abundance in the respective GT/ST group. The first and second columns report the protein accession number and the protein name and acronym. The last six columns illustrate in a heat map the average normalized protein abundance value/1000 calculated for each strain with Proteome Discoverer. Color intensity ranges from the highest observed value (dark red) to the lowest observed value (dark green). White: the protein was not detected. The proteins detected only in one genotype are marked with an asterisk.
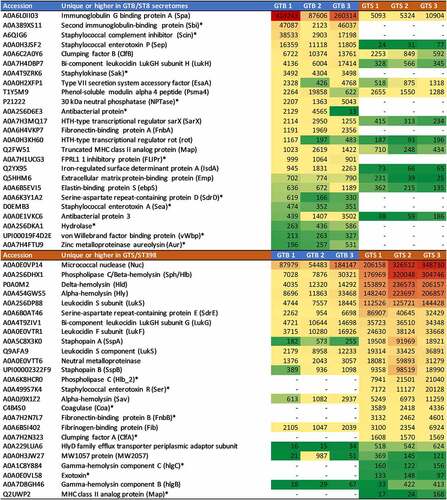
Figure 6. Heatmap of the extracellular metabolic enzymes showing significant differences between the two GT/ST, reported in order of abundance in the respective GT/ST group. The first and second columns report the protein accession number and the protein name and acronym. The third column indicates the metabolic pathway. The last six columns illustrate in a heat map the average normalized protein abundance value/1000 calculated for each strain with Proteome Discoverer. Color intensity ranges from the highest observed value (dark red) to the lowest observed value (dark green). White: the protein was not detected. The proteins detected only in one genotype are marked with an asterisk.
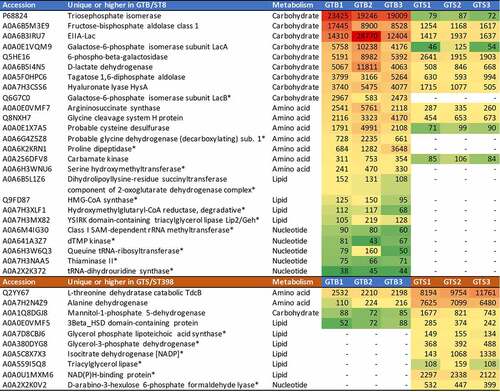
highlights the crucial differences in terms of identity and abundance of virulence factors released by the two strain groups. In GTB/ST8, the differential proteins with the highest abundances were Spa, Sbi and Scin, all mediating the evasion of innate and adaptive humoral immunity. Notably, the last two were not identified in the GTS/ST398 supernatants. On the other hand, the differential protein predominating in the GTS/ST398 supernatants was Nuc. This protein, however, was highly abundant also in the GTB/ST8 secretomes according to its normalized protein abundance value (the second in order of abundance after Spa). This was followed by leukocidins and hemolysins, mediating evasion of cellular immunity, adhesins and proteases. Another remarkable difference was the type of coagulase detected in the two genotypes, as GTB/ST8 secreted the von Willebrand factor while GTS/ST398 secreted the classical coagulase. The normalized protein abundance values of the two proteins were also quite different (about one order of magnitude).
reports the extracellular metabolic enzymes showing statistically significant differences in the two genotypes, with their respective normalized protein abundances. Metabolic enzymes were significantly more abundant in the GTB/ST8 than in the GTS/ST398 secretome (26 vs 10, respectively). Carbohydrate metabolism enzymes were the most represented, and almost all of them were consistently more abundant in the GTB/ST8 secretome (9 vs 1). The glycolytic enzyme triose phosphate isomerase was the highest, followed by another glycolytic enzyme, fructose-bisphosphate aldolase. Lactose and galactose metabolism enzymes were next. Carbohydrate metabolism enzymes were followed by aminoacid metabolism enzymes, also more represented and abundant in the GTB/ST8 secretome than in the GTS/ST398 secretome (7 vs 2). Nucleotide metabolism enzymes followed the same behavior. On the other hand, lipid metabolism enzymes were more represented and abundant in the GTS/ST398 than in the GT8/ST8 secretome (6 vs 4, respectively).
Cell viability assay
To evaluate the effect of GTB/ST8 and GTS/ST398 secretomes on cell viability, an MTT test was performed by incubating bovine PBMCs for 18 hours with the proteins secreted by the two genotypes at different concentrations. The proteins secreted in BHI by the GTS/ST398 and by the GTB/ST8 strains had a concentration of 10 and 8 µg/mL, respectively, and were used at a dilution of 0.5%, 1%, 2.5%, and 10% (). After 18 hours, the PBMCs incubated with 2.5% and 10% dilutions of the GTS/ST398 secretions showed a significant decrease in viability compared to the control (P < 0.05 and P < 0.005, respectively, calculated on six technical replicates per condition). On the other hand, no significant differences with the control were observed with the GTB/ST8 secretions up to the highest concentration tested.
Figure 7. Peripheral blood mononuclear cell (PBMC) viability after 18 h of incubation with the proteins secreted in brain-heart infusion (BHI) broth by the two Staphylococcus aureus GT (ST) evaluated in this work. The viability is expressed as fold-change compared to cells incubated without secreted bacterial proteins (control) in six technical replicates per condition. Significance was accepted at P < 0.05 (*) and P < 0.01 (**). The lines inside the boxes denote the median. The whiskers indicate the variability outside the upper and lower quartiles.
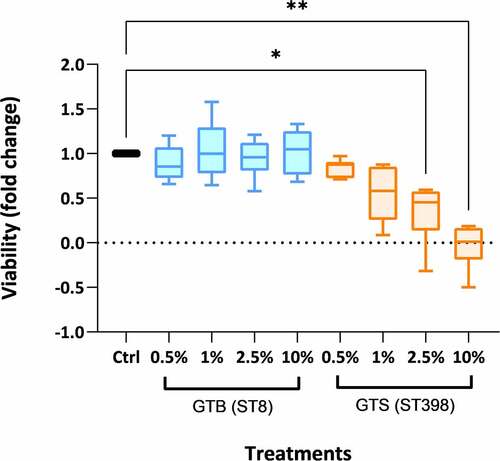
Discussion
GTB/ST8 S. aureus are associated with a very high within-herd prevalence as opposite to other genotypes including GTS/ST398 [Citation6,Citation24]. Strain diffusivity is a crucial issue in herds infected by S. aureus, because knowing the potential behavior of the strain responsible for the mastitis outbreak might prompt different intervention approaches. Moreover, the secreted proteins most associated with strain diffusivity and persistence may represent important future targets for diagnosis and prevention. Therefore, the aims of this study extend well beyond these two model GT/ST. A previous article compared the genome and transcriptome of six S. aureus strains belonging to the two genotypes to shed light on the relationships of virulence gene carriage and expression with epidemiological behavior [Citation9]. Here, we extended our investigation to the secretome by applying high-performance shotgun proteomics to further examine their virulence phenotypes. We found numerous and relevant differences, in accordance with other authors’ reports that S. aureus genotypes can have very diverse secretomes, which are related to their pathogenetic behavior [Citation16,Citation17,Citation25].
The most abundant differential proteins in the GTB/ST8 secretomes were the immunoglobulin G binding protein A (Spa), followed by the immunoglobulin-binding protein (Sbi) and the staphylococcal complement inhibitor (Scin). Spa protects S. aureus from antibody-mediated phagocytic killing with its ability to capture both the Fc and Fab region of immunoglobulins [Citation26]. The Sbi protein, a multifunctional immune evasion factor of S. aureus [Citation27], can bind IgG similarly to protein A as well as C3, promoting its unproductive consumption in the fluid phase and acting as a potent complement inhibitor of the alternative pathway-mediated lysis [Citation27]. Scin inhibits all three complement pathways: the alternative, classical, and lectin pathways [Citation26]. By inactivating C3 convertases, it blocks most complement functions including opsonization, phagocytosis, and neutrophil killing [Citation13]. The regulation appears to occur also at a transcriptomic level, as in our previous study Scin was over-expressed in GTB/ST8 and down-regulated in GTS/ST398 [Citation9].
Early expression of Scin together with chemotaxis-inhibitory proteins drives instant immune evasion [Citation28]; we detected FPRL-1, a protein of the CHIPS-FLIPr family, only in GTB/ST8. Another protein found only in GTB/ST8 was the 30 kDa neutral phosphatase, a highly cationic enzyme capable of binding immunoglobulins and serum albumin. S. aureus is also equipped with virulence factors that target complement without direct binding to C3 convertase. Staphylokinase (Sak) was detected only in the GTB/ST8 secretome. Sak is a secreted protein that binds plasminogen converting it into its active form plasmin [Citation29]. Plasmin, a serine protease, is bound externally to S. aureus and degrades C3 convertase-dependent C3b to prevent deposition on the bacterial surface. In light of the limited functionality of the classical pathway in milk [Citation30], the ability of GTB/ST8 to target both the adaptive and innate arms of the complement may represent an advantage in terms of immune evasion capabilities in the mammary gland.
Another crucial defense of the mammary gland against intramammary infection are antimicrobial peptides and proteins, including defensins and cathelicidins [Citation31,Citation32], released by both neutrophils and mammary epithelial cells [Citation33,Citation34]. In response, S. aureus secretes many proteins aimed at neutralizing them. Sak and aureolysin (Aur) have essential roles in binding defensin peptides and in cleaving and inactivating cathelicidins, respectively [Citation35]. Notably, cathelicidins are among the few antibacterial peptides with potent anti-staphylococcal activity; thus, S. aureus strains producing these two proteins are significantly more resistant to cathelicidins than Aur-negative strains [Citation36]. In our study, both Sak and Aur were found uniquely in the secretome of GTB/ST8 strains. The iron-regulated surface determinant protein A (IsdA) was also higher in their secretome. IsdA is a cell wall-anchored surface receptor that protects S. aureus against the bactericidal protease activity of apolactoferrin and bovine lactoferricin, relevant host defense mechanisms against bacterial infection in milk [Citation37]. IsdA also plays a crucial role in immune evasion by enhancing bacterial cellular hydrophobicity, thereby increasing the resistance of S. aureus to beta-defensins and cathelicidins [Citation35]. The presence of redundant mechanisms aimed at degrading and neutralizing antimicrobial peptides and proteins translates into a significant advantage to GTB/ST8 in terms of persistence in the mammary gland.
Metabolic enzymes, especially those belonging to the carbohydrate metabolism, were significantly more abundant in the secretome of GTB/ST8 strains. Enzymes degrading lactose and galactose may advantage bacterial growth in milk; extracellular metabolic enzymes, however, can have a quite more relevant role in the host/bacterium relationship. Mekonnen and coworkers [Citation38] observed that signatures of cytoplasmic proteins in the secretome represent a distinguishing feature of genotypes with different epidemiologic behavior and intracellular survival capabilities. According to these authors, cytoplasmic proteins liberated in the extracellular milieu contribute substantially to staphylococcal virulence by playing moonlighting and immune evasion functions [Citation39]. The concept of moonlighting proteins is one in which a single protein with alternative oligomeric conformations can carry out different functions when located inside or outside the cell. Several authors now agree that moonlighting proteins are crucial for S. aureus pathogenicity [Citation40]. Further, several moonlighting proteins can play multiple roles in different infection stages, thus enhancing the virulence of the bacterium [Citation40]. A relevant advantage of moonlighting proteins is their better ability to hide from the host immune system, as these typically exhibit high structural conservation toward their host counterparts. Accordingly, proteins involved in critical metabolic pathways and ancestral processes, including ribosomal proteins, molecular chaperones, and glycolytic enzymes, typically exhibit moonlighting activities. In line with this, we also detected several ribosomal proteins only in the GTB/ST8 secretome. The relevance of carbohydrate metabolism enzymes in moonlighting and immune evasion is highlighted by their presence in the secretome of both genotypes, albeit at consistently higher levels in GTB/ST8 than GTS/ST398.
On the other hand, almost all the hemolysins and leukocidins detected in this work were significantly more abundant in the GTS/ST398 secretome, in agreement with the previous genomic and transcriptomic characterization of the same strains [Citation9]. These included alpha, delta, beta, and gamma hemolysins, and various leukocidins. All these proteins target and kill leukocytes, the primary cellular defense of the mammary gland, by destabilizing their membrane or by forming pores leading to osmotic lysis [Citation41]. In addition, many hemolysins and pore-forming proteins also exploit cellular pathways to enhance cell killing, including the inflammasome pathway. For example, staphylococcal leukocidins activate the NOD-, LRR- and pyrin domain-containing protein 3 (NLRP3) inflammasome in macrophages and monocytes, potentiating lysis and leading to pyroptosis with enhanced production of pro-inflammatory cytokines [Citation41].
The associations between a higher abundance of cytotoxic proteins [including hemolysins and leukocidins) in GTS/ST398 and a higher cytotoxic/hemolytic potential were validated with an in vitro viability assay on bovine PBMCs. In fact, contrasting transcriptomic data had been obtained by Capra and coworkers [Citation9], who hypothesized a higher potential cytotoxicity of GTB/ST8 strains. In addition, it was shown earlier [Citation42] that alpha-hemolysin could be degraded by staphopain A (SspA), both proteins found in large amounts in GTS/ST398. Thus, the net effect could not be extrapolated even under the used growth conditions in BHI, and it was experimentally validated. The PBMC viability assay carried out in this work clearly showed that the observed protein abundance differences do indeed translate into a higher cytotoxic potential of the GTS/ST398 strains when compared to the GTB/ST8 strains. Strikingly, the latter did not produce visible effects on cell viability even after 18 h of incubation at the highest tested concentration.
Bi-component leukocidins consist of two separately secreted components, named S and F based on their elution by cationic exchange chromatography (slow vs fast, respectively) [Citation41]. The binding of S component to the cell membrane is required for the secondary binding of F component, leading to the formation of membrane pores and cellular lysis [Citation43]. In the LukGH (or LukAB), LukG is the S component, while LukH is the F component. LukH was the only leukocidin that increased in the GTB/ST8 secretomes. Accordingly, the higher abundance of LukH in GTB/ST8 should not increase the cytotoxic capabilities of this genotype, while in GTS/ST398 LukG may engage the LukF component to form heterocomplexes. All this considered, cows infected by strains behaving as GTS/ST398 might be at higher risk for developing clinical mastitis.
The GTS/ST398 secretomes were also significantly higher in extracellular proteases, including SspA and SspB and the neutral metalloprotease. Staphopains are proteases with broad specificity implicated in tissue colonization and connective tissue destruction and may act as an immune evasion factor by cleaving immunoglobulins and complement components. Staphopain A is required for proteolytic maturation of SspB, and both are involved in the inhibition of neutrophil recruitment and activation. Staphopain B also bocks phagocytosis of opsonized S. aureus by neutrophils and monocytes by inducing their death in a proteolytic activity-dependent manner [Citation44,Citation45]. Higher expression of staphopains in GTS/ST398 strains was also detected by transcriptomics [Citation9]. However, it should be noted that a high amount of SspA (as found in GTS/ST398) does not necessarily correlate with higher proteolytic activity, since the zymogen SspA needs to be exclusively activated by Aur [Citation46,Citation47], which was found in much higher amounts in GTB/ST8. Thus, even though GTB/ST8 strains exhibit lower amounts of SspA, substantial activation by Aur could finally lead to a higher proteolytic activity of GTB/ST8 compared to GTS/ST398.
One of the most abundant proteins found in the secretome of both genotypes, with significantly higher amounts in GTS/ST398, was the micrococcal nuclease (Nuc). This enzyme facilitates bacterial escape from neutrophil extracellular traps (NETs) [Citation48,Citation49]. NETs are structures composed of DNA, histones, and antimicrobial proteins that are released extracellularly by neutrophils as a means for trapping and killing invading pathogens [Citation50]. NETs represent a crucial defense against mammary gland pathogens [Citation22,Citation51], and the finding of Nuc as one of the most abundant secreted proteins in both genotypes underlines its relevance for bacterial virulence. Leukocidins can potentiate NET formation, exacerbating the inflammatory response [Citation52] and favoring the onset of clinical mastitis. Furthermore, fibronectin-binding proteins (Fnb) A was higher in GTB/ST8, while FnbB was higher in GTS/ST398. Fnbs are cell wall proteins possessing fibronectin, fibrinogen, and elastin-binding regions, but FnbB also confers resistance to the bactericidal activity of NETs. Intriguingly, in some specific contexts or tissues S. aureus and other pathogens may benefit more from inducing NETs and using them to damage host tissues, together with secreted proteases, than from blocking or avoiding their activation [Citation49,Citation53,Citation54]. This strategy can provide better access to metabolic resources, favor deeper tissue colonization, and ensure safer and optimal survival, and might enable GTS/ST398 strains to colonize different tissues and hosts. Conversely, in the case of GTB/ST8 strains, evasion of the immune response through inflammation-dampening mechanisms including moonlighting and molecular mimicry, Ig binding, complement inhibition and antimicrobial peptide neutralization, might represent a better “escape strategy” in the specific context of the mammary gland, enabling these strains to establish chronic, subclinical infections, and infect a higher number of animals in the herd. As type 3 immunity is probably the most relevant defense mechanism in the mammary gland [Citation55], a higher ability to avoid inflammation might translate into better chances for maintaining and spreading infection.
Strikingly, the staphylococcal coagulase was detected only in GTS/ST398 secretions. Coagulase (Coa) is an enzyme that specifically forms a complex with prothrombin and can clot fibrinogen without any proteolytic cleavage. The ability to clot fibrinogen is so typical that it is used in the microbiology laboratory to discriminate S. aureus from almost all other staphylococcal species, and it constitutes a crucial immune evasion strategy of this pathogen [Citation35]. Yet, plasma coagulation can be mediated by other S. aureus proteins, including the von Willebrand factor binding protein (vWbp) and Sak [Citation56] detected only in GTB/ST8. Therefore, we might speculate that proteins with different coagulase properties released by the GTB/ST8 strains, together with other proteins or processes to be identified, may render them more capable of acting on the high-abundance proteins typical of this host niche, in which fibrinogen and plasminogen are scarce but milk whey proteins are largely available. The differential abundances of clumping factor A (higher in GTS/ST398) and clumping factor B (higher in GTB/ST8) may also have a role in their adaptation to different host niches, as their binding specificities differ [Citation57]. Dedicated biochemical studies will be required to clarify these aspects. Notably, however, all GTB/ST8 strains coagulated milk whey proteins while growing in this medium, while GTS/ST398 did not.
Enterotoxins also showed relevant differences. Enterotoxin Sep was more abundant, and enterotoxin Sea was uniquely found in GTB/ST8 secretions. On the other hand, enterotoxin Ser was found uniquely in GTS secretions. Both observations were in line with genomic and transcriptomic studies [Citation9]. Acting as superantigens in the mammary gland, enterotoxins massively activate T lymphocytes and antigen-presenting cells, interfering with the generation of a proper adaptive immune response [Citation35].
MekonnenCitation38 observed that distinctive features in the bacterial secretomes associated with virulence were related primarily to the accessory genome. Phage-encoded proteins predominated in GTS/ST398 secretomes, reinforcing the notion that these mobile genetic elements play a relevant role in modulating S. aureus pathogenesis [Citation58].
Major differences in the composition of S. aureus proteomes are related to differences in transcriptional regulation by the agr system, resulting in the expression of diverse secreted virulence factors [Citation17]. Indeed, the comparative secretome analyses of dominant human- or livestock-associated lineages of ST8 and ST398 revealed that specific virulence factors are differentially secreted because of regulatory differences linked to agr activities [Citation16]. In this study, we found significantly higher amounts of the HTH-type transcriptional regulator SarX in GTB strains. This protein is involved in the regulation of virulence genes by binding directly to the agr promoter region and acting as a repressor of the agr locus. It consequently targets the genes regulated by the agr system such as sspA, hla and hlb. Furthermore, the HTH-type transcriptional regulator rot was also higher in GTB. This is a global regulator with both positive and negative effects that mediates modulation of several genes involved in virulence. GTB/ST8 showed a higher expression of Target of RNAIII activating Protein (TRAP) that leads to the activation of agr [Citation9]. Accordingly, the phenotypic differences seen in the two secretomes could be related to the differential expression of these critical transcriptional regulators that might be crucial in host adaptation. However, this will need to be investigated further with dedicated molecular approaches.
However, a limitation of this study lies in the generation of secreted proteins through bacterial culture in a conventional laboratory medium. We analyzed the secretome at 3.5 h of culture, in the exponential growth phase, but other secretome differences may emerge if analyzed at other time points. Adding to this, the presence of milk proteins and bacterial inhibitors, the interaction with host cells, and other stimuli provided by the in vivo environment, including other staphylococcal species, might influence the nature and relative levels of secreted proteins. Yet, applying proteomics to complex fluids such as milk or milk whey still poses tremendous challenges related to the massive amounts of caseins and high-abundance whey proteins that would severely hamper the detection of secreted S. aureus proteins. On the other hand, cell culture models introduce variables associated with the presence of eukaryotic cell proteins, other components of the growth medium, and bacterial internalization or cell invasion. This notwithstanding, further in vitro and in vivo investigations will be crucial for understanding the role of the bacterial secretome in host-pathogen interactions. Furthermore, strain-specific differences within a genotype can be present that will need to be considered.
Final considerations and conclusion
The transcriptomic comparison of the strains investigated here revealed the functional enrichment of genes related to adaptation and chronicity in GTB/ST8 versus GTS/ST398 S. aureus [Citation9]. In the present study, we confirmed and expanded those findings by observing the preferential release by GTB/ST8 of virulence factors favoring the establishment of chronic, subclinical infections with immune-dampening activities and a higher ability to evade both innate and adaptive humoral responses, versus a higher propensity of GTS/ST398 for establishing acute infections with pro-inflammatory activities, neutrophil killing, NETosis, and pyroptosis. We observed significant differences in the expression and secretion of crucial virulence genes present in both genotypes, such as leukocidins, hemolysins, proteases, complement-binding and immunoglobulin-binding proteins, as well as metabolic enzymes. Therefore, investigating gene carriage alone, although crucial for understanding strain circulation and virulence potential, is likely not sufficient for establishing meaningful correlations with the epidemiological behavior or clinical severity of a particular strain. As recently concluded in a large study investigating the correlation of virulence gene carriage with the clinical outcome, it is differential gene expression (and secretion) rather than gene carriage that affects the clinical presentation of IMI [Citation24]. Accordingly, investigating in more detail the secreted virulence factor characteristics will also help to unravel some controversial aspects concerning the role of cytotoxicity in S. aureus pathogenicity in bovine mastitis in general, and for GTS/ST398 in particular, as conflicting results are published [Citation3,Citation6,Citation59–62].
Such an impressive heterogeneity in the secretome of the two investigated S. aureus genotypes requires consideration also for its implications for mastitis control and prevention. So far, the development of an effective vaccine for S. aureus mastitis has encountered many difficulties. As nicely discussed recently by de Jong and coworkers [Citation26], this can be attributed to several factors, including the extreme variability of the bacterial surfaceome and exoproteome. Accordingly, vaccine strategies implementing single antigens without adjuvant have not been successful in providing protection. Future vaccine efforts should incorporate a combination of proteins, including evasion molecules, and raise antibodies against them. Nevertheless, the redundancy and multiplicity of immune evasion strategies, as clearly emerged also in this study, remains a challenge [Citation26]. We will also need to understand in better detail the roles of humoral immunity, innate immunity, tolerance, type 3 immunity, and the microbiota in the mammary gland response to infection, as well as the complex kinetics and interactions of immune evasion factors with host factors [Citation55,Citation63,Citation64,Citation65–66]. Still, understanding the complexities in the S. aureus secretome will be a crucial step toward this goal.
Data availability
The mass spectrometry proteomics data have been deposited to the ProteomeXchange Consortium [65] via the PRIDE partner repository [66] with the dataset identifier PXD029571.
Author contributions
Conceptualization: MFA, RP, PC, BC, ST. Study coordination: MFA, RP. Collection of samples, PC, BC, RP, JF. Bacterial culture and phenotypic analysis: VM, AG, MP, RP. Proteomic analysis: MFA, MP, SP, DP. Cell viability assays: SD, JF. Data curation and visualization: MFA, SP, VM, JF. Writing - original draft: MFA, VM, AG, RP, JF. Writing - review & editing: All authors.
Disclosure statement
No potential conflict of interest was reported by the author(s).
Additional information
Funding
References
- Cosandey A, Boss R, Luini M, et al. Staphylococcus aureus genotype B and other genotypes isolated from cow milk in European countries. J Dairy Sci. 2016;99:529–540.
- Gazzola A, Maisano AM, Bianchini V, et al. Short communication: characterization of Staphylococcus aureus from bulk tank milk of dairy cattle in Lombardy (northern Italy). J Dairy Sci. 2020;103:2685–2692.
- Graber HU, Naskova J, Studer E, et al. Mastitis-related subtypes of bovine Staphylococcus aureus are characterized by different clinical properties. J Dairy Sci. 2009;92:1442–1451.
- Magro G, Biffani S, Minozzi G, et al. Virulence genes of Staphylococcus aureus from dairy cow mastitis and contagiousness risk. Toxins (Basel). 2017;9:195.
- Sakwinska O, Giddey M, Moreillon M, et al. Staphylococcus aureus host range and human-bovine host shift. Appl Environ Microbiol. 2011;77:5908–5915.
- Cremonesi P, Pozzi F, Raschetti M, et al. Genomic characteristics of Staphylococcus aureus strains associated with high within-herd prevalence of intramammary infections in dairy cows. J Dairy Sci. 2015;98:6828–6838.
- Fournier C, Kuhnert P, Frey J, et al. Bovine Staphylococcus aureus: association of virulence genes, genotypes and clinical outcome. Res Vet Sci. 2008;85:439–448.
- McCarthy AJ, Lindsay JA, Loeffler A. Are all meticillin-resistant Staphylococcus aureus (MRSA) equal in all hosts? Epidemiological and genetic comparison between animal and human MRSA. Vet Dermatol. 2012;23:267–e54.
- Capra E, Cremonesi P, Pietrelli A, et al. Genomic and transcriptomic comparison between Staphylococcus aureus strains associated with high and low within herd prevalence of intra-mammary infection. BMC Microbiol. 2017;17:1–16.
- Foster TJ, Geoghegan JA, Ganesh VK, et al. Adhesion, invasion and evasion: the many functions of the surface proteins of Staphylococcus aureus. Nat Rev Microbiol. 2014;12(1):49–62.
- Geoghegan JA, Foster TJ. Cell wall-anchored surface proteins of Staphylococcus aureus: many proteins, multiple functions. In: Current topics in microbiology and immunology, 409. Cham: Springer; 2015. p. 95–120. DOI:10.1007/82_2015_5002
- Brouillette E, Talbot BG, Malouin F. The fibronectin-binding proteins of Staphylococcus aureus may promote mammary gland colonization in a lactating mouse model of mastitis. Infect Immun. 2003;71:2292–2295.
- Rooijakkers SHM, Ruyken M, Roos A, et al. Immune evasion by a staphylococcal complement inhibitor that acts on C3 convertases. Nat Immunol. 2005;6:920–927.
- Balaban N, Goldkorn T, Gov Y, et al. Regulation of Staphylococcus aureus pathogenesis via target of RNAIII-activating protein (TRAP). J Biol Chem. 2001;276:2658–2667.
- Korem M, Gov Y, Kiran MD, et al. Transcriptional profiling of target of RNAIII-activating protein, a master regulator of staphylococcal virulence. Infect Immun. 2005;73:6220–6228.
- Busche T, Hillion M, Van Loi V, et al. Comparative secretome analyses of human and zoonotic Staphylococcus aureus isolates CC8, CC22, and CC398. Mol Cell Proteomics. 2018;17:2412–2433.
- Ziebandt AK, Kusch H, Degner M, et al. Proteomics uncovers extreme heterogeneity in the Staphylococcus aureus exoproteome due to genomic plasticity and variant gene regulation. Proteomics. 2010;10:1634–1644.
- Kusch H, Engelmann S. Secrets of the secretome in Staphylococcus aureus. Int J Med Microbiol. 2014;304:133–141.
- Zhao X, Palma Medina LM, Stobernack T, et al. Exoproteome heterogeneity among closely related Staphylococcus aureus t437 isolates and possible implications for virulence. J Proteome Res. 2019;18:2859–2874.
- Le Maréchal C, Seyffert N, Jardin J, et al. Molecular basis of virulence in Staphylococcus aureus mastitis. PLoS One. 2011;6(11):e27354.
- Addis MF, Maffioli EM, Ceciliani F, et al. Influence of subclinical mastitis and intramammary infection by coagulase-negative staphylococci on the cow milk peptidome. J Proteomics. 2020;226: DOI:10.1016/j.jprot.2020.103885.
- Pisanu S, Cubeddu T, Pagnozzi D, et al. Neutrophil extracellular traps in sheep mastitis. Vet Res. 2015;46:59.
- Ceciliani F, Ávila Morales G, De Matteis G, et al. Methods in isolation and characterization of bovine monocytes and macrophages. Methods. 2021;186:22–41.
- Hoekstra J, Zomer AL, Rutten VPMG, et al. Genomic analysis of European bovine Staphylococcus aureus from clinical versus subclinical mastitis. Sci Rep. 2020;10:18172.
- Zhao X, Chlebowicz-Flissikowska MA, Wang M, et al. Exoproteomic profiling uncovers critical determinants for virulence of livestock-associated and human-originated Staphylococcus aureus ST398 strains. Virulence. 2020;11:947–963.
- de Jong NWM, van Kessel KPM, van Strijp JAG. Immune evasion by Staphylococcus aureus. Microbiol Spectr. 2019;7: DOI:10.1128/microbiolspec.gpp3-0061-2019.
- Smith EJ, Visai L, Kerrigan SW, et al. The Sbi protein is a multifunctional immune evasion factor of Staphylococcus aureus. Infect Immun. 2011;79:3801–3809.
- Rooijakkers SHM, Ruyken M, van Roon J, et al. Early expression of SCIN and CHIPS drives instant immune evasion by Staphylococcus aureus. Cell Microbiol. 2006;8:1282–1293.
- Guerra FE, Borgogna TR, Patel DM, et al. Epic immune battles of history: Neutrophils vs. Staphylococcus aureus. Front Cell Infect Microbiol. 2017. DOI:10.3389/fcimb.2017.00286
- Rainard P. The complement in milk and defense of the bovine mammary gland against infections. Vet Res. 2003;34:647–670.
- Tomasinsig L, De Conti G, Skerlavaj B, et al. Broad-spectrum activity against bacterial mastitis pathogens and activation of mammary epithelial cells support a protective role of neutrophil cathelicidins in bovine mastitis. Infect Immun. 2010;78:1781–1788.
- Zanetti M. The role of cathelicidins in the innate host defenses of mammals. Curr Issues Mol Biol. 2005;7:179–196.
- Cubeddu T, Cacciotto C, Pisanu S, et al. Cathelicidin production and release by mammary epithelial cells during infectious mastitis. Vet Immunol Immunopathol. 2017;189:66–70.
- Wheeler TT, Smolenski GA, Harris DP, et al. Host-defence-related proteins in cows’ milk. Animal. 2012;6:415–422.
- Foster TJ. Immune evasion by staphylococci. Nat Rev Microbiol. 2005;3(12):948–958.
- Sieprawska-Lupa M, Mydel P, Krawczyk K, et al. Degradation of human antimicrobial peptide LL-37 by Staphylococcus aureus-derived proteinases. Antimicrob Agents Chemother. 2004;48:4673–4679.
- Nasser A, Moradi M, Jazireian P, et al. Staphylococcus aureus versus neutrophil: scrutiny of ancient combat. Microb Pathog. 2019;131:259–269.
- Mekonnen SA, Palma Medina LM, Glasner C, et al. Signatures of cytoplasmic proteins in the exoproteome distinguish community- and hospital-associated methicillin-resistant Staphylococcus aureus USA300 lineages. Virulence. 2017;8:891–907.
- Dreisbach A, Hempel K, Buist G, et al. Profiling the surfacome of Staphylococcus aureus. Proteomics. 2010;10:3082–3096.
- Hemmadi V, Biswas M. An overview of moonlighting proteins in Staphylococcus aureus infection. Arch Microbiol. 2021;203(2):481–498.
- Spaan AN, Van Strijp JAG, Torres VJ. Leukocidins: staphylococcal bi-component pore-forming toxins find their receptors. Nat Rev Microbiol. 2017;15(7):435–447.
- Kwak Y-K, Högbom M, Colque-Navarro P, et al. Biological relevance of natural α-toxin fragments from Staphylococcus aureus. J Membr Biol. 2010 ;233(1):93–103.
- Colin DA, Mazurier I, Sire S, et al. Interaction of the two components of leukocidin from Staphylococcus aureus with human polymorphonuclear leukocyte membranes: sequential binding and subsequent activation. Infect Immun. 1994;62:3184–3188.
- Elmwall J, Kwiecinski J, Na M, et al. Galectin-3 is a target for proteases involved in the virulence of Staphylococcus aureus. Infect Immun. 2017;85: DOI:10.1128/IAI.00177-17.
- Laarman AJ, Mijnheer G, Mootz JM, et al. Staphylococcus aureus Staphopain A inhibits CXCR2-dependent neutrophil activation and chemotaxis. EMBO J. 2012;31:3607–3619.
- Massimi I, Park E, Rice K, et al. Identification of a novel maturation mechanism and restricted substrate specificity for the SspB cysteine protease of Staphylococcus aureus. J Biol Chem. 2002;277:41770–41777.
- Shaw L, Golonka E, Potempa J, et al. The role and regulation of the extracellular proteases of Staphylococcus aureus. Microbiology. 2004;150:217–228.
- Berends ETM, Horswill AR, Haste NM, et al. Nuclease expression by Staphylococcus aureus facilitates escape from neutrophil extracellular traps. J Innate Immun. 2010;2:576–586.
- Speziale P, Pietrocola G. Staphylococcus aureus induces neutrophil extracellular traps (NETs) and neutralizes their bactericidal potential. Comput Struct Biotechnol J. 2021;19:3451–3457.
- Brinkmann V, Reichard U, Goosmann C, et al. Neutrophil extracellular traps kill bacteria. Science. 2004;303:1532–1535.
- Lippolis JD, Reinhardt TA, Goff JP, et al. Neutrophil extracellular trap formation by bovine neutrophils is not inhibited by milk. Vet Immunol Immunopathol. 2006;113:248–255.
- Malachowa N, Kobayashi SD, Freedman B, et al. Staphylococcus aureus leukotoxin GH promotes formation of neutrophil extracellular traps. J Immunol. 2013;191:6022–6029.
- Archer N, Egan SA, Coffey TJ, et al. A paradox in bacterial pathogenesis: activation of the local macrophage inflammasome is required for virulence of Streptococcus uberis. Pathogens. 2020;9:1–23.
- Cacciotto C, Cubeddu T, Addis MF, et al. Mycoplasma lipoproteins are major determinants of neutrophil extracellular trap formation. Cell Microbiol. 2016;18:1751–1762.
- Rainard P, Gilbert FB, Germon P, et al. Invited review: a critical appraisal of mastitis vaccines for dairy cows. J Dairy Sci. 2021;104(10):10427–10448.
- Thomas S, Liu W, Arora S, et al. the complex fibrinogen interactions of the Staphylococcus aureus coagulases. Front Cell Infect Microbiol. 2019;9:106.
- Esteban J, Pérez-Tanoira R, Pérez-Jorge-Peremarch C, Gómez-Barrena, E. Bacterial adherence to biomaterials used in surgical procedures. Microbiol Surg Infect Diagnosis, Progn Treat. 2014;41–57. DOI:10.1016/B978-0-12-411629-0.00003-9
- Verkaik NJ, Benard M, Boelens HA, et al. Immune evasion cluster-positive bacteriophages are highly prevalent among human Staphylococcus aureus strains, but they are not essential in the first stages of nasal colonization. Clin Microbiol Infect. 2011;17:343–348.
- Bramley AJ, Patel AH, O’Reilly M, et al. Roles of alpha-toxin and beta-toxin in virulence of Staphylococcus aureus for the mouse mammary gland. Infect Immun. 1989;57:2489–2494.
- Grunert T, Stessl B, Wolf F, et al. Distinct phenotypic traits of Staphylococcus aureus are associated with persistent, contagious bovine intramammary infections. Sci Rep. 2018;8:15968.
- Mayer K, Kucklick M, Marbach H, et al. Within-host adaptation of iStaphylococcus aureus in a bovine mastitis infection is associated with increased cytotoxicity. Int J Mol Sci. 2021;22:8840 22, 8840.
- Monecke S, Müller E, Büchler J, et al. Staphylococcus aureus in vitro secretion of alpha toxin (hla) correlates with the affiliation to clonal complexes. PLoS One. 2014;9:e100427.
- Amadori M. Control of bovine mastitis in the 21st century: immunize or tolerize? Res Vet Sci. 2018;121:1–3.
- Rainard P, Foucras G, Fitzgerald JR, et al. Knowledge gaps and research priorities in Staphylococcus aureus mastitis control. Transbound Emerg Dis. 2018;65:149–165.
- Deutsch EW, Bandeira N, Sharma V, et al. The ProteomeXchange consortium in 2020: enabling “big data” approaches in proteomics. Nucleic Acids Res. 2020;48:D1145–D1152.
- Perez-Riverol Y, Csordas A, Bai J, et al. The PRIDE database and related tools and resources in 2019: improving support for quantification data. Nucleic Acids Res. 2019;47:D442–D450.