ABSTRACT
The ubiquity of methicillin-resistant Staphylococcus aureus (MRSA) and the mounting prevalence of antibiotic resistance necessitate the identification of novel therapeutic approaches to reduce the selective pressure of antibiotics. Targeting bacterial virulence factors, such as the pivotal Sortase A (SrtA) in S. aureus for adhesion and invasion, and the salient toxin α-Hemolysin (Hla), offers a sophisticated approach to attenuate pathogenicity without bacterial elimination. Herein, we report the discovery of a flavonoid, isosakuranetin, which inhibits the activity of S. aureus SrtA. A fluorescence resonance energy transfer assay revealed that isosakuranetin exhibited a low IC50 of 21.20 μg/mL. Furthermore, isosakuranetin significantly inhibited SrtA-related virulence properties, such as bacterial adhesion to fibrinogen, biofilm formation, and invasion of A549 cells. We employed fluorescence quenching and molecular docking to determine the interactions between isosakuranetin and SrtA, revealing the key amino acid sites for binding. Importantly, isosakuranetin inhibited the haemolytic activity of S. aureus in vitro at a concentration of 32 μg/mL. Moreover, isosakuranetin effectively suppressed the transcription and expression of Hla in a dose-dependent manner and regulated the transcription of RNAIII, the upstream operator of Hla. Notably, isosakuranetin demonstrated in vivo efficacy in a mouse model of S. aureus-induced pneumonia by significantly improving survival rates and reducing lung damage. This is a valuable finding, as isosakuranetin’s dual inhibitory effects on SrtA and haemolytic activity, as well as its anti-virulence activity against MRSA, make it an excellent candidate for therapeutic development.
Introduction
Staphylococcus aureus (S. aureus), particularly highly invasive methicillin-resistant S. aureus (MRSA), has reached pandemic proportions worldwide, including in hospitals and community populations [Citation1]. MRSA possesses the ability to colonize a wide spectrum of host tissues, ranging from the skin and nasal cavity to more internal structures such as bones, joints, and muscles and extending to critical organs such as the heart and lungs. Such extensive colonization can precipitate a life-threatening scenario, potentially leading to mortality [Citation2]. Additionally, MRSA is one of the important pathogens causing mastitis in dairy cows, which causes huge economic losses in the livestock industry [Citation3].
Antibiotics seem to be a “double-edged sword,” as they can control S. aureus infections while also posing the new challenge of drug resistance [Citation4]. If these tendencies do not even change when the post-antibiotic era begins, preventative measures against the most dangerous infections may become scarce [Citation5,Citation6]. Researchers have investigated various strategies, including the use of direct bactericidal agents such as antimicrobial peptides, nanoparticles or even antimicrobial adjuvants that target the mechanisms of resistance to existing antibiotics, effectively increasing bacterial susceptibility to the drug and restoring clinical efficacy [Citation7–9]. An alternative strategy targeting pathogen virulence factors has aroused interest. It does not affect the growth or viability of bacteria, so it does not impose strong selection pressures on bacteria in favour of the evolution of resistance and persistence mechanisms and avoids the disadvantage of antibiotics in disrupting the beneficial flora [Citation10–12].
The incidence and severity of S. aureus infection depend on various virulence factors, which help evade the host’s immune system and interfere with bacterial pathogenicity in various ways [Citation13]. As virulence expression is a highly coordinated process involving multiple transcription factors, targeting only a single virulence-related pathway may upregulate the expression of other virulence factors. In contrast, targeting multiple virulence factors seems to have a more significant advantage. In this study, we focused on the virulence factors SrtA and Hla of S. aureus.
Numerous gram-positive bacteria with low G+C contents have the transpeptidase SrtA, which is involved in a wide range of physiological functions [Citation14,Citation15]. It cleaves the polypeptide between the threonine and glycine of the LPXTG motif and catalyzes the formation of amide bonds between the amino groups of the carboxyl group of the threonine and amino groups of the peptidoglycan cross-bridge, which ultimately anchors proteins to the cell wall [Citation16–18]. For example, some surface proteins of S. aureus, such as clumping factors (ClfA, ClfB), fibronectin-binding proteins (FnbA, FnbB) and surface protein A (SpA), need to be transpeptidated and anchored to the cell wall for further function, which requires the assistance of SrtA [Citation17,Citation19]. The SrtA knockout mutant of S. aureus was shown to cause defective display of various LPXTG motif proteins and exhibited significantly reduced pathogenicity in mouse kidney abscess and acute infection models [Citation20,Citation21]. In summary, SrtA plays an irreplaceable role in bacterial adherence, host invasion, immune evasion, and biofilm formation. In addition, it does not share homology with eukaryotes, which makes it an attractive virulence target for anti-MRSA treatment [Citation22].
The adherence and invasion of bacteria to the host is the initial stage of bacterial pathogenesis and reducing the secretion of toxins is also a strategy to fight MRSA from the perspective of anti-virulence. Hla, a pore-forming toxin, is recognized as a major virulence determinant in S. aureus [Citation23]. Many S. aureus strains can produce Hla. It has no killing effect on human neutrophils [Citation24], but it can lyse other immune cells, such as macrophages, red blood cells and lymphocytes [Citation25]. In addition, it promotes the effects of neutrophil influx into infected lungs and neutrophil adhesion to endothelial cells, contributing to inflammation [Citation26,Citation27]. This process is based on Hla binding to the ADAM10 receptor, which oligomerizes in the cell membrane and forms holes in the host cell membrane, triggering cell death or regulating cytokine production and proliferation [Citation28,Citation29]. It has been shown that Hla expression is directly associated with the virulence of S. aureus, and immunization strategies against Hla have been shown to be effective in mouse models of S. aureus pneumonia, as well as in soft tissue infection [Citation30]. The crucial role of Hla in S. aureus has become recognized as a major virulence target for MRSA treatment.
In our experiments, FRET and haemolysis assays revealed that isosakuranetin (), a flavonoid derived from Citrus bergamia fruit, could effectively interfere with SrtA and the haemolytic activity of S. aureus. Isosakuranetin has also been reported to stimulate melanogenesis in B16BL6 mouse melanoma cells [Citation31] and its anti-analgesic effect in a rat model of peripheral neuropathy [Citation32]. However, the effects of isosakuranetin on bacterial aspects have not been reported. Here, we focused on validating the anti-SrtA and haemolytic effects of isosakuranetin and elucidating the underlying mechanism. The protective effect of isosakuranetin in a mouse model of pneumonia was also evaluated in vivo. Overall, these positive results suggest that isosakuranetin, as a dual inhibitor, is promising as a therapeutic candidate for MRSA infection.
Figure 1. Identification of isosakuranetin as a dual inhibitor of SrtA and Hla in S. aureus (a) First, SrtA inhibitors were screened from natural products. The FRET method was used to screen inhibitors based on the fluorescence changes in the LPXTG motif recognized and cleaved by SrtA and to screen for SrtA inhibitors (right side of Figure 1A). Subsequently, hemolysis assays were further utilized to finally obtain inhibitors that could inhibit both SrtA and hemolytic activity. (b) The chemical structure of isosakuranetin. (c) Isosakuranetin inhibited SrtA activity in a dose-dependent manner with a calculated IC50 of 21.20 μg/mL. (d) Reversible assay confirmed that isosakuranetin was a reversible inhibitor of SrtA. Untreated SrtA was used as a control. (e) Isosakuranetin suppressed the hemolytic activity of S. aureus USA300 in a dose-dependent manner. n.s. indicates P>0.05; **P < 0.01, *** P < 0.001. The IC50 was determined using the variable slope model in GraphPad, which plots the logarithm of the inhibitor concentration against the normalized response. Hemolysis inhibition rates were rigorously assessed via one-way ANOVA.
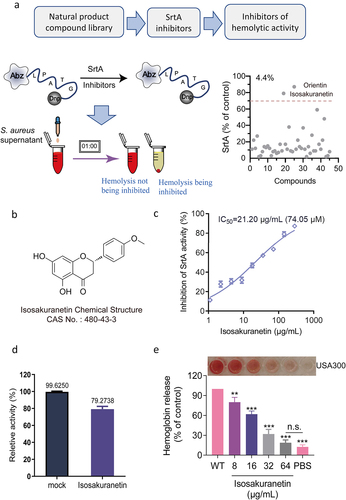
Materials and methods
Bacterial strains and growth conditions
S. aureus USA300 was provided by the ATCC and stored in tryptic soy broth (TSB) with 20% glycerol at −80 °C. Typically, bacteria were cultured in nutrient broth at 37 °C with shaking at 220 rpm/min and incubated overnight for downstream assays. Escherichia coli (E. coli) DH5α and BL21 (DE3) were grown on Luria – Bertani broth or agar at 37 °C. Kanamycin (50 μg/mL) was used for plasmid selection. A549 cells were grown in 1640 medium (Servicebio, Wuhan, China) containing 10% foetal bovine serum and 1% penicillin-streptomycin (Thermo Fisher Scientific). Cells were grown at 37 °C and 5% CO2 for 24 h.
Reagents
Isosakuranetin (purity >98%), a flavonoid, was purchased from Pufei De Biotech Co., Ltd. (Chengdu, China). A peptide substrate, suih, was purchased from LifeTein (Beijing, China). Rabbit anti-SrtA and anti-Hla polyclonal antibodies were made in the laboratory and provided by Sigma, respectively.
Expression and purification of the SrtA protein
The prokaryotic expression vector pET-28a was used for the cloning procedure in this experiment. The pET-28a-srtA plasmid was constructed by using the genomic DNA of S. aureus USA300 as a template and the primers presented in Table 1S. The SrtA fragment with a restriction site was amplified based on polymerase chain reaction (PCR). The PCR product was digested with NdeI and BamHI before being ligated into the pET-28a vector to produce pET28a-srtA. The recombinant plasmid was transferred to E. coli DH5α by the heat shock method. After sequencing, it was transferred to the host E. coli Bl21 by the same method. When E. coli containing the recombinant plasmid was cultured to an OD600 of approximately 0.8, isopropyl β-D-thiogalactoside (IPTG, 0.5 mM) was added to induce SrtA expression. Subsequently, a nickel column was used to purify the SrtA protein as described previously [Citation15], and a solution containing a low concentration of imidazole was used to wash off the miscellaneous proteins. SrtA protein was eluted at a high concentration of imidazole. The mutation vector was constructed and purified in accordance with the SrtA protein, and the relevant primers are shown in Supplementary Table 1.
Dual inhibition screening of SrtA and haemolytic activity
FRET assays were employed to screen for SrtA inhibitors, utilizing the specific ability of SrtA to cleave the substrate peptide Abz-LPATG-Dap (Dnp)-NH2. A natural small molecule library containing 45 flavonoids was screened. Specifically, the reaction system was comprised of a 100 μL volume, initially containing compounds at a concentration of 64 μg/mL and SrtA protein at 5 μM, followed by incubation at 37 °C for 1 hour. Subsequently, 10 μM fluorescent substrate peptide was added, and further incubation continued for 20 minutes, after which the fluorescence intensity at excitation and emission wavelengths of 309 nm and 420 nm was measured. Eriodictyol served as a positive control [Citation33]. Compounds exhibiting > 70% inhibition of SrtA activity were selected as candidate compounds for further analysis of their inhibition of haemolysis.
Determination of minimum inhibitory concentration (MIC) and growth curves
Details of the methods for the determination of the minimum inhibitory concentration (MIC) and growth curves are described in the Supplementary Material.
SrtA activity measurement
The reaction milieu, with compound concentrations ranging from 0.5 to 128 μg/mL in a total volume of 100 μL, was subjected to incubation alongside a final concentration of 5 μM SrtA for one hour at a temperature of 25 °C. After this, the reaction buffer was supplemented to a final concentration of 10 μM fluorescent substrate. After incubation for 20 min, the fluorescence intensity was determined with excitation and emission wavelengths at 309 and 420 nm, respectively. The inhibition rate of the compound at the concentration was calculated by GraphPad Prism software, and the IC50 value was determined by the dose-response relationship curve [Citation15]. Three repeated measurements were performed for each data point.
Reversible inhibition assay
Details of the reversible inhibition assay are described in the Supplementary Material.
Hemolysis assay
RBCs were washed three times with PBS before use and resuspended in PBS. S. aureus overnight cultures were given fresh TSB medium at a 1:100 ratio along with isosakuranetin, and the process was carried out at 37 °C until late logarithmic growth. The ability of S. aureus to destroy erythrocytes after drug treatment was assessed by collecting the bacterial supernatant. The reaction system consisted of 25 μL of red blood cell solution, 100 μL of bacterial supernatant, and 875 μL of PBS. After incubation at 37 °C for 1 h in an incubator, the supernatant was collected by low-speed centrifugation, and the absorbance at 543 nm was measured.
Adherence of S. aureus to immobilized fibrinogen
Adhesion assays measuring S. aureus binding to fibrinogen were performed on 96-well polystyrene plates. The plates were pretreated by adding 400 ng of fibrinogen to each well and incubating for 2 h at 37 °C. Overnight S. aureus cultures were diluted 1:100 and incubated with various concentration of isosakuranetin (0–64 μg/mL). After overnight incubation, the bacteria were collected and resuspended in PBS to an OD600 of 1.0. The pretreated plates were filled with the above bacterial solution and incubated at 37 °C for 2 h. After removing the solution, the wells were cleaned three times with PBS and then fixed for an hour at room temperature with 200 μL of glutaraldehyde (2% v/v in PBS). After conventional staining with crystal violet (0.5% v/v), the bacteria were gently rinsed with PBS instead of crystal violet, dried, and the absorbance was measured at 595 nm.
Cell invasion assay
Details of the cell invasion assay are described in the Supplementary Material.
Crystal violet biofilm assay
S. aureus overnight cultures were diluted 1:100 in fresh BHI supplemented with 0.5% glucose in a 96-well polystyrene microplate. In each well, 200 μL of the above solution and different concentrations of isosakuranetin were added and incubated at 37 °C for 18 h. Subsequently, the biofilm was stained with 0.1% (w/v) crystal violet solution for 15 min. Excess staining solution was decanted and the samples were rinsed 3 times with sterile distilled water and photographed. For quantification, the absorbance was measured at 595 nm with isopropyl alcohol-resuspended crystal violet stain.
FITC-IgG binding to staphylococci
Different concentrations of isosakuranetin were added to S. aureus at an initial OD600 of 0.1 and grown to an OD600 of 1.0 at 37 °C with shaking. Bacteria were collected by low-speed centrifugation and resuspended in appropriate PBS, followed by the addition of 4 μL FITC-conjugated rabbit IgG control polyclonal antibody (Proteintech, Wuhan, China) and incubation at room temperature in the dark for 20 min. Bacteria were collected again, washed with sterile PBS and resuspended. A microplate reader was used to measure the fluorescence intensity at wavelengths of 495 nm for emission and 520 nm for excitation (Tecan, Switzerland).
Neutralization assay
Defibrinated rabbit blood was pretreated with PBS before use. S. aureus overnight cultures were diluted 1:100 in fresh TSB medium and grown until late logarithmic growth. The neutralization assay reaction system included 100 μL of S. aureus supernatant, different concentrations of isosakuranetin (10 μL, 8 to 64 μg/mL), 25 μL of pretreated defibrinated rabbit blood, and 865 μL of PBS buffer. The above-mixed solutions were incubated at 37 °C for 1 h. Subsequently, the degree of erythrocyte disruption was assessed by low-speed centrifugation and collection of the supernatant, usually by measuring the absorbance at 543 nm.
Quantitative real-time PCR
Details of quantitative real-time PCR are described in the Supplementary Material.
Western blotting for SrtA
Overnight cultures of S. aureus USA300 at a ratio of 1:100 were inoculated into fresh TSB medium, and different concentrations of isosakuranetin were added. After 12 h, bacteria were collected by centrifugation, suspended in PBS supplemented with 1 μL of 4 mg/mL lysostaphin, and incubated at 37 °C for 30 min. After sonication, the supernatant was collected. S. aureus overnight cultures were diluted at a 1:100 ratio and kept until late logarithmic growth for the haemolysis assay. Supernatants of the cultures were then collected for subsequent assays.
Proteins were then transferred to PVDF membranes using a semidry membrane transfer device after the samples were separated using 10% SDS-PAGE. After blocking with 3% BSA, antibody incubation was performed with rabbit anti-SrtA antibody (laboratory-made 1:200) and HRP-labelled goat anti-rabbit secondary antibody (1:500). After washing the membrane, ECL chromogenic solution was dropped onto the membrane, and the immune bands were observed under a chemiluminescence imager. ImageJ was used for greyscale analysis.
Western blotting for Hla
S. aureus cultures, initialized at an OD600 of 0.3, were treated with varying concentrations of isosakuranetin, ranging from 8 to 64 μg/mL, and subsequently maintained under oscillatory cultivation until an OD600 of 2.5 was reached. The supernatants were collected post-centrifugation, with an equivalent volume of each sample (20 μL) subjected to 12% SDS-PAGE separation. Following conventional transfer onto membranes, the samples were blocked using a 3% solution of BSA. The membranes were then incubated at 4 °C for 2 hours with a rabbit anti-Hla antibody (Sigma, at a dilution of 1:3000) and a secondary antibody, goat anti-rabbit HRP-IgG (at a dilution of 1:5000). After secondary washing of the membranes, bands were visualized using an ECI-developing solution, and their intensities were subsequently analysed via ImageJ based on greyscale quantification.
In silico docking of SrtA
The 3D structure of isosakuranetin was provided by PubChem (PubChem ID: 160481) and subsequently docked to the SrtA crystal structure (PDB ID: 1T2P). The parameters used for molecular docking were exhaustiveness = 8, and the grid boxes were manually adjusted to center_x = −31.45, center_y = −19.71, center_z = 9.00; size_x = 30.0, size_y = 30.0, and size_z = 30.0. Then, AutoDock Vina1.57 was run for docking [Citation34], and PyMOL 2.3 was used to visualize the docking results.
Fluorescence quenching assay
Details of the fluorescence quenching assays are described in the Supplementary Material.
Mouse pneumonia infection model
S. aureus overnight cultures were diluted 1:100 into sterile TSB broth and allowed to continue growing until the OD600 value reached 1.0. Then, the bacterial precipitates were collected by low-speed centrifugation, washed three times in PBS and resuspended in saline.
Inbred C57BL/6J male mice at 8 weeks of age (20–22 g) were obtained from Liaoning Changsheng Biological Company. For lethal challenge and treatment experiments, C57BL/6 mice were anesthetized with ether and then infected with 2 × 108 CFU of S. aureus USA300 or ΔsrtA via intranasal inoculation. A single dose of isosakuranetin 50 mg/kg (n = 10) or an equal volume of saline was administered subcutaneously 1 h after infection [Citation33]. Mice were injected subcutaneously with isosakuranetin every 12 h, and survival was assessed at intervals of 12 to 96 h [Citation35]. Given the mouse’s average weight (20 g), the total dose per mouse was approximately 8 mg.
To investigate the therapeutic effect of isosakuranetin on bacterial load, lung pathological changes and degree of injury in a model of pneumonia, C57BL/6 mice were grouped in a manner and administered doses consistent with the lethality assay. Mice in each group were given 1 × 108 CFU of S. aureus USA300 or ΔsrtA. Isosakuranetin was administered by subcutaneous injection every 12 h after intranasal infection, and mice were euthanized after 48 h. Thus, four doses were given, amounting to an approximate total of 4 mg of isosakuranetin per mouse. The staphylococcal burden was subsequently calculated by spotting serial dilutions in 0.9% saline on BHI agar plates after a part of the right lung was removed using an aseptic procedure, weighed, and homogenized. The left lung of mice from each group was routinely processed with formalin and stained with haematoxylin and eosin (H&E); lung sections were imaged with a light microscope. The remaining left lung of each group was used to determine the wet-dry weight ratio of the lung to assess the degree of lung injury.
To evaluate the effect of isosakuranetin on inflammatory factors in the lungs of mice with S. aureus pneumonia, at 48 hours after infection, the bronchoalveolar lavage fluid (BALF) of mice in each group was collected, and the levels of the inflammatory factors TNF-α, IL-6, and IL-1β were determined by ELISA kits.
Statistical analysis
The results are presented as the mean ± SD unless otherwise stated. A P value of less than 0.05 indicated statistical significance. GraphPad Prism version 8.0, a statistical tool, was used for all statistical analyses in this study. Student’s t tests (two-tailed) were employed to compare two sets of data, while survival analysis was conducted using the log-rank test. Additionally, one-way ANOVA was conducted for comparison of more than two groups.
Results
Recognition of isosakuranetin as an inhibitor of SrtA and Hla in S. aureus
Based on the ability of SrtA to recognize and cleave the LPXTG motif, we first identified SrtA inhibitors by observing the changes in fluorescence intensity after drug addition by FRET assay. Given the multifaceted pharmacological properties exhibited by flavonoids, we screened 45 natural flavonoids for potential dual inhibitors. Notably, isosakuranetin and orientin emerged as remarkable candidates, demonstrating exceptional inhibition of SrtA activity (>70%) during the preliminary screening, with a notable SrtA inhibitor hit rate of 4.4% (). Subsequent investigations revealed that orientin lacked inhibitory activity against haemolysis, thereby directing our focus towards isosakuranetin as a promising candidate warranting further exploration.
FRET results revealed that isosakuranetin interfered with SrtA activity in a dose-dependent manner with an IC50 of 21.20 μg/mL (74.05 μM), exhibiting significant anti-SrtA activity (). We conducted a reversible assay to determine the mode of action of isosakuranetin on SrtA. Typically, if the recovery of SrtA activity is greater than 60%, it is considered to indicate noncovalent reversible binding [Citation36]. After incubating SrtA with isosakuranetin (10-fold IC50), 79.27 ± 3.64% of SrtA activity was recovered compared to that of the mock-treated group (), indicating that isosakuranetin functions as a reversible inhibitor that does not covalently modify the active site cysteine of SrtA.
In addition, isosakuranetin remarkably suppressed the haemolytic activity of S. aureus, exhibiting a remarkable inhibitory effect at 8 μg/mL (P < 0.01), and the haemolytic activity was only 18.84% at 64 μg/mL, which was not significantly different from that of the control group (n.s., P > 0.05) (). Isosakuranetin has the potential for further application as a dual inhibitor of SrtA and Hla.
Isosakuranetin did not interfere with the growth of S. aureus
Anti-virulence treatments may offer a way to reduce infectious disease without accelerating the emergence of a resistant phenotype. This requires that our screened anti-virulence compounds do not have antibacterial effects. To this end, we first determined the MIC of isosakuranetin against S. aureus USA300, and the results showed that the MIC of isosakuranetin was greater than 512 μg/mL (), indicating that isosakuranetin had almost no antibacterial effect. Subsequently, growth curves of S. aureus USA300 with or without isosakuranetin (32 or 64 μg/mL) treatment showed similar results. Meanwhile, 128 μg/mL and higher concentrations of isosakuranetin interfered with the growth of S. aureus USA300, and 512 μg/mL completely inhibited the growth of S. aureus USA300 (). In addition, haemoglobin release assays and acute toxicity assays in mice confirmed that isosakuranetin is virtually non-toxic (Figure 2S).
Figure 2. Isosakuranetin does not interfere with the growth of S. aureus USA300 (a) The minimum inhibitory concentration of isosakuranetin against S. aureus USA300 is greater than 512 μg/mL based on the multiplicative dilution method. (b) Growth curves of S. aureus USA300 in the presence or absence of isosakuranetin (32 or 64 μg/mL). The ΔsrtA strain without any treatment served as a control. n.s. indicates P>0.05. The statistical analysis of the MIC data was rigorously conducted using one-way ANOVA.
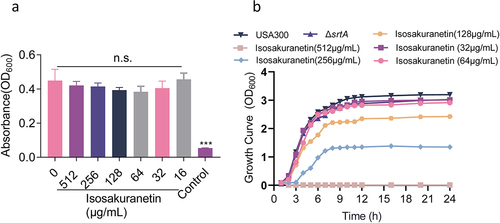
Isosakuranetin suppresses SrtA-related virulence phenotypes
Since SrtA has been proven to be associated with bacterial adhesion and invasion, we first evaluated whether isosakuranetin affects the adhesion of S. aureus to fibrinogen. As expected, the ability of S. aureus to adhere to fibrinogen decreased with increasing isosakuranetin concentrations in a dose-dependent manner. In the presence of 64 μg/mL isosakuranetin, the adhesion rate was only 31.85% ().
Figure 3. Isosakuranetin inhibition of SrtA and related virulence phenotypes of S. aureus (a) Isosakuranetin inhibited the adhesion of S. aureus USA300 to fibrinogen. (b) Isosakuranetin inhibition of S. aureus USA300 invasion into A549 cells. A549 cells were infected with S. aureus pretreated with different concentrations of isosakuranetin and lysed 2 hours after infection. The invasion of intracellular S. aureus was determined by serial dilution on TSB agar plates. (c) Effect of isosakuranetin on biofilm formation of S. aureus. Biofilm formation was quantified by crystal violet (CV) staining. (d) IgG could specifically bind to S. aureus surface protein (SpA), and FITC-labeled IgG was used to detect the fluorescence change to evaluate the effect of isosakuranetin on the SpA anchoring of the S. aureus cell wall. * P < 0.05, ** P < 0.01, *** P < 0.001. All experimental data depicted in this figure were analyzed using GraphPad, with statistical assessments conducted via one-way ANOVA.
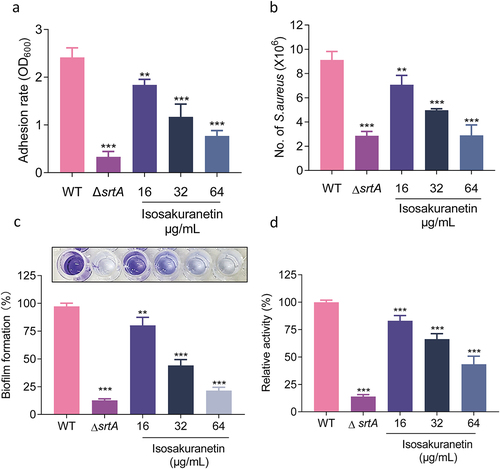
Subsequently, we performed an A549 cell invasion assay and measured the replication of invading S. aureus after treating A549 cells with gentamicin to eliminate the remaining extracellular bacteria. As expected, the addition of isosakuranetin resulted in a significant decrease in the number of S. aureus that invaded the cells ().
Biofilms, a thin layer of microbes that can attach to almost any surface, are associated with drug resistance [Citation37]. Deletion of SrtA has been reported to interfere with biofilm formation ability [Citation17]. Subsequently, we further evaluated whether isosakuranetin inhibited biofilm formation by crystal violet staining assay. In the control group, obvious biofilm formation was observed. However, biofilm formation gradually decreased with increasing concentrations of isosakuranetin. In the presence of 64 μg/mL isosakuranetin, the biofilm formation rate was only 21.49% (). The SrtA group exhibited the lowest adhesion rate of only 12.69% compared to the WT group.
SrtA affects a range of surface proteins that anchor to the cell wall, including SpA, an important virulence factor [Citation38]. SpA can bind to the Fcγ and Fab domains of host immunoglobulin, so the FITC-IgG antibody can roughly reflect the amount of SpA on the surface of the bacterial cell wall by detecting the fluorescence change. The addition of isosakuranetin caused a dose-dependent decrease in SpA, suggesting that isosakuranetin can reduce SpA anchoring to the cell wall (). Subsequently, we further explored the influence of isosakuranetin on the transcriptional profile of SpA, and the results revealed that isosakuranetin had no effect on the transcription level of S. aureus SpA (Supplementary Figure . 1).
In conclusion, isosakuranetin suppressed SrtA and its related virulence characteristics, such as fibrinogen adhesion, A549 cell invasion, biofilm formation, and SpA anchoring to the cell wall.
Isosakuranetin regulates the expression of Hla through the Agr system
To further reveal the mechanism by which isosakuranetin inhibited Hla, a neutralization assay was performed. Different concentrations of isosakuranetin were added to S. aureus to observe the rupture of erythrocytes. With increasing isosakuranetin concentration, erythrocyte rupture was not inhibited, indicating that isosakuranetin does not have neutralizing haemolytic activity, which means that isosakuranetin does not directly interact with Hla ().
Figure 4. Isosakuranetin regulates the expression of Hla through the agrA system. (a) Isosakuranetin did not affect the neutralizing activity of Hla in S. aureus. (b) Western blotting results showed that isosakuranetin reduced the expression of Hla in the supernatant of S. aureus in a dose-dependent manner. (c) qPCR revealed that isosakuranetin inhibited the transcription of Hla and (d) RNAIII in S. aureus in a dose-dependent manner. * P < 0.05, *** P < 0.001. All experimental data incorporated in this figure were analyzed using GraphPad, with statistical assessments conducted via one-way ANOVA. Grayscale analysis of western blotting results was performed utilizing ImageJ.
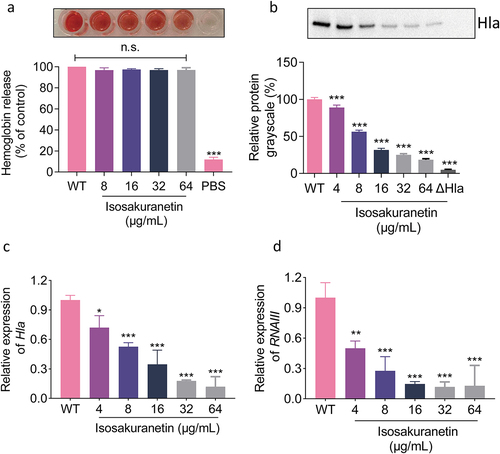
In addition to directly binding to Hla to affect haemolytic activity, molecules can also affect the upstream system of Hla to affect its transcription and translation and interfere with its haemolytic activity. Subsequently, western blotting assays were performed to evaluate the effect of isosakuranetin on Hla expression in S. aureus. When S. aureus was treated with 64 μg/mL isosakuranetin, the level of Hla expression was only 14.57% compared to that of the untreated group (). Similarly, consistent results were observed by qPCR, and the Hla transcription level was significantly decreased after isosakuranetin treatment (). Hla is mainly regulated by the Agr system. To this end, we determined the transcription level changes of RNAIII, a direct regulator of Hla, by qPCR. RNAIII transcript levels were significantly decreased in isosakuranetin-treated S. aureus, with a 7.75-fold decrease at 64 μg/mL ().
In conclusion, our results suggest that isosakuranetin did not affect haemolysis by directly binding to Hla but may inhibit haemolysis by interfering with the Agr system to regulate the expression of the downstream Hla gene.
Direct interaction between isosakuranetin and SrtA
Based on previous findings, we first determined whether isosakuranetin affected the expression of SrtA. S. aureus was treated with different concentrations of isosakuranetin (0–64 µg/mL) for total protein extraction. Subsequently, SrtA expression levels were determined by western blotting, and the data were quantified by greyscale image analysis. As illustrated in , isosakuranetin did not interfere with SrtA expression.
Figure 5. Mechanistic study of the interaction between isosakuranetin and SrtA. (a) S. aureus was treated with different concentrations of isosakuranetin, and the expression level of SrtA protein was evaluated by western blotting. ClpP was used as an internal reference. (b) A fluorescence quenching assay was used to evaluate the binding affinity of isosakuranetin to SrtA. (c) Molecular docking of isosakuranetin binding to SrtA and (d) analysis of the major binding amino acid residues and interaction forces of isosakuranetin binding to SrtA. (e) The total binding free energy of isosakuranetin to SrtA was -8.4 kcal/mol. (f) SrtA and SrtA mutants were incubated with 64 μg/mL isosakuranetin, and the activity of recombinant SrtA was determined by FRET. Three independent experiments were used for analysis. The data are presented as the mean ± SD. ***P <0.001 vs. the vehicle or WT-srtA; n.s. represents no significant difference. The western blotting data were statistically analyzed via one-way ANOVA, while the reversible inhibition experiments were examined using Student’s t test.
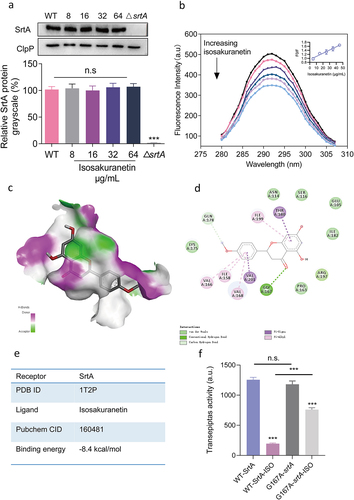
In addition, fluorescence quenching assays were used to assess the direct interaction between isosakuranetin and SrtA. SrtA (fluorescent molecule) emits fluorescence when excited by a specific wavelength of light. When isosakuranetin (quencher) binds to SrtA, it alters the fluorescence characteristics of SrtA, leading to a decrease or “quenching” of its fluorescence intensity. Since the binding of the SrtA protein is static quenching, the binding constant KA can be calculated according to the static quenching formula Lg[(F0-F)/F] = Lg KA + nlg Q. The binding constant (KA) of SrtA after the addition of isosakuranetin was calculated to be 3.88 × 104 L/mol, which is greater than 104 L/mol and is considered a strong interaction [Citation39], indicating that isosakuranetin binds to SrtA ().
To better elucidate the direct interaction between isosakuranetin and SrtA, molecular simulations were performed. As illustrated in , isosakuranetin was able to bind to the active pocket of SrtA. Isosakuranetin forms a hydrogen bond with Gly167 of the SrtA protein. The total free energy was calculated to be −8.3 kcal/mol, demonstrating significant binding between isosakuranetin and SrtA (). Informed by our molecular simulations, we introduced a point mutation at the energetically significant site, G167. We initially assessed the activity of the mutated G167 SrtA protein, which showed no marked difference from native SrtA. We then further investigated the effects of 64 μg/mL isosakuranetin on the G167A-SrtA mutant protein. Significantly, the administration of isosakuranetin to the G167A-SrtA mutant precipitated a substantial diminution in transpeptidase activity. Contrastingly, a marked disparity was still observed with the isosakuranetin-treated WT-SrtA cohort (P < 0.001). This insight suggests that G167 constitutes a pivotal binding locus for isosakuranetin on the SrtA protein, a finding in consonance with our initial postulate deduced from simulation outcomes ()
Isosakuranetin attenuates S. aureus pneumonia
S. aureus, the perfect respiratory pathogen, not only persists in the respiratory tract but also colonizes and rapidly multiplies within the lung tissue. Given the critical roles of SrtA and Hla in the pathogenesis of S. aureus pneumonia, we established a model of pneumonia infection to evaluate the in vivo anti-MRSA effect of isosakuranetin. The model of pneumonia infection was first established by intranasal administration of S. aureus (2 × 108 CFU/mL) as described in , followed by subcutaneous treatment with 50 mg/kg isosakuranetin.
Figure 6. Isosakuranetin protected mice from S. aureus-induced pneumonia. (a) An S. aureus pneumonia infection model was established by nasal drip administration of an S. aureus suspension (2×108 CFUs). Isosakuranetin was administered after infection, and the survival rate of mice within 96 h was evaluated. (b) Determination of the effect of isosakuranetin on the survival of mice (n=10) with S. aureus-induced pneumonia. (c) An S. aureus pneumonia model was established by intranasal administration of an S. aureus suspension (1×108 CFUs). One hour after infection, the mice were treated with isosakuranetin, and the lung bacterial load, wet-to-dry weight ratio, pathological changes and major inflammatory factors were evaluated after infection for 48 h. (d) Effect of isosakuranetin treatment on bacterial load in the lungs of mice (n = 6). (e) Effect of isosakuranetin treatment on the wet to dry weight ratio in the lungs of mice. (f) Histopathological changes (H&E staining) in the lungs of S. aureus-infected mice with or without isosakuranetin (50 mg/kg). (f-h) Effect of isosakuranetin on the secretion of the validation factors TNF-α, IL-1β, and IL-6 in the bronchoalveolar lavage fluid of mice in each group. Animal data were obtained by two experiments (magnification: 400×; scale bar, 50 μm). * P < 0.05, *** P < 0.001. In Fig. 6D, the lower detection limit in our study signifies the absence of discernible bacterial presence. Survival rates were determined using the Kaplan-Meier method, and survival analyses were executed employing the log-rank test. All remaining statistical analyses were rigorously conducted using ANOVA.
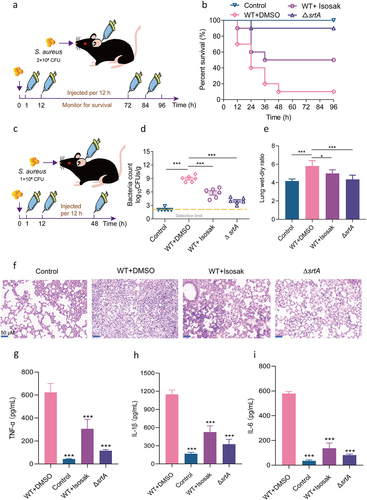
The survival rate of the mice in the infection group was only 10%, which confirmed the successful establishment of the pneumonia infection model. After treatment with 50 mg/kg isosakuranetin, the survival rate was increased by 40%, especially in the early stages of infection, indicating that isosakuranetin exhibited a significant anti-infective effect in a mouse model of acute infection with pneumonia ().
The severity of lung injury and the levels of inflammatory cytokines were evaluated by intranasal administration of isosakuranetin to mice given a sublethal concentration of S. aureus (). After isosakuranetin treatment, the bacterial load in the lungs of mice decreased from 9.36 log10 CFU/g to 6.54 log10 CFU/g. Further examination of the histopathological alterations in the lung tissues revealed that the lungs of the infected group had inflammatory cell infiltration, which was markedly reduced by isosakuranetin therapy. Similar results were also observed in the lung wet-dry weight ratio, indicating that isosakuranetin could reduce the degree of lung injury in mice with S. aureus pneumonia. We collected bronchoalveolar lavage fluid (BALF) from each group of mice and measured the levels of several important inflammatory factors. After receiving isosakuranetin therapy, the levels of TNF-, IL-1, and IL-6 drastically decreased ().
In conclusion, our results confirm the therapeutic role of isosakuranetin in a model of S. aureus-induced pneumonia.
Discussion
Tackling the antibiotic resistance crisis will require a multifaceted approach that includes improved antibiotic stewardship, the development of new antibiotics, the development of alternative therapies such as host-directed therapies and anti-virulence drugs, and the development of adjuvants to inhibit bacterial resistance mechanisms [Citation40]. The high infectivity and pathogenicity of MRSA strains have been attributed to the high expression of virulence factors encoded by key genomes, such as Hla and PSMα, which weaken host defences by interfering with immune effector cells to cause cell lysis [Citation1,Citation41,Citation42]. Virulence factors have a unique role in the pathogenesis of MRSA, and research has shown that targeting virulence factors is an effective strategy against several of the most ubiquitous and troublesome bacterial pathogens [Citation43].
Therapeutic effects can be achieved by reducing bacterial adhesion, invasion, and immune evasion or by decreasing the secretion of key pathogenic factors. SrtA and Hla of S. aureus are closely related to the above effects. Wild-type Hla is expressed extracellularly by S. aureus as a water-soluble monomer and self-assembles into a homomultimer with transmembrane domains, which disrupts the cell membrane, leading to the release of contents and cell death [Citation44,Citation45]. Hla has emerged as a promising candidate vaccine target to protect high-risk populations, especially hospitalized surgical patients, from antibiotic-resistant staphylococcal infections [Citation46]. In addition, many proteins involved in the biofilm formation, adhesion, and invasion of S. aureus are closely related to SrtA [Citation47,Citation48]. The SrtA mutant was also confirmed to be susceptible to phagocytosis by macrophages. Targeting either S. aureus Hla or SrtA has been demonstrated to reduce the pathogenicity of strains in a variety of animal infection models.
The advantage of our approach is that targeting two virulence targets at the same time, a “two-prong approach,” would theoretically yield a greater therapeutic effect than a single target. For this purpose, we first screened SrtA inhibitors based on a FRET assay. Isosakuranetin reversibly inhibited SrtA activity at a low concentration. To our delight, isosakuranetin also inhibited the haemolytic activity of MRSA USA300. Isosakuranetin suppressed S. aureus virulence. This advantage made isosakuranetin even more promising for exploitation. It has been reported that suppression of SrtA in S. aureus is inhibited by specific compounds or by deletion of the SrtA gene, which weakens its virulence by the loss of binding to IgG and fibrin. This was also confirmed by our results showing that biofilm formation, SpA anchoring and bacterial adhesion and invasion were inhibited in isosakuranetin-treated S. aureus. Subsequently, fluorescence quenching further revealed a direct interaction between isosakuranetin and SrtA. To carry out its transpeptidase function, S. aureus SrtA employs a ping-pong double shift mechanism that depends on three conserved active site residues, His120, Cys184, and Arg197 [Citation49–51]. Molecular docking further revealed the key amino acid site of isosakuranetin binding to SrtA, which included Arg197, and provided evidence for the significant anti-SrtA effect of isosakuranetin in vitro.
Based on the inhibitory effect of isosakuranetin on the S. aureus haemolytic phenotype, we further explored its mechanism of action. The neutralization test confirmed that isosakuranetin had no neutralizing activity against haemolysis, so it was assumed that isosakuranetin inhibited haemolysis indirectly. In addition to direct binding, it is possible to inhibit haemolysis by regulating Hla expression through an upstream system. The Agr system is influenced by bacterial density and activates the Agr regulatory network through the accumulation of autoinducing peptide (AIP), while RNAIII is a major effector regulating a range of downstream virulence factors, such as Hla and pvl. The qPCR assays confirmed our conjecture that isosakuranetin inhibited RNAIII transcription in a dose-dependent manner. Isosakuranetin may regulate the expression of haemolysin through the Agr system to inhibit haemolysis.
Pneumonia accounts for a very large proportion of infections caused by S. aureus. Having demonstrated the potent inhibition of SrtA and Hla by isosakuranetin in vitro, we next established a pneumonia infection model to investigate the therapeutic effects of isosakuranetin in the context of relevant in vivo infection. Isosakuranetin showed significant therapeutic effects in a model of acute pneumonia induced by S. aureus, increasing the survival rate of mice at lethal doses, especially in the early stages of infection. At the same time, it also reduced the bacterial load in the lungs, the degree of pulmonary oedema, and the secretion of inflammatory cells in the lungs of the infected mice, showing a significant anti-MRSA effect in vivo. Isosakuranetin exhibited substantial in vitro inhibition of both SrtA and Hla. However, considering its diverse pharmacological activities, which encompass anti-inflammatory and antioxidative actions, its protective effect against pneumonia likely transpires through a multifaceted mechanism.
In conclusion, our study reveals the anti-SrtA and haemolytic effects of isosakuranetin and provides a novel candidate compound active against MRSA in a non-bactericidal manner (). A promising strategy is anti-virulence medications that block the pathogenic elements of bacteria. However, the lack of standardized preclinical tests similar to those used to guide the development of antibiotics has limited their development [Citation52]. It is believed that in the near future, anti-virulence drugs will be used to combat drug-resistant pathogens.
Figure 7. Isosakuranetin reduces S. aureus virulence and fights MRSA infection by inhibiting SrtA activity and interfering with the upstream pathway of Hla. Isosakuranetin is a potent compound that effectively reduces the virulence of S. aureus. It reduces bacterial adhesion to host cells by specifically inhibiting SrtA enzyme activity while inhibiting the formation of S. aureus biofilms. In addition, it disruptively interferes with the pathway upstream of Hla and affects Hla secretion through the Agr system. This mechanism of action illustrates the therapeutic promise of isosakuranetin in the face of MRSA infections, offering a ray of hope for the sustained fight against antibiotic-resistant strains.
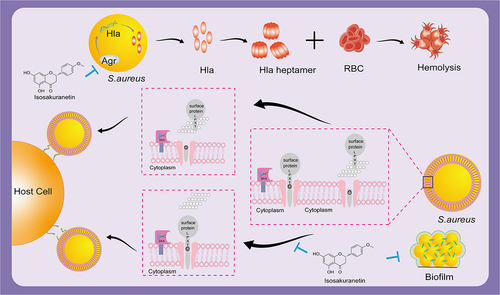
Author contributions
Hong Jiang and Tiezhong Zhou conceived and designed the experiments; Fengying Yang, Lili Tian and Li Wang performed and analysed the experiments; Lili Tian and Li Wang revised the manuscript.
Ethical statement
The animal experiments were conducted in accordance with the principles of the Experimental Animal Ethics Committee of Jinzhou Medical University.
Supplemental Material
Download MS Word (65.1 KB)Supplementary material
Supplemental data for this article can be accessed online at https://doi.org/10.1080/21505594.2023.2260675.
Disclosure statement
No potential conflict of interest was reported by the authors.
Data Availability statement
The data that support the findings of this study are available from the corresponding author.
Additional information
Funding
References
- Otto M. Basis of virulence in community-associated methicillin-resistant Staphylococcus aureus. Annu Rev Microbiol. 2010;64:143–16. doi: 10.1146/annurev.micro.112408.134309
- Lindsay JA. Evolution of Staphylococcus aureus and MRSA during outbreaks. Infect Genet Evol. 2014;21:548–553. doi: 10.1016/j.meegid.2013.04.017
- Zhang L, Gao J, Barkema HW, et al. Virulence gene profiles: alpha-hemolysin and clonal diversity in Staphylococcus aureus isolates from bovine clinical mastitis in China. BMC Vet Res. 2018;14(1):63. doi: 10.1186/s12917-018-1374-7
- Venter H. Reversing resistance to counter antimicrobial resistance in the World Health Organisation’s critical priority of most dangerous pathogens. Biosci Rep. 2019;39(4). doi: 10.1042/bsr20180474
- Laxminarayan R, Duse A, Wattal C, et al. Antibiotic resistance-the need for global solutions. Lancet Infect Dis. 2013;13(12):1057–1098. doi: 10.1016/s1473-3099(13)70318-9
- Medina E, Pieper DH. Tackling threats and future problems of multidrug-resistant bacteria. Curr Top Microbiol Immunol. 2016;398:3–33. doi: 10.1007/82_2016_492
- Beltran JA, Del Rio G, Brizuela CA. An automatic representation of peptides for effective antimicrobial activity classification. Comput Struct Biotechnol J. 2020;18:455–463. doi: 10.1016/j.csbj.2020.02.002
- Chawla M, Verma J, Gupta R, et al. Antibiotic potentiators against Multidrug-Resistant Bacteria: discovery, development, and clinical relevance. Front Microbiol. 2022;13:887251. doi: 10.3389/fmicb.2022.887251
- Wang CH, Hsieh YH, Powers ZM, et al. Defeating antibiotic-resistant bacteria: exploring alternative therapies for a post-antibiotic era. Int J Mol Sci. 2020;21(3). doi: 10.3390/ijms21031061
- Kirienko NV, Rahme L, Cho YH. Editorial: beyond antimicrobials: non-traditional approaches to combating multidrug-resistant bacteria. Front Cell Infect Microbiol. 2019;9:343. doi: 10.3389/fcimb.2019.00343
- Mühlen S, Dersch P. Anti-virulence strategies to target bacterial infections. Curr Top Microbiol Immunol. 2016;398:147–183. doi: 10.1007/82_2015_490
- Zucca M, Savoia D. The post-antibiotic era: promising developments in the therapy of infectious diseases. Int J Biomed Sci. 2010;6(2):77–86.
- Cheung GYC, Bae JS, Otto M. Pathogenicity and virulence of Staphylococcus aureus. Virulence. 2021;12(1):547–569. doi: 10.1080/21505594.2021.1878688
- Si L, Li P, Liu X, et al. Chinese herb medicine against sortase a catalyzed transformations, a key role in gram-positive bacterial infection progress. J Enzyme Inhib Med Chem. 2016;31(sup1):184–196. doi: 10.1080/14756366.2016.1178639
- Zhang J, Liu H, Zhu K, et al. Antiinfective therapy with a small molecule inhibitor of Staphylococcus aureus sortase. Proc Natl Acad Sci U S A. 2014;111(37):13517–13522. doi: 10.1073/pnas.1408601111
- Alharthi S, Alavi SE, Moyle PM, et al. Sortase a (SrtA) inhibitors as an alternative treatment for superbug infections. Drug Discov Today. 2021;26(9):2164–2172. doi: 10.1016/j.drudis.2021.03.019
- Cascioferro S, Totsika M, Schillaci D. Sortase A: an ideal target for anti-virulence drug development. Microb Pathog. 2014;77:105–112. doi: 10.1016/j.micpath.2014.10.007
- Hussain M, Kohler C, Becker K. Role of SrtA in pathogenicity of Staphylococcus lugdunensis. Microorganisms. 2020;8(12). doi: 10.3390/microorganisms8121975
- Mazmanian SK, Ton-That H, Schneewind O. Sortase-catalysed anchoring of surface proteins to the cell wall of Staphylococcus aureus. Mol Microbiol. 2001;40(5):1049–1057. doi: 10.1046/j.1365-2958.2001.02411.x
- Mazmanian SK, Liu G, Jensen ER, et al. Staphylococcus aureus sortase mutants defective in the display of surface proteins and in the pathogenesis of animal infections. Proc Natl Acad Sci U S A. 2000;97(10):5510–5515. doi: 10.1073/pnas.080520697
- Weiss WJ, Lenoy E, Murphy T, et al. Effect of srtA and srtB gene expression on the virulence of Staphylococcus aureus in animal models of infection. J Antimicrob Chemother. 2004;53(3):480–486. doi: 10.1093/jac/dkh078
- Maresso AW, Schneewind O. Sortase as a target of anti-infective therapy. Pharmacol Rev. 2008;60(1):128–141. doi: 10.1124/pr.107.07110
- Bhakdi S, Tranum-Jensen J. Alpha-toxin of Staphylococcus aureus. Microbiol Rev. 1991;55(4):733–751. doi: 10.1128/mr.55.4.733-751.1991
- Valeva A, Walev I, Pinkernell M, et al. Transmembrane beta-barrel of staphylococcal alpha-toxin forms in sensitive but not in resistant cells. Proc Natl Acad Sci U S A. 1997;94(21):11607–11611. doi: 10.1073/pnas.94.21.11607
- Kennedy AD, Bubeck Wardenburg J, Gardner DJ, et al. Targeting of alpha-hemolysin by active or passive immunization decreases severity of USA300 skin infection in a mouse model. J Infect Dis. 2010;202(7):1050–1058. doi: 10.1086/656043
- Krüll M, Dold C, Hippenstiel S, et al. Escherichia coli hemolysin and Staphylococcus aureus alpha-toxin potently induce neutrophil adhesion to cultured human endothelial cells. J Immunol. 1996;157(9):4133–4140. doi: 10.4049/jimmunol.157.9.4133
- Liang X, Ji Y. Alpha-toxin interferes with integrin-mediated adhesion and internalization of Staphylococcus aureus by epithelial cells. Cell Microbiol. 2006;8(10):1656–1668. doi: 10.1111/j.1462-5822.2006.00740.x
- Berube BJ, Bubeck Wardenburg J. Staphylococcus aureus α-toxin: nearly a century of intrigue. Toxins (Basel). 2013;5(6):1140–1166. doi: 10.3390/toxins5061140
- Virreira Winter S, Zychlinsky A, Bardoel BW. Genome-wide CRISPR screen reveals novel host factors required for Staphylococcus aureus α-hemolysin-mediated toxicity. Sci Rep. 2016;6:24242. doi: 10.1038/srep24242
- Nygaard TK, Pallister KB, DuMont AL, et al. Alpha-toxin induces programmed cell death of human T cells, B cells, and monocytes during USA300 infection. PLoS One. 2012;7(5):e36532. doi: 10.1371/journal.pone.0036532
- Drira R, Sakamoto K. Isosakuranetin, a 4’-O-methylated flavonoid, stimulates melanogenesis in B16BL6 murine melanoma cells. Life Sci. 2015;143:43–49. doi: 10.1016/j.lfs.2015.10.009
- Jia S, Zhang Y, Yu J. Antinociceptive effects of isosakuranetin in a rat model of peripheral neuropathy. Pharmacology. 2017;100(3–4):201–207. doi: 10.1159/000478986
- Wang L, Li Q, Li J, et al. Eriodictyol as a potential candidate inhibitor of sortase a protects mice from methicillin-resistant Staphylococcus aureus-induced pneumonia. Front Microbiol. 2021b;12:635710. doi: 10.3389/fmicb.2021.635710
- Trott O, Olson AJ. AutoDock Vina: improving the speed and accuracy of docking with a new scoring function, efficient optimization, and multithreading. J Comput Chem. 2009;31(2):NA–NA. doi: 10.1002/jcc.21334
- Wang L, Jing S, Qu H, et al. Orientin mediates protection against MRSA-induced pneumonia by inhibiting sortase a. Virulence. 2021a;12(1):2149–2161. doi: 10.1080/21505594.2021.1962138
- Song W, Wang L, Jin M, et al. Punicalagin, an inhibitor of sortase A, is a promising therapeutic drug to combat methicillin-resistant Staphylococcus aureus infections. Antimicrob Agents Chemother. 2022;66(6):e0022422. doi: 10.1128/aac.00224-22
- Hou X, Wang M, Wen Y, et al. Quinone skeleton as a new class of irreversible inhibitors against Staphylococcus aureus sortase a. Bioorg Med Chem Lett. 2018;28(10):1864–1869. doi: 10.1016/j.bmcl.2018.04.005
- Falugi F, Kim HK, Missiakas DM, et al. Role of protein a in the evasion of host adaptive immune responses by Staphylococcus aureus. MBio. 2013;4(5):e00575–00513. doi: 10.1128/mBio.00575-13
- Qin C, Xin X, Pei X, et al. Amorphous Nanosuspensions Aggregated from paclitaxel–hemoglobulin complexes with enhanced cytotoxicity. Pharmaceutics. 2018;10(3):92. doi: 10.3390/pharmaceutics10030092
- Melander RJ, Zurawski DV, Melander C. Narrow-Spectrum antibacterial Agents. Med Chem Commun. 2018;9(1):12–21. doi: 10.1039/c7md00528h
- Vandenesch F, Lina G, Henry T. Staphylococcus aureus hemolysins, bi-component leukocidins, and cytolytic peptides: a redundant arsenal of membrane-damaging virulence factors? Front Cell Infect Microbiol. 2012;2:12. doi: 10.3389/fcimb.2012.00012
- Wolfmeier H, Mansour SC, Liu LT, et al. Liposomal therapy attenuates dermonecrosis induced by community-associated methicillin-resistant Staphylococcus aureus by targeting α-type phenol-soluble modulins and α-hemolysin. EBioMedicine. 2018;33:211–217. doi: 10.1016/j.ebiom.2018.06.016
- Rasko DA, Sperandio V. Anti-virulence strategies to combat bacteria-mediated disease. Nat Rev Drug Discov. 2010;9(2):117–128. doi: 10.1038/nrd3013
- Powers ME, Kim HK, Wang Y, et al. ADAM10 mediates vascular injury induced by Staphylococcus aureus α-hemolysin. J Infect Dis. 2012;206(3):352–356. doi: 10.1093/infdis/jis192
- Wilke GA, Bubeck Wardenburg J. Role of a disintegrin and metalloprotease 10 in Staphylococcus aureus alpha-hemolysin-mediated cellular injury. Proc Natl Acad Sci U S A. 2010;107(30):13473–13478. doi: 10.1073/pnas.1001815107
- Wei Y, Xiong J, Larson NR, et al. Effect of 2 emulsion-based adjuvants on the structure and thermal stability of Staphylococcus aureus Alpha-Toxin. J Pharm Sci. 2018;107(9):2325–2334. doi: 10.1016/j.xphs.2018.05.019
- Kruger RG, Otvos B, Frankel BA, et al. Analysis of the substrate specificity of the Staphylococcus aureus sortase transpeptidase SrtA. Biochemistry. 2004;43(6):1541–1551. doi: 10.1021/bi035920j
- Wang J, Li H, Pan J, et al. Oligopeptide targeting sortase a as potential anti-infective therapy for Staphylococcus aureus. Front Microbiol. 2018;9:245. doi: 10.3389/fmicb.2018.00245
- Frankel BA, Kruger RG, Robinson DE, et al. Staphylococcus aureus sortase transpeptidase SrtA: insight into the kinetic mechanism and evidence for a reverse protonation catalytic mechanism. Biochemistry. 2005;44(33):11188–11200. doi: 10.1021/bi050141j
- Frankel BA, Tong Y, Bentley ML, et al. Mutational analysis of active site residues in the Staphylococcus aureus transpeptidase SrtA. Biochemistry. 2007;46(24):7269–7278. doi: 10.1021/bi700448e
- Huang X, Aulabaugh A, Ding W, et al. Kinetic mechanism of Staphylococcus aureus sortase SrtA. Biochemistry. 2003;42(38):11307–11315. doi: 10.1021/bi034391g
- Verderosa AD, Dhouib R, Hong Y, et al. A high-throughput cell-based assay pipeline for the preclinical development of bacterial DsbA inhibitors as antivirulence therapeutics. Sci Rep. 2021;11(1):1569. doi: 10.1038/s41598-021-81007-y