ABSTRACT
Candida albicans is an important opportunistic pathogenic fungus that frequently causes serious systemic infection in humans. Due to the vital roles of biofilm formation and the yeast-to-hypha transition in the infection process, we have selected a series of diaryl chalcogenides and tested their efficacy against C. albicans SC5314 pathogenicity by the inhibition of biofilm formation and the yeast-to-hypha transition. The compounds 5-sulfenylindole and 5-selenylindole were found to have excellent abilities to inhibit both biofilm formation and hyphal formation in C. albicans SC5314. Intriguingly, the two leading compounds also markedly attenuated C. albicans SC5314 virulence in human cell lines and mouse infection models at micromolar levels. Furthermore, our results showed that the presence of the compounds at 100 µM resulted in a marked decrease in the expression of genes involved in the cAMP-PKA and MAPK pathways in C. albicans SC5314. Intriguingly, the compounds 5-sulfenylindole and 5-selenylindole not only attenuated the cytotoxicity of Candida species strains but also showed excellent synergistic effects with antifungal agents against the clinical drug-resistant C. albicans strain HCH12. The compound 5-sulfenylindole showed an obvious advantage over fluconazole as it could also restore the composition and richness of the intestinal microbiota in mice infected by C. albicans. Together, these results suggest that diaryl chalcogenides can potentially be designed as novel clinical therapeutic agents against C. albicans infection. The diaryl chalcogenides of 5-sulfenylindole and 5-selenylindole discovered in this study can provide new direction for developing antifungal agents against C. albicans infection.
Introduction
Candida albicans causes some of the most frequently encountered hospital-acquired infections, including oral thrush in children, colpomycosis in adults and candidemia in immunocompromised patients [Citation1]. It is distinguished by the yeast-to-hypha transition, which is one important virulence factor for C. albicans during pathogenesis [Citation2–4]. Due to the drug resistance of C. albicans continues to develop, studies have focused on new strategies of inhibiting yeast-to-hypha transition and biofilm formation [Citation5–8].
The yeast cell type is initially non-toxic, and the subsequent conversion of yeast to mycelium causes disease, as the new cell type can induce virulence factors such as adhesion and invasion factors [Citation9–13]. When an abundant aggregation of polymorphic structure include yeast, pseudo hyphae and hyphae cells has accumulated, they form a biofilm, a polymorphic structure that can exceed 200 micrometres in depth and increase resistance to antimicrobial drugs and immune surveillance [Citation14].
Hyphal formation is one of the most important virulence characteristics of C. albicans [Citation9]. Previous studies have shown that farnesol is a quorum sensing molecule that inhibits mycelial formation in solid-phase cells of C. albicans [Citation15]. Moreover, tyrosol has also been identified as a QS molecule involved in eliminating the lag before stationary phase cells resume growth [Citation16]. Farnesol can also regulate the expression of virulence genes and the formation of biofilm [Citation17,Citation18]. Our previous studies have already demonstrated the efficient treatment of C. albicans infection by targeting the hyphal formation, inhibiting hypha formation and biofilm formation in vitro and in vivo [Citation19–22].
Diaryl chalcogenides are an important class of compounds that are common in a variety of functional products and have a variety of biological and therapeutic activities, especially antimicrobial activity [Citation23–25]. Many compounds containing these active groups are drugs with potential applications in the treatment of inflammation, cancer, human immunodeficiency virus (HIV), asthma, and Alzheimer’s and Parkinson’s diseases. Owing to these significant biological functions, our previous studies have identified a new and efficient approach to accessing a wide array of diaryl chalcogenides [Citation26], so we chose these compounds to test their anti-virulence activity against C. albicans infection. In this work, we have demonstrated two small-molecule compounds, 5-sulfenylindole and 5-selenylindole that show a strong inhibitory effect on the phenotypes of biofilm formation, hyphal morphogenesis and virulence of C. albicans. Furthermore, our results showed that the two compounds significantly increased the clearance of fungal pathogen, and showed favourable synergistic effects with antifungal agents against the clinical drug-resistant C. albicans strain. More interestingly, we found that the compound could restore the composition and richness of the intestinal microbiota of mice infected by C. albicans. Our findings suggested that 5-sulfenylindole and 5-selenylindole may offer a potential solution for C. albicans infection.
Material and methods
Strains and culture
The C. albicans strains used in this study were SC5314 (ATCC MYA-2876), ATCC 10,231, ATCC 90,028, and ATCC 14,053. And the other Candida pathogens analysed were C. tropicalis ATCC 750, Nakaseomyces glabrata ATCC 2001 and a clinical drug-resistant C. albicans strain. These fungal cells were grown either in yeast nitrogen broth (YNB) or in yeast extract, peptone, dextrose (YPD) medium at 30 °C in a shaking incubator [Citation20–22]. Human lung epithelial A549 and normal human small intestinal epithelial (HIEC) cells were incubated in Dulbecco modified Eagle medium (DMEM) with 10% foetal bovine serum (FBS) at 37°C with 5% CO2. The compounds used in this study were synthesized using the published methodologies [Citation26].
Filamentation assays and colony morphology
Overnight cultures of C. albicans were collected and washed in PBS, then diluted to OD600 = 0.1 in fresh YNB medium. Then the C. albicans were induced for 6 h at 37 °C with different concentrations of compounds, the same volume of 0.2% DMSO as the control [Citation27–29]. Images of cells were captured by a Lecia inverted fluorescence microscope with 20× lenses. Three images were taken per group and performed three times.
The colony morphology assay was carried out using Spider media agar plates. C. albicans cells were diluted using Spider medium to 1000 cells/mL, and 50 µL was plated [Citation30]. Then, the plates were incubated at 37 °C for 48 h. Fields of the C. albicans colonies were observed by Leica DMi8 microscope.
Biofilm formation assays
Direct measurement of biofilm formation using crystal violet (CV) staining as described previously [Citation31]. Overnight cultured C. albicans cells were diluted to OD600 = 0.05 in fresh YNB media with different concentrations of compounds, the same volume of 0.2% DMSO as the control. The C. albicans cells were grown in 96-well polystyrene plate at 37 °C for 24 h without shaking. Next, removed the supernatants and washed three times with sterile phosphate-buffered saline (PBS), and added 0.02% CV for 45 min. Then, the plate was washed six times with PBS, and added 200 µL of 75% ethanol to dissolve the biofilm. The quantity of CV was determined by measuring the absorbance at 590 nm. The experiment was performed in triplicate, and the experimental group in each well was compared to the DMSO group.
Cytotoxicity measurement
The toxicity of compounds was evaluated by measuring the release amount of LDH from A549 and HIEC cells as described previously [Citation22]. A549 and HIEC cells were seeded in 96-well tissue culture plates with 1.5 × 104 cells/well one night in advance. Then the medium was replaced by fresh DMEM medium with different concentrations of compounds, the same volume of 0.2% DMSO as the control. The LDH release was measured after 8 h and 24 h of incubation, and the cytotoxicity was calculated as the ratio of LDH from the treated cells to the difference between the LDH values of the chemically lysed cells (maximum) and those of the DMSO-treated cells (control), then calculated the cytotoxicity of the compounds.
The effects of compounds on C. albicans cytotoxicity were tested as described previously [Citation22]. Briefly, the C. albicans cells (OD600 = 0.1) in DMEM medium with different concentrations of compounds replaced the DMEM medium, the same volume of 0.2% DMSO as the control. The multiplicity of infection (MOI) is approximately 200. The LDH release was measured, then the cytotoxicity was calculated, after 8 h of incubation. The C. albicans cytotoxicity of DMSO group was defined as 100%, and used to normalized C. albicans cytotoxicity of compounds treatment groups.
Mouse mortality and oral mucosal infection
All animal experiments were conducted in strict accordance with the Guide for the Care and Use of Laboratory Animals and confirmed by the Institutional Animal Care and Use Committee (IACUC), Sun Yat-Sen University. The approval No. SYSU-YXYSZ20210803. The initial infective inoculum of C. albicans was collected and washed three times with PBS. Cells were diluted to different OD600 values to prepare the inocula.
For the mouse mortality model [Citation32], 200 µL of C. albicans suspension (OD600 = 0.5) was infused into 6–8-week-old male BALB/C mice through the tail vein. After 8 hours postinoculation, the treatment groups were infused 200 µL compounds of 100 µM in PBS through the tail vein. The fluconazole of 10 µg/mL was as the positive control group. The control group was treated with the same protocol but injected only with PBS buffer. Data were recorded and analysed using GraphPad Prism 8 to determine the survival curves for two weeks.
For the OPC model [Citation33]. Mice were subcutaneously injected cortisone acetate one day before inoculation and the third day after injection for immunosuppression. C. albicans cells were dissolved in Hank’s Balanced Salt Solution (HBSS) of 3 × 106 cells/mL, and added the sterile calcium alginate swab to soak. The mice were anesthetized with 10% chloral hydrate, placed on isothermal mats. The mice are inoculated by placing a calcium alginate swab saturated with C. albicans blastospores sublingually for 75 min. One day later, the mice were given 400 µL compounds of 100 µM or PBS to by gavage once every day for a total of three times. Then the mice were euthanized, and the tongues were bisected longitudinally for colony-forming unit (CFU) analysis and tissue histology.
Histopathological diagnosis
In order to study the oral cavity of mice infected with C. albicans, the tongues of mice were observed by pathological section. The histological sections were stained with Periodic Acid-Schiff stain for histopathological diagnosis [Citation34]. The mouse tongues were fixed with 4% formaldehyde, then dehydrated and paraffin embedded. The sections were dewaxed to water and stained with Periodic Acid-Schiff stain for histopathological diagnosis. Finally, they were observed and imaged with a microscope.
Analysis of C. albicans cell numbers in mouse tongue
The mouse tongue was collected and weighed, then ground in sterilized water. The mouse tongue suspension was serially diluted and spread on SD plates, and grown at 30 °C for two days. Then the number of C. albicans CFU on the plates was counted.
Analysis of susceptibility of Candida strains to compounds or antifungal agents
MIC values of antifungal agents and compounds against Candida spp. used in this study were determined based on the broth microdilution protocol of Clinical and Laboratory Standards Institute (CLSI) document M23-A7 with small modifications [Citation35]. Briefly, overnight cultured cells were inoculated at an OD600 of 0.05 in YPD medium supplemented with compounds or antifungal agents, the same volume of 0.2% DMSO as the control. 100 µL of cultures in 96-well polystyrene plate were grown at 30 °C with shaking at 250 rpm for 24 hours. The MIC was defined as the lowest concentration of antibiotics in which Candida spp. cannot grow in the well.
Statistics
All data are expressed as the mean ± standard deviations of three independent experiments. Statistical analysis was performed using GraphPad Prism 8 with the unpaired t-test at a *, P < 0.05; **, P < 0.01; ***, P < 0.001 level of significance.
Results
Diaryl chalcogenides inhibit C. albicans SC5314 biofilm formation, hyphal formation and virulence
The yeast-to-hypha transition is a central cue of fungal-host interactions in C. albicans, and the filamentous forms are always proven pathogenic; however, yeasts are considered symbiotic [Citation29]. A series of diaryl chalcogenide compounds synthesized in the previous studies were therefore evaluated for the inhibitory effects on the hypha formation of C. albicans SC5314 (Figure S1). We continued to investigate the capacity of compounds to inhibit the yeast-to-hypha transition in C. albicans SC5314 cells under hyphal-inducing conditions. After hyphal formation was induced for 6 h, almost all of the cells in the control group with the addition of DMSO formed hyphae, whereas the hyphal formation was notably reduced in the presence of several compounds (). The addition of compounds 1, 2, 3, 5, 6, and 8 at 100 µM reduced hyphal formation by more than 70%. Biofilms of C. albicans cells are important virulence factors. Surprisingly, two compounds, compound 3 and compound 8, at 100 µM, reduced the biofilm formation by approximately 65% and 70%, respectively ().
Figure 1. Influences of diaryl chalcogenides on C. albicans SC5314 hyphal formation, biofilm formation and virulence. Effects of diaryl chalcogenides on the hyphal formation (a) and biofilm formation (b) of C. albicans SC5314 at 100 µM. (c) analysis of the effect of compounds and fluconazole (1 µg/mL) on the cytotoxicity of C. albicans SC5314 to A549 cells. Cell cytotoxicity was detected and measured as LDH release. The LDH released by A549 cells in (c) after inoculation with C. albicans in the absence of compounds was defined as 100% and used to normalize the LDH release ratios of other treatments. The compounds were dissolved in DMSO, and the equivalent amount of DMSO was added as a control. Each experiment was performed at least three times in triplicate. Data represent the means ± standard deviation of three independent experiments. *, P < 0.05; **, P < 0.01; ***, P < 0.001 (unpaired t-test).
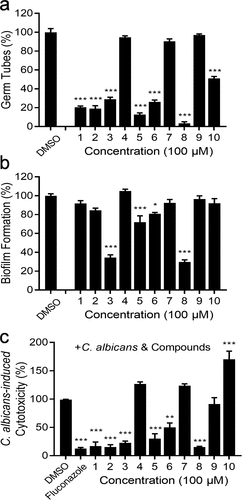
To assess the effects of diaryl chalcogenides on C. albicans pathogenicity, it was investigated whether the compounds influenced the virulence of C. albicans SC5314 in human A549 cell line. All the tested compounds showed no toxic effects on A549 cells at 100 µM (Figure S2a). We also tested the cytotoxicity of compounds to normal HIEC cells. It was shown that only compound 3 displayed a slightly toxic effect and other compounds were non-toxic (Figure S2b). The LDH release was also measured after 24 h of incubation, and the result also showed that the compounds 3 and 8 showed no toxic effects on A549 and HIEC cells at 100 µM (Figure S2c). In addition, compounds 1, 2, 3, 5, 6, and 8 attenuated C. albicans SC5314 cytotoxicity by more than 50% at 100 µM (), which was considered highly effective. Compounds 1, 2, 3 and 8 exhibited reductions of 83.02%, 84.66%, 77.37% and 84.66%, respectively (). Intriguingly, compounds 3 and 8 showed consistently efficient inhibition of biofilm formation and hyphal formation in C. albicans SC5314 (), then they were selected for evaluation of inhibitory effects on C. albicans.
Compounds 3 and 8 inhibit hyphal formation, biofilm formation and virulence of C. albicans SC5314 in a dose-dependent manner
As compounds 3 and 8 showed excellent inhibition of hyphal and biofilm formation, and virulence in C. albicans, they were selected for further investigation at different concentrations. And we also detected its MIC values of different C. albicans strains, the application concentration of the compounds was much lower than their MICs (). To investigate whether the inhibitory effects of compounds 3 and 8 on the hyphal formation of C. albicans are related to their doses, the inhibitory activity of compounds 3 and 8 were tested at different concentrations from 6.25 to 100 µM (). The addition of compounds 3 and 8 from 6.25 to 100 µM reduced hyphal formation by 32.67% to 76.74% and 67.33% to 93.56%, respectively (, Figure S3a). As the colony morphology of fungal cells grown on solid Spider media could reflect the transition between hypha and yeast forms [Citation36,Citation37], we further compared the colony morphologies of C. albicans cells grown in the absence and presence of compounds 3 and 8. In good agreement with the inhibition of mycelium formation, compounds 3 and 8 also significantly influenced the colony morphology of C. albicans SC5314 (Figure S3b). At 100 µM of compounds 3 and 8, the colonies changed from wrinkled to smooth (Figure S3b). Consistent with their ability to block hyphal formation in a dose-dependent manner, compounds 3 and 8 also showed dose-dependent activity: compound 3 reduced C. albicans biofilm formation by more than 25% at 12.5 µM, while compound 8 decreased biofilm formation by approximately 30% at 12.5 µM (). The biofilm was also analysed by scanning electron microscopy (SEM), in which we observed that mature biofilm was formed after 48 h cultured on the acrylic resin discs. The biofilms of C. albicans treated with DMSO showed a thick and dense mycelium structure, however, the biofilm and hyphae treated with the compounds decreased significantly (Figure S4).
Figure 2. Different concentrations (6.25–100 µM) of compounds 3 and 8 and fluconazole (1 µg/mL) on C. albicans SC5314 hyphal formation (a), biofilm formation (b) and cytotoxicity (c). Data are means ± standard deviations from three independent experiments. ***P < 0.001 vs DMSO (unpaired t-test).

Table 1. Minimum inhibitory concentrations (MICs) of the compounds 3, 8 and fluconazole against the Candida strains and N. glabrata used in this study.
As compounds 3 and 8 showed consistently efficient inhibition of biofilm formation and hyphal formation in C. albicans SC5314 (, Figure S3, S4), and they were then selected for evaluation of inhibitory effects on C. albicans SC5314 virulence at different concentrations from 6.25 to 100 µM. It was indicated that compounds 3 and 8 showed strong protection of A549 cells even at 6.25 µM (). And under these conditions, both compounds 3 and 8 showed a certain inhibitory effect on the growth rate of C. albicans cells (Figure S5). The Evan’s blue staining experiment further supported this finding. We also found that the mortalities of C. albicans cells treated with compounds 3 and 8 at 100 µM were only 6.36% and 6.76%, respectively (Figure S6).
Compounds 3 and 8 attenuate C. albicans SC5314 pathogenicity in mouse infection models
In the normal human oral flora, C. albicans is a non-pathogenic resident species, whereas it can lead to life-threatening systemic infections in immunocompromised individuals [Citation38–40]. Since compounds 3 and 8 have excellent activities in inhibiting the biofilm and hyphal formation, and virulence of C. albicans, we further studied whether compounds 3 and 8 reduced pathogenicity of C. albicans in the mice infection models, including OPC model [Citation33]. The results of mouse model assays were comparable to those in the cell line model. Treatment with compound 3 or compound 8 led to an obvious reduction in C. albicans virulence in the mouse infection models (). The mortalities of mouse infected with C. albicans SC5314 without compounds, and with the treatment of fluconazole, compound 3 and compound 8 were 100%, 12.5%, 62.5% and 62.5%, respectively, at 14 d postinfection ().
Figure 3. Efficacy of diaryl chalcogenide compound 3 (100 µM) and compound 8 (100 µM) against C. albicans SC5314 in the mouse infection model. (a) survival rate of mice after infection with C. albicans SC5314 without treatment with (●) and by treated with fluconazole (10 µg/mL, ○), compound 3 (▲) and compound 8 (▽). (b) the in vivo pathogen cell numbers of C. albicans SC5314 in the mouse tongue after infection without treatment with and by treated with compound 3 and compound 8 (100 µM). (c) pathological sections were evaluated to determine the effects of compounds 3 and 8 (100 µM) on C. albicans SC5314 infection. The red arrows showed the C. albicans SC5314 infection sites in the mouse tongues. Data are the mean ± standard deviation of three independent experiments. ***, P < 0.001 (unpaired t-test). CA: C. albicans.

The further study is to analyse the symptoms of mice tongues infected with C. albicans, as the tongue is one of the most important niches for C. albicans cells. After treating with compound 3 or compound 8, the number of C. albicans cells collected in the pathological tissues was much lower than that in the tissues infected with C. albicans in the absence of either compound (), and the addition of compound 3 or compound 8 restored the tissues to a similar state to the uninfected group ().
Compounds 3 and 8 inhibit C. albicans SC5314 hyphal formation by interfering with the cAMP-PKA and MAPK pathways
To establish a hypothetical model to elucidate the effects of diaryl chalcogenides on the yeast-to-hypha transition of C. albicans, it was further investigated whether compounds 3 and 8 impaired the signalling pathways in mycelium development [Citation36,Citation41–43]. The formation of C. albicans mycelia is mostly related to two known signalling pathways: cyclic adenosine monophosphate/protein kinase A (cAMP-PKA) and mitogen-activated protein kinase cascade (MAPK) pathways. We used the RNA sequencing (RNA-seq) to analyse and compare the transcriptomic properties of C. albicans cultivated in the presence or absence of compound 3. Differential gene expression analysis showed that upon the addition of compound 3, 162 genes were upregulated and 182 genes were downregulated (log double change ≥ 1.5) (Figure S7a, Table S2). These differentially expressed genes were associated with a range of biological functions, including proteins such as heat shock proteins, cell wall proteins, transcription factors, filamentous growth proteins, and transporters (Figure S7b, Table S2). Then the effects of compounds on the mycelium-specific genes were analysed by using the quantitative real-time PCR analysis. The quantitative real-time PCR results also demonstrated that compounds 3 and 8 clearly decreased the relevant gene expression levels in both the cAMP-PKA and MAPK pathways (Figure S7c). EFG1 is a transcription factor that is activated by the PKA pathway and directly interacts with hypha-specific promoters during filament development. TEC1 is regulated by EFG1 and also regulates filamentous formation. HWP1 is a cell wall protein critical for cellular adhesion and filamentous formation. Then we used western blotting assay to analyse the effects of compounds on these two pathways. The results showed that the addition of compounds 3 and 8 significantly inhibited the protein levels of EFG1, HWP1 and TEC1 (Figure S7d). Taken together, these results demonstrated that compounds 3 and 8 influenced at least two signalling transduction pathways to interfere with C. albicans hyphal and biofilm formation.
Compounds 3 and 8 inhibit biofilm formation and cytotoxicity in other clinical Candida isolates
In order to determine whether the efficacy of diaryl chalcogenides on C. albicans is widespread, we also collected different clinical isolates of Candida species, including C. albicans ATCC 90,028, ATCC 10,231, ATCC 14,053, C. tropicalis ATCC 750 and N. glabrata ATCC 2001, and studied the effects of compounds 3 and 8 on their biofilm formation and virulence. Intriguingly, compounds 3 and 8 exerted strong inhibition on biofilm formation in C. albicans ATCC 90,028, C. albicans ATCC 10,231, C. tropicalis ATCC 750 and N. glabrata ATCC 2001 (). The addition of compounds 3 and 8 at 100 µM reduced biofilm formation in C. albicans ATCC 10,231, ATCC 90,028, C. tropicalis ATCC 750 and N. glabrata ATCC 2001 to approximately 22.41% and 19.71%, 26.54% and 38.91%, 33.78% and 33.75%, and 51.13% and 55.62% of that in the untreated group, respectively (). Moreover, compounds 3 and 8 at 100 µM reduced the cytotoxicity of C. albicans ATCC 10,231, ATCC 90,028, ATCC 14,053, C. tropicalis ATCC 750 and N. glabrata ATCC 2001 against A549 cells to 29.07% and 26.37%, 32.54% and 40.25%, 50.59% and 40.01%, 33.44% and 30.08%, and 44.46% and 38.62% of that in the untreated group, respectively (). Additionally, compounds 3 and 8 clearly inhibited hyphal formation in C. albicans ATCC 10,231, ATCC 90,028 and ATCC 14,053 ().
Figure 4. Analysis of biofilm formation (a), cytotoxicity (b) and hyphal formation (c) of different Candida spp. and N. glabrata clinical isolates in the absence or presence of compound 3 and compound 8 (100 µM). Data represent the means ± standard deviation of three independent experiments. **, P < 0.01; ***, P < 0.001 (unpaired t-test).
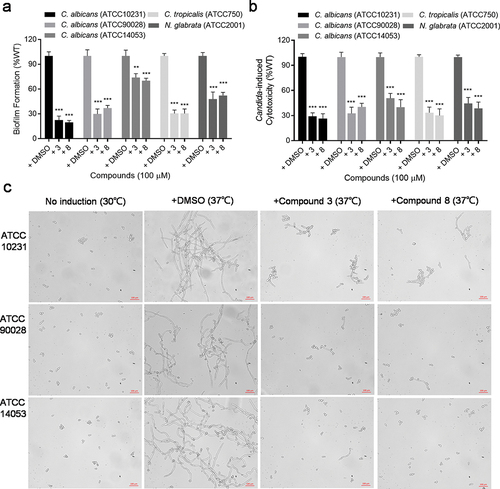
Combination of compounds with fluconazole synergistically decreases the virulence of a clinical drug-resistant C. albicans strain in vitro
Due to the good inhibitory effect of the compounds on the biological function and cytotoxicity of C. albicans, we continue to study whether these compounds have synergistic effect with the antifungal drugs on the drug-resistant C. albicans. We isolated a clinical fluconazole-resistant C. albicans strain from the Hunan Children’s Hospital. The results showed that fluconazole at 16 μg/mL did not inhibit biofilm formation and cytotoxicity in the drug-resistant strain (). Intriguingly, addition of compounds 3 and 8 can obviously decrease the biofilm formation and cytotoxicity of this strain (). And the combination of compound 3 or 8 with fluconazole showed stronger inhibition on biofilm formation and cytotoxicity of this drug-resistant strain than the addition of compounds alone (). At the same time, our results showed that the compounds and antifungal drugs (including fluconazole, nystatin and voriconazole) have a synergistic effect on the wild-type strain of C. albicans (). In addition, the compounds and fluconazole also have a synergistic effect on the drug-resistant strain (). These results not only proved the synergistic effect of compounds with antifungal drugs on the clinical drug-resistant C. albicans strain, but also proved the possible use of the compounds as antifungal drugs.
Figure 5. Effects of compounds (100 µM) and their synergistic activities with fluconazole on the biofilm formation (a) and virulence (b) of the clinical drug-resistant C. albicans strain. Data are means ± standard deviations from three independent experiments. **, P < 0.01; ***, P < 0.001 (unpaired t-test).
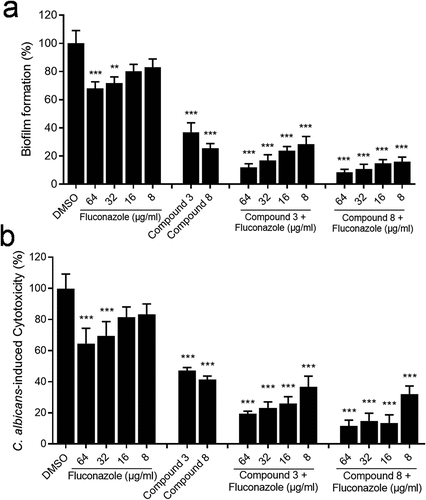
Table 2. Synergistic activities of compounds 3 and 8 with fluconazole, nystatin, and voriconazole against the C. albicans SC5314 wild-type strain.
Table 3. Synergistic activities of compounds 3 and 8 with fluconazole against the clinical drug-resistant C. albicans strain HCH12.
Compound 3 impairs the fitness of C. albicans in mouse intestinal microbiota and restores the richness of mouse intestinal microbiota
Intestinal microbiota is very important for the host. It can synthesize a variety of nutrients required for human growth and development. It also participates in the metabolism and absorption of a variety of nutrients [Citation44]. C. albicans is a normal resident of human intestinal microbiota, which causes opportunistic disseminated infection in individuals with immunocompromised [Citation45]. We sequenced 16S rRNA (bacteria) and ITS region (fungi) genes to investigate the impacts of C. albicans infection and fluconazole or compound treatment on intestinal microbiome in mice. We found that, after C. albicans infection and fluconazole treatment, the composition of intestinal microbiome of mice changed significantly. More than 70% of the bacterial flora abundance changed. As shown in Figure S8a, the relative abundance of Sphingomonas, Burkholderia, Chryseobacterium, and Gluconobacter was significantly reduced after C. albicans infection and fluconazole treatment, and the relative abundance of Acetobacter was significantly increased. However, in the compound 3 treatment group, the main microflora was similar as that in the control group, and the richness of Bradyrhizobium, Vulcaniibacterium, Acetobacter, Chitinophagaceae, Cutibacterium and Methlovirgula were increased. As shown in Figure S8b, the intestinal microflora of mice was mainly Candida after C. albicans infection, and in the fluconazole treatment group, the main fungi were Brettanomyces. In the compound 3 treatment group, the main flora was similar as that in the control group, and the richness of Botryoderma and Pichia were increased. In conclusion, compared with those of the control group, the composition and abundance of gut microbiota in C. albicans infection group and fluconazole treatment group changed significantly. Interestingly, the composition and richness of intestinal microbiota treated with compound were clearly restored to those under normal conditions, which was better than fluconazole ingesting, and tended to be more normal faecal microbiota ecology (Figure S8).
Discussion
Diaryl chalcogenides are frequently existed in many agents with a variety of biological and therapeutic activities, such as antiviral agents [Citation46] and anticancer agents [Citation47]. Several previous reports of the antifungal activities of this kind of compounds were mainly focused on molecules with a quinoline motif [Citation48,Citation49], but there were no previous reports about compounds containing chalcogenyl N-heteroaromatics. Our previous studies have demonstrated a new and efficient approach for accessing novel diaryl chalcogenides and this study verified that the products could be developed as novel antifungal drugs against C. albicans infection by targeting virulence factors. Due to the increasing numbers of drug-resistant fungal pathogens, C. albicans infections continue to represent a major challenge in clinical treatment. Biofilm is one of the key causes for C. albicans’ resistance to traditional antifungal agents. Some natural products and small-molecule compounds have been reported to exhibit activity against the biofilm formation of C. albicans [Citation17,Citation50,Citation51]. Our results in this study demonstrate for the first time that diaryl chalcogenides could be designed as antibiofilm agents against C. albicans (). Among our tested compounds, compounds 3 and 8 showed an excellent ability to inhibit the biofilm formation of C. albicans cells ().
Previous studies have shown that indole analogues [Citation52] and quinazoline derivatives [Citation53] can inhibit the formation of biofilm and hyphae of C. albicans. Our findings also indicated that the structural features of diaryl chalcogenides contribute to their biological activities. Compounds 3 and 8, which are unique diaryl chalcogenides containing an indole core different from other sulfenyl N-heteroaromatic compounds (1, 2, 6, 7, 9 and 10) (, S1), showed excellent antibiofilm activity. Furthermore, the substituted position on the indole ring of thioaryl indole also affected the activity. The 5-chalcogenyl compounds (compounds 3 and 8) showed stronger inhibition of biofilm formation than the corresponding 3-chalcogenyl compounds (compounds 4 and 5) (, S1). Moreover, we found that compounds 1, 2, 3, and 8 showed similarly strong inhibitory activity against the hyphal formation and pathogenicity of C. albicans (), which might indicate that the biological functions are attributable to the quinoline and indole skeletons. Our data indicate that the main backbone of these compounds could provide a design direction for new drugs to treat C. albicans infection. Surprisingly, minor modification of the branched structures may lead to a large change in the biological activities, suggesting important points for the further design of diaryl chalcogenides as antimicrobial agents against fungal pathogens.
The transformation from yeast to hypha is considered an essential step in the C. albicans pathogenesis [Citation54–56]. As morphological transitions play a vital role in the pathogenesis of C. albicans, previous studies have already focused on how to inhibit hyphal formation in fungal pathogens [Citation5,Citation19,Citation57]. Our results showed that diaryl chalcogenide compounds have a strong inhibitory effect on the hyphal formation of C. albicans (, S3, S4). Intriguingly, some compounds displayed good effects on both biofilm and hyphal formation of C. albicans (, S3, S4). These compounds also weakened the C. albicans pathogenicity in both cell lines and mouse infection models (). These compounds have therapeutic effects on a clinical drug-resistant C. albicans strain and exhibit favourable synergy with antifungal agents on this drug-resistant strain (). At the same time, the compound can significantly reduce the content of Candida in mouse intestinal microbiota and restore the composition and richness of mouse intestinal microbiota (Figure S8). Taken together, our findings in this study provide additional evidence for the design of antifungal drugs or adjuvants of antimicrobial agents by using a function-based approach that relies on the inhibition of biofilm and hyphal formation.
In conclusion, we have screened and evaluated the effectiveness of a series of diaryl chalcogenide derivatives against C. albicans infection. Interestingly, several compounds remarkably reduced the virulence of C. albicans by inhibiting hyphal formation and biofilm formation; but showed no cytotoxicity to human cells. Our results also showed that these compounds exert excellent synergistic effects with antifungal drugs against the clinical drug-resistant C. albicans strain HCH12. The compound 5-sulfenylindole showed an obvious advantage over fluconazole as it could also restore the composition and richness of the intestinal microbiota in mice infected by C. albicans. Together, these results suggest that diaryl chalcogenides can potentially be designed as novel clinical therapeutics against C. albicans infection. But how to use the compounds for OPC? One idea is to add the compounds to the mouthwash or wound mask, which can also be used in combination with other antifungal drugs. This strategy could also be used in immunocompromised patient.
Author contributions
Conceptualization, Y.D. and S.S.; methodology, S.S., S.Z. and X.S.; validation, S.S., S.Z., X.S., L.M., Z.W., J.L., H.T.; formal analysis, S.S., S.Z., X.S., L.M., Z.W., J.L., H.T., M.Z. and Y.D.; investigation, Y.D. and S.S.; resources, Y.D.; data curation, Y.D., S.S., S.Z. and X.S.; writing-original draft preparation, Y.D., S.S. and S.Z.; writing-review and editing, Y.D. and S.S.; supervision, Y.D. and S.S.; funding acquisition, Y.D. and S.S. All authors have read and agreed to the published version of the manuscript.
Supplemental Material
Download MS Word (3.3 MB)Disclosure statement
No potential conflict of interest was reported by the author(s).
Data Availability statement
The data presented in this study are available in the supplementary materials.
Supplementary material
Supplemental data for this article can be accessed online at https://doi.org/10.1080/21505594.2023.2265012
Additional information
Funding
References
- Yano J, Peters BM, Noverr MC, et al. Novel mechanism behind the immunopathogenesis of vulvovaginal candidiasis: “neutrophil anergy”. Infect Immun. 2018;86(3):e00684–13. doi: 10.1128/IAI.00684-17
- Madhani HD, Fink GR. The control of filamentous differentiation and virulence in fungi. Trends Cell Biol. 1998;8(9):348–353. doi: 10.1016/s0962-8924(98)01298-7
- Brown AJ, Gow NA. Regulatory networks controlling Candida albicans morphogenesis. Trends Microbiol. 1999;7:333–338. doi: 10.1016/s0966-842x(99)01556-5
- Staib P, Kretschmar M, Nichterlein T, et al. Differential activation of a Candida albicans virulence gene family during infection. Proc Natl Acad Sci. 2000;97(11):6102–6107. doi: 10.1073/pnas.110031497
- Fazly A, Jain C, Dehner AC, et al. Chemical screening identifies filastatin, a small molecule inhibitor of Candida albicans adhesion, morphogenesis, and pathogenesis. Proc Natl Acad Sci. 2013;110(33):13594–13599. doi: 10.1073/pnas.1305982110
- Graham CE, Cruz MR, Garsin DA, et al. Enterococcus faecalis bacteriocin EntV inhibits hyphal morphogenesis, biofilm formation, and virulence of Candida albicans. Proc Natl Acad Sci. 2017;114(17):4507–4512. doi: 10.1073/pnas.1620432114
- Wu S, Wang Y, Liu N, et al. Tackling fungal resistance by biofilm inhibitors. J Med Chem. 2017;60(6):2193–2211. doi: 10.1021/acs.jmedchem.6b01203
- Wolfmeier H, Pletzer D, Mansour SC, et al. New perspectives in biofilm eradication. ACS Infec Dis. 2018;4:93–106. doi: 10.1021/acsinfecdis.7b00170
- Noble SM, Gianetti BA, Witchley JN. Candida albicans cell-type switching and functional plasticity in the mammalian host. Nat Rev Microbiol. 2017;15(2):96–108. doi: 10.1038/nrmicro.2016.157
- Nantel A, Dignard D, Bachewich C, et al. Transcription profiling of Candida albicans cells undergoing the yeast-to-hyphal transition. Mol Biol Cell. 2002;13(10):3452–3465. doi: 10.1091/mbc.e02-05-0272
- Kadosh D, Johnson AD. Induction of the Candida albicans filamentous growth program by relief of transcriptional repression: a genome-wide analysis. Mol Biol Cell. 2005;16(6):2903–2912. doi: 10.1091/mbc.e05-01-0073
- Carlisle PL, Banerjee M, Lazzell A, et al. Expression levels of a filament-specific transcriptional regulator are sufficient to determine Candida albicans morphology and virulence. Proc Natl Acad Sci. 2009;106(2):599–604. doi: 10.1073/pnas.0804061106
- Moyes DL, Wilson D, Richardson JP, et al. Candidalysin is a fungal peptide toxin critical for mucosal infection. Nature. 2016;532(7597):64–68. doi: 10.1038/nature17625
- Nobile CJ, Johnson AD. Candida albicans biofilms and human disease. Ann Rev Microbiol. 2015;69:71–92. doi: 10.1146/annurev-micro-091014-104330
- Hornby JM, Jensen EC, Lisec AD, et al. Quorum sensing in the dimorphic fungus Candida albicans is mediated by farnesol. Appl Environ Microbiol. 2001;67(7):2982–2992. doi: 10.1128/AEM.67.7.2982-2992.2001
- Chen H, Fujita M, Feng Q, et al. Tyrosol is a quorum-sensing molecule in Candida albicans. Proc Natl Acad Sci. 2004;101(14):5048–5052. doi: 10.1073/pnas.0401416101
- Ramage G, Saville SP, Wickes BL, et al. Inhibition of Candida albicans biofilm formation by farnesol, a quorum-sensing molecule. Appl Environ Microbiol. 2002;68:5459–5463. doi: 10.1128/AEM.68.11.5459-5463.2002
- Cao YY, Cao YB, Xu Z, et al. cDNA microarray analysis of differential gene expression in Candida albicans biofilm exposed to farnesol. Antimicrob Agents Ch. 2005;49(2):584–589. doi: 10.1128/AAC.49.2.584-589.2005
- Zhao S, Huang JJ, Sun X, et al. (1-aryloxy-2-hydroxypropyl)-phenylpiperazine derivatives suppress Candida albicans virulence by interfering with morphological transition. Microbiol Biotechnol. 2018;11(6):1080–1089. doi: 10.1111/1751-7915.13307
- Meng L, Zhao H, Zhao S, et al. Inhibition of yeast-to-hypha transition and virulence of Candida albicans by 2-alkylaminoquinoline derivatives. Antimicrob Agents Ch. 2019;63(4):e01891–18. doi: 10.1128/AAC.01891-18
- Meng L, Sun C, Zhang C, et al. Efficacy of compounds isolated from Streptomyces olivaceus against the morphogenesis and virulence of Candida albicans. Mar Drugs. 2019;17:442. doi: 10.3390/md17080442
- Song S, Sun X, Meng L, et al. Antifungal activity of hypocrellin compounds and their synergistic effects with antimicrobial agents against Candida albicans. Microbiol Biotechnol. 2021;14(2):430–443. doi: 10.1111/1751-7915.13601
- El-Sharief AM, Moussa Z. Synthesis, characterization and derivatization of some novel types of mono- and bis-imidazolidineiminothiones and imidazolidineiminodithiones with antitumor, antiviral, antibacterial and antifungal activities–part I. Eur J Med Chem. 2009;44:4315–4334. doi: 10.1016/j.ejmech.2009.07.019
- Ilardi EA, Vitaku E, Njardarson JT. Data-mining for sulfur and fluorine: an evaluation of pharmaceuticals to reveal opportunities for drug design and discovery. J Med Chem. 2014;57(7):2832–2842. doi: 10.1021/jm401375q
- Patel VM, Schwartz RA, Lambert WC. Topical antiviral and antifungal medications in pregnancy: a review of safety profiles. J Eur Acad Dermatol Venereol. 2017;31(9):1440–1446. doi: 10.1111/jdv.14297
- Tan Z, Liang Y, Yang J, et al. Site-specific oxidative C-H chalcogenation of (hetero) aryl-fused cyclic amines enabled by nanocobalt oxides. Org Lett. 2018;20:6554–6558. doi: 10.1021/acs.orglett.8b02889
- Lee KL, Buckley HR, Campbell CC. An amino acid liquid synthetic medium for the development of mycelial and yeast forms of Candida albicans. Sabouraudia. 1975;13:148–153. doi: 10.1080/00362177585190271
- Gimeno CJ, Ljungdahl PO, Styles CA, et al. Unipolar cell divisions in the yeast S. cerevisiae lead to filamentous growth: regulation by starvation and RAS. Cell. 1992;68(6):1077–1090. doi: 10.1016/0092-8674(92)90079-r
- Maidan MM, De Rop L, Serneels J, et al. The G protein-coupled receptor Gpr1 and the galpha protein Gpa2 act through the cAMP-protein kinase a pathway to induce morphogenesis in Candida albicans. Mol Biol Cell. 2005;16:1971–1986. doi: 10.1091/mbc.e04-09-0780
- Daniels KJ, Park YN, Srikantha T, et al. Impact of environmental conditions on the form and function of Candida albicans biofilms. Eukaryotic Cell. 2013;12:1389–1402. doi: 10.1128/EC.00127-13
- Reynolds TB, Fink GR. Bakers’ yeast, a model for fungal biofilm formation. Science. 2001;291(5505):878–881. doi: 10.1126/science.291.5505.878
- Barchiesi F, Calabrese D, Sanglard D, et al. Experimental induction of fluconazole resistance in Candida tropicalis ATCC 750. Antimicrob Agents Ch. 2000;44:1578–1584. doi: 10.1128/AAC.44.6.1578-1584.2000
- Solis NV, Filler SG. Mouse model of oropharyngeal candidiasis. Nat Protoc. 2012;7(4):637–642. doi: 10.1038/nprot.2012.011
- Chen L, Wang Z, Liu L, et al. Cinnamaldehyde inhibits Candida albicans growth by causing apoptosis and its treatment on vulvovaginal candidiasis and oropharyngeal candidiasis. Appl Microbial Biotechnol. 2019;103:9037–9055. doi: 10.1007/s00253-019-10119-3
- Konaté K, Mavoungou JF, Lepengué AN, et al. Antibacterial activity against β-lactamase producing methicillin and Ampicillin-resistant Staphylococcus aureus: fractional inhibitory concentration Index (FICI) determination. Ann Clin Microb Anti. 2012;11:18. doi: 10.1186/1476-0711-11-18
- Sudbery P, Gow N, Berman J. The distinct morphogenic states of Candida albicans. Trends Microbiol. 2004;12(7):317–324. doi: 10.1016/j.tim.2004.05.008
- Homann OR, Dea J, Noble SM, et al. A phenotypic profile of the Candida albicans regulatory network. PLoS Genet. 2009;5(12):e1000783. doi: 10.1371/journal.pgen.1000783
- Sangeorzan JA, Bradley SF, He X, et al. Epidemiology of oral candidiasis in HIV-infected patients: colonization, infection, treatment, and emergence of fluconazole resistance. Am J Med. 1994;97(4):339–346. doi: 10.1016/0002-9343(94)90300-x
- Rhodus NL, Bloomquist C, Liljemark W, et al. Prevalence, density, and manifestations of oral Candida albicans in patients with Sjogren’s syndrome. J Otolaryng. 1997;26:300–305. PMID: 9343767.
- Willis AM, Coulter WA, Fulton CR, et al. Oral candidal carriage and infection in insulin-treated diabetic patients. Diabet Med. 1999;16(8):675–679. doi: 10.1046/j.1464-5491.1999.00134.x
- Lu Y, Su C, Wang A, et al. Hyphal development in Candida albicans requires two temporally linked changes in promoter chromatin for initiation and maintenance. PLoS Biol. 2011;9(7):e1001105. doi: 10.1371/journal.pbio.1001105
- Shareck J, Belhumeur P. Modulation of morphogenesis in Candida albicans by various small molecules. Eukaryot Cell. 2011;10:1004–1012. doi: 10.1128/EC.05030-11
- Hnisz D, Bardet AF, Nobile CJ, et al. A histone deacetylase adjusts transcription kinetics at coding sequences during Candida albicans morphogenesis. PLoS Genet. 2012;8(12):e1003118. doi: 10.1371/journal.pgen.1003118
- Kunz C, Kuntz S, Rudloff S. Intestinal Flora. Adv Exp Med Biol. 2009;639(4):67–79. doi: 10.1007/978-1-4020-8749-3_6
- Heng X, Jiang Y, Chu W. Influence of fluconazole Administration on gut microbiome, intestinal barrier, and immune response in mice. Antimicrob Agents Ch. 2021;65(6):e02552–20. doi: 10.1128/AAC.02552-20
- Kurihara T, Sakamoto Y, Kimura T, et al. Meisenheimer rearrangement of azetopyridoindoles. VIII. Synthesis and antiviral activities of 12-carbaeudistomin analogs. Chem Pharm Bull. 1996;44(5):900–908. doi: 10.1248/cpb.44.900
- Marciniec K, Pawelczak B, Latocha M, et al. Synthesis, anti-breast cancer activity, and molecular docking study of a new group of acetylenic quinolinesulfonamide derivatives. Molecules (Basel, Switzerland). 2017;22:300. doi: 10.3390/molecules22020300
- Xu GF, Song BA, Bhadury PS, et al. Synthesis and antifungal activity of novel s-substituted 6-fluoro-4-alkyl(aryl)thioquinazoline derivatives. Bioorgan Med Chem. 2007;15(11):3768–3774. doi: 10.1016/j.bmc.2007.03.037
- Empel A, Kisiel E, Wojtyczka RD, et al. Synthesis and antimicrobial activity of sulfur derivatives of quinolinium salts. Molecules (Basel, Switzerland). 2018;23:218. doi: 10.3390/molecules23010218
- Liu RH, Shang ZC, Li TX, et al. In vitro antibiofilm activity of eucarobustol E against Candida albicans. Antimicrob Agents Ch. 2017;61(8):2707–2716. doi: 10.1128/AAC.02707-16
- Zhong H, Hu DD, Hu GH, et al. Activity of sanguinarine against Candida albicans biofilms. Antimicrob Agents Ch. 2017;61(5):2259–2316. doi: 10.1128/AAC.02259-16
- Pandolfi F, D’Acierno F, Bortolami M, et al. Searching for new agents active against Candida albicans biofilm: a series of indole derivatives, design, synthesis and biological evaluation. Eur J Med Chem. 2019 [Epub 2019 Jan 9];165:93–106.
- Reginatto P, Joaquim A, Rocha D, et al. 8-hydroxyquinoline and quinazoline derivatives as potential new alternatives to combat Candida spp biofilm. Lett Appl Microbiol. 2022;74:395–404. doi: 10.1111/lam.13607
- Braun BR, Johnson AD. TUP1, CPH1 and EFG1 make independent contributions to filamentation in Candida albicans. Genetics. 2000;155(1):57–67. doi: 10.1093/genetics/155.1.57
- Saville SP, Lazzell AL, Monteagudo C, et al. Engineered control of cell morphology in vivo reveals distinct roles for yeast and filamentous forms of Candida albicans during infection. Eukaryotic Cell. 2003;2:1053–1060. doi: 10.1128/EC.2.5.1053-1060.2003
- Zheng XD, Wang YM, Wang Y. CaSPA2 is important for polarity establishment and maintenance in Candida albicans. Mol Microbiol. 2003;49(5):1391–1405. doi: 10.1046/j.1365-2958.2003.03646.x
- Shirtliff ME, Krom BP, Meijering RA, et al. Farnesol-induced apoptosis in Candida albicans. Antimicrob Agents Ch. 2009;53:2392–2401. doi: 10.1128/AAC.01551-08