ABSTRACT
Clostridium innocuum is an emerging spore-forming anaerobe that is often observed in Clostridioides difficile-associated inflammatory bowel disease (IBD) exacerbations. Unlike C. difficile, C. innocuum neither produces toxins nor possesses toxin-encoding genetic loci, but is commonly found in both intestinal and extra-intestinal infections. Membrane lipid rafts are composed of dynamic assemblies of cholesterol and sphingolipids, allowing bacteria to gain access to cells. However, the direct interaction between C. innocuum and lipid rafts that confers bacteria the ability to disrupt the intestinal barrier and induce pathogenesis remains unclear. In this study, we investigated the associations among nucleotide-binding oligomerization domain containing 2 (NOD2), lipid rafts, and cytotoxicity in C. innocuum-infected gut epithelial cells. Our results revealed that lipid rafts were involved in C. innocuum-induced NOD2 expression and nuclear factor (NF)-κB activation, triggering an inflammatory response. Reducing cholesterol by simvastatin significantly dampened C. innocuum-induced cell death, indicating that the C. innocuum-induced pathogenicity of cells was lipid raft-dependent. These results demonstrate that NOD2 mobilization into membrane rafts in response to C. innocuum-induced cytotoxicity results in aggravated pathogenicity.
Introduction
Clostridium innocuum, a spore-forming Gram-positive anaerobe, is a crucial pathogen for extraintestinal clostridial infections (EICIs) [Citation1], such as peritonitis [Citation2], bacteraemia [Citation3], and endocarditis [Citation4]. We recently reported that C. innocuum is the second most common pathogen causing EICIs, with a higher mortality rate than other clostridial species [Citation5]. Vancomycin-resistant C. innocuum is found in patients with recurrent C. difficile-associated diarrhoea [Citation6]. The incidence of C. innocuum infection in hospitalized patients with inflammatory bowel disease (IBD) is higher than that of C. difficile infection [Citation7]. Notably, the severity of the disease is higher in patients infected with C. innocuum than those infected with C. difficile [Citation8], indicating that C. innocuum is a bona-fide pathogen that causes gastrointestinal and extraintestinal infections.
The virulence factors of C. difficile include germination and outgrowth of spores and two glucosylating toxins (toxin A and toxin B) [Citation9]. Cytotoxins produced by C. difficile play a crucial role in intestinal pathogenesis and are associated with severe colitis [Citation10]. Unlike C. difficile, C. innocuum does not contain any genes encoding cytotoxins, and the bacterial pellets rather than the culture supernatant indeed exhibit cytotoxicity in human colon epithelial cells [Citation11].
Nucleotide-binding oligomerization domain containing 2 (NOD2), a human membrane-associated innate immune receptor, is abundantly expressed in the gut epithelium and sensitizes muramyl dipeptide (MDP) in the bacterial cell wall [Citation12]. Activation of NOD2 induces receptor-interacting protein 2 (RIP2) recruitment, which then initiates nuclear factor (NF)-κB signalling to produce inflammatory cytokines [Citation13]. C. innocuum contains cell wall proteins, similar to C. difficile surface layer proteins, causing cytotoxicity [Citation11]. However, whether NOD2 is involved in C. innocuum-induced pathogenesis remains unknown and requires further investigation.
Lipid rafts are membrane microdomains composed primarily of dynamic assemblies of sphingolipids and cholesterol [Citation14]. Several pathogens exploit lipid rafts for initial attachment to the cell membrane and gain access to the host cells [Citation15–18]. C. innocuum can be predominantly identified from a bacterial consortium in the creeping fat of IBD ileal surgical resections [Citation19]. Destruction of the intestinal barrier enhances C. innocuum translocation from the gut lumen across the barrier and tropism to the mesenteric adipose tissue, implying a preference for lipid-rich environments. However, whether the direct interaction between C. innocuum and lipid rafts confers the bacteria the ability to disrupt the intestinal barrier and induce pathogenesis needs to be investigated. In this study, we explored the molecular mechanisms of C. innocuum-induced pathogenesis in colonic epithelial cells. We further investigated whether NOD2 mobilized to membrane rafts was implicated in C. innocuum-induced cytotoxicity.
Materials and methods
Bacterial culture
C. innocuum was cultured on CDC anaerobe agar containing 5% sheep blood (BD, Franklin Lake, NJ, USA) under anaerobic condition for 2 days at 37°C. C. innocuum was inoculated in Brain-Heart Infusion broth supplement with 0.5% yeast extract and 0.1% L-cysteine as described previously [Citation5].
Cell culture
HT-29 cells (ATCC HTB-38) were cultured in Dulbecco’s Modified Eagle Medium (DMEM) containing 10% foetal bovine serum (FBS) and incubated at 37°C with 5% CO2. For simvastatin treatment, HT-29 cells were cultured in DMEM containing 2% FBS.
Western blot assay
HT-29 cells were infected with C. innocuum at different multiplicity of infections (MOI) for different times. Cell lysates (60 µg) were loaded in 7.5% SDS-PAGE and then transferred onto polyvinylidene difluoride membranes (Millipore, Billerica, MA, USA) for 90 minutes. The membranes were blocked with Tris-buffered saline with Tween 20 (TBST) containing 5% skim milk for 1 h at room temperature. The membranes were then incubated with primary antibodies against NOD2 (GTX306615, dilution 1: 200), RIP2 (CST4142, dilution 1:1000), and β-actin (dilution 1:5000) at 4°C overnight. Th membranes were then incubated with horseradish peroxidase (HRP)-conjugated antibody for 1 h. The proteins of interest were detected with ECL Western Blotting Detection Reagent (BIOMAN, Taipei, Taiwan) and visualized by Azure c400 system and AzureSpot analysis Software (Azure Biosystems, Dublin, CA, USA).
Immunofluorescence assay
HT-29 cells (1 × 105) were seeded in 6-cm dishes and cultured for 16–18 h. Cells were pretreated with simvastatin (20 µM) for 30 min followed by infection with C. innocuum at MOI of 100 for 4 h. Cells were fixed with 2% paraformaldehyde for 1 h and blocked with 3% skim milk for 1 h at room temperature. Cells were incubated with primary antibodies against NOD2 (GTX306615, dilution 1:400) overnight at 4°C, followed by Alexa FITC 488-conjugated goat anti-mouse IgG (dilution 1:1000, Invitrogen) and CTx-B Alexa Fluor 555-conjugate (dilution 1:200, Invitrogen) for 1 h at room temperature. Cells were stained with Hoechst 33,342 (dilution 1:1000) for 30 min at room temperature. The samples were observed under a confocal laser-scanning microscope (LSM780, Carl Zeiss, Germany) and the intensity of fluorescence was analysed by ZEN software (Carl Zeiss).
Cell viability assay
HT-29 cells (1 × 104) were seeded in 96-well plates and cultured for 16–18 h. Cells were respectively treated with C. innocuum (MOI = 500), C. innocuum culture broth, heat-treated C. innocuum, and cell lysate of C. innocuum for 24 h. Cell viability was determined by 3-(4,5-dimethylthiazol-2-yl)–2,5-diphenyltetrazolium bromide (MTT) assay (Sigma-Aldrich, St. Louis, MO, USA). Cells were incubated with MTT reagent (0.5 mg/ml) at 37°C for 3 h. Isopropanol containing HCl was used to resolve the purple crystal for 30 min. The optical density at 570 nm was measured by SpectraMax iD3 Multi-Mode Microplate Reader (Molecular Device, San Jose, CA, USA).
Analysis of apoptotic cells
HT-29 cells (2 × 106) were seeded in 6-cm dishes and cultured for 16–18 h. Cells were respectively treated with C. innocuum (MOI = 500), C. innocuum culture broth, heat-treated C. innocuum, and cell lysate of C. innocuum for 24 h. BHI broth was used as a negative control. The treated cells were collected and incubated with FITC-Annexin V in a buffer containing propidium iodide (PI) using the FITC Annexin V Apoptosis Detection Kit (BD Biosciences, San Jose, CA, USA) according to the manufacturer’s instructions. Fluorescence was analysed by FACS Calibur System (BD Biosciences) by collecting 10,000 cells from each sample. All samples were performed of three independent experiments.
Luciferase activity assay
HT-29 cells (2 × 104) were seeded in 24-well plates and cultured for 16–18 h. pNF-κB-Luc (1 μg) and pGL3-Luc (1 μg) plasmids were co-transfected to cells by using jetPRIME (Polyplus-transfection, Illkirch-Graffenstaden, France) for 4 h. After transfection, cells were treated with simvastatin (100 μM) or treated with simvastatin then replenished with cholesterol (400 μg/ml), following by infection with C. innocuum (MOI = 500) for an additional 24 h. Dual-Luciferase Reporter Assay System (Promega, Madison, WI, USA) was used to analyse the luciferase reporter activity by following the manufacturer’s instruction [Citation20]. The luciferase activity was measured by SpectraMax iD3 Multi-Mode Microplate Reader (Molecular Device).
Analysis of IL-8 production
HT-29 cells (2 × 104) were seeded in 24-well plates and cultured for 16–18 h. Cells were treated with simvastatin (100 μM) or treated with simvastatin and replenished with cholesterol (400 μg/ml), following by infection with C. innocuum (MOI = 500) for an additional 24 h. The culture supernatant was collected and subjected to enzyme-linked immunosorbent assay (ELISA) according to the manufacturer’s instructions (R&D Systems, Minneapolis, MN, USA).
Statistical analysis
All experiments were performed in triplicate repeats independently. Student’s t-test was used to determine the statistical difference between two groups. The difference was considered significant when P < 0.05. Statistical analysis was conducted by using Prism Program (version 9.0.0 for Windows, GraphPad Software, La Jolla, CA, USA).
Results
C. innocuum induces NOD2 expression in colonic epithelial cells
Human colonic epithelial cells, HT-29, were used to determine NOD2 expression during C. innocuum infection. Our results showed that NOD2 and RIP2 expression levels in HT-29 cells were increased upon C. innocuum infection for 0–4 h (). The cells were then infected with C. innocuum at different MOIs (0–100). Both NOD2 and RIP2 levels were gradually elevated in cells infected with C. innocuum with an increased MOI (). These results suggest that NOD2 elevation may be involved in C. innocuum-induced inflammation of colonic epithelial cells.
Figure 1. C. innocuum induces nucleotide-binding oligomerization domain containing 2 (NOD2) and receptor-interacting protein 2 (RIP2) expression. HT-29 cells were infected with C. innocuum at (A) multiplicity of infection (MOI) of 100 for 0–4 h, and (B) MOI of 0–100 for 4 h. Expression levels of NOD2 and RIP2 were determined using western blotting. The protein expression levels of NOD2 and RIP2 were quantified by densitometric analysis and normalized to β-actin level, respectively, and indicated at the bottom of each lane.
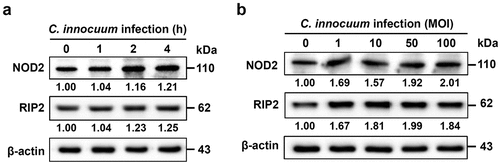
Mobilization of NOD2 to lipid rafts in C. innocuum-infected cells
Next, we explored whether C. innocuum triggered NOD2 recruitment to membrane rafts. NOD2 was located on the cell membrane in the absence of C. innocuum (). In cells infected with C. innocuum, NOD2 formed punctures at sites of bacterial attachment around the inner membrane and co-localized with CTX-B, which bound to ganglioside GM1 and served as a raft-marker. The merged image was then analysed using confocal microscopy z-section, which showed that the distribution of fluorescence intensity for C. innocuum and NOD2 was consistent with that of the raft-marker CTx-B across the cytoplasmic membrane (, right panels). These findings indicate that during C. innocuum infection, attached bacteria coalesce with NOD2, which is abundantly located in the membrane rafts.
Figure 2. C. innocuum recruits NOD2 to membrane rafts. HT-29 cells were infected with C. innocuum at MOI of 100 for 4 h. Cells were probed with (A and E) Hoechst 33,342 (blue), (B and F) anti-NOD2 (green), and (C and G) CTx-B (red), followed by performing confocal microscopy analysis. (E) arrowheads indicate the attached bacteria on the cell membrane. (D and H) yellow arrows indicate the area of confocal z-section analysis. Bars, 5 μm. White lines across the membrane shown in z-section indicate the distribution of fluorescence signals for C. innocuum (blue line), NOD2 (green line), and CTx-B (red line), which present as line intensity histograms in the right panels.
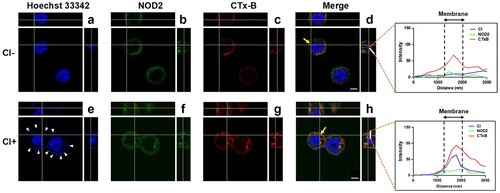
Live C. innocuum is essential for inducing cell death
We recently reported that C. innocuum was cytotoxic to gut epithelial cells and caused severe pathogenic changes in mouse ileum [Citation11]. Here, we further explored the mechanism by which C. innocuum induced cell death in human colonic epithelial cells. Live C. innocuum, bacterial culture supernatant, heat-killed cells, and bacterial lysates were examined for their cytotoxic activity in HT-29 cells. Our results showed that only live C. innocuum significantly decreased the cell viability, whereas other bacterial constituents did not influence the cell survival, as analysed by the MTT assay (). We then explored whether C. innocuum-induced cell death was mediated via the apoptotic pathway. Cells were treated with each bacterial preparation and stained with annexin V/PI, followed by flow cytometric analysis. Consistently, the proportion of apoptotic cells was remarkably increased in cells treated with live C. innocuum (). However, this trend was not observed in the bacterial supernatant or heat-killed bacteria. These results demonstrate that C. innocuum-induced cell death occurs via apoptosis and viable bacteria are required for this effect.
Figure 3. Live C. innocuum is essential for inducing cell death. HT-29 cells were treated with live C. innocuum, bacterial culture supernatant, heat-killed bacteria, and bacterial lysate for 24 h, followed by the (A) analysis of cell survival using 3-(4,5-dimethylthiazol-2-yl)-2,5-diphenyl tetrazolium bromide (MTT) assay, (B) stained with annexin V/propidium iodide, and analyzed using flow cytometry. (C) percentage of apoptotic cells was quantitated. *, P < 0.05.
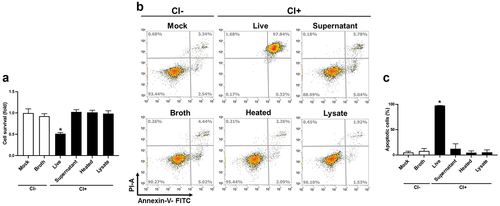
Reducing cholesterol levels ameliorates C. innocuum-induced cell death
We further investigated whether cholesterol was crucial for C. innocuum-induced pathogenesis. Simvastatin, an inhibitor of 3-hydroxy-3-methylglutaryl-coenzyme A reductase (HMG-CoA reductase), was used to impede cellular cholesterol synthesis, and NOD2 expression on the cell membrane was analysed. Immunofluorescence staining showed that C. innocuum infection recruited a large proportion of NOD2 co-localized with CTx-B to the inner membrane compared to the bacterial uninfected group (). In contrast, pretreatment of cells with simvastatin resulted in the reduction of NOD2 and CTx-B levels.
Figure 4. Inhibition of cholesterol synthesis reduces C. innocuum-induced NOD2 expression in lipid rafts. HT-29 cells were pretreated with simvastatin (100 μM) for 1 h prior to C. innocuum infection. Cells were probed with Hoechst 33,342 (blue), anti-NOD2 (green), and CTx-B (red), followed by confocal microscopy analysis. Bars, 5 μm.
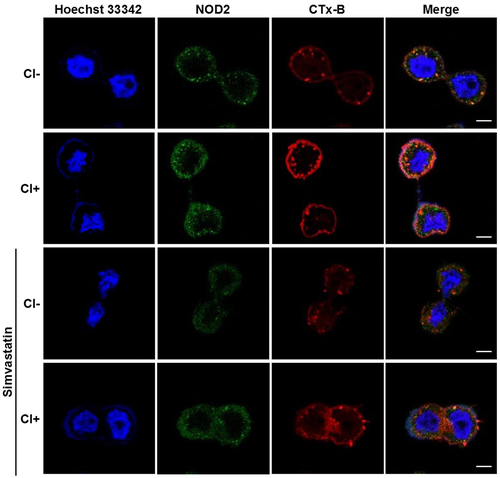
Effect of cholesterol inhibition on the cytotoxicity of HT-29 cells was then analysed. As shown in , pretreatment of cells with simvastatin, the cells were exposed to C. innocuum for 24 h, and the proportion of apoptotic cells was reduced. In contrast, the inhibitory effect of simvastatin on C. innocuum-induced apoptosis was abolished by cholesterol replenishment (). We then examined whether cholesterol is crucial for C. innocuum-induced inflammation in gut epithelial cells. Our results showed that C. innocuum infection remarkably increased NF-κB luciferase activity along with IL-8 production (). Consistently, after pretreatment of cells with simvastatin significantly decreased the C. innocuum-induced NF-κB promoter activity and IL-8 production. These results demonstrate that sufficient cholesterol, present in lipid rafts, is essential for C. innocuum-associated pathogenesis.
Figure 5. Simvastatin attenuates C. innocuum-induced apoptosis. (A) HT-29 cells were pretreated with simvastatin or pretreated with simvastatin then replenished with cholesterol (400 μg/mL). Cells were infected with C. innocuum for 24 h and stained with annexin V/propidium iodide, followed by flow cytometry analysis. (B) percentage of apoptotic cells was quantitated. (C) HT-29 cells were co-transfected with the nuclear factor (NF-κB) and pGL3 luciferase reporters and treated with simvastatin (100 μM) for 1 h, followed by C. innocuum infection for 24 h. NF-κB promoter activity was determined and normalized to pGL3 luciferase activity. (D) IL-8 production was analyzed by using ELISA. *, P < 0.05.
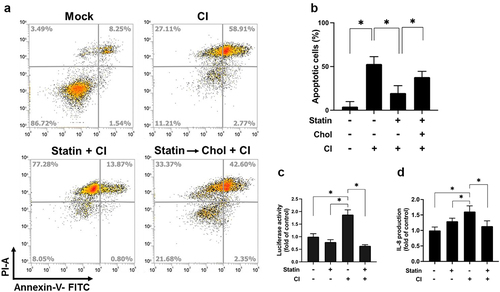
Discussion
NOD2 can recognize MDP, which is mainly derived from the peptidoglycan of Gram-positive bacteria [Citation12,Citation21]. NOD2 activation promotes the recruitment of RIP2 to elicit the NF-κB and mitogen-activated protein kinase (MAPK) pathways [Citation22], leading to the production of several cytokines/chemokines, such as interleukin (IL)-1β, IL-6, IL-8, and tumour necrosis factor (TNF)-α [Citation23,Citation24]. In contrast, NOD2 mutations render it unresponsive to the bacterial cell wall component, MDP, revealing its important role in disease susceptibility and pathogenesis [Citation25]. In line with previous studies, the current study demonstrated the impact of C. innocuum on the NOD2 pathway, which is crucial for the interaction of bacteria to trigger pathogenicity.
The present study showed that membrane-associated NOD2 was mobilized by C. innocuum to the inner membrane rather than the cell surface. These results are consistent with previous findings that NOD2 interacts with the inner side of the membrane rather than outer leaflet [Citation26]. In addition, NOD2 recruits autophagy-related 16-like 1 (ATG16L1) to the cell membrane and facilitates the formation of autophagosomes around the invading bacteria [Citation27]. Biochemical analyses showed that FERM and PDZ domain-containing 2 (FRMPD2) was selectively localized at the basolateral membrane in polarized epithelial cells interacting with the leucine-rich repeats of NOD2, indicating that FRMPD2 acts as a membrane anchor for NOD2 [Citation28]. These findings indicate that membrane localization of NOD2 is not only important for bacterial entry, but also for maintaining the intestinal epithelial cell tolerance to commensal bacteria present at the apical side of the cells.
Membrane rafts are enriched in cholesterol and sphingolipids, which provide entry gates for bacteria and their toxin internalization, resulting in the deterioration of infectious diseases [Citation29–31]. C. difficile transferase (CDT)-induced microtubule-based membrane protrusions rely on lipid rafts in human colon cells, indicating that CDT-action is lipid raft-dependent [Citation32]. In addition, C. difficile SLP binds to membrane cholesterol microdomains and triggers inflammasome activation [Citation17]. In the present study, we demonstrated that C. innocuum infection mobilizes NOD2 to lipid rafts, leading to the activation of NF-κB signalling, IL-8 production, and exacerbation of the cytotoxic effect on colonic epithelial cells. These results suggest that the virulence factors of C. innocuum are similar to those of C. difficile cell wall components (SLP and CwpV) [Citation5,Citation11,Citation33], indicating that lipid rafts are essential for the action of virulence factors of both C. innocuum and C. difficile. Although two hypothetical proteins (CI_01447 and CI_01448) have been identified in C. innocuum, they are not cytotoxic to mammalian cells [Citation34]. Different from C. difficile, the exact virulence factors that respond to C. innocuum-induced pathogenesis of colonic epithelial cells remain unknown.
Cholesterol usurping/depleting agents have been employed to ameliorate infectious agents by inhibiting their toxin-actions or preventing pathogen entry into host cells [Citation35]. For example, statins, inhibitors of HMG-CoA reductase, effectively alleviate microbial infectivity [Citation36–38]. Most importantly, statins can lower the cholesterol levels, which significantly decreases the risk [Citation39] and improves the mortality of C. difficile infection [Citation40,Citation41] Consistently, a retrospective matched case-control study showed that statin prescription has a protective effect against new-onset IBD, including ulcerative colitis and Crohn’s disease [Citation42]. C. innocuum promotes the formation of creeping fat and prefers a lipid-rich environment [Citation19,Citation43]. However, to date, no studies have reported the association of C. innocuum with cholesterol and statin use for treating C. innocuum-related diseases. To the best of our knowledge, the present study is the first to reveal the essential role of cholesterol in the association of C. innocuum with membrane rafts, followed by the exacerbation of cytotoxicity. Our findings indicate that pharmaceutical agents that lower cholesterol levels can be developed to alleviate both C. innocuum- and C. difficile-related complications.
Owing to its intrinsic vancomycin-resistant nature, C. innocuum appears to be an opportunistic gut pathogen that causes gut dysbiosis [Citation44]. C. innocuum co-infection with C. difficile commonly leads to a poorer clinical outcome than C. difficile infection alone in ulcerative colitis [Citation7]. C. innocuum bacteraemia can be complicated by cytomegalovirus colitis [Citation45]. In a patient with coronavirus disease 2019 (COVID-19), co-infection with cytomegalovirus and C. innocuum causes ulcer bleeding in the rectum [Citation46]. Furthermore, patients with persistent respiratory symptoms are positively correlated with gut microbiota, including C. innocuum [Citation47]. Together, these findings indicate that gut dysbiosis may provide a beneficial environment for C. innocuum, which has a survival advantage and ultimately causes various diseases. However, C. innocuum can be misidentified during co-infections with C. innocuum and C. difficile [Citation48]. Virulence factors of C. innocuum responsible for its pathogenicity remain to be identified. In addition, the detailed mechanism of the interactions of C. innocuum with other gut pathogens that collaboratively exacerbate disease complications remains unclear. Moreover, precise approaches for identifying C. innocuum infections need to be explored in future studies.
In conclusion, our results showed that C. innocuum infection coalesced NOD2 to mobilize it in membrane rafts (). We further demonstrated that C. innocuum-induced apoptosis of cells and viable bacteria were essential for the effect. Moreover, reducing cellular cholesterol levels using simvastatin decreased the NF-κB promoter activity, IL-8 production, and ameliorated C. innocuum-induced cytotoxicity, revealing that the C. innocuum-induced pathogenicity of cells is lipid raft-dependent. Unraveling the mechanism of C. innocuum-induced cytotoxicity in cells may contribute to the development of new strategies for controlling and treating C. innocuum-associated diseases.
Figure 6. Hypothesized model illustrates C. innocuum-induced pathogenicity of intestinal epithelial cells. C. innocuum infection coalesces lipid rafts on the cell membrane, which then activates NOD2 pathway to promote NF-κB translocation in the nucleus, resulting in exacerbates cytotoxicity and inflammation of intestinal epithelial cells.
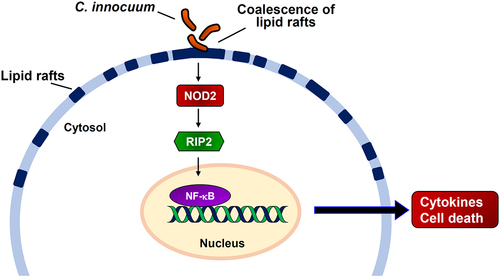
Author’s contributions
Conception and design of this work: CCN, YMC, CHC, CHL
Experimental study: HYW, CJK, CHC, MWH, CLC, TSH, YCC
Data analysis and interpretation: HYW, CJK, CHC, MWH, CLC
Writing the manuscript: CHC, CHL
Final approval: all authors.
Acknowledgements
The authors would like to thank the editor and reviewers for the editorial assistance and their valuable comments. The authors sincerely thank the Microscopy Center at Chang Gung University for technical assistance.
Disclosure statement
No potential conflict of interest was reported by the author(s).
Data availability statement
The authors confirm that the data supporting the findings of this study are available within the article.
Additional information
Funding
References
- Cherny KE, Muscat EB, Reyna ME, et al. Clostridium innocuum: microbiological and clinical characteristics of a potential emerging pathogen. Anaerobe. 2021;71(102418):1–10. doi: 10.1016/j.anaerobe.2021.102418
- Aroca-Ferri M, Suarez-Hormiga L, Bosch-Benitez-Parodi E, et al. Peritonitis by Clostridium innocuum associated to peritoneal dialysiss. Rev Esp Quimioter. 2019;32(2):192–193. doi: 10.1016/j.eimc.2012.01.023
- Castiglioni B, Gautam A, Citron DM, et al. Clostridium innocuum bacteremia secondary to infected hematoma with gas formation in a kidney transplant recipient. Transpl Infect Dis. 2003;5(4):199–202. doi: 10.1111/j.1399-3062.2003.00037.x
- Cutrona AF, Watanakunakorn C, Schaub CR, et al. Clostridium innocuum endocarditis. Clin Infect Dis. 1995;21(5):1306–1307. doi: 10.1093/clinids/21.5.1306
- Chia JH, Feng Y, Su LH, et al. Clostridium innocuum is a significant vancomycin-resistant pathogen for extraintestinal clostridial infection. Clin Microbiol Infect. 2017;23(8):560–566. doi: 10.1016/j.cmi.2017.02.025
- Ackermann G, Tang YJ, Jang SS, et al. Isolation of Clostridium innocuum from cases of recurrent diarrhea in patients with prior Clostridium difficile associated diarrhea. Diagn Microbiol Infect Dis. 2001;40(3):103–106. doi: 10.1016/S0732-8893(01)00259-0
- Le PH, Chiu CT, Yeh PJ, et al. Clostridium innocuum infection in hospitalised patients with inflammatory bowel disease. J Infect. 2022;84(3):337–342. doi: 10.1016/j.jinf.2021.12.031
- Chen YC, Kuo YC, Chen MC, et al. Case-control study of Clostridium innocuum infection, Taiwan. Emerg Infect Dis. 2022;28(3):599–607. doi: 10.3201/eid2803.204421
- Janoir C. Virulence factors of Clostridium difficile and their role during infection. Anaerobe. 2016;37:13–24. doi: 10.1016/j.anaerobe.2015.10.009
- Voth DE, Ballard JD. Clostridium difficile toxins: mechanism of action and role in disease. Clin Microbiol Rev. 2005;18(2):247–263. doi: 10.1128/CMR.18.2.247-263.2005
- Chia JH, Wu TS, Wu TL, et al. Clostridium innocuum is a vancomycin-resistant pathogen that may cause antibiotic-associated diarrhoea. Clin Microbiol Infect. 2018;24(11):1195–1199. doi: 10.1016/j.cmi.2018.02.015
- Girardin SE, Boneca IG, Viala J, et al. Nod2 is a general sensor of peptidoglycan through muramyl dipeptide (MDP) detection. J Biol Chem. 2003;278(11):8869–8872. doi: 10.1074/jbc.C200651200
- Hasegawa M, Fujimoto Y, Lucas PC, et al. A critical role of RICK/RIP2 polyubiquitination in nod-induced NF-kappaB activation. EMBO J. 2008;27(2):373–383. doi: 10.1038/sj.emboj.7601962
- Simons K, Ehehalt R. Cholesterol, lipid rafts, and disease. J Clin Invest. 2002;110(5):597–603. doi: 10.1172/JCI0216390
- Rogers TJ, Thorpe CM, Paton AW, et al. Role of lipid rafts and flagellin in invasion of colonic epithelial cells by Shiga-toxigenic Escherichia coli O113: H21. Infect Immun. 2012;80(8):2858–2867. doi: 10.1128/IAI.00336-12
- Hsu CY, Yeh JY, Chen CY, et al. Helicobacter pylori cholesterol-alpha-glucosyltransferase manipulates cholesterol for bacterial adherence to gastric epithelial cells. Virulence. 2021;12(1):2341–2351. doi: 10.1080/21505594.2021.1969171
- Chen Y, Huang K, Chen LK, et al. Membrane cholesterol is crucial for Clostridium difficile surface Layer protein binding and triggering inflammasome activation. Front Immunol. 2020;11:1675. doi: 10.3389/fimmu.2020.01675
- Chen Y, Jin H, Tang X, et al. Cell membrane-anchored anti-HIV single-chain antibodies and bifunctional inhibitors targeting the gp41 fusion protein: new strategies for HIV gene therapy. Emerg Microbes Infect. 2022;11(1):30–49. doi: 10.1080/22221751.2021.2011616
- CWY H, Martin A, Sepich-Poore GD, et al. Translocation of viable gut microbiota to mesenteric adipose drives formation of creeping fat in humans. Cell. 2020;183(3):666–683 e617. doi: 10.1016/j.cell.2020.09.009
- Lai CH, Lin TL, Huang MZ, et al. Gut commensal parabacteroides goldsteinii MTS01 alters gut microbiota composition and reduces cholesterol to mitigate Helicobacter pylori-induced pathogenesis. Front Immunol. 2022;13(916848). doi: 10.3389/fimmu.2022.916848
- Kobayashi KS, Chamaillard M, Ogura Y, et al. Nod2-dependent regulation of innate and adaptive immunity in the intestinal tract. Science. 2005;307(5710):731–734. doi: 10.1126/science.1104911
- Caruso R, Warner N, Inohara N, et al. NOD1 and NOD2: signaling, host defense, and inflammatory disease. Immunity. 2014;41(6):898–908. doi: 10.1016/j.immuni.2014.12.010
- Biswas A, Liu YJ, Hao L, et al. Induction and rescue of Nod2-dependent Th1-driven granulomatous inflammation of the ileum. Proc Natl Acad Sci U S A. 2010;107(33):14739–14744. doi: 10.1073/pnas.1003363107
- Magalhaes JG, Fritz JH, Le Bourhis L, et al. Nod2-dependent Th2 polarization of antigen-specific immunity. J Immunol. 2008;181(11):7925–7935. doi: 10.4049/jimmunol.181.11.7925
- Kim YG, Shaw MH, Warner N, et al. Cutting edge: Crohn’s disease-associated Nod2 mutation limits production of proinflammatory cytokines to protect the host from Enterococcus faecalis-induced lethality. J Immunol. 2011;187(6):2849–2852. doi: 10.4049/jimmunol.1001854
- Barnich N, Aguirre JE, Reinecker HC, et al. Membrane recruitment of NOD2 in intestinal epithelial cells is essential for nuclear factor kappa B activation in muramyl dipeptide recognition. J Cell Bio. 2005;170(1):21–26. doi: 10.1083/jcb.200502153
- Travassos LH, Carneiro LA, Ramjeet M, et al. Nod1 and Nod2 direct autophagy by recruiting ATG16L1 to the plasma membrane at the site of bacterial entry. Nat Immunol. 2010;11(1):55–62. doi: 10.1038/ni.1823
- Kabi A, McDonald C. FRMBP2 directs NOD2 to the membrane. Proc Natl Acad Sci U S A. 2012;109(52):21188–21189. doi: 10.1073/pnas.1219395110
- Lafont F, Tran Van Nhieu G, Hanada K, et al. Initial steps of shigella infection depend on the cholesterol/sphingolipid raft-mediated CD44-IpaB interaction. EMBO J. 2002;21(17):4449–4457. doi: 10.1093/emboj/cdf457
- Abrami L, Liu S, Cosson P, et al. Anthrax toxin triggers endocytosis of its receptor via a lipid raft-mediated clathrin-dependent process. J Cell Bio. 2003;160(3):321–328. doi: 10.1083/jcb.200211018
- Du SY, Wang HJ, Cheng HH, et al. Cholesterol glucosylation by Helicobacter pylori delays internalization and arrests phagosome maturation in macrophages. J Microbiol Immunol Infect. 2016;49(5):636–645. doi: 10.1016/j.jmii.2014.05.011
- Schwan C, Nolke T, Kruppke AS, et al. Cholesterol- and sphingolipid-rich microdomains are essential for microtubule-based membrane protrusions induced by Clostridium difficile transferase (CDT). J Biol Chem. 2011;286(33):29356–29365. doi: 10.1074/jbc.M111.261925
- Reynolds CB, Emerson JE, de la Riva L, et al. The Clostridium difficile cell wall protein CwpV is antigenically variable between strains, but exhibits conserved aggregation-promoting function. PLOS Pathog. 2011;7(4):e1002024. doi: 10.1371/journal.ppat.1002024
- Cherny KE, Balaji A, Mukherjee J, et al. Identification of Clostridium innocuum hypothetical protein that is cross-reactive with C. difficile anti-toxin antibodies. Anaerobe. 2022;75(102555):102555. doi: 10.1016/j.anaerobe.2022.102555
- Gagliardi MC, Iwabuchi K, Lai CH. Editorial: role of lipid rafts in anti-microbial immune response. Front Immunol. 2021;12(654776). doi: 10.3389/fimmu.2021.654776
- Boyd AR, Hinojosa CA, Rodriguez PJ, et al. Impact of oral simvastatin therapy on acute lung injury in mice during pneumococcal pneumonia. BMC Microbiol. 2012;12(73). doi: 10.1186/1471-2180-12-73
- Skerry C, Pinn ML, Bruiners N, et al. Simvastatin increases the in vivo activity of the first-line tuberculosis regimen. J Antimicrob Chemother. 2014;69(9):2453–2457. doi: 10.1093/jac/dku166
- Liao WC, Huang MZ, Wang ML, et al. Statin decreases Helicobacter pylori burden in macrophages by promoting autophagy. Front Cell Infect Microbiol. 2017;6(203). doi: 10.3389/fcimb.2016.00203
- Motzkus-Feagans CA, Pakyz A, Polk R, et al. Statin use and the risk of Clostridium difficile in academic medical centres. Gut. 2012;61(11):1538–1542. doi: 10.1136/gutjnl-2011-301378
- Gaviola ML, Scribe EC, Leverett HN, et al. Statin effects on incidence, treatment success, and mortality of Clostridium difficile infections. J Pharm Pract. 2020;33(4):497–505. doi: 10.1177/0897190019854956
- Argamany JR, Lee GC, Duhon BD, et al. A possible association between statin use and improved clostridioides difficile infection mortality in veterans. PLoS One. 2019;14(5):e0217423. doi: 10.1371/journal.pone.0217423
- Ungaro R, Chang HL, Cote-Daigneault J, et al. Statins associated with decreased risk of New onset inflammatory bowel disease. Am J Gastroenterol. 2016;111(10):1416–1423. doi: 10.1038/ajg.2016.233
- Dickson I. Creeping fat in Crohn’s disease explained. Nat Rev Gastroenterol Hepatol. 2020;17(12):713. doi: 10.1038/s41575-020-00379-0
- Chen YC, Le PH, Wang YH, et al. Gut colonization and Antibiotic-associated diarrhea by Clostridium innocuum in children and adults. Clin Infect Dis. 2022;76(2):369–371. doi: 10.1093/cid/ciac696
- Hung YP, Lin HJ, Wu CJ, et al. Vancomycin-resistant Clostridium innocuum bacteremia following oral vancomycin for Clostridium difficile infection. Anaerobe. 2014;30:24–26. doi: 10.1016/j.anaerobe.2014.07.009
- Huang SW, Lin SE, Lee CS, et al. Cytomegalovirus colitis combined with Clostridium innocuum as a cause of lower GI bleeding in a patient with COVID-19. Gastrointest Endosc. 2022;95(2):388–390. doi: 10.1016/j.gie.2021.10.004
- Liu Q, Mak JWY, Su Q, et al. Gut microbiota dynamics in a prospective cohort of patients with post-acute COVID-19 syndrome. Gut. 2022;71(3):544–552. doi: 10.1136/gutjnl-2021-325989
- Skinner AM, Petrella L, Spandoni S, et al. Can Clostridium innocuum masquerade as clostridioides difficile? Clin Infect Dis. 2022;75(7):1268–1269. doi: 10.1093/cid/ciac377