ABSTRACT
Chronic implant-related bone infections are a severe complication in orthopaedic surgery. Biofilm formation on the implant impairs the immune response, leading to bacterial persistence. In a previous study, we found that Staphylococcus aureus (SA) induced interferon regulatory factor 3 (IRF3) activation and Ifnb expression only in its planktonic form but not in the biofilm. The aim of this study was to clarify the role of the stimulator of interferon genes (STING) in this process. We treated RAW 264.7 macrophages with conditioned media (CM) generated from planktonic or biofilm cultured SA in combination with agonists or inhibitors of the cyclic GMP-AMP synthase (cGAS)/STING pathway. We further evaluated bacterial gene expression of planktonic and biofilm SA to identify potential mediators. STING inhibition resulted in the loss of IRF3 activation and Ifnb induction in SA planktonic CM, whereas STING activation induced an IRF3 dependent IFN-β response in SA biofilm CM. The expression levels of virulence-associated genes decreased during biofilm formation, but genes associated with cyclic dinucleotide (CDN) synthesis did not correlate with Ifnb induction. We further observed that cGAS contributed to Ifnb induction by SA planktonic CM, although cGAS activation was not sufficient to induce Ifnb expression in SA biofilm CM. Our data indicate that the different degrees of virulence associated with SA planktonic and biofilm environments result in an altered induction of the IRF3 mediated IFN-β response via the STING pathway. This finding suggests that the STING/IRF3/IFN-β axis is a potential candidate as an immunotherapeutic target for implant-related bone infections.
Introduction
The chronicity of implant-related bone infections is associated with biofilm formation on implant surfaces. Staphylococci are the most common cause of implant-associated biofilm formation. Within such biofilms, bacteria are protected against most antibiotics and host defence mechanisms [Citation1,Citation2]. Additionally, the biofilm environment has been shown to shift the immune response towards tolerance, which further promotes bacterial persistence [Citation2,Citation3]. This can be caused by the increased metabolic activity of the biofilm bacteria, leading to glucose deprivation and lactate accumulation in the local environment, as well as through the adaption of gene expression in biofilm bacteria, resulting in a reduced production of immunogenic and toxic factors [Citation4,Citation5].
We recently showed that the presence of bacterial factors is more important for the differential activation of macrophages in planktonic and biofilm environments, respectively, than glucose and lactate levels [Citation6,Citation7]. Additionally, we found that stimulation with a Staphylococcus aureus (SA) planktonic environment leads to the activation of interferon regulatory factor 3 (IRF3) and a subsequent induction of interferon beta gene (Ifnb), which is absent in the respective biofilm environment [Citation6]. Together with IRF7, IRF3 contributes to the production of type I IFNs. Both IRFs are activated by phosphorylation which can occur downstream of Toll-like receptors (TLRs) and cytosolic pattern recognition receptors (PRRs) [Citation8]. In our model, activation of TLR-2 and -9 led to IRF7 activation but did not mediate the observed IRF3 phosphorylation. Thus, the underlying mechanism of SA-triggered IRF3 phosphorylation still remains unclear. The cytosolic PRRs retinoic-acid-inducible gene I (RIG-I) and melanoma-differentiation-associated gene 5 (MDA5) detect RNA and lead to IRF3/7 phosphorylation via activation of mitochondrial antiviral-signalling protein (MAVS) and are known to play an important role in antiviral immunity [Citation9]. The stimulator of interferon genes (STING) is another cytosolic sensor molecule and gets activated by cyclic dinucleotides (CDNs) leading to IRF3 phosphorylation and IFN-β production [Citation10,Citation11]. CDNs can either be of endogenous or foreign origin. The cyclic dinucleotides, c-di-AMP, c-di-GMP and 3’3’-cGAMP are produced by bacteria and can directly interact with STING [Citation12,Citation13]. CDN synthesis is of essential importance for the survival of bacteria as these molecules serve as secondary messengers to maintain bacterial homoeostasis, and to allow environmental adaptation, biofilm formation, and virulence [Citation14]. Especially, c-di-GMP became an interesting candidate in biofilm research as it is closely related with planktonic-to-biofilm transition and biofilm dispersal, respectively. The importance of these processes makes c-di-GMP an attractive target for biofilm treatment [Citation15,Citation16].
2’3’-cGAMP is a eukaryotic CDN produced by the cyclic GMP-AMP synthase (cGAS) in response to cytosolic DNA and acts as an endogenous ligand for STING [Citation17,Citation18]. Cytosolic DNA can either be of microbial origin or released from the mitochondria or the nucleus upon cell damage [Citation19]. Downstream of STING, TANK-binding kinase 1 (TBK1)-dependent phosphorylation leads to the activation of IRF3 and subsequent induction of type I interferon gene expression [Citation20]. Furthermore, STING can activate the canonical NF-κB pathway and inflammation-associated gene expression [Citation11,Citation21]. The STING pathway has been investigated in cancer, autoimmune disorders, and infections [Citation22] and is of high therapeutic interest as a potential target for immunomodulatory approaches [Citation23,Citation24]. In addition, the contribution of STING to host defence mechanisms against SA infections has been previously described [Citation25]. However, the deletion of STING showed varying effects on the host defence against SA between different infection models [Citation26–28]. Biofilm formation is associated with anti-inflammation and tolerance and is a main cause for infection chronicity [Citation2,Citation29]. Biofilm-derived lactate, which accumulates in the local biofilm environment seems to be an important driver of the anti-inflammatory immune response [Citation30]. Additionally, direct activation of STING by c-di-AMP released from biofilm SA and subsequent IFN-β production were shown to be connected to anti-inflammatory macrophage polarization and bacterial persistence [Citation31]. Nevertheless, the role of STING in the adaptation of the immune response during the transition from an acute to chronic infection associated with biofilm formation is unclear, and a direct comparison of the impact of STING activation on the immune response against planktonic or biofilm SA is lacking.
The aim of our study was to investigate whether STING plays a role in the IRF3 mediated induction of Ifnb gene expression in an SA planktonic environment and why this response cannot be detected in the respective biofilm environment. Therefore, we stimulated RAW 264.7 cells with conditioned media (CM) from SA planktonic and biofilm cultures in the presence of the STING inhibitor H-151 or STING ligand 3’3’-cGAMP, respectively, and evaluated IRF3 phosphorylation as indicator for STING pathway activation, subsequent Ifnb induction, and downstream IFN-β signalling and target gene expression. We further compared the bacterial gene expression levels of CDN synthases, quorum sensing (QS) molecules, and virulence-associated factors between planktonic and biofilm-cultured SA. Additionally, we investigated the contribution of cGAS to Ifnb induction and whether the difference in Ifnb induction between planktonic or biofilm CM was conserved between different SA strains.
Materials and methods
Bacteria culture and preparation of conditioned media
Staphylococcus aureus strain ATCC 49230 (UAMS-1, isolated from a patient with chronic osteomyelitis) [Citation32] was used to prepare conditioned media (CM) as described in a previous study [Citation6]. Bacteria were cultured on Columbia agar plates containing 5% sheep blood (BD Biosciences). For CM preparation, three to five colonies were transferred into trypticase soy bouillon (TSB; BD, Germany) and grown for 3 h at 37°C under shaking to obtain log phase bacteria. 6 × 105 CFU/ml were cultivated in Dulbecco’s Modified Eagle Medium (DMEM) high glucose (Anprotec, Germany) and 10% heat-inactivated foetal calf serum (FCS; PAN-Biotech, Germany) at 37°C and 5% CO2 either under continuous shaking (200 rpm) for planktonic culture or under static conditions in 24 well plates for biofilm culture. Biofilms were grown for 6 days with daily medium exchange. Medium was harvested after 24 h of planktonic culture or the last 24 h medium change of day 6 biofilm culture by centrifugation at 4000 rpm for 15 min at 4°C. 10 µl of medium was streaked on agar plates and incubated overnight to exclude contamination with other bacteria. Before transfer in cell culture, CM were sterile filtered (0.2 µm filter) and pH adjusted to physiological pH of growth medium. CM aliquots were stored at −80°C to avoid repeated freezing and thawing cycles. Growth medium (DMEM high glucose and 10% FCS) of the respective approach was treated similarly to CM but without bacterial inoculation and included in the experiments as the unstimulated medium control (Medium, Med.). For comparison of SA strains, USA300 FPR3757, ATCC 25923, and SA113 were included. The CM from these strains was prepared as described above. Biofilm CM was collected on days 1, 3, and 6 of biofilm formation.
Cell culture and stimulation of macrophages
The murine macrophage cell line RAW 264.7 (ATCC TIB-71, USA) was used for the experiments [Citation33]. Cells were cultivated in DMEM high glucose supplemented with 10% heat-inactivated FCS and 1% Penicillin/Streptomycin (Pen/Strep) at 37°C and 5% CO2. Cells were transferred into culture well plates as follows: 1.5 × 105 cells/well in 24 well culture plates or 3 × 105 cells/well in 12 well culture plates for gene expression analysis and 4 × 105 cells/well in 24 well culture plates or 8 × 105 cells/well in 12 well culture plates for western blot analysis. Cells were stimulated with the CM 1:1 diluted in fresh cell growth medium. For STING or cGAS inhibition, cells were pre-incubated with the respective antagonists in cell growth medium, as suggested by the manufacturer’s protocol: H-151 was added for 1–2 h for STING inhibition and RU.521 was added for 3 h for cGAS inhibition (both InvivoGen, France). SA planktonic CM was then added to the samples. Different inhibitor concentrations were included in the experiments and were generated from inhibitor stocks (H-151: 1 mg/ml; RU.521: 2 mg/ml) by serial dilution in growth medium. As the inhibitor stocks were solubilized in dimethyl sulphoxide (DMSO), effects of the solvent on macrophage activation upon stimulation with SA planktonic CM was evaluated. Therefore, cells were stimulated with SA planktonic CM with or without DMSO added in the volume corresponding to the highest used inhibitor concentration ranging from 0.004% to 0.5% as stated in the figure legends. For STING and cGAS activation, the respective agonists for STING: 3’3’-cGAMP and cGAS: G3-YSD in the transfection agent LyoVecTM (all InvivoGen, France) were added to the stimulation with SA biofilm CM. Activation of the IRF3 pathway was evaluated after 4 h by detection of p-IRF3 levels, and subsequent Ifnb induction. IFN-β signalling was analysed after 20 h by measuring phosphorylation of STAT1 and expression of the target gene Isg15. Gene expression levels of Il6 were included as marker for NF-κB mediated immune activation. The effect of the inhibitors on cell viability was determined after the maximum incubation period used in the experiments.
Isolation and stimulation of primary cells from mouse bone marrow and human whole blood
IRF3 activation by CM has been validated in primary cells. Bone marrow cells (BMCs) were isolated from adult C57BL/6 mice, in accordance with the National Guidelines for Animal Care and approved by the local authorities (Regierungspräsidium Karlsruhe, T-14/22). Bone marrow was centrifuged out of the femora and tibiae at 8000 rpm for 3 min and cultivated in α-MEM (Anprotec, Germany) supplemented with 10% heat-inactivated FCS and 1% Pen/Strep at 37°C and 5% CO2. The next day, the suspended cells were harvested, centrifuged, and resuspended in the respective growth medium. 10 million cells per 6 wells were stimulated with medium, SA planktonic or biofilm CM 1:1 diluted in fresh growth medium (3 ml in total) for 4 h at 37°C and 5% CO2.
Human peripheral blood mononuclear cells (PBMCs) were isolated from whole blood donated by healthy volunteers upon informed consent and approved by the local ethic committee (S-716/2017) via Percoll® density gradient centrifugation. Cells were washed and resuspended in RPMI (Anprotec, Germany) supplemented with 10% heat-inactivated FCS and 1% Pen/Strep. 10 million cells per 6 wells were stimulated with medium, SA planktonic or biofilm CM 1:1 diluted in fresh growth medium (3 ml in total) for 4 h at 37°C and 5% CO2.
Gene expression analysis of mammalian cells
Total RNA was extracted using the innuPREP RNA Mini Kit 2.0 (AJ Innuscreen GmbH, Germany) according to the manufacturer’s protocol and as previously described [Citation6]. Cells were treated with lysis buffer and lysates were transferred to a DNA elimination column to remove contaminating DNA. After addition of 70% ethanol, precipitated RNA was transferred to an RNA column, washed and eluted with RNAse free H2O. cDNA synthesis was performed with 1 µg of total RNA using the RevertUPTM II cDNA synthesis Kit (Biotechrabbit GmbH, Germany) according to the manufacturer’s protocol with a combination of oligo (dT) and hexamer primers. A noRT sample (without Reverse Transcriptase) was included. Two microlitres of an 1:1 diluted cDNA template were transferred into a two-step PCR reaction (StepOnePlus Real-Time PCR Cycler, Applied Biosystems, USA) using the 2× qPCRBIO SyGreen Mix Hi-ROX (PCR Biosystems Ltd., UK). Specificity of PCR products were controlled by melting curve comparison with noRT and water samples. The reference gene Hprt1 was used for normalization and mRNA levels of the genes of interest were calculated by the 2−∆Cq method. The sequences of the primers used in RT-qPCR analysis of mammalian cells are listed in . Primers were designed with the primer 3 software (https://primer3.ut.ee) using the sequence of the respective mouse gene extracted from the Ensembl genome browser (https://www.ensembl.org). If possible, the primers were designed intron-flanking and included all transcript variants. Primer specificity and quality were checked by blasting them against the NCBI database (https://www.ncbi.nlm.nih.gov/tools/primer-blast). The primers were obtained from Biomers.net GmbH, Germany.
Table 1. List of mouse specific oligonucleotides used for quantitative RT-PCR analysis.
Gene expression analysis of bacteria
Planktonic bacteria were harvested by centrifuging the liquid culture. Biofilms were harvested after removing the supernatants by scraping the biofilm matrix off the bottom of the well and centrifuging the pooled material. The pellets were resuspended in TE buffer and sonicated (SONOREX SUPER RK 102 H ultrasonic bath, BANDELIN electronic GmbH & Co. KG, Germany) and glass bead disruption (MINI-BEADBEATER™, Bio Spec Products Inc., USA). Lysis was performed using the innuPREP Bacteria Lysis Booster (AJ Innuscreen GmbH, Germany) and subsequent RNA isolation according to the manufacturer’s protocol. The RNA samples were treated with DNase I (Promega GmbH, Germany) to eliminate DNA contamination. RNA was transcribed into cDNA using hexamer primers following the manufacturer’s instructions. qPCR was performed as previously described for mammalian cells. The mRNA levels of the genes of interest were normalized to the reference gene gyrB and calculated using the 2−∆Cq method. Sequences of primers used in RT-qPCR analysis of bacteria are listed in .
Table 2. List of bacteria specific oligonucleotides used for quantitative RT-PCR analysis.
Bacterial primer design
SA specific primers were designed on the basis of the genome sequence of Staphylococcus aureus subsp. aureus USA300_FPR3757 (GCA_000013465). Genome sequences were extracted from the Ensembl Bacteria genome browser (http://bacteria.ensembl.org/index.html) and primers were obtained from Biomers.net GmbH, Germany. Primers for the gene encoding c-di-GMP synthase (diguanylate cyclase, DGC) were designed based on the transcript sequence of SAUSA300_0730 which encodes a protein containing a GGDEF-domain relevant for DGC activity. This gene shows high sequence similarity to SA0701 which is associated with c-di-GMP synthesis [Citation34]. Furthermore, the amino acid sequence of SAUSA300_0730 was blasted against the Staphylococcus aureus protein database using BLAST®, blastp suite (https://blast.ncbi.nlm.nih.gov/Blast.cgi), and revealed 100% sequence similarity to SA DGC.
Staining for dead cells
After incubation with the inhibitors, adherent cells were scraped off the well bottom directly into the culture medium without prior washing to include dead cells. Culture medium including living and dead cells was then centrifuged, cell pellet was washed, and resuspended in PBS. 30 nM SYTOXTM Green Nucleic Acid Stain (Invitrogen, USA) was added to the cell suspension and the percentage of fluorescein isothiocyanate (FITC)-positive to FITC-negative cell was measured after 5 min of incubation using a BD FACSCanto™ Flow Cytometer (BD Biosciences, USA). The results were analysed using the Flowing Software (version 2.5.1, Turku Bioscience, Finland).
Cytometric bead array
Supernatants of experiments for gene expression analysis were used for cytometric bead array (CBA, LEGENDplexTM, BioLegend, USA). CBA was performed according to the manufacturer’s protocol and as described in a previous study [Citation6]. In short: A Mouse Inflammation Panel (Mix and Match Subpanel) including TNF-α, IL-10, IFN-β, and IL-6 beads was used. Centrifuged supernatants were diluted 1:2.5 with Assay Buffer, and transferred into a V-bottom plate. A standard was included. After addition of the bead mix to the samples, the plate was incubated over night at 4°C in the dark while shaking. After washing, samples were incubated with the detection antibody for 1 h at RT, streptavidin-phycoerythrin (SA-PE) was added and the mixture was further incubated for 30 min at RT with shaking. Samples were analysed using the MACSQuant 10 (Miltenyi Biotech, Germany), and cytokine concentrations were calculated with the LEGENDplexTM Data Analysis Software (https://legendplex.qognit.com).
Immunoblotting
Western blot was performed as previously described [Citation6]. Cell lysis was performed in RIPA buffer (1% v/v NP-40 (FACSCanto™ CA-630), 0.25% sodium deoxycholate, 50 mM Tris pH 8.0, 150 mM NaCl, 1 mM EDTA pH 8.0, 1 mM Na3VO4) containing protease inhibitors (Protease Inhibitor Mix G) and phosphatase inhibitors (Phosphatase Inhibitor Mix II, both Serva Electrophoresis GmbH, Germany) for 1 h at 4°C under rotation. Protein concentration of centrifuged lysates was determined by BCA assay (Cyanagen Srl, Italy). 10 µg protein was loaded on pre-cast gradient 4–20% Tris-glycine gels (anamed Elektrophorese GmbH, Germany) covered with Laemmli buffer for SDS PAGE (Serva Electrophoresis GmbH, Germany) and separated at 120 V. A broad range, colour prestained protein standard (New England Biolabs GmbH, Germany) was added to each gel. Proteins were transferred onto an AmershamTM ProtranTM 0.45 µm nitrocellulose membrane (GE Healthcare, UK) covered in 4 Whatman papers of each membrane side (GE Healthcare, UK) soaked in transfer buffer (192 mM glycine, 25 mM Tris, 2.6 mM SDS, 0.5 mM NA3VO4, 15% v/v methanol) for 1 h at 2 mA/cm2. Ponceau S staining (0.5% Ponceau S, 3% trichloroacetic acid, 96.5% ddH2O) was performed for protein visualization. The membranes were blocked with BlueBlock PF (Serva Electrophoresis GmbH, Germany) for 30 min at RT and incubated with primary antibodies in BlueBlock PF over night at 4°C. Protein visualization was done after incubation with the respective Horseradish peroxidase (HRP)-linked secondary antibody for 1 h at RT with ECL substrate (WESTAR ETA C ULTRA 2.0, Cyanagen Srl, Italy) in a ChemoStar ECL and Fluorescence Imager (Intas Science Imaging Instruments GmbH, Germany). Antibodies used for immunoblotting are listed in . Antibodies were all recommended for use in mice and humans (HSP90 and p-IRF3) and were already successfully used in previous studies [Citation6,Citation35].
Table 3. List of antibodies used for immunoblotting (western blot).
Quantification of Protein A in CM
Protein A was quantified in the CM using a Protein A ELISA Kit (Abcam, UK) according to the manufacturer’s protocol. In short, CM was diluted 1:50 for medium and biofilm CM, and 1:5000 for planktonic CM. Samples were pretreated with a boiling step in sample diluent + enhancer (kit components) for 5 min, and a standard was prepared. The samples and standards were transferred to a ready-to-use ELISA plate. An antibody cocktail was then prepared and added to each well. The plates were then incubated for 1 h at RT. After three washing steps, TMB development solution was added and incubated for 10 min. Stop solution was added, and the OD at 450 nm was detected using the CLARIOstar® Plus microplate reader (BMG Labtech, Germany).
Quantification of 3’3’-cGAMP in CM
3’3’-cGAMP was quantified in the CM using the FACSCanto™ 3’3’-cGAMP ELISA Kit (Arbor Assays, USA) according to the manufacturer’s protocol. Briefly, CM was used undiluted, and the standard was prepared. The samples and standards were transferred to a ready-to-use ELISA plate. The DetectX® 3,’3’-cGAMP Conjugate and Antibody were added to the wells. The plates were then incubated for 1 h at RT with shaking. After four washing steps, TMB substrate was added and incubated for 30 min. Stop solution was added, and the OD at 450 nm was detected usingthe CLARIOstar® Plus microplate reader (BMG Labtech, Germany).
Statistical analysis
Three to six independent replicates were included in each experiment as stated in the figure legends. Mean ± SEM is shown with single values presented as dots. For statistical evaluation, an ordinary one-way analysis of variance (ANOVA) including post-hoc multiple comparison testing and Bonferroni correction was performed. A p-value < 0.05 was considered statistically significant. Further information regarding the comparisons in the respective experiments is given in the figure legends. Statistical analysis was done with GraphPad Prism for Windows (version 9.3.1; GraphPad Software Inc., USA).
Results
Inhibition of STING prevents IRF3 mediated Ifnb induction by macrophages upon stimulation with SA planktonic CM
Stimulation of RAW 264.7 macrophages with CM of SA planktonic or biofilm cultures showed that the SA planktonic environment activates the IRF3 pathway, whereas an increase in p-IRF3 levels was not detected in the respective biofilm environment (, upper blot). This effect was also observed in primary mouse BMCs and human PBMCs (, middle and lower panel), indicating that IRF3 pathway activation upon stimulation with SA planktonic CM is conserved across different cell lines and species. In human PBMCs, low levels of p-IRF3 were detectable in the unstimulated medium control and the biofilm treated sample. Densitometric analysis, however, showed that the p-IRF3 levels of these conditions were comparable whereas stimulation with SA planktonic CM caused a 3.3-fold increase in p-IRF3 levels compared to the respective biofilm CM (Suppl. Figure S1). STING inhibition by the small-molecule inhibitor H-151 dose-dependently prevented the induction of Ifnb and its target gene Isg15 (), due to the absence of IRF3 and STAT1 activation () upon stimulation with SA planktonic CM. Il6 mRNA levels, however, were only slightly and non-significantly reduced by H-151 (), indicating that the general inflammatory response, independent of IFN-β, was still induced by the SA planktonic environment. As the solvent control (DMSO, 0.04%) showed no effect on Ifnb, Isg15 and Il6 gene expression levels and IRF3 activation, respectively, (, separate graphs) the effects were indeed mediated by the action of the inhibitor. As H-151 treatment had adverse effects on cell viability at high concentrations (4000 ng/ml; 12.83 ± 3.18%, ), this concentration was not further included. Within the range of 4–400 ng/ml, inhibitor treatment had no (4 and 40 ng/ml; 91.98 ± 1.22% and 90.67 ± 4.32%, respectively) or tolerable (400 ng/ml; 76.52 ± 8.65%) effects on cell viability (untreated and DMSO treated cells shows 90.73 ± 0.79% and 91.59 ± 2.395, respectively). Thus, our data indicate that STING activation is crucial for the IRF3 induced IFN-β response in SA planktonic environments.
Figure 1. Effect of STING inhibition on the IRF3/IFN-β response upon stimulation with SA planktonic CM. Cells were stimulated with control medium (Med.) or SA planktonic CM 1:1 diluted in fresh growth media (DMEM high glucose + 10% FCS + 1% Pen/Strep) and effect of the STING antagonist H-151 on the IRF3 mediated IFN-β response was evaluated. a) Activation of the IRF3 pathway in different cell populations. RAW 264.7 cells, primary mouse BMCs and primary human PBMCs were treated with SA planktonic or biofilm CM for 4 hours and presence of phospho-IRF3 as activated form of the transcription factor was visualized by western blot. HSP90 was used as loading control. n = 3 experiments. b+c) Gene expression analysis of Ifnb and target gene Isg15. RAW 264.7 cells were treated with H-151 in different concentrations (4, 40 and 400 ng/ml) and SA planktonic CM for 20 hours and mRNA levels of Ifnb (b) and Isg15 (c) were quantified by RT-qPCR. DMSO as solvent control was added to SA planktonic CM in the volume according to the highest inhibitor concentration (400 ng/ml, corresponding to 0.04%) and effects on Ifnb and Isg15 gene expression are shown in the additional bar graphs. Data are presented as relative gene expression of gene of interest related to the reference gene Hprt1 and normalized to the unstimulated medium control. n = 4 (DMSO) or 6 (H-151) experiments. d) Activation of the IRF3 pathway. RAW 264.7 cells were treated with H-151 in different concentrations (4, 40 and 400 ng/ml) and SA planktonic CM for 4 hours and presence of phospho-IRF3 as activated form of the transcription factor was visualized by western blot. DMSO as solvent control was added to SA planktonic CM in the volume according to the highest inhibitor concentration (400 ng/ml, corresponding to 0.04%) and effects on p-IRF3 levels are shown on the additional blot. HSP90 was used as loading control. n = 3 experiments. e) Activation of the IFN-β pathway. RAW 264.7 cells were treated with H-151 in different concentrations (4, 40 and 400 ng/ml) and SA planktonic CM for 20 hours and presence of phospho-STAT1 as activated form of the transcription factor and downstream target of the IFN-β receptor was visualized by western blot. IFN-β stimulation was included as positive control. β-Actin was used as loading control. n = 3 experiments. f) Gene expression analysis of stress marker IL-6. RAW 264.7 cells were treated with H-151 in different concentrations (4, 40 and 400 ng/ml) and SA planktonic CM for 20 hours and mRNA levels of Il6 were quantified by RT-qPCR. DMSO as solvent control was added to SA planktonic CM in the volume according to the highest inhibitor concentration (400 ng/ml, corresponding to 0.04%) and effects on Il6 gene expression are shown in the additional bar graph. Data are presented as relative gene expression of gene of interest related to the reference gene Hprt1 and normalized to the unstimulated medium control. n = 4 (DMSO) or 6 (H-151) experiments. g) Viability of cells after treatment with H-151. RAW 264.7 cells were treated with H-151 in different concentrations (4, 40, 400 and 4000 ng/ml) for 22 hours (duration of the experiments covering 2 hours of pre-incubation and 20 hours of CM stimulation) and cell viability was measured by SYTOXTM-staining and FACS analysis. DMSO as solvent control was added in the volume according to the highest inhibitor concentration (4000 ng/ml, corresponding to 0.4%). Percentage of SYTOXTM-negative (living) cells are shown. n = 4 experiments. For b+c/f+g: Data are presented as mean ± SEM and single values are shown as dots. p-values were calculated by ordinary one-way ANOVA with post-hoc Bonferroni corrected multiple comparison. * is indicating significance against medium (Med.), + is indicating significance against DMSO, # is showing significance between inhibitor concentrations. *p < 0.05, **p < 0.01, ***p < 0.001; + p < 0.05, ++ p < 0.01, +++ p < 0.001; #p < 0.05, ##p < 0.01, ###p < 0.001.
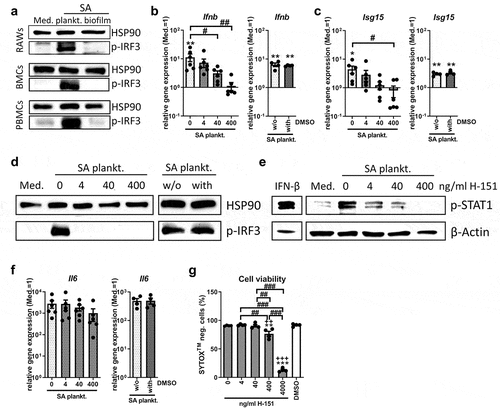
STING activation leads to an IRF3 induced IFN-β response by macrophages upon stimulation with SA biofilm CM
We next investigated whether the SA biofilm environment actively inhibited STING activation and if this was the cause of the missing IRF3 activation. Therefore, we added the bacterial STING agonist 3’3’-cGAMP to wells stimulated with SA biofilm CM and evaluated IRF3 activation and the subsequent IFN-β induction. Interestingly, 3’3’-cGAMP significantly induced Ifnb and Isg15 gene expression upon stimulation with SA biofilm CM (). This was accompanied by the phosphorylation of IRF3 in samples treated with 3’3’-cGAMP and SA biofilm CM (). Il6 gene expression was induced by SA biofilm CM and was further increased when 3’3’-cGAMP was added (). The observed Ifnb induction and increased Il6 expression by 3’3’-cGAMP were also confirmed at the protein level (IFN-β: 188.3 ± 79.4 fold and IL-6: 15.8 ± 6.2 fold; ). Protein levels of the pro-inflammatory cytokine TNF-α remained unaffected (1.42 ± 0.56 fold), whereas protein levels of the anti-inflammatory cytokine IL-10 were significantly elevated by the addition of 3’3’-cGAMP to SA biofilm CM (6.96 ± 1.70 fold). The ratio of pro-inflammatory TNF-α to anti-inflammatory IL-10 can be used as indicator for the macrophage polarization as previously evaluated [Citation6], and resulted in a significant decrease in pro-inflammatory macrophage polarization upon treatment with 3’3’-cGAMP (0.19 ± 0.04 fold; ). Interestingly, the effects of 3’3’-cGAMP on IFN-β production were significantly higher in combination with SA biofilm CM than when used alone (12.0 ± 5.7 fold). This indicates that the SA biofilm environment does not generally prevent STING pathway activation and that the addition of 3’3’-cGAMP to the SA biofilm CM lead to a pronounced IFN-β response.
Figure 2. Effect of STING activation on the IRF3/IFN-β response upon stimulation with SA biofilm CM. RAW 264.7 cells were stimulated with control medium (Med.) or SA biofilm CM 1:1 diluted in fresh growth media (DMEM high glucose + 10% FCS + 1% Pen/Strep) and effect of the bacterial STING agonist 3’3’-cGAMP on the IRF3 mediated IFN-β response was evaluated. a) Gene expression analysis of Ifnb and target gene Isg15. RAW 264.7 cells were treated with 3’3’-cGAMP in different concentrations (1 and 10 µg/ml) and SA biofilm CM for 20 hours and mRNA levels of Ifnb and Isg15 were quantified by RT-qPCR. Data are presented as relative gene expression of gene of interest related to the reference gene Hprt1 and normalized to the unstimulated medium control. n = 4 experiments. b) Activation of the IRF3 pathway. RAW 264.7 cells were treated with 3’3’-cGAMP in different concentrations (1 and 10 µg/ml) and SA biofilm CM for 4 hours and presence of phospho-IRF3 as activated form of the transcription factor was visualized by western blot. HSP90 was used as loading control. n = 3 experiments. c) Gene expression analysis of stress marker Il6. RAW 264.7 cells were treated with 3’3’-cGAMP in different concentrations (1 and 10 µg/ml) and SA biofilm CM for 20 hours and mRNA levels of Il6 were quantified by RT-qPCR. Data are presented as relative gene expression of gene of interest related to the reference gene Hprt1 and normalized to the unstimulated medium control. n = 4 experiments. d-f) Cytokine release of IFN-β, IL-6, pro-inflammatory TNF-α and anti-inflammatory IL-10. RAW 264.7 cells were treated with 3’3’-cGAMP in different concentrations (1 and 10 µg/ml) and SA biofilm CM for 20 hours and protein concentrations of IFN-β (d) and IL-6 (e) as well as pro-inflammatory TNF-α and anti-inflammatory IL-10 (f) were quantified in the supernatant by cytometric bead array (CBA; LEGENDplexTM). Data are presented as absolute concentration (pg/ml). Ratio of TNF-α to IL-10 protein levels was used as indicator for macrophage polarization. n = 4 experiments. For a/c-f: Data are presented as mean ± SEM and single values are shown as dots. p-values were calculated by ordinary one-way ANOVA with post-hoc Bonferroni corrected multiple comparison. * is indicating significance against medium (Med.), + is indicating significance between 10 µg/ml 3’3’-cGAMP in medium or SA biofilm CM, # is showing significance between 3’3’-cGAMP concentrations. *p < 0.05, **p < 0.01, ***p < 0.001; + p < 0.05, ++ p < 0.01, +++ p < 0.001; #p < 0.05, ##p < 0.01, ###p < 0.001.
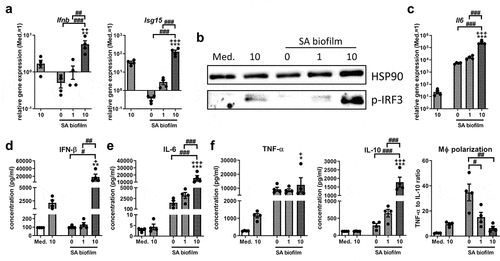
Gene expression levels associated with virulence but not CDN synthesis are decreasing with biofilm formation and Ifnb induction
Our data indicated that stimulation with SA planktonic CM led to STING activation, whereas this stimulus seemed to be missing in the respective biofilm CM. Bacteria-derived CDNs are known ligands for STING [Citation12] and play a role in bacterial adaptation processes such as biofilm formation [Citation14]. Therefore, we evaluated the effect of biofilm formation on the gene expression levels of CDN synthases at different time points during biofilm formation. As shown in , both planktonic and biofilm SA expressed the genes encoding the CDN synthases for c-di-AMP (diadenylate cyclase dacA) and c-di-GMP (diguanylate cyclase dgc), as well as the phosphodiesterase gdpP, the enzyme that degrades c-di-AMP. The mRNA levels of dacA did not differ significantly between planktonic and biofilm SA, whereas the gene expression levels of diguanylate cyclase dgc were highest on day 1 biofilm SA. In line with this, the mRNA levels of the phosphodiesterase gdpP were lowest on day 1 biofilm SA and increased with biofilm age (). We could not detect relevant amounts of the bacterial CDN 3’3’-cGAMP in both, SA planktonic and biofilm CM (). These data indicate that CDNs are not the main mediators of STING/IRF3 activation and Ifnb induction in SA planktonic CM. We further investigated the gene expression levels of molecules associated with QS and virulence, which are known to be regulated during SA biofilm formation [Citation36,Citation37]. Here, we found that mRNA levels of the effector molecule of the accessory global regulator (agr) QS system RNAIII and the RNAIII-encoded gene of the phenol-soluble modulin δ-toxin (hld) significantly decreased during biofilm formation (). Gene expression levels of sarS, a member of the staphylococcal accessory regulator (SarA) QS system and direct activator of Protein A (spa) [Citation38] did not differ significantly between planktonic and biofilm cultures, but was lowest in day 1 biofilm SA (). Protein A expression significantly decreased with biofilm formation and maturation at both, the mRNA and protein level (0.0077 ± 0.0007 fold; ). Overall, gene expression analysis of planktonic and biofilm bacteria revealed that mRNA levels of CDN synthases did not correlate with IRF3 mediated Ifnb induction in SA planktonic CM. Our data show that genes associated with QS and virulence are highly expressed in planktonic SA and get downregulated as a consequence of biofilm formation, suggesting that bacterial factors other than CDNs are involved in STING pathway activation.
Figure 3. Differences in bacterial gene expression and release of 3’3’-cGAMP or protein a between SA planktonic and biofilm cultures. SA was cultured in DMEM high glucose with 10% heat-inactivated FCS at 37°C and 5% CO2 either under shaking for planktonic or static for biofilm conditions. Bacteria and supernatants were harvested after 24 hours of planktonic or after 1 day, 3 days or 6 days of biofilm culture. a) Gene expression analysis of c-di-AMP synthase (diadenylate cyclase, DacA), c-di-GMP synthase (GGDEF domain protein, diguanylate cyclase, DGC) and phosphodiesterase (GdpP). mRNA levels of dacA, dgc and gdpP were quantified by RT-qPCR. Data are presented as relative gene expression of gene of interest related to the reference gene gyrB. n = 3 experiments. b) 3’3’-cGAMP release by planktonic and biofilm SA. 3’3’-cGAMP concentration was measured in medium without bacteria, SA planktonic CM and 6 days biofilm CM. For validation of the assay, known concentrations of 3’3’-cGAMP (0, 100, 1000 and 10,000 ng/ml) in medium were included in the measurement. Concentrations were quantified in the supernatants by competitive ELISA. Data are presented as absolute concentration (ng/ml). n = 3 different CM batches in duplicates. c+d) Gene expression analysis of agr/SarA quorum sensing (QS) molecules. mRNA levels of the agr effector molecule RNAIII and RNAIII encoded PSM δ-toxin (hld gene, c) and SarA regulator protein sarS (d) were quantified by RT-qPCR. Data are presented as relative gene expression of gene of interest related to the reference gene gyrB. n = 3 experiments. e) Protein A production by planktonic and biofilm SA. mRNA levels of spa were quantified by RT-qPCR (left graph). Data are presented as relative gene expression of gene of interest related to the reference gene gyrB. n = 3 experiments. Protein A concentration was measured in SA planktonic CM and 6 days biofilm CM (right graph). For validation of the assay, known concentrations of Protein A (SpA; 50 and 100 pg/ml) in medium were included in the measurement. Concentrations were quantified in the supernatants by quantitative sandwich ELISA. Data are presented as absolute concentration (ng/ml). n = 3 different CM batches in duplicates. For all: Data are presented as mean ± SEM and single values are shown as dots. p-values were calculated by ordinary one-way ANOVA with post-hoc Bonferroni corrected multiple comparison. # is showing significance between different CM. #p < 0.05, ##p < 0.01, ###p < 0.001.
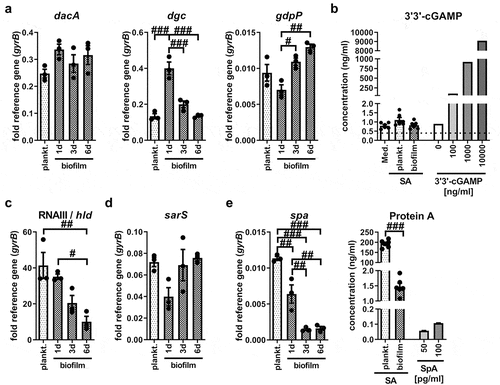
Cytosolic DNA sensor cGAS is involved in the IRF3 mediated Ifnb induction upon stimulation with SA planktonic CM
As direct STING activation by bacteria-derived CDNs did not seem to be the mechanism behind IRF3 mediated Ifnb induction in SA planktonic CM, we investigated whether the cytosolic DNA sensor cGAS, upstream of STING, was involved. Therefore, we inhibited cGAS by treating the cells with the small-molecule inhibitor RU.521 prior to CM stimulation. shows that inhibition of cGAS by RU.521 efficiently and completely blocked activation of the cGAS pathway by its agonist G3-YSD. Cell viability was not affected by treatment with the cGAS inhibitor, RU.521, at the concentrations used (0 µg/ml: 91.33 ± 1.02%, 5 µg/ml: 89.90 ± 0.66%, 10 µg/ml: 91.19 ± 1.15%, DMSO: 91.44 ± 0.73%; ). Furthermore, cGAS inhibition resulted in a dose-dependent reduction of Ifnb and Isg15 induction upon stimulation with planktonic SA CM (). Induction of Il6 gene expression was reduced only slightly, and statistically not significantly, by cGAS inhibition (). Addition of the corresponding DMSO concentration (0.5%) to stimulation with the cGAS agonist G3-YSD or SA planktonic CM led to a slight increase in Ifnb, Isg15 and Il6 gene expression levels (Suppl. Figure S2a-c) indicating that the reduction was caused by cGAS inhibition and was not mediated by the solvent. Unexpectedly, adding the cGAS agonist G3-YSD to the stimulation with SA biofilm CM was not sufficient to induce expression of Ifnb and its target gene Isg15 compared to cGAS activation by G3-YSD in the medium control (Ifnb: 3.33 ± 0.45 compared to 0.92 ± 0.08, Isg15: 7.50 ± 2.02 compared to 1.15 ± 0.11; ). Induction of Il6 gene expression by SA biofilm CM was not affected by the cGAS agonist (470.3 ± 244.9 compared to 515.2 ± 235.5; ). Thus, unlike direct STING activation with 3’3’-cGAMP, treatment of the cells with the cGAS agonist G3-YSD did not induce Ifnb gene expression and downstream targets upon stimulation with SA biofilm CM. This suggests that the necessary stimulus is not only missing in the biofilm environment but also that the biofilm environment actively prevents cGAS activation.
Figure 4. Role of cGAS on the Ifnb induction upon stimulation with SA planktonic or biofilm CM. Cells were stimulated with control medium (Med.), SA planktonic or biofilm CM 1:1 diluted in fresh growth media (DMEM high glucose + 10% FCS + 1% Pen/Strep) and effect of the cGAS antagonist RU.521 or cGAS agonist G3-YSD/LyoVecTM on the induction of Ifnb and its target gene expression was evaluated. a) Evaluation of the cGAS inhibitor RU.521. RAW 264.7 cells were treated with RU.521 in different concentrations (5 and 10 µg/ml) and 100 ng/ml of the cGAS agonist G3-YSD or its control was added to the cells together with the transfection reagent LyoVecTM for 20 hours. mRNA levels of Ifnb and Isg15 were quantified by RT-qPCR. Data are presented as relative gene expression of gene of interest related to the reference gene Hprt1 and normalized to the unstimulated medium control. n = 2 experiments. b) Viability of cells after treatment with RU.521. RAW 264.7 cells were treated with RU.521 in different concentrations (5 and 10 µg/ml) for 23 hours (duration of the experiments covering 3 hours of pre-incubation and 20 hours of CM stimulation) and cell viability was measured by SYTOXTM -staining and FACS analysis. DMSO as solvent control was added in the volume according to the highest inhibitor concentration (10 µg/ml, corresponding to 0.5%). Percentage of SYTOXTM -negative (living) cells are shown. n = 4 experiments. c+d) Gene expression analysis of Ifnb, target gene Isg15 and stress marker Il6. RAW 264.7 cells were treated with RU.521 in different concentrations (5 and 10 µg/ml) and SA planktonic CM was added to the cells for 20 hours. Cells stimulated with SA biofilm CM were included for comparison. mRNA levels of Ifnb and Isg15 (c) and Il6 (d) were quantified by RT-qPCR. Data are presented as relative gene expression of gene of interest related to the reference gene Hprt1 and normalized to the unstimulated medium control. n = 4 experiments. e+f) Gene expression analysis of Ifnb, target gene Isg15 and stress marker Il6. RAW 264.7 cells were treated with medium or SA biofilm CM and 100 ng/ml of the cGAS agonist G3-YSD or its control together with the transfection reagent LyoVecTM for 20 hours. mRNA levels of Ifnb and Isg15 (e) and Il6 (f) were quantified by RT-qPCR. Data are presented as relative gene expression of gene of interest related to the reference gene Hprt1 and normalized either to the control/LyoVecTM sample (Ifnb + Isg15) or to the unstimulated medium control (Il6). n = 4 experiments. For b-f: Data are presented as mean ± SEM and single values are shown as dots. p-values were calculated by ordinary one-way ANOVA with post-hoc Bonferroni corrected multiple comparison. * is indicating significance against medium (Med.), # is showing significance between different inhibitor concentrations or treatments. *p < 0.05, **p < 0.01, ***p < 0.001; #p < 0.05, ##p < 0.01, ###p < 0.001.
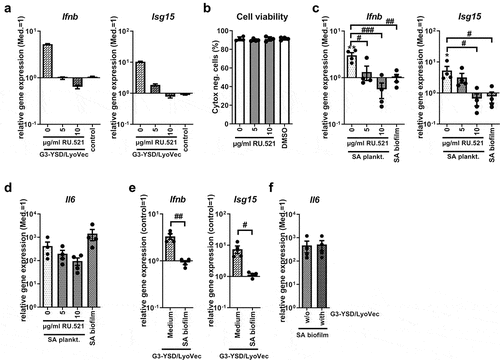
Induction of Ifnb gene expression by SA planktonic CM is not conserved throughout different SA strains
To understand whether the observed effects were dependent on the characteristics of the specific SA strain used for CM preparation, we included the MRSA strain USA300 and the reference SA strain ATCC 25923 in our study. USA300 (FPR3757) was isolated from a wrist abscess and ATCC 49230 (UAMS-1) from an osteomyelitis; therefore, both can be considered invasive and virulent SA strains. The strains were cultivated in parallel using the same conditions and prepared CM from planktonic and biofilm cultures at different ages. Interestingly, the induction of Ifnb and Isg15 gene expression, which we had observed for planktonic CM using the strain ATCC 42930 (), directly disappeared as early as day 1 when changing the culture conditions from planktonic to biofilm. For CM generated from USA300, the highest induction of Ifnb and Isg15 gene expression was detected in planktonic CM, but was still detectable on day 1 with biofilm CM and then declined during biofilm maturation until day 6. Stimulation with planktonic or biofilm CM of the SA strain ATCC 25923 and SA strain SA113 (data not shown) did not result in the induction of Ifnb and Isg15 gene expression (). Cells responded to all CM with increased Il6 mRNA levels, indicating that planktonic and biofilm environments of all SA strains were able to induce an adequate immune response (). Ifnb induction by planktonic CM of USA300 was dependent on STING activation (), whereas induction of Il6 gene expression was not affected by STING inhibition (). This is in line with the results obtained for the SA strain ATCC 49230 (). Taken together, these results indicate that STING-dependent Ifnb induction is likely associated with the degree of virulence and the release of bacterial factors into the environment.
Figure 5. Ifnb induction upon stimulation with SA planktonic or biofilm CM of different SA strains. CM were generated from planktonic or biofilm cultures of SA strains ATCC 49230, the MRSA USA300 and the reference strain ATCC 25923. Planktonic CM were harvested after 24 hours of shaking culture and biofilm CM were harvested on day 1 (young), day 3 (maturating) and day 6 (mature) of static culture. RAW 265.7 cells were stimulated with CM 1:1 diluted in fresh growth media (DMEM high glucose + 10% FCS + 1% Pen/Strep) and induction of an IFN-β response was evaluated. a+b) Gene expression analysis of Ifnb, target gene Isg15 and stress marker Il6. RAW 264.7 cells were stimulated with the CM for 20 hours and mRNA levels of Ifnb and Isg15 (a) and Il6 (b) were quantified by RT-qPCR. c+d) STING inhibition on gene expression analysis of Ifnb and stress marker Il6. RAW 264.7 cells were treated with 40 ng/ml H-151 and SA planktonic CM generated from the MRSA strain USA300 for 20 hours and mRNA levels of Ifnb (c) and Il6 (d) were quantified by RT-qPCR. DMSO as solvent control was added to SA planktonic CM in the volume according to the inhibitor concentration (40 ng/ml, corresponding to 0.004%) and effects on Ifnb and Il6 gene expression are shown in the additional bar graph. Data are presented as relative gene expression of the gene of interest related to the reference gene Hprt1 and normalized to the unstimulated medium control (Med.). n = 2 (DMSO) or 6 (H-151) experiments. For all: Data are presented as mean ± SEM and single values are shown as dots. p-values were calculated by ordinary one-way ANOVA with post-hoc Bonferroni corrected multiple comparison. * is indicating significance against medium (Med.), # is showing significance between planktonic and biofilm CM or inhibitor concentrations. *p < 0.05, **p < 0.01, ***p < 0.001; + p < 0.05, ++ p < 0.01, +++ p < 0.001; #p < 0.05, ##p < 0.01, ###p < 0.001.
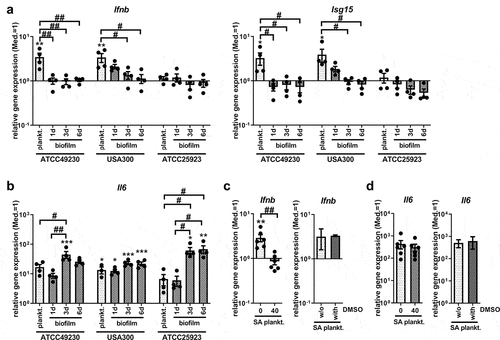
Discussion
Impaired immune response reactions during biofilm formation are a major problem in implant-related bone infections and present an attractive target for innovative therapeutic strategies. In our previous study, we found that the presence of an SA planktonic environment induces IFN-β production, presumably via IRF3 activation, which was not detectable in the respective biofilm environment [Citation6]. STING activation leads to IRF3 mediated production of IFN-β and although the STING/IRF3 pathway has gained increasing attention in bacterial infection research, this is, to the best of our knowledge, the first study that compared the effects of STING pathway activation between planktonic and biofilm environments. Indeed, we found that Ifnb induction by SA planktonic CM was dependent on STING and that STING activation resulted in Ifnb gene expression in SA biofilm CM. This could, however, not be explained by the different expression levels of CDN synthases in planktonic or biofilm SA. Furthermore, although cGAS seems to play a role in Ifnb induction by SA planktonic CM, addition of a cGAS agonist was not sufficient to induce Ifnb expression in biofilm CM. This is an unexpected observation, as addition of a STING agonist was able to do so. Our results suggest that differently regulated expression levels of genes associated with virulence are involved. Interestingly, Ifnb gene expression was detected upon stimulation with planktonic CM generated from two virulent and invasive SA strains, ATCC 49230 and USA300, whereas this was not observed for the SA strains ATCC 25923 and SA113 which are less virulent. Overall, our data indicate that STING activation is associated with a higher degree of virulence. As virulence decreases during the conversion to biofilm, increasing biofilm maturation prevents STING-dependent Ifnb induction.
Our data are in line with other studies showing a STING dependent IFN-β production in RAW 264.7 cells or mouse BMDMs upon infection with clinically relevant SA strains [Citation28,Citation39]. STING activation was only observed for living SA and disappeared when the bacteria were heat-killed, indicating that the stimulus had to be actively produced by the bacteria. Hence, it can be assumed that in the indirect infection model used in our study, the relevant bacterial factor is produced during planktonic culture and released into the environment.
C-di-NMPs, and in particular c-di-GMP, are thought to play a role in the initiation of the switch from a motile to a sessile lifestyle in bacteria, and this might be involved in the immune recognition of early biofilm formation via activation of the STING pathway [Citation40]. Indeed, c-di-AMP released by SA biofilms has been shown to induce an IFN-β response in macrophages [Citation31]. Thus, we investigated whether differences in c-di-NMP production between planktonic and biofilm SA could be relevant factors in our model system. In line with this study, the diadenylate cyclase dacA was expressed in our SA biofilm cultures. Furthermore, we found that mRNA levels of the diguanylate cyclase dgc were increased in day 1 biofilm SA, which fits with the function of c-di-GMP as a factor involved in planktonic-to-biofilm transition [Citation16,Citation40]. Unexpectedly, the observed gene expression levels of CDN synthases did not correlate with the ability to induce Ifnb induction. In addition, as we did not detect Ifnb gene expression upon stimulation with biofilm CM, we propose that differential production of bacterial c-di-NMPs is not responsible for the observed STING-dependent Ifnb induction in our model.
Biofilm formation is associated with changes in gene expression towards environmental adaption and reduced virulence [Citation5,Citation41]. Thus, we compared the expression levels of the agr QS effector molecule RNAIII, the encoded δ-toxin (hld), and Protein A (spa) between planktonic and biofilm SA. In line with the existing literature, the expression of these virulence-associated genes was highest in planktonic SA and decreased with progressing biofilm formation. Additionally, high c-di-GMP concentrations are associated with the reduced expression of virulence factors [Citation42]. As dgc mRNA levels were highest during early biofilm SA, this indicated that the virulence of biofilm-cultured SA indeed decreased. Thus, it seems that the observed STING-dependent Ifnb induction may be related to the different production of virulence-associated bacterial factors. For example, Protein A can induce IFN-β production in airway epithelial cells upon endocytic uptake [Citation43]. Additionally, the ability to induce an IFN-β response via the NOD2-IRF5 pathway is associated with the virulence of the respective SA strains [Citation44]. To strengthen our hypothesis that bacterial factors associated with virulence are the main mediators resulting in Ifnb induction via the STING/IRF3 pathway, we aimed to exclude a potential inhibitory effect of biofilm CM on STING induction. In a previous study, we had shown that low glucose and high lactate levels, characteristic for the biofilm environment, did not prevent IRF3 activation and Ifnb induction by SA planktonic CM [Citation6]. Here we show, that addition of the bacterial STING agonist 3’3’-cGAMP results in a pronounced IRF3 mediated IFN-β production in SA biofilm CM. This indicates that the stimulus for STING activation is rather missing in the SA biofilm environment but not inactivated.
As our results suggest that bacterial CDNs are not responsible for STING activation in the SA planktonic environment, we investigated the role of the upstream cytosolic DNA sensor cGAS. cGAS has already been described to be involved in STING activation in SA infections [Citation28,Citation45], but our study is the first to investigate the role of cGAS in the context of biofilm formation. Indeed, inhibition of cGAS resulted in the loss of Ifnb induction when stimulated with SA planktonic CM. cGAS can be activated by foreign DNA derived from invading microbes or by endogenous DNA released from damaged and dying host cells, leading to subsequent STING activation via the synthesis of 2’3’-cGAMP [Citation21,Citation46]. In a previous study, we had shown that SA biofilm CM contained higher amounts of free bacterial DNA than the respective planktonic CM [Citation7] indicating that a lack of bacterial DNA in the environment can be excluded as the cause for the absent cGAS activation by SA biofilm CM. More likely, the uptake of bacterial DNA is mediated by endocytosis and results in the activation of endosomal TLR-9 signalling [Citation47,Citation48]. An increased production of virulence factors by planktonic SA will result in host cell damage and the release of endogenous DNA. This is in line with our previous finding that stimulation with planktonic CM of the less virulent Staphylococcus epidermidis (SE) did not result in IRF3 activation [Citation6] and that Ifnb induction via the STING pathway is dependent on the virulence of the SA strain. Additionally, a study by Scumpia et al. showed that IFN-β production via the cGAS/STING pathway increased with increasing virulence of staphylococci strains, which the authors also explained with a higher rate of cell death [Citation28]. These data collectively suggest that the biofilm environment is less harmful to the cells due to a decrease in virulence when compared to the planktonic situation. Eventually, this results in reduced cell damage and cGAS-mediated 2’3’-cGAMP production and subsequently loss of STING activation. Unexpectedly, the addition of the cGAS agonist G3-YSD leads to Ifnb gene expression in the medium control, but still was not sufficient to induce Ifnb gene expression in SA biofilm CM. Thus, in addition to the missing stimulus, the biofilm environment seems to actively prevent cGAS activation even in the presence of a cGAS agonist. Inhibitory mechanisms for cGAS activation have been described for viruses as part of their immune evasion strategies [Citation49] and might also occur in biofilm infections. Possible mechanisms include the restriction of cGAS protein levels by suppressed protein synthesis or enhanced protein degradation [Citation19,Citation46], the disruption of cGAS activation by interfering with DNA binding or the reduction of cGAS activity by post-translational modifications [Citation50]. An increased degradation of the cGAS product 2’3’-cGAMP by PDEs could be a further cause for the missing down-stream STING activation [Citation19]. At this point, we cannot explain exactly how the biofilm environment prevents cGAS/STING pathway activation, and this point warrants further investigation.
The influence of IFN-β on the outcome of the immune response in bacterial infections is discussed controversially [Citation51,Citation52]. In SA pneumonia, IFN-β is associated with suppressed immune cell recruitment and cytokine production resulting in increased morbidity [Citation43,Citation44]. However, in an SA skin infection model, IFN-β supported an effective immune response and enhanced bacterial clearance [Citation53]. Thus, it has to be evaluated if activation of the cGAS/STING/IRF3 pathway and the subsequent production of IFN-β is beneficial or detrimental in the context of implant-related bone infections. As IFN-β acts as a negative feedback signal for the formation of bone resorbing osteoclasts [Citation7,Citation54], a limiting effect of IFN-β on infection-associated bone destruction is possible. STING activation also caused an increased production of IL-6 and IL-10 upon stimulation with SA biofilm CM, and the anti-inflammatory cytokine IL-10 was shown to contribute to bacterial persistence in an SA orthopaedic biofilm infection model [Citation55], indicating that increased IL-10 production upon exogenous STING activation is disadvantageous. Thus, it has to be evaluated whether the risk that anti-inflammatory processes and immune tolerance are supported by the induction of IFN-β outweighs its potential beneficial impact. Therefore, a more detailed analysis of the effects of STING activation on the immune response against biofilms is needed.
Overall, our results show for the first time that the cGAS/STING/IRF3 pathway is differentially regulated in SA planktonic and biofilm environments, and strongly suggest that it might be an attractive target for immunomodulatory interventions that aims to strengthen the immune response during biofilm formation. Nevertheless, the role of IFN-β in this process and its implications must be fully clarified to assess whether interfering with STING pathway activation is a conceivable approach in the treatment of implant-related bone infections.
Author contribution
ES was responsible for the study conception and design, acquisition, analysis, and interpretation of data, and wrote the manuscript. GS participated in the data acquisition and analysis. KFK contributed to the data interpretation and critically revised the manuscript. All authors have read and approved the final manuscript.
Supplemental Material
Download MS Word (231.3 KB)Acknowledgements
We would like to thank Dafina Kadrijaj and Tabea Elschner for their assistance with the experiments. Furthermore, we thank Dr. Lan-Sun Chen for providing the human PBMCs.
Disclosure statement
The authors declare that they have no financial or non-financial interests to disclose.
Data Availability statement
The data supporting the findings of this study are available from the corresponding author upon reasonable request.
Supplemental data
Supplemental data for this article can be accessed online at https://doi.org/10.1080/21505594.2023.2254599
Additional information
Funding
References
- Arciola CR, Campoccia D, Montanaro L. Implant infections: adhesion, biofilm formation and immune evasion. Nat Rev Microbiol. 2018;16(7):397–16. doi: 10.1038/s41579-018-0019-y
- Seebach E, Kubatzky KF. Chronic implant-related bone infections-can immune modulation be a therapeutic strategy? Front Immunol. 2019;10:1724. doi: 10.3389/fimmu.2019.01724
- Gries CM, Kielian T. Staphylococcal biofilms and immune polarization during prosthetic joint infection. J Am Acad Orthop Surg. 2017;25(Suppl 1):S20–S4. doi: 10.5435/JAAOS-D-16-00636
- Yamada KJ, Kielian T. Biofilm-leukocyte cross-talk: impact on immune polarization and immunometabolism. J Innate Immun. 2018;11(3):1–9. doi: 10.1159/000492680
- Resch A, Leicht S, Saric M, et al. Comparative proteome analysis of Staphylococcus aureus biofilm and planktonic cells and correlation with transcriptome profiling. Proteomics. 2006;6(6):1867–1877. doi: 10.1002/pmic.200500531
- Seebach E, Elschner T, Kraus FV, et al. Bacterial and metabolic factors of staphylococcal planktonic and biofilm environments differentially regulate macrophage immune activation. Inflammation. 2023;46(4):1512–1530. doi: 10.1007/s10753-023-01824-3
- Seebach E, Kraus FV, Elschner T, et al. Staphylococci planktonic and biofilm environments differentially affect osteoclast formation. Inflamm Res. 2023;72(7):1465–1484. doi: 10.1007/s00011-023-01745-9
- Honda K, Taniguchi T. Irfs: master regulators of signalling by toll-like receptors and cytosolic pattern-recognition receptors. Nat Rev Immunol. 2006;6(9):644–658. doi: 10.1038/nri1900
- Rehwinkel J, Gack MU. RIG-I-like receptors: their regulation and roles in RNA sensing. Nat Rev Immunol. 2020;20(9):537–551. doi: 10.1038/s41577-020-0288-3
- Liu S, Cai X, Wu J, et al. Phosphorylation of innate immune adaptor proteins MAVS, STING, and TRIF induces IRF3 activation. Science. 2015;347(6227):aaa2630. doi: 10.1126/science.aaa2630
- Ishikawa H, Barber GN. STING is an endoplasmic reticulum adaptor that facilitates innate immune signalling. Nature. 2008;455(7213):674–678. doi: 10.1038/nature07317
- Marinho FV, Benmerzoug S, Oliveira SC, et al. The Emerging roles of STING in bacterial infections. Trends Microbiol. 2017;25(11):906–918. doi: 10.1016/j.tim.2017.05.008
- Burdette DL, Monroe KM, Sotelo-Troha K, et al. STING is a direct innate immune sensor of cyclic di-GMP. Nature. 2011;478(7370):515–518. doi: 10.1038/nature10429
- Krasteva PV, Sondermann H. Versatile modes of cellular regulation via cyclic dinucleotides. Nat Chem Biol. 2017;13(4):350–359. doi: 10.1038/nchembio.2337
- Valentini M, Filloux A. Biofilms and cyclic di-GMP (c-di-GMP) signaling: lessons from Pseudomonas aeruginosa and other bacteria. J Biol Chem. 2016;291(24):12547–12555. doi: 10.1074/jbc.R115.711507
- Park S, Sauer K. Controlling biofilm development through cyclic di-GMP signaling. Adv Exp Med Biol. 2022;1386:69–94. doi: 10.1007/978-3-031-08491-1_3
- Sun L, Wu J, Du F, et al. Cyclic GMP-AMP synthase is a cytosolic DNA sensor that activates the type I interferon pathway. Science. 2013;339(6121):786–791. doi: 10.1126/science.1232458
- Wu J, Sun L, Chen X, et al. Cyclic GMP-AMP is an endogenous second messenger in innate immune signaling by cytosolic DNA. Science. 2013;339(6121):826–830. doi: 10.1126/science.1229963
- Motwani M, Pesiridis S, Fitzgerald KA. DNA sensing by the cGAS–STING pathway in health and disease. Nat Rev Genet. 2019;20(11):657–674. doi: 10.1038/s41576-019-0151-1
- Tanaka Y, Chen ZJ. STING specifies IRF3 phosphorylation by TBK1 in the cytosolic DNA signaling pathway. Sci Signal. 2012;5(214):ra20. doi: 10.1126/scisignal.2002521
- Hopfner KP, Hornung V. Molecular mechanisms and cellular functions of cGAS–STING signalling. Nat Rev Mol Cell Biol. 2020;21(9):501–521. doi: 10.1038/s41580-020-0244-x
- Barber GN. STING: infection, inflammation and cancer. Nat Rev Immunol. 2015;15(12):760–770. doi: 10.1038/nri3921
- Ahn J, Barber GN. STING signaling and host defense against microbial infection. Exp Mol Med. 2019;51(12):1–10. doi: 10.1038/s12276-019-0333-0
- Zhang Z, Zhou H, Ouyang X, et al. Multifaceted functions of STING in human health and disease: from molecular mechanism to targeted strategy. Signal Transduct Target Ther. 2022;7(1):394. doi: 10.1038/s41392-022-01252-z
- Liu N, Pang X, Zhang H, et al. The cGAS-STING pathway in bacterial infection and bacterial immunity. Front Immunol. 2021;12:814709. doi: 10.3389/fimmu.2021.814709
- Liu ZZ, Chen W, Zhou CK, et al. Stimulator of interferon genes (STING) promotes staphylococcus aureus-induced extracellular traps formation via the ROS-ERK signaling pathway. Front Cell Dev Biol. 2022;10:836880. doi: 10.3389/fcell.2022.836880
- Liu ZZ, Yang YJ, Zhou CK, et al. STING contributes to host defense against Staphylococcus aureus pneumonia through suppressing necroptosis. Front Immunol. 2021;12:636861. doi: 10.3389/fimmu.2021.636861
- Scumpia PO, Botten GA, Norman JS, et al. Opposing roles of toll-like receptor and cytosolic DNA-STING signaling pathways for Staphylococcus aureus cutaneous host defense. PLOS Pathog. 2017;13(7):e1006496. doi: 10.1371/journal.ppat.1006496
- Yamada KJ, Kielian T. Biofilm-leukocyte cross-talk: impact on immune polarization and immunometabolism. J Innate Immun. 2019;11(3):280–288. doi: 10.1159/000492680
- Heim CE, Bosch ME, Yamada KJ, et al. Lactate production by Staphylococcus aureus biofilm inhibits HDAC11 to reprogramme the host immune response during persistent infection. Nat Microbiol. 2020;5(10):1271–1284. doi: 10.1038/s41564-020-0756-3
- Gries CM, Bruger EL, Moormeier DE, et al. Cyclic di-AMP released from Staphylococcus aureus biofilm induces a macrophage type I interferon response. Infect Immun. 2016;84(12):3564–3574. doi: 10.1128/IAI.00447-16
- Gillaspy AF, Hickmon SG, Skinner RA, et al. Role of the accessory gene regulator (agr) in pathogenesis of staphylococcal osteomyelitis. Infect Immun. 1995;63(9):3373–3380. doi: 10.1128/IAI.63.9.3373-3380.1995
- Raschke WC, Baird S, Ralph P, et al. Functional macrophage cell lines transformed by Abelson leukemia virus. Cell. 1978;15(1):261–267. doi: 10.1016/0092-8674(78)90101-0
- Ishihara Y, Hyodo M, Hayakawa Y, et al. Effect of cyclic bis(3â?²â??5â?²)diguanylic acid and its analogs on bacterial biofilm formation. FEMS Microbiol Lett. 2009;301(2):193–200. doi: 10.1111/j.1574-6968.2009.01825.x
- Gundogdu MS, Liu H, Metzdorf D, et al. The haematopoietic GTPase RhoH modulates IL3 signalling through regulation of STAT activity and IL3 receptor expression. Mol Cancer. 2010;9(1):225. doi: 10.1186/1476-4598-9-225
- Cheung GYC, Bae JS, Otto M. Pathogenicity and virulence of Staphylococcus aureus. Virulence. 2021;12(1):547–569. doi: 10.1080/21505594.2021.1878688
- Archer NK, Mazaitis MJ, Costerton JW, et al. Staphylococcus aureus biofilms: properties, regulation, and roles in human disease. Virulence. 2011;2(5):445–459. doi: 10.4161/viru.2.5.17724
- Cheung AL, Schmidt K, Bateman B, et al. SarS, a SarA homolog repressible by agr, is an activator of protein a synthesis in Staphylococcus aureus. Infect Immun. 2001;69(4):2448–2455. doi: 10.1128/IAI.69.4.2448-2455.2001
- Zhou Y, Zhao S, Gao X, et al. Staphylococcus aureus induces IFN-β production via a CARMA3-independent mechanism. Pathogens. 2021;10(3):300. doi: 10.3390/pathogens10030300
- Valle J, Solano C, Garcia B, et al. Biofilm switch and immune response determinants at early stages of infection. Trends Microbiol. 2013;21(8):364–371. doi: 10.1016/j.tim.2013.05.008
- Beenken KE, Dunman PM, McAleese F, et al. Global gene expression in Staphylococcus aureus biofilms. J Bacteriol. 2004;186(14):4665–4684. doi: 10.1128/JB.186.14.4665-4684.2004
- Tamayo R, Pratt JT, Camilli A. Roles of cyclic diguanylate in the regulation of bacterial pathogenesis. Annu Rev Microbiol. 2007;61(1):131–148. doi: 10.1146/annurev.micro.61.080706.093426
- Martin FJ, Gomez MI, Wetzel DM, et al. Staphylococcus aureus activates type I IFN signaling in mice and humans through the Xr repeated sequences of protein A. J Clin Invest. 2009;119:1931–1939. doi: 10.1172/jci35879
- Parker D, Planet PJ, Soong G, et al. Induction of type I interferon signaling determines the relative pathogenicity of Staphylococcus aureus strains. PLOS Pathog. 2014;10(2):e1003951. doi: 10.1371/journal.ppat.1003951
- Zhou CM, Wang B, Wu Q, et al. Identification of cGAS as an innate immune sensor of extracellular bacterium Pseudomonas aeruginosa. iScience. 2021;24(1):101928. doi: 10.1016/j.isci.2020.101928
- Mosallanejad K, Kagan JC. Control of innate immunity by the cGAS-STING pathway. Immunol Cell Biol. 2022;100(6):409–423. doi: 10.1111/imcb.12555
- Dalpke A, Frank J, Peter M, et al. Activation of toll-like receptor 9 by DNA from different bacterial species. Infect Immun. 2006;74(2):940–946. doi: 10.1128/IAI.74.2.940-946.2006
- Hacker H, Mischak H, Miethke T, et al. CpG-DNA-specific activation of antigen-presenting cells requires stress kinase activity and is preceded by non-specific endocytosis and endosomal maturation. EMBO J. 1998;17(21):6230–6240. doi: 10.1093/emboj/17.21.6230
- Lee HC, Chathuranga K, Lee JS. Intracellular sensing of viral genomes and viral evasion. Exp Mol Med. 2019;51(12):1–13. doi: 10.1038/s12276-019-0299-y
- Yu L, Liu P. Cytosolic DNA sensing by cGAS: regulation, function, and human diseases. Signal Transduct Target Ther. 2021;6(1):170. doi: 10.1038/s41392-021-00554-y
- Kovarik P, Castiglia V, Ivin M, et al. Type I Interferons in bacterial infections: a balancing act. Front Immunol. 2016;7:652. doi: 10.3389/fimmu.2016.00652
- Boxx GM, Cheng G. The roles of type I interferon in bacterial infection. Cell Host Microbe. 2016;19(6):760–769. doi: 10.1016/j.chom.2016.05.016
- Kaplan A, Ma J, Kyme P, et al. Failure to induce IFN-β production during Staphylococcus aureus infection contributes to pathogenicity. J Immunol. 2012;189(9):4537–4545. doi: 10.4049/jimmunol.1201111
- Xiong Q, Zhang L, Ge W, et al. The roles of interferons in osteoclasts and osteoclastogenesis. Joint Bone Spine. 2016;83(3):276–281. doi: 10.1016/j.jbspin.2015.07.010
- Heim CE, Vidlak D, Kielian T. Interleukin-10 production by myeloid-derived suppressor cells contributes to bacterial persistence during Staphylococcus aureus orthopedic biofilm infection. J Leukocyte Biol. 2015;98(6):1003–1013. doi: 10.1189/jlb.4VMA0315-125RR