ABSTRACT
Model organisms are crucial in research as they can provide key insights applicable to other species. This study proposes the use of the amphibian species Hymenochirus boettgeri, widely available through the aquarium trade, as a model organism for the study of chytridiomycosis, a disease caused by the fungus Batrachochytrium dendrobatidis (Bd) and linked to amphibian decline and extinction globally. Currently, no model organisms are used in the study of chytridiomycosis, particularly because of the lack of availability and nonstandardized methods. Thus, laboratories around the world use wild local species to conduct Bd infection experiments, which prevents comparisons between studies and reduces reproducibility. Here, we performed a series of Bd infection assays that showed that H. boettgeri has a dose- and genotype-dependent response, can generalize previous findings on virulence estimates in other species, and can generate reproducible results in replicated experimental conditions. We also provided valuable information regarding H. boettgeri husbandry, including care, housing, reproduction, and heat treatment to eliminate previous Bd infections. Together, our results indicate that H. boettgeri is a powerful and low-ecological-impact system for studying Bd pathogenicity and virulence.
Introduction
Model organisms and systems are essential components of research that facilitate the study of diverse biological processes in experimental contexts, providing results and theoretical insights that can be applied to other species [Citation1]. Suitable models provide several experimental benefits, including ease of breeding and maintenance in substantial quantities within laboratory environments, inexpensive, short generation times, small size, comprehensive genome sequencing, and ease of genetic manipulation [Citation1]. In particular, infectious disease research critically requires the use of model systems to understand the many facets of host–pathogen interactions, such as pathology, virulence (i.e. ability to cause harm to the host), host immune response, and pathogen evolution [Citation1,Citation2]. Models are particularly relevant for studying human or endangered species pathogens, as experimentation with them is impractical or unethical [Citation1]. Additionally, model organism research provides a key opportunity for the study of widespread generalist pathogens by providing a foundation for methodological comparisons among different research groups.
Chytridiomycosis, an emergent infectious disease caused by the fungus Batrachochytrium dendrobatidis (Bd), is linked to the population decline and extinction of hundreds of amphibian species on a global scale [Citation3,Citation4]. After more than two decades of intensive research on the Bd-amphibian system, no model organism has been widely used to study Bd pathogenicity. Finding a suitable model species for studying chytridiomycosis is challenging due to variability in susceptibility among species, ethical and legal concerns, animal availability, genetic diversity of hosts and of microbes within hosts, and the need for relevance to threatened wildlife species [Citation5]. Xenopus laevis has been used as a model amphibian species for many decades and is readily accessible, however, its resistance to Bd prevents it from being an ideal model for chytridiomycosis [Citation6]. Furthermore, attempts to use invertebrate hosts as proxy hosts have yielded inconsistent results [Citation7], and zebrafish larvae require pre-treatment with high doses of antibiotics to acquire Bd infection [Citation8]. To date, many experimental studies have relied on the use of numerous amphibian host species [Citation9,Citation10], most of which are obtained from the wild and have different life histories. This creates a great challenge for the replication of results and leads to a lack of consistency among laboratories, preventing data from being validated and compared among different research groups.
Herein, we propose the use of Hymenochirus boettgeri [Citation11] as a model host to conduct infection experiments with Bd. Originally inhabiting pools, rivers, and stagnant waters in forested regions of subequatorial Africa, H. boettgeri can be readily purchased from the aquarium trade, allowing laboratories worldwide to use it. In addition, H. boettgeri is a promising species for use as a model organism in the Bd-amphibian system as it: i) is susceptible to Bd [Citation12–14]; ii) tolerates high temperatures that eliminate Bd infection ([Citation12,Citation15]; also supported by our data); iii) reproduces throughout the year and completes metamorphosis in approximately 5 weeks [Citation16,Citation17]; iv) is small and fully aquatic, which simplifies husbandry and environmental manipulation; v) has a complete genome sequence [Citation18] and belongs to the same family as X. laevis [Citation19], which means that tools that work on X. laevis may be applied here; and vi) has a characterized antimicrobial peptide repertoire [Citation20], and general inferences about the functioning of its immune system can be made based on the extensively characterized immune system of the closely related species, X. laevis [Citation21].
In this study, we define some of the husbandry protocols and the advantages of working with H. boettgeri. We evaluated model species applicability in Bd virulence studies through three experiments in which we: i) performed a dosage response challenge using two Bd genotypes in an attempt to capture both dose- and genotype-dependent host survival; ii) tested the generalizability of virulence estimates in H. boettgeri to other amphibian species by comparing the virulence (measured as host mortality) of hybrid Bd genotypes to the parental lineages Bd-Brazil and Bd-GPL previously compared in native Brazilian hosts [Citation22] (hereafter model generalizability experiment); and iii) examined model reliability by partially repeating the model generalizability experiment and comparing results in terms of host mortality and infection burden (hereafter validation experiment). Our results suggest that H. boettgeri showed a variable response to Bd genotypes and doses, reproduced previous findings in other hosts, and generated reliable results in experimental replicates. Therefore, we advocate H. boettgeri as a promising model organism to study Bd pathogenicity, because the system shows biological relevance and can produce consistent results with low cost and ecological impact.
Methods
All procedures described here were conducted at the University of Michigan Animal Care Facilities following protocols approved by the University’s Institutional Animal Care and Use Committee (IACUC protocol PRO00009614). Upon arrival at our facility, we visually inspected all animals and removed dead animals or euthanized them (5% of the animals acquired for this study) if they showed evidence of disease (e.g. lethargy, red spots, bloating, abnormal floating or swimming patterns, and lack of righting response) using a solution of tricaine methanesulfonate (hereafter MS-222; 0.5 g/L; Syndel, Ferndale, WA, USA).
Species identification
Both H. boettgeri and Hymenochirus curtipes [Citation23] species are commonly called “African dwarf frogs” (ADF) and can be purchased from aquarium suppliers. However, H. curtipes is rarely sold, probably because of the difficulty in captive breeding [Citation17]. To avoid species misidentification, aquarium suppliers usually sell these frogs as Hymenochirus sp., although H. boettgeri are readily distinguished by their greater leg-length, broad heads, less oval body shape, and indented webbing of digits [Citation23,Citation24]. Because of this uncertainty, we began by determining the ADF species sold by the main suppliers in the United States. We purchased 160 Hymenochirus sp. frogs from VWR International (Radnor, PA, USA), Live Aquaria (Rhinelander, WI, USA), and Carolina Biological Supply Company (Burlington, NC, USA), visually identified all of them as H. boettgeri based on Noble [Citation23] and Channing & Rödel [Citation24], and then subjected them to mitochondrial DNA sequencing using two animals from each supplier to confirm our identification (n = 6). We also sequenced two samples from animals sold as H. curtipes by the same supplier (Live Aquaria) from a previous experiment in our laboratory [Citation12].
To obtain DNA for species identification, we rinsed six healthy frogs (two from each supplier) with sterilized distilled water for 5 s and collected skin swabs following a standard protocol using disposable gloves [Citation25]. Swabs were extracted using the DNeasy Blood and Tissue Kit (Qiagen, Valencia, CA, USA) following the manufacturer’s protocol with a minor modification that consisted of an extended incubation time (3 h) in the lysis step to increase DNA yield.
We used skin swab DNA extractions (n = 8) as input for PCR to amplify the 16S rRNA gene of the mitochondrial DNA (mtDNA) in 12.5 μl volumes composed of 5 μl of the sample template, 6.25 μl of GoTaq® Green master mix 2X (Promega, Madison, WI, USA), and 0.625 μl (10 μM) for each 16Sc-L (5’-GTRGGCCTAAAAGCAGCCAC-3’) and 16Sd-H (5’-CTCCGGTCTGAACTCAGATGACGTAG-3’) gene primer [Citation26]. Thermocycling conditions consisted of 94°C for 2 min, then 94°C for 1 min, 55°C for 1 min, and 72°C for 1 min for 35 cycles followed by 72°C for 7 min. PCR products were confirmed by gel electrophoresis, purified using an enzymatic reaction ExoSAP-ITTM (Thermo Fisher Scientific, Waltham, MA, USA) following the manufacturer’s protocol and subjected to Sanger Sequencing. Chromatograms were edited using Geneious Prime, and consensus sequences were generated. Two low-quality sequences were excluded: one from the supplier Carolina Biological and the other from supplier VWR International. A BLAST search was used to retrieve related sequences from the NCBI database. Alignment was performed using the muscle algorithm [Citation27] with default parameters in seaview v5.0.5 [Citation28]. A maximum likelihood phylogeny was constructed in the seaview using PhyML 3.1 [Citation29] with a GTR+I+G model and 100 bootstrap pseudo-replicates.
Quarantine and heat treatment
As our initial visual inspection for disease may not detect animals carrying low Bd loads, we also swabbed (as described above) a subsample of our population (n = 50) and used skin swab DNA extractions to assess Bd presence and intensity using a standard qPCR assay [Citation30] (Boyle et al. 2004) on QuantStudio 3 (Applied Biosystems, Thermo Fisher Scientific). Standard curves were produced using seven serial dilutions (106-100 zoospore equivalents, ZE) of JEL 423, a Bd-GPL culture isolated from Agalychnis lemur in Panama. Samples were run in duplicate to ensure accurate quantification, and only those samples that amplified ≥1 ZE in both replicates were considered positive for Bd. Samples with inconsistent results were repeated and considered positive when two out of three replicates were positive. The mean load in the ZE was recorded for each positive value. Negative controls were included in qPCR assays to account for contamination.
Because some animals were positive for Bd (see results for details), all 152 animals were quarantined in 38 L glass tanks in the standard system (see details below) and subjected to heat treatment to clear Bd infection. All individuals that tested positive for Bd were kept in the same aquarium during and after the heat treatment and isolated from the others, and none of them were used in the experiments of this study. We gradually increased water temperature from 20°C to 30°C over a period of 10 d, followed by 10 d at 30°C, and then a gradually decreased to 25°C over a period of 5 d. At the end of the heat treatment, we retested the animals that were positive for Bd before heat treatment. After this period of quarantine and heat treatment, we allocated the frogs to permanent aquaria, where they remained until the beginning of the experiment.
Additionally, we conducted a heat treatment on 11 H. boettgeri adults that were exposed to Bd and survived until the end of a parallel experimental study in our laboratory. All 11 animals were swabbed and tested for Bd before and after the heat treatment. All skin swabs, DNA extraction, and Bd quantification performed in this study followed the same procedures as described in this section.
Housing and care
Our facilities were equipped with three different aquatic systems, defined by the mechanisms used to remove waste products. We used a semi-open recirculation system (ZebTec, Tecniplast®, West Chester, PA, USA) (hereafter Tecniplast system), in which clean water flowed into the tanks and a portion of the effluent was discarded, while the rest was pumped to an external treatment zone where the residues were removed before the clean water returned to the tanks (). We also adopted a closed recirculation system (hereafter referred to as the standard system), in which water was treated using an internal filter and continuously circulated throughout the aquarium; therefore, no water was discarded (except in scheduled water changes; ). Finally, we used a static closed system (hereafter static system), in which clean water was added and waste was removed through frequent water changes (). The care required to keep the different systems safe for animals varies (see details in the text below), but all three systems have proven to be efficient and safe for H. boettgeri husbandry. Here, their usage depended on the experimental phase since all were available, but the non-availability of a more expensive system (such as Tecniplast system) will not influence the experimental use of the species. All procedures described here were performed in a temperature-controlled room set at 20°C ±1°C (water temperature when no heater was used) and 13:11 light–dark cycle.
Figure 1. Photographs of housing systems and eggs, tadpoles and adults of H. boettgeri. Tecniplast (1a), standard (1b), and static (1c) systems, female (black arrow; 1d, e) and male (filled white arrow pointing to post-axillary subdermal gland and unfilled white arrow pointing to obiculated tympanum; 1d, e) adult individuals, nursery tank of the Tecniplast system (1f), eggs inside the nursery tank (1g), 4-d-old tadpoles feeding on powdered food (1h), and 12-d-old tadpoles feeding on brine shrimp (1i). Black bars represent 1 cm scale.
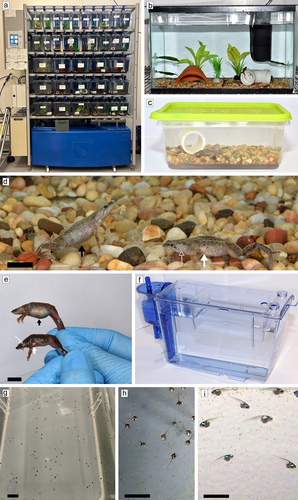
Prior to the experiments, we housed mixed-sex animal groups in 38 L glass tanks (25 × 30 × 50 cm) in the standard system or in 8 L plastic tanks (17 × 23 × 28 cm) in the Tecniplast system at a density of at least 2 L or 0.8 L of water per individual in the standard system or Tecniplast system, respectively. We enriched all tanks with 1 cm of gravel substrate (with a diameter larger than 1 cm to prevent frogs from ingesting them), artificial plants, PVC pipes, and broken terracotta pots for retreat. We observed that the addition of enrichment improved the animals’ quality of life. All enrichment items were sterilized using 1% Virkon for 20 min and then rinsed with reverse osmosis (RO) water and checked to be free from rough surfaces or sharp edges to minimize possible skin damage. Standard system tanks were equipped with an internal Aquarium Heater (Eheim®, Germany thermocontrol 3,613,090 Aquarium Heater 75 W) and internal aquarium filter (Tetra, Spectrum Inc., Blacksburg, VA, USA). All housing was covered to prevent frogs from escaping, with plastic lids containing small holes for air circulation.
Since H. boettgeri adults must come to the water surface to breathe, we filled the aquaria with RO water and kept a minimum space of 1 cm between the lid and the water surface. As RO water is extremely hypoosmotic, we adjusted the water conductivity to 900 ± 100 µS/cm by adding 0.5 g/L of formulated synthetic sea salt mixture (Instant Ocean® Sea Salt, Blacksburg, VA, USA) and maintained pH levels at 7 ± 0.4 by adding sodium bicarbonate when necessary. For the Tecniplast and standard systems, the water temperature was adjusted and kept constant at 25°C ± 0.5°C. Frogs were monitored once a day and fed newly hatched brine shrimp (Artemia sp.; BIO-MARINE®, Hawthorne, CA, USA) and frozen gamma-irradiated bloodworms (Chironomus sp.; Tropical Marine Center, UK) ad-libitum three times per week.
Continuous biological filtration and water changes removed nitrogenous residues resulting from excretory products, predominantly ammonia, in the Pipidae family [Citation31], as well as those generated from uneaten feed. We initiated the biological cycle by adding a single dose of 1 ml/L of nitrifying bacterial suspension (ProLine® Aquaculture Bacteria Concentrate, Apopka, FL, USA), which settled on the filter or on other surfaces to oxidize toxic ammonia/ammonium (NH3/NH4+) to nitrite (NO2−), and later into nitrate (NO3−) [Citation32], both less toxic products. The Tecniplast system was programmed to change 15% of the total water volume in the entire system daily, while water recirculation within the tanks occurred at 0.20 L/min. In the standard system, we performed water changes of approximately 70% of the total volume weekly in cycled aquariums (i.e. established nitrifying bacteria were in sufficient abundance to maintain ammonia and nitrite values near 0 and increase nitrate values), and as needed to maintain nitrogenous compound levels within the normal safe range (see below) in uncycled aquariums – in our system water changes were performed approximately twice a week for approximately 6 weeks in tanks at full capacity. To avoid frog stress, we adjusted RO water conductivity and temperature (i.e. 900 ± 100 µS/cm and 25°C, respectively) before the water changes in tanks of the standard system. When water changes coincided with feeding days, they were performed at least 4 h after feeding.
We monitored the Tecniplast system water weekly for pH (7 ± 0.4), temperature (25°C ±0.5°C), and conductivity (900 ± 100 µS/cm), all available in real time on the system’s digital display, and tested using a water testing kit (API Liquid Test Kits, API®, Chalfont, PA, USA) for ammonia (≤0.25 ppm), nitrite (0 ppm), and nitrate (≤40 ppm). Note that the Tecniplast system automatically measures pH, conductivity, and temperature. In standard system cycled tanks, we tested the water in all aquaria weekly using an EcoTestr CTS1 meter (Oakton, Vernon Hills, IL, USA) for temperature (25°C ±0.5°C) and conductivity (900 ± 100 µS/cm), and using a water testing kit for pH (7 ± 0.4), ammonia (≤0.25 ppm), nitrite (0 ppm), and nitrate (≤40 ppm). Standard uncycled tanks were tested three times a week for pH (7 ± 0.4), ammonia (≤1 ppm), nitrite (≤1 ppm), and nitrate (≤50 ppm). We performed water changes in uncycled tanks whenever we detected 1 ppm ammonia, 1 ppm nitrite, or 50 ppm nitrate – values that we considered the maximum limits for animal safety. These conditions seem to be safe for H. boettgeri because no animals died or presented behavioural changes compared to animals in cycled tank conditions. All system configurations and techniques described here were maintained using these procedures except when noted otherwise.
During experiments that required individual housing (i.e. Bd dosage response, model generalizability, and validation experiments), we housed the frogs individually in 1 L plastic tanks (16 cm × 6.5 cm × 11 cm; ) in the static system enriched with 1 cm layer of gravel substrate and a PVC tube. Water was maintained at room temperature. We adjusted RO water and fed frogs as described above and performed water changes three times per week of approximately 70% of the total water volume (4 h after feeding) in order to maintain safe nitrogenous waste levels for the animals. We tested water quality in at least 10% of total tanks with live animals (randomly selected at each measurement) three times per week (before water change) using an EcoTestr CTS1 meter for temperature and conductivity and using a water testing kit for pH, ammonia, nitrite, and nitrate (see Table S1 for mean and standard deviation of parameter values obtained in each measurement throughout the experiments). These procedures allowed us to maintain safe water nitrogen levels, and additional water changes were unnecessary during the experiments. Before starting any experiment that used individual housing at room temperature, we gradually decreased the water temperature from 25°C to 20°C over a period of 5 d and provided at least 15 d as an adaptation period.
Breeding trial
H. boettgeri is a sexually dimorphic species, in which females usually present a larger oval-shaped body (up to 35 mm in snout-vent length (SVL)), whereas males present a large obiculated tympanum and a lateral post-axillary subdermal gland that increase in size and vascularity during sexual activity [Citation16] (, e). We selected males from supplier VWR with heavily vascularized glands and the largest females from supplier Live Aquaria and transferred them to two 38 L glass tanks in the standard system separated by sex. Based on our previous observations, we believe that breeding behaviour is stimulated in H. boettgeri when feeding frequency and quality are increased. Therefore, we fed them ad libitum for 10 consecutive days with newly hatched brine shrimp and either frozen gamma-irradiated bloodworms, live blackworms (Lumbriculus variegatus), or small-granule fish food (BUG BITESTM Tropical Formula, Mansfield, MA, USA), varying the second option daily. After 10 d, we selected two breeding pairs and placed each pair in a separate 8 L static system tank for mating without enrichment, filter, or heater to facilitate egg visualization and collection. The pairs remained together for 1 or 3 d, during which we observed one or three matings with subsequent egg release. The exact ages of adult pairs are unknown, but we estimated that they were at least 1-y old, given that we received them as adults 10 months before the breeding events. During the breeding period, we conducted 70% water change daily, 4 h after feeding.
To test the best environment for tadpole development, we performed two different housings with spawns from each pair. We collected approximately 600 eggs from one of the pairs over 3 d from the water surface using a sterile 15 ml tablespoon and a sterile siphon for eggs that had sunk in the tank. We transferred them to a 1 L nursery tank (6 × 9 × 15 cm) lined with a 300 µm net at the bottom and attached the nursery tank to a 3.5 L plastic tank in the Tecniplast system (). The nursery tanks kept the eggs and tadpoles close to the surface, facilitating access to food and oxygen (). We maintained approximately 75 eggs (collected on the same day or ± 1 d) in each nursery tank, except for two nursery tanks that contained 35 eggs each. With the other breeding pairs, we removed the adults after egg release to prevent the animals from feeding on the eggs, and returned the 8 L tank (without a nursery tank) containing approximately 200 eggs to the Tecniplast system.
We started feeding on the tadpoles when they started swimming (1–2 d after hatching). We fed them ad libitum daily with powdered fry food (Sera® Micron Nature, Heinsberg, Germany) for a week (), followed by newly hatched brine shrimp for at least 4 weeks after completing metamorphosis (), after which they were fed the same diet as the adults. We monitored animals twice daily and removed any uneaten food, unhatched eggs, or dead tadpoles. All newly metamorphosed animals were divided into 8 L plastic tanks in the Tecniplast system.
Bd virulence experiments
Bd culturing and inoculation procedures
Prior to the experiment, all isolates were cryopreserved at −80 ºC in the Collection of Zoosporic Eufungi at the University of Michigan (CZEUM) and revived for this experiment. To prepare Bd suspensions for experimental infection, we transferred liquid cultures of each genotype to individual agar plates containing 1% tryptone and allowed them to grow for 6 d at 20°C. We then gently collected zoospores of each Bd genotype by flooding culture plates with 2 ml of sterile distilled water for 40 min to stimulate zoospore release. The same procedure was performed using Bd-free 1% tryptone agar plates for the control group. From each zoospore suspension, we prepared standard inoculation solutions by collecting a 20 μl subsample and quantifying the zoospore density using a Coulter Counter (Multisizer™ 4e, Beckman Coulter Inc., USA) based on the cell diameter (2.5–5.0 μm).
For pathogen exposure, individual hosts were placed in 50 ml Falcon tubes with 10 ml of zoospore suspension containing a fixed inoculum dose (depending on the experiment, Table S2) and were exposed for 5 h. Frogs from the control group were exposed to 10 ml of Bd-free suspension. After inoculation, we housed animals individually in the static system, with the addition of the original inoculum. We monitored frogs for signs of morbidity, and individuals that showed a lack of righting response, a sign of advanced stages of chytridiomycosis [Citation33], were euthanized using MS-222.
Bd dosage response
We used H. boettgeri frogs from the supplier Live Aquaria and challenged them with two Bd doses (high = 5 × 105 zoospores/ml and low = 5 × 104 zoospores/ml) of two Bd-GPL genotypes (JEL423 and CFLT073, Table S3). We selected adults of approximately the same size (mean SVL = 2.46 ± sd 0.17 cm, n = 35) and weight (mean = 0.69 ± sd 0.14 g, n = 35) and randomly assigned them to one of four single-genotype treatments or a control group (Table S2). Because one frog from the control group died during the experiment, we swabbed all control frogs at the end of the experiment to quantify Bd infection.
Model generalizability and validation experiments
Experimental treatments included six different Bd genotypes: two Bd-Brazil, two Bd-GPL, and two hybrid genotypes, which comprised six of the nine live Bd genotypes used in Greenspan et al. [Citation22] and a control group (Tables S2 and S3). The zoospore suspensions from all six treatments/isolates used for pathogen exposure contained a fixed inoculum dose of 2.5 × 105 zoospores/ml. We swabbed animals from two treatments (CLFT039 and CLFT150) and the control group after death or at the end of the experiment to quantify Bd infection and compared these results with those of the validation experiment.
Three weeks after the end of the model generalizability experiment, we conducted a second challenge (validation experiment) using a subset (hybrid CLFT039 and Bd-Brazil CLFT150) of the previous genotypes with the same inoculum dose and a control group (table S2). For these experiments, we used adults of approximately the same size (SVL mean = 2.41 ± sd 0.18 cm, n = 100) and weight (mean = 0.85 ± sd 0.19 g, n = 100) from the supplier VWR.
Statistical analyses
Using survival probability as a proxy for pathogen virulence, we built survival curves to i) investigate the host response to different Bd doses and genotypes using treatments (dose/genotype) as fixed effects; ii) test for the effects of both Bd-Brazil, Bd-GPL, and hybrids (lineage or hybrid as fixed effects), and six Bd genotypes (genotypes as fixed effect), independently, on host survival in the model generalizability experiment; and iii) evaluate whether our patterns are repeatable by comparing the survival outcomes of the CLFT039 and CLFT150 genotypes from the model generalizability and validation experiments, using treatments (genotype/experiment) as fixed effects. Survival curves were computed using the Kaplan–Meier method, which was implemented using the survfit function from the package survival [Citation34], and survival curves were compared by conducting log-rank tests using the function survdiff (also from the package survival). We conducted post-hoc comparisons of survival curves using the function pairwise_survdiff from the package survminer [Citation35], which computes adjusted p-values to correct for false discovery rate (we used the method “BH” [Citation36]). To test whether Bd load (log-transformed) patterns at the time of death was repeatable among individuals exposed to CLFT039 and CLFT150 genotypes from the model generalizability and validation experiments, we performed an analysis of variance (ANOVA) using the aov function of the stats package [Citation37], followed by Tukey’s post-hoc test using the glht function of the multcomp package [Citation38]. We included treatments (genotype/experiment) as fixed effects in the ANOVA. All analyses were performed using R version 4.0.2 [Citation37].
Results
Species identification
All eight frog sequence data showed high similarity with the species H. boettgeri from NCBI (99.3–100%) indicating that the ADFs sold by suppliers as Hymenochirus sp. and those used in Jenkinson et al. [Citation12] were H. boettgeri. This was also supported by a phylogenetic analysis, which showed multiple sequence types within H. boettgeri but no supported subgroups, as estimated by bootstrapping (Figure S1).
Heat treatment
Our qPCR results showed that 18% of tested animals from suppliers and all 11 animals exposed to Bd in the laboratory were Bd positive prior to heat treatment (Suppliers: mean load = 1045.27 ± sd 1617.03 ZE, n = 9; experimentally infected: mean load = 1716.45 ± sd 3216.61 ZE, n = 11; Table S4). All Bd-positive animals from either suppliers or experimentally infected animals, including an animal with a load greater than 10,000 ZE, tested negative for Bd after heat treatment (Table S4).
Breeding trial
Eggs of both pairs hatched within 2 d. We observed a natural variation in size among similarly fed tadpoles in both housing types (i.e. with or without a nursery tank), although this variation was more evident in nursery tanks with higher densities. In addition, tadpoles from nursery tanks with lower densities grew and metamorphosed slightly more rapidly than tadpoles from nursery tanks with higher densities. Tadpoles in 8 L tanks without nursery tanks were able to swim the entire water column (15 cm) and forage for food both at the bottom of the tank and on the water surface, and no differences were observed in tadpole growth when compared to tadpoles from nursery tanks.
The tadpole survival rate did not vary between housings. From both pairs, approximately 30% of the eggs were not fertilized or did not hatch, and of those that did hatch, 65% of the tadpoles survived to adulthood (which means approximately 45% of the 800 eggs collected). Tadpole death was recorded from days 5 to 21 after hatching, with most occurring in the second week of life.
The time required for metamorphosis was short, as noted here and previously detailed by Sokol [Citation17]. Overall, tadpoles from both housings completed metamorphosis an average of 5 weeks after egg collection, and a minority of them (approximately 10%) completed it after 7 weeks.
Bd virulence experiments
The Bd dosage response experiment showed that host survival was both dose- and genotype-dependent (log-rank test: X2 = 56.1, df = 4, p < 0.0001, ). Animals exposed to higher Bd doses succumbed faster than those exposed to low Bd doses, and at equivalent doses, the JEL423 genotype was more virulent than CLFT073, which at low dose did not differ from the control group (, Table S5).
Figure 2. Survival probability curves of JEL423 and CLFT073 Bd genotypes at high (HD) or low (LD) dose. p-value is from log-rank test.

In the model generalizability experiment, H. boettgeri survival varied at the lineage level (hybrids, Bd-GPL or Bd-Brazil; Log-rank test: X2 = 31.6, df = 2, p < 0.0001). Hybrids were more virulent than Bd-GPL (p = 0.0001) and Bd-Brazil (p < 0.0001) lineages, while Bd-GPL and Bd-Brazil did not differ in host survival probability (p = 0.32) (). Overall, at the genotype level, significant differences in host survival among genotypes (log-rank test: X2 = 41.1, df = 5, p < 0.0001) also indicated higher virulence of hybrid genotypes and similar virulence among Bd-GPL and Bd-Brazil genotypes (, Table S6).
Figure 3. Survival curves and Bd load boxplot from the model generalizability and validation experiments. Survival curves for H. boettgeri exposed to six Bd genotypes from the model generalizability experiment, built at (a) lineage or (b) genotype level. (c) survival curves and (d) Bd load (log transformed at time of death) boxplots for H. boettgeri exposed to CLFT039 and CLFT150 genotypes from model generalizability (solid line/MG boxes) and validation experiments (dotted line/V boxes). Yellow, blue and green lines/boxes indicate that the Bd genotype belongs to hybrids, Bd-GPL and Bd-Brazil lineages, respectively. p-values are from log-rank test. Letters 'a' and 'b' inside the boxplots represent statistically significant differences from post-hoc multiple comparisons of means test among Bd genotype/experiments.

The validation experiment repeated the patterns of the model generalizability experiment for both survival probability (log-rank test: X2 = 45.7, df = 3, p < 0.0001) and Bd load average at the time of death (F(3,36) = 7.8, p < 0.001, Table S7). The hybrid (CLFT039) genotype was more virulent than the Bd-Brazil (CLFT150) genotype in both experiments (p < 0.0001 for all pairwise comparisons; ). Frog survival probability from the validation experiment when exposed to CLFT039 and CLFT150 genotypes did not differ in relation to the model generalizability experiment (p = 0.18 and p = 0.36, respectively; ). The average Bd load at the time of death for animals exposed to CLFT039 genotype was lower than those exposed to CLFT150 genotype in both experiments (, Table S7). In addition, Bd load averages of CLFT039 and CLFT150 genotypes from the validation experiment did not differ in relation to the model generalizability experiment (p = 0.99 for both; ).
Across all experiments, frogs from the control groups did not show infection symptoms and tested negative for Bd at the time of death (one frog from the Bd dosage response experiment) or at the end of the experiment, suggesting that mortality was directly associated with experimental pathogen infections.
Discussion
Extraordinary efforts have been made to explore the pathogenicity of amphibian-killing chytrid fungus, but the lack of a controlled and standardized system for studying Bd-amphibian disease dynamics is a major problem for synthesizing existing knowledge. This issue affects the reliability and reproducibility of results and hinders the comparison of research findings across different laboratories, posing a significant challenge to advancing the field. Here, we provide protocols related to the care, breeding, Bd inoculation and heat treatment of H. boettgeri, which could be adopted by other laboratories. We also developed a standardized Bd infection protocol and performed a series of experiments to test H. boettgeri efficiency as a model organism for exploring Bd infection. The results of the Bd dosage response experiment corroborated a previous study showing that H. boettgeri has a Bd dose- and genotype-dependent response [Citation12], which is a critical trait when choosing a relevant model organism to study Bd virulence. In addition, we showed that we could recover similar Bd genotype virulence patterns from other amphibian species, including species with both direct and indirect development, demonstrating that newly emerged hybrid genotypes have the potential to increase disease risk [Citation22]. In addition, both host mortality and Bd infection burden from the validation experiment were similar to those from the model generalizability experiment, indicating that the system could be replicated. Taken together, our results show that H. boettgeri is a powerful model organism within which Bd–host interactions and pathogenicity can be fully investigated.
We acknowledge that species identity and life history can profoundly alter disease outcomes [Citation3,Citation5,Citation10] and that the use of H. boettgeri in experimental studies must consider whether these host-specific traits would influence the results. For example, in Greenspan et al. [Citation22], Bd-Brazil, a spatially restricted lineage that occurs in an enzootic infection stage with local hosts [Citation39,Citation40], showed lower virulence than the panzootic and hypervirulent Bd-GPL and hybrid genotypes in Brazilian amphibian hosts. In contrast, our results showed that Bd-Brazil can be as virulent as Bd-GPL for H. boettgeri with which it does not share an evolutionary history. However, under infection with newly emerged hybrid genotypes, which do not have a coevolutionary history with any host species, both H. boettgeri and Brazilian hosts showed that hybrids can be more virulent than their parents. In the meantime, our results support those of previous studies, indicating that ancestral or enzootic lineages are not attenuated in virulence [Citation41,Citation42]. These findings underscore an important point that is often overlooked by current theory: the coevolutionary history of Bd and its hosts may play a stronger role in determining the outcome of infection than lineages per se. Furthermore, it is worth highlighting that our fully aquatic model species recovered similar patterns of virulence to Bd genotypes as the highly susceptible direct development species, Brachycephalus ephippium [Citation22]. This suggests that H. boettgeri can provide insights into general patterns of Bd virulence that can be extrapolated to a diversity of anurans.
Our animals grew and bred under both simple (standard tank) and sophisticated (recirculation system) conditions, providing hundreds of juveniles in a few weeks. Such an easy and effective breeding system not only reduces the need to remove animals from nature but also solves or simplifies a variety of challenges involved in experimentation with amphibians and Bd. These challenges include finding susceptible species, obtaining licences to collect wild animals, finding Bd-free animals, working with a reduced sample size, and very commonly in the Bd field, reporting results without repetitions to validate the findings. In addition, unless spawning is used, adult animals captured from the wild or even from suppliers can carry pathogens common to amphibians [Citation10], and different ecological histories dependent on previous infections can culminate in a more or less robust immune system [Citation43,Citation44]. Thus, the use of H. boettgeri not only facilitates research with a low ecological impact but also avoids possible confounding factors. Unfortunately, we could not control the effects of ecological histories in our experiments because we did not have adult animals bred in our facility available when the experiments were conducted. However, we reduced this effect by choosing related animals from the same supplier to conduct each experiment, and despite this concern, we found strong repeatability in the validation experiment.
H. boettgeri has already been used as a model organism in a co-infection study to explore how competition between Bd genotypes can shape pathogen evolution [Citation12], but its characteristics allow for further exploration of a diversity of other mechanisms that potentially affect disease outcome. Although we did not test H. boettgeri thermal tolerance, previous studies demonstrate that H. boettgeri can breed at temperatures that fluctuate daily between 19°C and 30°C [Citation16], and possibly, the species can be maintained in an even broader temperature range. This broad temperature range offers the opportunity to explore the underlying mechanisms by which temperature (including its variability, anomalies, and fluctuations) affects host–pathogen interactions, which would reveal the consequences of global climate change on chytridiomycosis outbreaks. Furthermore, the social behaviour of H. boettgeri allows these small animals to be group-housed, and experiments that explore the effects of host density or diversity on chytridiomycosis dynamics can be easily conducted. Heat treatment also enables the analysis of how a previous infection can affect the outcome of a future infection, either by the same or a different genotype. More importantly, heat treatment reduces the number of sacrificed animals through the reuse of heat-treated animals after infection experiments. Finally, the fully aquatic habitat of H. boettgeri facilitates i) Bd transmission experiments between H. boettgeri individuals or between wild amphibian hosts and H. boettgeri, which can be used to assess the competitiveness of Bd genotypes or isolate Bd from wild amphibian species, and ii) experiments that account for the effects of aquatic Bd zoospore predators (e.g. cladocerans, ciliates, and rotifers [Citation45–48]), alternative non-amphibian Bd hosts (e.g. zebrafish [Citation8] and crayfish [Citation49]), or manipulation of the amphibian skin microbiome (by adding antibiotics or probiotics to the water) on disease outcome.
This study was performed using adult frogs; however, future studies should assess the applicability of different life stages of H. boettgeri in chytridiomycosis research. For example, it is known that recently metamorphosed frogs can be severely affected by Bd due to significant changes in their immune system [Citation50], while Bd infection in tadpoles can trigger silent population declines by reducing foraging, slowing growth, and impacting tadpole metamorphosis [Citation51,Citation52]. While H. boettgeri tadpoles lack keratinized mouthparts [Citation17], which is the primary site of Bd infection in tadpoles, the possibility of Bd infecting other keratinized regions, such as the feet and tail, in the late development stages, still requires investigation [Citation53]. Furthermore, future studies addressing preference and thermal limits of all life stages of H. boettgeri are pivotal for advancing the use of this model organism, particularly in the context of thermal treatment for chytridiomycosis clearance and experiments with varying temperatures. Extreme temperatures in recently metamorphosed and adult amphibians can result in profound alterations in physiological processes, the immune system, and the microbiome, potentially affecting life history traits and survival [Citation54–57]. Additionally, it is known that heat waves can have side effects such as sex reversal, delayed metamorphosis, and reduced body mass in tadpoles [Citation58]. Lastly, the host microbiome plays a crucial role in susceptibility to Bd [Citation59]. Therefore, it is imperative to profile the H. boettgeri microbiome to gain a better understanding of its impact on disease outcomes in our model organism. However, it is worth acknowledging that raising animals in captivity or transferring them from their natural habitat to the laboratory can significantly influence microbiome composition [Citation60]. As a result, we recognize that while our model is valuable for certain studies, certain questions related to the microbiome may be better addressed by investigating animals in their natural environments.
In this manuscript, we propose the use of H. boettgeri as a model organism for studying interactions between Bd and its amphibian host and highlight its applicability to explore Bd virulence. In addition to the points discussed earlier, we suggest future advances in the use of this model organism to generate gnotobiotic frogs using the already established protocol of embryo surface sterilization for X. laevis and Xenopus tropicalis [Citation61]. Furthermore, testing the susceptibility of H. boettgeri to other emerging amphibian pathogens, such as ranaviruses and Batrachochytrium salamandrivorans, would allow us to explore not only the threats from single infections but also from genetically diverse infections to amphibian diversity. We acknowledge the complexity of the amphibian-Bd system and recognize that our model does not substitute for the necessity of conducting experiments in other species to gain specific insights influenced by host identity. Nevertheless, we hope that our model system will serve as a valuable tool in advancing our understanding of disease, in particular the pathogen side, such as understanding the relationship between genotype and virulence. Finally, the alignment between our results and Greenspan et al. [Citation22], demonstrating high virulence in Bd hybrid genotypes, reinforces the threat of genetic recombination between Bd lineages – facilitated by global trade [Citation62,Citation63] – to global amphibian diversity and highlights the urgent need for increased monitoring of Bd’s genetic distribution.
Author contribution
TC and TYJ conceptualized this study. TC, CS, RC, and EF conducted laboratory experiments. TC analysed the data and drafted the manuscript. All authors critically revised the manuscript.
Supplemental Material
Download MS Word (57.4 KB)Acknowledgements
We thank Diego Moura-Campos for helpful comments on an earlier version of this manuscript, Yi-Hong Ke for helping with the photographs, and Mia Sinks and Ana Felgner for helping with amphibian care. We are grateful to the members of U. Michigan Unit for Laboratory Animal Medicine for their advice and support in the development of our model, Dra Jill Keller, Kate Clifton, Allison Kulka, and Josh Kirk.
Disclosure statement
No potential conflict of interest was reported by the author(s).
Data availability statement
The data and full codes for all statistical tests are available at https://doi.org/10.5281/zenodo.8434900.
Supplemental data
Supplemental data for this article can be accessed online at https://doi.org/10.1080/21505594.2023.2270252.
Additional information
Funding
References
- Ankeny RA, Leonelli S. Model organisms. Cambridge: Cambridge University Press;2020.
- Müller B, Grossniklaus U. Model organisms — a historical perspective. J Proteomics. 2010;73(11):2054–13. doi: 10.1016/j.jprot.2010.08.002
- Fisher MC, Garner TWJ. Chytrid fungi and global amphibian declines. Nat Rev Microbiol. 2020;18(6):332–343. doi: 10.1038/s41579-020-0335-x
- Scheele BC, Pasmans F, Skerratt LF, et al. Amphibian fungal panzootic causes catastrophic and ongoing loss of biodiversity. Science. 2019;363(6434):1459–1463. doi: 10.1126/science.aav0379
- Fisher MC, Pasmans F, Martel A. Virulence and pathogenicity of chytrid fungi causing amphibian extinctions. Annu Rev Microbiol. 2021;75(1):673–693. doi: 10.1146/annurev-micro-052621-124212
- Ramsey JP, Reinert LK, Harper LK, et al. Immune defenses against Batrachochytrium dendrobatidis, a fungus linked to global amphibian declines, in the South African Clawed frog, Xenopus laevis. Infect Immun. 2010;78(9):3981–3992. doi: 10.1128/IAI.00402-10
- Betancourt-Román CM, O’neil CC, James TY. Rethinking the role of invertebrate hosts in the life cycle of the amphibian chytridiomycosis pathogen. Parasitology. 2016;143(13):1723–1729. doi: 10.1017/S0031182016001360
- Liew N, Mazon Moya MJ, Wierzbicki CJ, et al. Chytrid fungus infection in zebrafish demonstrates that the pathogen can parasitize non-amphibian vertebrate hosts. Nat Commun. 2017;8(1):15048. doi: 10.1038/ncomms15048
- Belasen AM, Russell ID, Zamudio KR, et al. Endemic lineages of Batrachochytrium dendrobatidis are associated with reduced chytridiomycosis-induced mortality in amphibians: evidence from a meta-analysis of experimental infection studies. Front Vet Sci. 2022;9. doi: 10.3389/fvets.2022.756686
- Blaustein AR, Urbina J, Snyder PW, et al. Effects of emerging infectious diseases on amphibians: a review of experimental studies. Diversity. 2018;10(3):81. doi: 10.3390/d10030081
- Tornier G. Reptilien, Amphibien. In: Möbius K, editor. Deutsch Ost-Afrika. Die Thierwelt Ost-Afrikas (Part 4). Vol. 3. Berlin: Dietrich Reimer; 1896. p. 1–164.
- Jenkinson TS, Rodriguez D, Clemons RA, et al. Globally invasive genotypes of the amphibian chytrid outcompete an enzootic lineage in coinfections. Proc R Soc B Biol Sci. 2018;285(1893):20181894. doi: 10.1098/rspb.2018.1894
- Murphy BG, Hillman C, Groff JM. Chytridiomycosis in dwarf African frogs Hymenochirus curtipes. Dis Aquat Org. 2015;114(1):69–75. doi: 10.3354/dao02851
- Raverty S, Reynolds T. Cutaneous chytridiomycosis in dwarf aquatic frogs (Hymenochirus boettgeri) originating from southeast Asia and in a western toad (bufo boreas) from northeastern British Columbia. Can Vet J. 2001;42(5):385–386.
- Chatfield MWH, Richards-Zawacki CL. Elevated temperature as a treatment for Batrachochytrium dendrobatidis infection in captive frogs. Dis Aquat Org. 2011;94(3):235–238. doi: 10.3354/dao02337
- Rabb GB, Rabb MS. On the behavior and breeding biology of the African pipid frog: hymenochirus boettgeri. Zeitschrift für Tierpsychologie. 1963;20(2):215–241. doi: 10.1111/j.1439-0310.1963.tb01151.x
- Sokol OM. The tadpole of Hymenochirus boettgeri. Copeia. 1962;1962(2):272–284. doi: 10.2307/1440890
- Mudd AB. Comparative genomics and chromosome evolution. eScholarship, University of California, Berkeley. 2019. https://escholarship.org/uc/item/1sp703wf
- Daudin F. “An. XI”. Histoire Naturelle des Rainettes, des Grenouilles et des Crapauds. Quarto version. Paris: Levrault Quarto version; 1802.
- Matthijs S, Ye L, Stijlemans B, et al. Low structural variation in the host-defense peptide repertoire of the dwarf clawed frog Hymenochirus boettgeri (pipidae). PLoS One. 2014;9(1):e86339. doi: 10.1371/journal.pone.0086339
- Ruiz VL, Robert J. The amphibian immune system. Philos Trans R Soc B. 2023;378(1882):20220123. doi: 10.1098/rstb.2022.0123
- Greenspan SE, Lambertini C, Carvalho T, et al. Hybrids of amphibian chytrid show high virulence in native hosts. Sci Rep. 2018;8(1):9600. doi: 10.1038/s41598-018-27828-w
- Noble GK. Contributions to the herpetology of the Belgian Congo based on the collection of the American Museum Congo Expedition, 1909-1915. Part 3, amphibia. Bull AMNH. 1924;49:147–347.
- Channing A, Rödel M-O. Field guide to the frogs & other amphibians of Africa. Cape Town, South Africa: Struik Nature; 2019. p. 40.
- Hyatt AD, Boyle DG, Olsen V, et al. Diagnostic assays and sampling protocols for the detection of Batrachochytrium dendrobatidis. Dis Aquat Org. 2007;73:175–192. doi: 10.3354/dao073175
- Evans BJ, Brown RM, McGuire JA, et al. Phylogenetics of fanged frogs: Testing biogeographical hypotheses at the Interface of the Asian and Australian Faunal Zones. Syst Biol. 2003;52(6):794–819. doi: 10.1080/10635150390251063
- Edgar RC. MUSCLE: multiple sequence alignment with high accuracy and high throughput. Nucleic Acids Res. 2004;32(5):1792–1797. doi: 10.1093/nar/gkh340
- Gouy M, Guindon S, Gascuel O. SeaView version 4: a multiplatform graphical user interface for sequence alignment and phylogenetic tree building. Mol Biol Evol. 2010;27(2):221–224. doi: 10.1093/molbev/msp259
- Guindon S, Dufayard J-F, Lefort V, et al. New algorithms and Methods to estimate Maximum-Likelihood Phylogenies: assessing the performance of PhyML 3.0. Syst Biol. 2010;59(3):307–321. doi: 10.1093/sysbio/syq010
- Boyle DG, Boyle DB, Olsen V, et al. Rapid quantitative detection of chytridiomycosis (Batrachochytrium dendrobatidis) in amphibian samples using real-time Taqman PCR assay. Dis Aquat Org. 2004 Aug 9;60(2):141–148. doi: 10.3354/dao060141
- Cragg MM, Balinsky JB, Baldwin E. A comparative study of nitrogen excretion in some amphibia and reptiles. Comp Biochem Physiol. 1961;3(4):227–235. doi: 10.1016/0010-406X(61)90008-1
- Lees H. The biochemistry of the nitrifying organisms. 1. The ammonia-oxidizing systems of Nitrosomonas. Biochem J. 1952;52(1):134–139. doi: 10.1042/bj0520134
- Voyles J, Young S, Berger L, et al. Pathogenesis of chytridiomycosis, a cause of catastrophic amphibian declines. Science. 2009;326(5952):582–585. doi: 10.1126/science.1176765
- Therneau TM. A package for survival analysis in R. 2021.
- Kassambara A, Kosinski M, Biecek P, et al. Survminer: drawing survival curves using “ggplot2. 2021.
- Benjamini Y, Hochberg Y. Controlling the false discovery rate: a practical and powerful approach to multiple testing. J R Stat Soc Ser B Methodol. 1995;57(1):289–300. doi: 10.1111/j.2517-6161.1995.tb02031.x
- R Core Team. R: a language and environment for statistical computing. 2020.
- Hothorn T, Bretz F, Westfall P. Simultaneous inference in general parametric models. Biometrical J. 2008;50(3):346–363. doi: 10.1002/bimj.200810425
- Jenkinson TS, Betancourt Román CM, Lambertini C, et al. Amphibian-killing chytrid in Brazil comprises both locally endemic and globally expanding populations. Mol Ecol. 2016;25(13):2978–2996. doi: 10.1111/mec.13599
- Carvalho T, Medina D, P. Ribeiro L, et al. Coinfection with chytrid genotypes drives divergent infection dynamics reflecting regional distribution patterns. Commun Biol. 2023;6(1). doi: 10.1038/s42003-023-05314-y
- Becker CG, Greenspan SE, Tracy KE, et al. Variation in phenotype and virulence among enzootic and panzootic amphibian chytrid lineages. Fungal Ecol. 2017;26:45–50. doi: 10.1016/j.funeco.2016.11.007
- Fu M, Waldman B. Ancestral chytrid pathogen remains hypervirulent following its long coevolution with amphibian hosts. Proc R Soc B. 2019;286(1904):20190833. doi: 10.1098/rspb.2019.0833
- Greener MS, Verbrugghe E, Kelly M, et al. Presence of low virulence chytrid fungi could protect European amphibians from more deadly strains. Nat Commun. 2020;11(1):5393. doi: 10.1038/s41467-020-19241-7
- Grogan LF, Robert J, Berger L, et al. Review of the amphibian immune response to chytridiomycosis, and future directions. Front Immunol. 2018;9. doi: 10.3389/fimmu.2018.02536
- Buck JC, Truong L, Blaustein AR. Predation by zooplankton on Batrachochytrium dendrobatidis: biological control of the deadly amphibian chytrid fungus? Biodivers Conserv. 2011;20(14):3549–3553. doi: 10.1007/s10531-011-0147-4
- De Troyer N, Bruneel S, Lock K, et al. Ratio-dependent functional response of two common cladocera present in farmland ponds to Batrachochytrium dendrobatidis. Fungal Ecol. 2021;53:101089. doi: 10.1016/j.funeco.2021.101089
- Hamilton PT, Richardson JML, Anholt BR. Daphnia in tadpole mesocosms: trophic links and interactions with Batrachochytrium dendrobatidis. Freshwater Biol. 2012;57(4):676–683. doi: 10.1111/j.1365-2427.2011.02731.x
- Schmeller DS, Blooi M, Martel A, et al. Microscopic aquatic predators strongly affect infection dynamics of a globally emerged pathogen. Curr Biol. 2014;24(2):176–180. doi: 10.1016/j.cub.2013.11.032
- McMahon TA, Brannelly LA, Chatfield MWH, et al. Chytrid fungus Batrachochytrium dendrobatidis has nonamphibian hosts and releases chemicals that cause pathology in the absence of infection. Proc Natl Acad Sci, USA. 2013;110(1):210–215. doi: 10.1073/pnas.1200592110
- Humphries JE, Lanctôt CM, Robert J, et al. Do immune system changes at metamorphosis predict vulnerability to chytridiomycosis? An update. Dev Comp Immunol. 2022;136:104510. doi: 10.1016/j.dci.2022.104510
- Carvalho T, Becker CG, Toledo LF. Historical amphibian declines and extinctions in Brazil linked to chytridiomycosis. Proc R Soc B. 2017;284(1848):20162254. doi: 10.1098/rspb.2016.2254
- Venesky MD, Parris MJ, Storfer A. Impacts of Batrachochytrium dendrobatidis infection on tadpole foraging performance. Ecohealth. 2009;6(4):565–575. doi: 10.1007/s10393-009-0272-7
- Marantelli G, Berger L, Speare R, et al. Distribution of the amphibian chytrid Batrachochytrium dendrobatidis and keratin during tadpole development. Pac Conserv Biol. 2004;10(3):173–179. doi: 10.1071/PC040173
- Angilletta MJ. Thermal adaptation: a theoretical and Empirical Synthesis. Oxford, UK: Oxford Univ. Press; 2009.
- Greenspan SE, Migliorini GH, Lyra ML, et al. Warming drives ecological community changes linked to host-associated microbiome dysbiosis. Nat Clim Chang. 2020;10(11):1057–1061. doi: 10.1038/s41558-020-0899-5
- Neely WJ, Greenspan SE, Ribeiro LP, et al. Synergistic effects of warming and disease linked to high mortality in cool-adapted terrestrial frogs. Biol Conserv. 2020;245:108521. doi: 10.1016/j.biocon.2020.108521
- Rollins-Smith LA, Ramsey JP, Pask JD, et al. Amphibian immune Defenses against Chytridiomycosis: impacts of changing environments. Integr Comp Biol. 2011;51(4):552–562. doi: 10.1093/icb/icr095
- Ujszegi J, Bertalan R, Ujhegyi N, et al. “Heat waves” experienced during larval life have species-specific consequences on life-history traits and sexual development in anuran amphibians. Sci Total Environ. 2022;835:155297. doi: 10.1016/j.scitotenv.2022.155297
- Rollins-Smith LA, Le Sage EH. Batrachochytrium fungi: stealth invaders in amphibian skin. Curr Opin Microbiol. 2021;61:124–132. doi: 10.1016/j.mib.2021.04.002
- Medina D, Greenspan SE, Carvalho T, et al. Co-infecting pathogen lineages have additive effects on host bacterial communities. FEMS Microbiol Ecol. 2021;97(4):fiab030. doi: 10.1093/femsec/fiab030
- Wlizla M, McNamara S, Horb ME. Generation and care of Xenopus laevis and Xenopus tropicalis embryos. Methods Mol Biol. 2018;1865:19–32.
- Byrne AQ, Vredenburg VT, Martel A, et al. Cryptic diversity of a widespread global pathogen reveals expanded threats to amphibian conservation. Proc Natl Acad Sci, USA. 2019;116(41):20382–20387. doi: 10.1073/pnas.1908289116
- O’Hanlon SJ, Rieux A, Farrer RA, et al. Recent Asian origin of chytrid fungi causing global amphibian declines. Science. 2018;360(6389):621–627. doi: 10.1126/science.aar1965