ABSTRACT
Shigella spp. are the causative agent of shigellosis (or bacillary dysentery), a diarrhoeal disease characterized for the bacterial invasion of gut epithelial cells. Among the 4 species included in the genus, Shigella flexneri is principally responsible for the disease in the developing world while Shigella sonnei is the main causative agent in high-income countries. Remarkably, as more countries improve their socioeconomic conditions, we observe an increase in the relative prevalence of S. sonnei. To date, the reasons behind this change in aetiology depending on economic growth are not understood. S. flexneri has been widely used as a model to study the pathogenesis of the genus, but as more research data are collected, important discrepancies with S. sonnei have come to light. In comparison to S. flexneri, S. sonnei can be differentiated in numerous aspects; it presents a characteristic O-antigen identical to that of one serogroup of the environmental bacterium Plesiomonas shigelloides, a group 4 capsule, antibacterial mechanisms to outcompete and displace gut commensal bacteria, and a poorer adaptation to an intracellular lifestyle. In addition, the World Health Organization (WHO) have recognized the significant threat posed by antibiotic-resistant strains of S. sonnei, demanding new approaches. This review gathers knowledge on what is known about S. sonnei within the context of other Shigella spp. and aims to open the door for future research on understanding the increasing spread of this pathogen.
Introduction
Diarrhoea is a major life-threatening concern that strongly affects children under 5 years of age. It accounts for 525,000 annual deaths in this age group, mostly occurring in countries under economic development [Citation1,Citation2]. Shigellosis, also known as bacillary dysentery, is the disease caused by species of the genus Shigella when they invade and damage the colonic epithelium. Clinical symptoms of the disease can vary from mild, self-limiting diarrhoea to severe diarrhoea with blood in stools and other complications [Citation3,Citation4]. Noteworthy, according to the Global Enteric Multicentre Study (GEMS), Shigella is the most common pathogen associated with diarrhoea in children aged 2–5 years in low-income countries [Citation5]. Bacillary dysentery is the second cause of diarrhoeal mortality, occasioning 212,438 deaths annually of which 30% are children under 5 years of age [Citation6]. Exposure to Shigella has also been associated with long-term growth problems and neurological development delays in children in low-income countries [Citation7,Citation8].
Belonging to the family Enterobacteriaceae, the genus Shigella are Gram-negative, facultatively anaerobic, rod-shaped, non-motile bacteria that classify into four species: Shigella flexneri, Shigella sonnei, Shigella dysenteriae and Shigella boydii [Citation9]. The current method of classifying the genus is based on serology, by the analysis of their O-antigen type. This divides Shigella into groups or species: group A, or S. dysenteriae, with 15 serotypes; group B, or S. flexneri, with 19 serotypes and subserotypes; group C, or S. boydii, with 20 serotypes; and as an exception, group D or S. sonnei, which presents only one serotype [Citation10].
Shigella spp. differ extensively in their geographical distribution and their contribution to the burden of disease. Currently, S. dysenteriae and S. boydii are seldom isolated, while S. flexneri and S. sonnei contribute to 90% of all cases [Citation5]. In low-income countries in Africa, Asia and South America, the main burden of shigellosis is infection by S. flexneri, although approximately 25% of cases are still caused by S. sonnei [Citation11,Citation12]. In contrast, for the bulk of cases in wealthy countries, predominantly in Europe and North America, the predominant aetiological agent is S. sonnei [Citation13]. Remarkably, global socioeconomic improvements are leading to a shift in shigellosis causative agents, with S. sonnei replacing S. flexneri in many diagnoses in transitional countries [Citation14].
The pathobiology of Shigella spp. has traditionally been studied using S. flexneri as the primary model, but assumptions based on this organism are insufficient to explain the findings of S. sonnei research. As such, further investigations specifically targeting the latter are crucial. This review aims to provide an overview of the biology and virulence of S. sonnei against the backdrop of other Shigella spp., summarizing the current knowledge and pinpointing areas for future study.
Epidemiology
Among the four species of Shigella, S. dysenteriae is considered the most severe and has provoked four pandemics in the second half of the 20th century in America, Africa, and Asia [Citation15,Citation16]. The high virulence profile is associated with the production of the Shiga-toxin Stx [Citation17]. Shigella boydii is acknowledged to be restricted to Bangladesh, India, Nigeria, Yemen, and other South-Eastern Asian countries; this species hardly affects outside these territories except for travel-associated episodes [Citation18]. S. flexneri predominantly causes episodes of shigellosis in low-income countries with inadequate hygienic and sanitary conditions, where problems such as poor water sanitation and malnutrition are common. S. flexneri is accountable for 62% and S. sonnei for 25% of shigellosis cases in Asia and sub-Saharan Africa [Citation19]. However, as hygiene conditions and the economy of the country grows, S. sonnei causes the highest number of cases. 80% of shigellosis episodes across Europe and North America result from S. sonnei infections [Citation13,Citation19–21]. A current issue that demands closer examination is the fact that as more countries industrialize, S. sonnei is surpassing S. flexneri as the aetiological agent of disease [Citation14]. An eight-year analysis conducted between 2004 and 2011 in Beijing (China) showed how S. sonnei has replaced S. flexneri as most prevalent since 2009 [Citation22]. In Vietnam, throughout a period of 14 years (1995–2008) S. sonnei cases increased from 29% to the 78% of Shigella isolations [Citation23]. In Bangladesh, S. sonnei increased from around 10% of all Shigella cases in 2001 to nearly 50% in rural areas and over 50% in urban settings by 2020 [Citation24]. More countries experiencing this phenomenon include Brazil [Citation25], India [Citation26], and Iran [Citation27], among others. In fact, country gross domestic product (GDP) positively correlates with an increased isolation of S. sonnei as the main cause of shigellosis [Citation28].
Improvements in sanitation standards are accompanied by a reduced risk of diarrhoeal diseases, however this does not entirely apply for shigellosis as it appears to not fully extend to S. sonnei. The reason for this shift in the aetiological agent linked to economic and healthcare prosperity is not completely understood, although various hypotheses have been under consideration [Citation14]. One hypothesis was the utilization of amoebae as protective hosts that could increase S. sonnei survival in the environment. Amoebae can be encountered in numerous aquatic (sea, lakes) and terrestrial (soil, dust) niches, but they have also been found to resist water chlorination in drinking water and pools [Citation29]. While amoebae are known to predate on bacteria, some bacterial species have evolved defensive tactics to either replicate within the amoebic cell or live alongside them as a saprophyte [Citation30]. Examples of these associations include Legionella pneumophila, Francisella tularensis, Vibrio cholerae, Mycobacterium leprae or Chlamydia pneumoniae among others [Citation31–33]. However, the theory suggesting that S. sonnei might use amoebae to enhance its survival even in places with good living conditions has proved false; S. sonnei and S. flexneri survive equally in long-term cultures with amoebae, but neither species can survive inside the protozoan cells [Citation34].
One of the most compelling explanations to explain the differences between S. flexneri and S. sonnei geographical distributions has to do with the O-antigen component of lipopolysaccharide (LPS) of the bacterium. The genetic machinery of O-antigen biosynthesis and export in Shigella is mostly located on the chromosome with minor exceptions [Citation35,Citation36]. For instance, some S. flexneri encode a second wzz co-polymerase on the plasmid pHS-2 [Citation36]. S. sonnei diverges from this pattern; the chromosomal locus is disrupted, and the O-antigen cluster is instead harboured on a large virulence plasmid (LVP). The O-antigen locus was acquired via horizontal transfer from the distant gram-negative bacterium Plesiomonas shigelloides [Citation35,Citation37,Citation38]. P. shigelloides is commonly found in contaminated water in low-income countries which may provide natural protection against S. sonnei [Citation37,Citation39]. Conversely, in resource-rich or transitional countries that improve water sanitation, where people have little contact with P. shigelloides, cross-immunity cannot occur and S. sonnei may emerge as the primary cause of shigellosis. Further data collection is required to verify whether exposure to a single serotype of P. shigelloides (O17) occurs at sufficient levels to protect the population against S. sonnei infections.
Key aspects in the evolution of Shigella spp
Shigella spp. are specialized lineages arisen from different E. coli ancestors that evolved convergently. Advances in genome sequencing have enabled the classification of Shigella spp. into three genomic clades, where serotypes distribute without clade-species correlation. This implies that the conventional serological classification of Shigella does not agree with their evolutionary history. In addition to these three clusters, there are several outliers that contain some members of S. dysenteriae, S. boydii and all those belonging to S. sonnei. Confirming the evolutionary relationship between Shigella spp. and E. coli, all clusters and outliers intersperse with E. coli members [Citation10,Citation40]. Phylogenomic analyzes underscore clade differentiation within Shigella [Citation41].
Shigella and E. coli share a core genome of around 3 Mb, however; insertions, deletions, and genetic rearrangements operating in different ancestors have led to a reduction of genome size, accumulation of pseudogenes and loss of biological functions, especially in Shigella [Citation42,Citation43]. Insertion sequences are key to comprehend the evolution of Shigella and many other well-adapted human pathogens [Citation44–47]. Compared to other bacterial species, Shigella genomes contain the highest number of insertion sequences relative to their size [Citation42,Citation48,Citation49]. Owing to the effect of insertion sequences, some core metabolic pathways present in E. coli members are absent in Shigella species. For example, Shigella spp. are unable to ferment lactose due to various alterations in the lac operon [Citation50]. Insertion sequences also inhibit the utilization of tryptophan as a carbon and nitrogen donor [Citation51] and stop conversion of the amino acid lysine into cadaverine due to the mutation of the lysine decarboxylase gene cadA [Citation52]. cadA is considered an antivirulence gene in Shigella that has undergone a high evolutionary pressure to be eliminated from the genome of these species. When cadA is expressed at normal levels (or cadaverine is present), cell invasion and evasion of host immune defences are impaired [Citation53]. In addition, one of the main phenotypical characteristics of Shigella, i.e. the absence of flagellar motility, comes from the accumulation of mutations and insertion sequences in different genes and has been related to reduced recognition of the pathogen by the host immune system [Citation42,Citation54,Citation55]. Insertion sequences operate in the progressive reduction of the genomes of S. flexneri and S. sonnei, resulting in smaller genomes that resemble the hyper-reduced genome of S. dysenteriae. Interestingly, mutations in genes that have already disappeared in S. dysenteriae are emerging in S. flexneri and S. sonnei clones [Citation49]. When certain biochemical pathways are lost in a parallel pattern, it suggests that their absence confers an adaptive advantage to exploit resources in a new environment [Citation52]. In the case of Shigella spp., these convergent alterations that lead to a smaller genome could be improving adaptation to the human host and contributing to pathogenicity.
Horizontal gene transfer is another key phenomenon that explains Shigella spp. evolution. The acquisition of the large virulence plasmid (LVP) pINV on multiple occasions by various E. coli founder ancestors was a crucial factor that gave rise to major Shigella lineages. The plasmid has an approximate length of 220 Kb and harbours a particularly important locus for Shigella pathogenicity, the 30 Kb ipa–mxi–spa region that encodes a type 3 secretion system (T3SS) along with regulatory proteins and effectors [Citation56]. The LVP gives Shigella the capacity to invade host cells and alter the host immune system [Citation15]. This plasmid can be found in two mutually incompatible isoforms, A and B, defined by the sequence of genes mxiA, mxiC, and IpgD [Citation57]. Isoform A is present in clade 1, Isoform B in clade 3 and both in clade 2 [Citation57,Citation58]. Outliers to the clades may harbour either of the two versions [Citation59]. S. sonnei harbours isoform B, and the LVP additionally encodes for the P. shigelloides serogroup 17 O-antigen and has shaped the epidemiology and emergence of this species [Citation35]. Notably, pINV loss is very frequent and higher in S. sonnei compared to S. flexneri. This disparity is attributed to the removal of toxin-antitoxin systems facilitated by the presence of insertion sequences in S. sonnei [Citation60,Citation61].
S. sonnei originated more recently than the rest of Shigella from a common European ancestor, estimated to be less than 500 years old. This ancestral strain underwent subsequent expansion and diversification, leading to the formation of four distinct lineages (Lineage I to Lineage IV). Notably, Europe exhibits a higher level of genetic diversity with representatives of the four lineages [Citation13,Citation35]. Lineage III has exhibited a higher level of success in terms of its global spread, accounting for most strains present in South America, Africa, and Asia [Citation13,Citation14,Citation62,Citation63]. One of key characteristics of lineage III is its higher accumulation of resistances to antibiotics, a fact that is key for its global expansion [Citation55,Citation64].
Shigellosis
Transmission
Humans constitute the only natural reservoir for Shigella species [Citation3]. One of the key factors contributing to their high spread is their very low infective dose, with as few as 10–100 being enough to cause disease [Citation65]. As a result of such small inoculum, the principal way Shigella transmits is through the faecal-oral route from person-to-person. Infections are more frequent in regions or environments without safe conditions of hygiene and water potability [Citation3]. Shigella can also be spread by swallowing water that has been in contact with infected faecal matter [Citation66–68] and is considered a foodborne pathogen, especially associated with unsuitable food-handling practices [Citation69,Citation70]. The clinical management of food and waterborne outbreaks may be complicated because of secondary person-to-person dissemination [Citation70,Citation71]. Propagation may also take place through flies as a mechanical vector [Citation72]. Recent Shigella outbreaks have been transmitted during sexual activity through contact with an infected person’s excrement [Citation73,Citation74].
Symptomatology
All species of Shigella cause a similar range of symptoms, although S. dysenteriae type 1 infections are the most severe [Citation9,Citation75]. S. sonnei infections are often described as less severe than those caused by S. flexneri [Citation76] but clinical comparisons indicate similar symptom severity [Citation77,Citation78]. The time interval between infection and becoming symptomatic is usually 1 to 4 days, although it can extend to 8 days in the case of S. dysenteriae [Citation79]. In some individuals, no symptoms are present, which is crucial for the transmission of the pathogen [Citation80]. In general, in countries with higher resources, while individuals with shigellosis may experience severe symptoms, the illness typically resolves within a short time period. In contrast, in lower resource countries shigellosis is linked with increased levels of morbidity and mortality [Citation4,Citation9,Citation81].
Most infections produced by Shigella spp. cause fever, vomiting, watery diarrhoea, cramps, and abdominal pain [Citation3]. When cases evolve in severity, the presence of blood and/or mucus in faeces (a clinical manifestation of gut epithelial damage) and tenesmus may appear. Patients can also show a wide variety of complications such as intestinal perforation and obstruction, rectal prolapse, recurrent diarrhoea, dehydration, seizures, vaginitis and encephalopathy among others [Citation3,Citation82,Citation83]. Complications have been reported to occur in infections with any Shigella species [Citation23,Citation75]. Young age, HIV immunodeficiency and malnutrition are regarded as risk factors, however it is not a prerequisite since complications may be observed in apparently healthy individuals [Citation3,Citation84].
Groups at higher risk
Children under 5 years of age in low- and middle- income countries
Shigella infections are one of the major life-threatening hazards to which children from low- and middle- income countries, particularly in sub-Saharan Africa and South Asia, are exposed [Citation3,Citation23,Citation24,Citation82,Citation85]. Shigella is the most prevalent cause of diarrhoeal illness among children between the ages of two and five, and the second most common cause in children below two years of age [Citation86]. The true burden of child shigellosis in low-resource settings is often underestimated. The World Health Organization (WHO) recommends antibiotic administration for children only when dysentery (bloody depositions) is observed. However, dysentery by itself is not an accurate predictor of Shigella since many fatal infections occur without this symptom [Citation87]. Therefore, antibiotic administration to particularly vulnerable children without dysentery should be considered in order to minimize the risk of complications [Citation85,Citation87,Citation88].
Children in day-care centres and crowded settings
In high-income nations, children are also particularly targeted by the disease. The close contact between children as well as their poor toileting skills facilitate Shigella outbreaks in day care centres, schools, and other crowded settings. Secondary cases affecting other members of the family or the community are common [Citation89–92]. As an example, in northwest Missouri almost half of the cases of shigellosis reported from May to October 2005, occurred in children in day-care centres, their families or people working in these centres [Citation90].
Men who have sex with men (MSM)
Men who have sex with men (MSM) are at a higher risk of contracting shigellosis due to the increased likelihood of direct or indirect faecal-oral contact during sexual activity [Citation93]. Although S. flexneri serotypes 2a and 3a account for numerous cases, the majority of sexually transmitted Shigella infections are caused by S. sonnei [Citation20,Citation73]. In recent years, multidrug resistant S. sonnei has caused multiple outbreaks among MSM in European countries [Citation94–98], Australia [Citation74] and in the United States [Citation99]. According to recent data, the incidence of shigellosis among MSM in England has almost doubled during the last 10 years, with more than 90% of all isolates showing high resistance to multiple antibiotics [Citation100]. The existence of recurrent and persistent infections among MSM highlights the need for new strategies to tackle the infection [Citation101].
Persons experiencing homelessness (PEH)
People experiencing homelessness (PEH) face conditions that may predispose for contracting shigellosis both sleeping outdoors or when in shelters i.e. high infectious dose, exposure to contaminated food and water, overcrowding, drug use and poor access to hygiene and sanitation [Citation102–104]. An increasing number of highly antibiotic resistant outbreaks of shigellosis, mostly caused by S. sonnei, affecting PEH in HIC has been described in recent years [Citation78,Citation102,Citation103,Citation105–107]. Over half of the shigellosis infections identified in Seattle (US) between 2017 and 2022 affected PEH [Citation78]. Heavy precipitations are associated with a higher propagation in PEH to higher contamination of drinking water sources and overcrowding [Citation105]. Bacteriemia may occur, although immunocompromise does not fully account for the severity of cases affecting PEH [Citation102].
International travellers to endemic regions
Travelling to zones where Shigella is endemic can pose health risks due to a lack of natural immunity and prophylaxis measures [Citation108]. Shigella spp. are the cause of a significant number of diarrhoeal outbreaks during travel or military deployment in endemic countries [Citation109–111]. In 2022, an outbreak caused by a multidrug-resistant (MDR) S. sonnei occurred among travellers from different European countries that were found to have stayed in the same facilities during a visit to Cape Verde [Citation112]. Beyond the impact on the individual, contracting shigellosis while travelling can result in the introduction of antibiotic-resistant strains into new populations [Citation113].
Pathogenesis of Shigella spp
Blood and mucus in faeces are prominent clinical features of shigellosis that derive from extensive colonic epithelial damage and a highly inflammatory response. To comprehend these clinical manifestations, a thorough understanding of Shigella pathogenesis is crucial. Most data on Shigella pathogenesis have been elucidated from studies carried out in S. flexneri. However, notable discrepancies with S. sonnei have recently been highlighted. The absence of an animal model that thoroughly recapitulates human shigellosis restricts pathogenesis studies. Mice present limitations as they are poorly colonized by Shigella without prior antibiotic treatment [Citation114]. Other animals such as rabbits and guinea pigs have been valuable to study Shigella-host interactions [Citation115–119]. In recent years, the zebrafish model has emerged as a promising tool to study the pathogenesis of both S. flexneri and S. sonnei [Citation120–122], and human intestinal organoids have shown great potential [Citation123].
The prevailing dogma for Shigella pathogenesis encompasses the following phases: bacterial cells reach the colonic epithelium and undergo transcytosis to the submucosa by M-cells, they are subsequently internalized by macrophages inducing their pyroptosis, they invade intestinal epithelial cells where they replicate in the cytosol, and they spread to neighbouring cells by actin polymerization. Shigella pathogenesis is summarized in . These processes will be discussed in more detail below using research from S. flexneri. But where available, research on S. sonnei pathogenesis will be highlighted.
Figure 1. Shigella spp. pathogenesis. After ingestion and in its transit towards the intestine, Shigella must resist hostile conditions of the gastrointestinal tract e.g. stomach acidic pH and bactericidal action of bile salts. Once in the gut, Shigella competes with members of the commensal microbiota for space and resources. In the colonic epithelium, M cells transcytose Shigella from the apical to the basolateral side where it is internalized by macrophages. Shigella avoids vacuole-mediated macrophage degradation and induces their inflammatory pyroptotic cell death. Inflammation recruits neutrophils, which are efficient killers of Shigella. However, neutrophil migration damages the epithelial layer. Released from macrophages, Shigella invades epithelial cells through their basolateral side, multiplies intracellularly suppressing immune responses and cell death to extend the life of the infected cell. Bacteria then spread into neighbouring cells through actin-based motility. Finally, Shigella is released from the host through faecal matter.
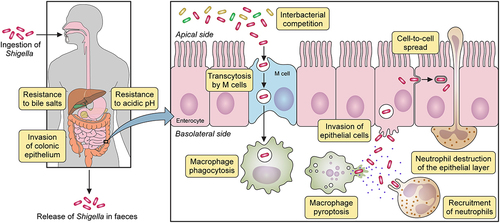
Shigella type III secretion system (T3SS)
Type III secretion systems (T3SSs), present in a wide range of Gram-negative species, mediate bacteria-host cellular interactions [Citation124]. The T3SS of Shigella is a fundamental element of its pathogenesis, as it enables the invasion of intestinal epithelial cells and the manipulation of host immune responses in various cell types. The system sequentially translocates effectors from the bacterial cytoplasm to the surrounding space or directly into target cells [Citation125]. All genes encoding for T3SS structural components, and most regulators and effectors are found on the LVP pINV [Citation15,Citation56,Citation126].
Shigella T3SS is a syringe-shaped complex that consists of a cytosolic sorting platform, a basal body spanning alongside the outer and inner membrane, and a needle that projects towards the extracellular space. The detailed structure of Shigella T3SS has been reviewed elsewhere [Citation125,Citation127]. The needle tip senses contact with the host and recruits IpaB and IpaC, also known as translocon proteins, which are secreted to form pores in the host membrane through which other effectors are delivered [Citation128–130].
The expression of T3SS structural components and effectors is tightly regulated [Citation131]. Under non-physiological temperatures (below 30°C), repressor histone-like nucleoid structuring protein (H-NS) represses expression of LVP-encoded transcriptional regulators VirF and VirB, and members of their regulons [Citation125,Citation132,Citation133]. Apart from alleviating such repression, after colonziation of a human body, temperatures over 37°C cause a change in DNA topology that allow the production of VirF [Citation134], which activates the transcription of virB [Citation132] and adhesin icsA [Citation135]. VirB counteracts H-NS-mediated silencing of virulence genes [Citation136]. The presence of VirB leads to the upregulation of MxiE which activates the expression of late effectors and also relieves H-NS silencing [Citation125,Citation137,Citation138].
Phases of Shigella infectious cycle
Resistance to host barriers along the gastrointestinal tract
Upon entering in the human body, Shigella must tolerate the pH changes along their progression through the gastrointestinal tract towards the colon. In the stomach, it encounters highly acid conditions with a pH ranging from 1.5 to 2.8 [Citation139]. However, as it continues heading to the small intestine, the pH becomes more alkaline (around 7.7). Ultimately, Shigella reaches its preferred site of infection, the colon, where the pH becomes slightly lower at around 6.4 [Citation140]. Early studies demonstrated that Shigella can resist acidic environments for 2 hours at a pH as low as 2.5 [Citation141]. Acid resistance is crucial for such a low bacterial dose to cause disease [Citation142]. In addition, acidic conditions trigger virulence functions such as expression of the T3SS and biofilm formation [Citation143].
Shigella mainly uses two pathways to counteract acidic conditions: the glutamate-dependent acid-resistance (GDAR) pathway and the oxidative pathway. The GDAR pathway is formed by decarboxylases GadA and GadB, and the glutamate/GABA antiporter GadC. The oxidative pathway works in the absence of glutamate and is inhibited by fermentative glucose-rich conditions [Citation142,Citation144]. Chaperones HdeAB also play a crucial role in the ability of Shigella to survive in these conditions [Citation144,Citation145]. The RNA polymerase RpoS, the transcriptional regulator SlyA and the RNA-binding chaperone Hfq have been found to be positive regulators of hdeAB and GDAR pathway genes [Citation142,Citation146,Citation147]. Hfq regulates the expression of T3SS regulator VirB and a mutant exhibits reduced virulence and antibiotic resistance [Citation148]. The transcriptomic study of this regulator revealed a genetic network that requires further characterization [Citation149].
Bile, produced by the liver and secreted to the intestine, helps digestion and contains bile salts which facilitate bacterial clearance [Citation150]. LPS and the AcrAB efflux pump play an important role in the resistance of Shigella to bile salts. Primary bile salt cholate and secondary bile salt deoxycholate promote biofilm formation (when glucose is present), which facilitates transit through the small intestine [Citation151]. Bile salts also induce OspE1 and OspE2 expression and OspE1/2-mediated cell adherence and type 1 fimbriae expression in S. flexneri [Citation151–153]. Deoxycholate promotes the recruitment of IpaB and IpaD to the tip of the T3SS complex [Citation130,Citation154] and induces IcsA-mediated adhesion and biofilm formation in S. flexneri [Citation155,Citation156]. However, deoxycholate downregulates IcsA expression and invasion in S. sonnei [Citation157]. The different responses of S. flexneri and S. sonnei to bile salts and its relationship to cell invasion deserve further characterization.
Enteric pathogens must also penetrate the mucus layer that protects intestinal epithelial cells. The major component of mucus is the glycoprotein mucin [Citation158]. Shigella both triggers host mucus production, a process accompanied by high inflammation [Citation159–162], and degrades mucins [Citation163,Citation164]. Shigella protease Pic (from the family of Serine Protease Autotransporters of Enterobacteriaceae (SPATE)) presents mucolytic activity [Citation165], promotes mucus secretion by specialized intestinal cells [Citation160]. Mucus restricts oxygen diffusion and the relatively higher oxygen concentration at the epithelium surface compared to the lumen activates the T3SS promoting invasion [Citation166].
Translocation to the basolateral side of the colonic epithelium
After overcoming the above-mentioned barriers, Shigella arrives at the apical side of the intestinal epithelium. While Shigella may adhere to the apical surface of intestinal epithelial cells [Citation153,Citation167] its ability to invade intestinal epithelial cells is greater through their basolateral side [Citation162,Citation168,Citation169]. Shigella takes advantage of the function of microfold (M) cells to be transcytosed and access the basolateral side [Citation115]. M cells are located in the follicle-associated epithelium (FAE). They work in the presentation of antigens from the intestinal lumen to the underlying subepithelial lymphoid tissue i.e. criptopatches, Peyer’s patches and other lymphoid follicles [Citation170]. Transcytosis is not generally harmful to M cells as the bacteria remain within vacuoles [Citation115], although some M cell cytosolic invasion and subsequent lateral spread to adjacent epithelial cells can occur [Citation169].
Shigella-induced macrophage pyroptosis and interactions with neutrophils
Once at the basolateral side, Shigella encounters macrophages [Citation168]. Other pathogens have evolved to replicate inside macrophages, but Shigella induces macrophage cell death to be able to reach its favourite niche i.e. colonic epithelial cells [Citation171]. Following engulfment by macrophages, Shigella cells are sequestered within vacuoles. Shigella employs the T3SS to escape the vacuole and gain entry to the macrophage cytosol. Notably, vacuole escape also occurs during invasion of epithelial cells and the molecular mechanisms are thought to occur in a similar way in both cell types, although this requires further assessment. IpaB appears to be a major player in macrophage vacuole escape, as it creates pores in the phagosome membrane leading to its rupture [Citation172,Citation173].
Once in the cytosol, Shigella induces macrophage pyroptosis to evade antimicrobial action and allow invasion of epithelial cells [Citation171,Citation173–176]. Pyroptosis is an inflammatory form of cell death, driven by the assembly of inflammasomes and activation of caspase 1, that culminates with the release of proinflammatory cytokines interleukin-1β (IL-1β) and IL-18, the entry of excessive fluid into the cell, osmotic lysis, and leakage of contents [Citation176–180]. Major inducers of pyroptosis in Shigella include T3SS constituents MxiH, MxiI [Citation181,Citation182] and cytosolic potassium decrease post-vacuole escape [Citation183,Citation184]. From a host perspective, pyroptosis is beneficial as it eliminates infected cells and exposes intracellular pathogens to an immune response [Citation185]. Thus, the role of pyroptosis in Shigella infections is ambiguous, leaving questions about its impact for the host and the bacterium. Early observations described that S. sonnei caused less macrophage death than S. flexneri [Citation186]. Recent findings highlight that S. sonnei induces reduced macrophage pyroptosis due to reduced internalization and vacuole escape, and induces less inflammation, likely due to shielding of the T3SS by its O-antigen. S. sonnei entry into macrophages appears to be by phagocytosis rather than bacterial-mediated invasion [Citation187] ().
Figure 2. Differences in the interactions with macrophages and epithelial cells between Shigella flexneri and Shigella sonnei. (a) Shigella flexneri (depicted in magenta) shows increased internalization into macrophages mediated by its type 3 secretion system (T3SS), whereas the low internalization of Shigella sonnei (depicted in blue) depends predominantly on phagocytosis. Shigella sonnei also escapes from the shigella containing vacuole less efficiently in comparison to Shigella flexneri, which leads to a reduced cytosolic bacterial count. As a consequence, shigella sonnei induces less macrophage pyroptosis than Shigella flexneri. These differences are attributed to the double layer of O-antigen (found in the LPS and the group 4 capsule) in the cell envelope of Shigella sonnei (b) in contrast to Shigella flexneri infections, characteristic membrane ruffling is not observed upon invasion of epithelial cells by Shigella sonnei. The reduced invasion results in reduced intracellular growth of shigella sonnei compared to Shigella flexneri. Similar to macrophage interactions, these differences are linked to the presence of the double layer of O-antigen in Shigella sonnei.
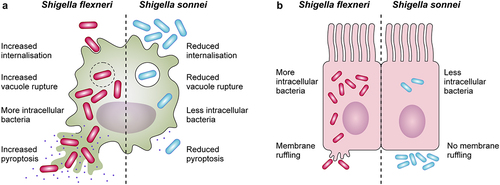
The highly inflammatory environment resulting from Shigella-induced macrophage pyroptosis exposes the bacterium to other immune components and cells e.g. neutrophils. Neutrophils resist induced pyroptosis better than macrophages and kill Shigella more efficiently by the action of reactive oxygen species (ROS), granule-contained antimicrobial molecules, and neutrophil extracellular traps (NETs) [Citation188–191]. However, their migration to the infection site also contributes to damage since it disrupts the intestinal layer and favours further entry of Shigella to the basolateral side [Citation192–194]. S. flexneri and S. sonnei have been shown to cause neutrophil death by necrosis [Citation122,Citation195]. Interestingly, the O-antigen of S. sonnei is crucial for protection from neutrophil clearance in zebrafish [Citation122]. The study of interactions between Shigella and neutrophils is still in its infancy.
Adhesion and invasion of epithelial cells
Liberation from macrophages allows Shigella to contact the basolateral side of intestinal epithelial cells, where it binds different surface components. T3SS effectors IpaB, IpaC and IpaD bind fibronectin receptor α1β5 integrin [Citation196]. IpaB can additionally bind hyaluronic acid receptor CD44 in cholesterol-rich domains [Citation197,Citation198]. Other components involved in adhesion include IcsA [Citation155,Citation157], MAM SSO1327 (exclusive to S. sonnei) [Citation157], and the LPS [Citation199].
Shigella invasion of epithelial cells is T3SS-dependent [Citation126,Citation200]. After contact with the host and IpaB-IpaC-mediated pore formation [Citation201], effectors delivered into epithelial cells enable efficient attachment e.g. the IpaC-vimentin association [Citation202], and manipulate host cytoskeletal actin architecture [Citation203,Citation204]. Interestingly, a microscopic analysis of S. sonnei interaction with epithelial cells did not show typical T3SS-mediated membrane protrusions and ruffling [Citation187] (). On the basis of this result, the way S. sonnei invades epithelial cells requires further investigation.
Shigella intracellular survival in epithelial cells and cell-to-cell spread
Inside epithelial cells, like in macrophages, Shigella is first contained within vacuoles from where it must escape [Citation172,Citation205]. In order to survive intracellularly, Shigella must resist epithelial mechanisms of defence e.g. autophagy. Autophagy maintains cellular homoeostasis by recycling cellular components and eliminating intracellular pathogens in a lysosome-dependent manner [Citation206]. NOD receptors detect cytosolic peptidoglycan and activate autophagy markers [Citation207]. However, IcsA and IcsB can counteract autophagy by preventing bacterial recognition by such markers [Citation208,Citation209].
The cytosol of intestinal epithelial cells are Shigella’s preferred niche for replication. A proteomic study of intracellular S. flexneri revealed metabolic adaptations to this lifestyle. Intracellular iron levels are kept low due to its high toxicity, so Shigella up-regulates iron acquisition systems (Lut, Sit, FhuA and Feo) and the iron starvation-responsive protein Suf. As well, low intracellular oxygen levels make Shigella rely on mixed-acid fermentation (rather than respiration) using primarily pyruvate as a source of energy [Citation210].
When the cytosolic niche of Shigella is eventually compromised, cell-to-cell spread is facilitated by actin polymerization by the autotransporter IcsA [Citation211,Citation212]. Double membrane vacuoles (DMVs) containing bacteria during intercellular spread can be degraded by IpgB1 [Citation213]. As S. sonnei exhibits reduced invasion into epithelial cells [Citation187,Citation214] it will be interesting to evaluate whether it is also adapted to intracellular survival.
Prevention of epithelial cell death
Unlike the rapid pyroptosis induced in macrophages Shigella prevents cell death (apoptosis, necrosis and pyroptosis) in epithelial cells to safeguard its replicative niche [Citation215–217]. Effectors VirA and IpgD promote degradation of pro-apoptotic factor p53 [Citation218,Citation219] and Spa15 prevents caspase 3 activation [Citation220]. Necrosis is inhibited by NOD recognition of peptidoglycan, dampening BNIP3-CypD signalling [Citation221]. S. flexneri induces less pyroptosis by changing its LPS from a hexaacylated to a tetraacylated form that is poorly recognized by the host [Citation222]. Effectors OspC3, VirA, IpaJ, and IpaB reduce inflammatory responses [Citation223–227]. Therefore, in epithelial cells S. flexneri induces a delayed necrotic cell death.
Molecular mechanisms of S. sonnei virulence
The differences between S. flexneri and S. sonnei discussed throughout this review have served to re-evaluate the study of specific virulence factors in S. sonnei. Even though both species share a T3SS involved in cell invasion and host immune manipulation, invasion differs between the two. S. sonnei also has been found to possess unique virulence factors: the adhesin MAM SSO1327, a group 4 capsule, a unique O-antigen and mechanisms to compete with commensal bacteria. A schematic representation of S. sonnei virulence factors is shown in . Uncovering S. sonnei virulence particularities is fundamental to tackle its escalating prevalence and to develop tailored prevention and control measures.
Figure 3. Shigella sonnei virulence factors. The large virulence plasmid (LVP) of Shigella sonnei plays a pivotal role in its virulence since it encodes for adhesin IcsA, the type 3 secretion system (T3SS) and the O antigen (O-Ag) synthesis cluster. The type 6 secretion system (T6SS), adhesin MAM and the capsule export locus are encoded on the chromosome, and colicins are usually encoded on other plasmids. During the invasion of epithelial cells, outer membrane proteins MAM and IcsA act as adhesins that facilitate T3SS-mediated invasion. The T3SS forms pores in epithelial cell membranes that enable the subsequent delivery of effectors that manipulate host cells. The interaction of Shigella sonnei with epithelial cells needs further characterization. Shigella sonnei has also evolved mechanisms to compete with other members of the intestinal microbiota, including a T6SS that requires close contact and colicins that target phylogenetically closely related bacteria and are released after bacterial lysis. Shigella sonnei produces a double O-Ag layer that is present in the LPS and the group 4 capsule and is involved in serum resistance.
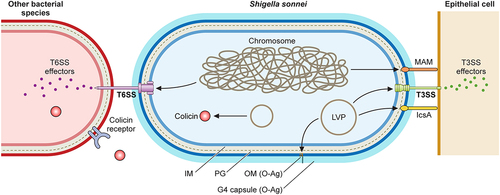
Adhesins
Adhesion is key in the first stages of pathogen-host interactions. So far, two adhesins have been discovered in S. sonnei: multivalent adhesion molecule (MAM) SSO1327 encoded on the chromosome and IcsA encoded on the LVP.
MAMs (multivalent adhesion molecules) are outer membrane proteins broadly distributed among Gram negative bacteria that act in host recognition and attachment [Citation228]. S. sonnei MAM SSO1327 is an adhesin that initiates, via fibronectin and phosphatidic acid, bacteria-host cell close contact needed for posterior cell invasion. Interestingly, in S. flexneri the MAM SSO1327 homologue is a pseudogene. A deletion mutant in S. sonnei SSO1327 presented less ability to adhere and invade epithelial cells and macrophages. In addition, it displayed low T3SS effector translocation and invasion of cells, despite secreting these effectors at wild type levels. Therefore, it has been suggested that this intimate contact, facilitated by SSO1327, activates the T3SS to translocate effectors into the target cell and allows subsequent invasion. Mutants lacking SSO1327 function cause less mortality in animal challenges [Citation157].
The S. sonnei autotransporter IcsA acts synergistically with SSO1327 [Citation157]. IcsA regulation differs in S. sonnei and S. flexneri. The bile salt deoxycholate upregulates IcsA-mediated cell adherence, invasion and biofilm formation in S. flexneri [Citation151,Citation155,Citation229] but downregulates the expression of both SSO137 and IcsA, and consequently cell invasion, in S. sonnei [Citation157]. These differences in the inducing stimuli may be key to understand early stages of S. sonnei infections.
O-antigen
The LPS of Gram-negative bacteria is constituted by lipid A, core, and O-antigen. Unlike other Shigella spp. whose O-antigen synthesis genes are mostly encoded on the chromosome, those of S. sonnei are encoded on the LVP and were horizontally acquired from the environmental bacterium P. shigelloides [Citation35,Citation36,Citation38,Citation230]. The composition of this acquired O-antigen is unique as it contains two very infrequently encountered sugars: 2-acetamido-2-deoxy-L-altruronic acid and 2-acetamido-2-deoxy-L-fucose [Citation230]. These sugars are also incorporated into the capsule of S. sonnei [Citation214]. The genetic determinants for the synthesis of the O-antigen are encoded on the LVP but the export locus for capsule is encoded on the chromosome enabling genetic distinction [Citation38,Citation214].
S. sonnei O antigen plays a major role in virulence. In the zebrafish model, S. sonnei exhibits higher virulence than S. flexneri since a lower infective dose causes higher mortality. It was also found that a mutant deficient in O antigen synthesis exhibits a large attenuation of its infectivity. S. sonnei O-antigen confers resistance to neutrophil acidification [Citation122]. Compared to S. flexneri, when in contact with macrophages S. sonnei induces less pyroptosis as it does not reach the cytosol efficiently. This is due to the double layer of O-antigen around S. sonnei cells that can act as a canopy to impair the action of the T3SS [Citation187]. This is also seen in epithelial cells where mutants in which all O-antigen (LPS and capsule) are removed, and therefore the T3SS is more accessible, show greater invasion [Citation214]. During interactions with macrophages a similar pattern occurs, so mutants where these layers are absent show greater internalization and cause increased pyroptosis [Citation187].
Group 4 capsule
Polysaccharides capsules are common bacterial virulence factors that act in protection against environmental stresses and evasion of the host immune system. They classify into four groups depending on genetic and biochemical factors [Citation231]. Group 4 capsules utilize the O-antigen sugars to form a high molecular weight capsule, but how these sugars are anchored to the membrane remains to be identified [Citation214]. S. flexneri O-antigen can only be found associated with the LPS and its capsule export operon is inactive [Citation232].
The S. sonnei capsule plays an important role in resistance to serum complement [Citation214]. Interestingly, S. flexneri regulates the length of its O-antigen, with short O-antigens resulting in higher levels of T3SS-mediated invasion and longer O-antigens mediating complement killing resistance [Citation222,Citation233,Citation234]. S. sonnei has a shorter LPS in comparison but serum complement resistance can be mediated by the high-molecular weight capsule. Therefore the S. sonnei group 4 capsule may be an alternative to regulation of O-antigen length in S. flexneri. S. sonnei non-capsular mutants are less able to cause systemic infections, however they show enhanced capacity to invade intestinal epithelial cells, induce more inflammation, cytokine production and tissue damage. This has been attributed to the fact that IpaB effector of the T3SS is less accessible when the capsule is present [Citation214]. The regulation of capsule production in S. sonnei and how it modulates the equilibrium between virulence and immune evasion deserve further study.
Interference competition: T6SS and colicins
The human gut is one of the richest microbial ecosystems in terms of diversity, including more than 1000 different species, and of density, with an estimation of 1011 bacterial cells per gram of colonic content [Citation235,Citation236]. Gut flora plays a key role in nutrition but also constitutes a defence mechanism against microbial pathogens. To establish infection, gut pathogens must compete with resident commensal bacteria for nutrients and space [Citation237]. Two mechanisms have been described in S. sonnei that would allow it to compete with resident gut bacteria: the type 6 secretion system (T6SS) and colicins.
More than 25% Gram-negative bacteria harbour T6SSs, including intestinal pathogens such as Vibrio cholerae, Salmonella enterica or Yersinia enterocolitica [Citation238,Citation239]. T6SS are syringe-like molecular machines that operate by injecting toxic proteins into other bacterial species, or in some cases into eukaryotes. A T6SS has been identified in S. sonnei but is absent in S. flexneri. The T6SS was shown to be active against both S. flexneri and E. coli in vitro and in vivo in a mouse model [Citation240]. In terms of the interaction with eukaryotic cells, knockout T6SS mutants displayed no differences in virulence compared to wild type strains in a zebrafish model, suggesting that toxic effects are exerted only against other bacterial species [Citation122]. The main function of this T6SS system would therefore be to provide S. sonnei with an advantage over other bacteria in colonizing the gut.
Colicins are a subset of bacteriocins that are produced by some bacterial strains/species to displace other closely related bacterial strains/species [Citation241]. The killing of target cells does not require bacterial contact; colicins are secreted to the external medium by lysis and subsequently introduced into the target cells either by diffusion or by active transport. Bacteria carrying colicins can express and harbour these toxins because they also encode for immunity genes to prevent toxicity. The spB plasmid, which encodes for class E colicins, has been found in S. sonnei but not in S. flexneri [Citation242]. Unlike the T6SS that is beneficial to the bacteria that encode it, colicins aid the general population by removing closely related species. A recent large-scale genomic analysis of S. sonnei strains identified genes encoding for colicins active against E. coli rather than the T6SS as being responsible for successful S. sonnei expansion [Citation243]. These observations demonstrate that further studies are needed to characterize and elucidate the mechanisms that allow S. sonnei to displace the gut microbiota and establish infection.
Treating S. sonnei infections: Current options and their limitations
A licenced and effective vaccine against Shigella is not yet available despite ongoing research into different approaches. Given that serotype-specific immunity occurs, one of the strategies where most progress has been made is the development of an O-antigen glycoconjugate vaccine. Another goal is to use conserved proteins that may give multivalent protection against different serotypes, simplifying production and cost [Citation244]. Considering the current lack of a vaccine, antimicrobials are key to mitigate the impact of the disease. While rehydration therapy is typically sufficient for mild shigellosis, severe cases require antibiotics to minimize illness duration, severity, and transmission.
According to WHO guidelines, the fluoroquinolone ciprofloxacin is the primary antibiotic recommended for the treatment of shigellosis occurring with dysentery. Alternatives include azithromycin (macrolide), ceftriaxone, cefixime (both cephalosporins), co-trimoxazole (a combination of sulfamethoxazole and trimethoprim) and ampicillin [Citation245,Citation246]. In recent years, there has been a concerning rise in the isolation of S. sonnei strains exhibiting resistance to these antimicrobials ([Citation247–249]. Some isolates are resistant to nearly all used antibiotics [Citation250]. This trend has prompted the WHO and the Centers for Disease Control and Prevention (CDC) to categorize S. sonnei as a priority organism, demanding immediate attention for the development of innovative treatment lines [Citation251,Citation252].
Antibiotic resistance emergence in S. sonnei is associated with diverse mutations or the incorporation of drug-resistance plasmids acquired by horizontal transfer. Commensal intestinal bacteria act as a reservoir for mobile genetic elements present in S. sonnei [Citation253]. S. sonnei lineage III, the most prevalent lineage, exhibits the highest degree of antibiotic resistance. The first resistances that appeared were against quinolones with mutations in the gyrA and parC genes common [Citation254]. Resistance has also been reported against azithromycin through acquisition of the mdhA gene present in a plasmid [Citation253]. Of particular concern is the emergence of resistance against alternative lines of therapy. The presence of B lactamases acting against cephalosporins such as ceftriaxone have also been described in S. sonnei, associated with the presence of the blaCTX-M gene [Citation250]. Antibiotic availability to treat Shigella sonnei infections continues to decrease [Citation255]. For example, a recent study carried out in China reported several outbreaks caused by S. sonnei isolates showing low susceptibility to fluoroquinolones, resistance to ceftriaxone, azithromycin and colistin (associated with a plasmid-encoded mcr1 gene) [Citation250]. Extensively drug-resistant (XDR) shigellosis in the US rose from 0% (2015) to 5% (2022) with S. sonnei being responsible for two-thirds of infections [Citation256]. One of the main scientific challenges in Shigella research will be to find novel measures to treat these multi drug-resistant (MDR) and XDR infections without promoting resistance in other bacteria.
Concluding remarks and future directions
S. sonnei is the major cause of bacillary dysentery in the developed world. No definitive answer has been found to link the association of economic prosperity and S. sonnei prevalence, and there remains much to explore about the biology of this emerging human-restricted pathogen.
Throughout this review, it has been discussed how S. sonnei has evolved distinctive features that set it apart from other species of the genus. It is distinguished by a very high person-to-person spread and high levels of antimicrobial resistance. There is an urgent need for effective treatment alternatives, as conventional first- and second-line antibiotics are increasingly rendering ineffective. The lack of an effective vaccine poses a significant challenge in mitigating this issue. Research into the species pathogenesis have brought to light discrepancies from the model bacterium S. flexneri, highlighting the fact that S. sonnei is less adapted to an intracellular lifestyle and interacts differently with host defences. In addition, S. sonnei encodes for specific virulence determinants (a unique O-antigen, the presence of capsule, a novel adhesin) and has mechanisms to displace other enteric bacteria. More studies are needed on the identification of novel virulence factors, their regulation and how they act altogether during the infection process. A well-established animal model that reproduces human shigellosis caused by S. sonnei would benefit this research.
Gaining deeper insights into its virulence determinants, antibiotic resistance mechanisms, and interactions with the host, will pave the way for new approaches to prevent and control S. sonnei infections.
Disclosure statement
No potential conflict of interest was reported by the author(s).
Data availability statement
Data sharing is not applicable to this article as no new data were created or analysed in this study.
Additional information
Funding
References
- Das JK, Salam RA, Bhutta ZA. Global burden of childhood diarrhea and interventions. Curr Opin Infect Dis. 2014;27(5):451–21. doi: 10.1097/QCO.0000000000000096
- World Health Organization. Diarrhoeal disease. https://www.who.int/news-room/fact-sheets/detail/diarrhoeal-disease.
- Kotloff KL, Riddle MS, Platts-Mills J, et al. Shigellosis. Lancet. 2018;391(10122):801–812. doi: 10.1016/S0140-6736(17)33296-8
- Baker S, The HC. Recent insights into Shigella: a major contributor to the global diarrhoeal disease burden. Curr Opin Infect Dis. 2018;31(5):449. doi: 10.1097/QCO.0000000000000475
- Kotloff KL, Nataro JP, Blackwelder WC, Nasrin D, Farag TH, Panchalingam S, et al. Burden and aetiology of diarrhoeal disease in infants and young children in developing countries (the global enteric multicenter study, GEMS): a prospective, case-control study. Lancet (London, England). 2013;382(9888):209-222. 10.1016/S0140-6736(13)60844–2.
- Khalil IA, Troeger C, Blacker BF, Rao PC, Brown A, Atherly DE, et al. Morbidity and mortality due to shigella and enterotoxigenic Escherichia coli diarrhoea: the global burden of disease study 1990–2016. Lancet Infect Dis. 2018;18(11):1229–1240.
- Rogawski ET, Liu J, Platts-Mills J, et al. Use of quantitative molecular diagnostic methods to investigate the effect of enteropathogen infections on linear growth in children in low-resource settings: longitudinal analysis of results from the MAL-ED cohort study. Lancet Glob Health. 2018;6(12):e1319–e1328.
- Anderson JD, Bagamian KH, Pecenka CJ, et al. Potential impact and cost-effectiveness of Shigella vaccination in 102 low-income and middle-income countries in children aged 5 years or younger: a modelling study. The Lancet Global Health. 2023;11(6):e880–e891. doi: 10.1016/S2214-109X(23)00192-4
- Niyogi SK. Shigellosis. J Microbiol. 2005;43(2):133–143.
- The HC, Thanh DP, Holt KE, Thomson NR, Baker S. The genomic signatures of Shigella evolution, adaptation and geographical spread. Nature Rev Microbiol. 2016;14(4):235–250. doi: 10.1038/nrmicro.2016.10
- Livio S, Strockbine NA, Panchalingam S, Tennant SM, Barry EM, Marohn ME, et al. Shigella isolates from the global enteric multicenter study inform vaccine development. Clinl Infect Dis. 2014;59(7):933–941.
- Kahsay AG, Muthupandian S. A review on Sero diversity and antimicrobial resistance patterns of Shigella species in Africa, Asia and South America, 2001–2014. BMC Res Notes. 2016;9(1):1–6. doi: 10.1186/s13104-016-2236-7
- Holt KE, Baker S, Weill F, et al. Shigella sonnei genome sequencing and phylogenetic analysis indicate recent global dissemination from Europe. Nat Genet. 2012;44(9):1056–1059.
- Thompson CN, Duy PT, Baker S, Clements ACA. The rising dominance of Shigella sonnei: an intercontinental shift in the etiology of bacillary dysentery. PLoS negl trop dis. 2015;9(6):e0003708. doi: 10.1371/journal.pntd.0003708
- Mattock E, Blocker AJ. How do the virulence factors of Shigella work together to cause disease? Front Cell Infect Microbiol. 2017;7:64. doi: 10.3389/fcimb.2017.00064
- Kotloff KL. Shigella infection in children and adults: a formidable foe. Lancet Glob Health. 2017;5(12):e1166–e1167. doi: 10.1016/S2214-109X(17)30431-X
- Melton-Celsa A, Sperandio V, Hovde CJ. Shiga toxin (Stx) classification, structure, and function. Microbiol Spectr. 2014;2(4):2–4. doi: 10.1128/microbiolspec.EHEC-0024-2013
- Kotloff KL, Winickoff JP, Ivanoff B, et al. Global burden of shigella infections: implications for vaccine development and implementation of control strategies. Bullet World Health Organ. 1999;77(8):651.
- Gu B, Cao Y, Pan S, et al. Comparison of the prevalence and changing resistance to nalidixic acid and ciprofloxacin of Shigella between Europe–America and Asia–Africa from 1998 to 2009. Int J Antimicrob Agents. 2012;40(1):9–17.
- Bardsley M, Jenkins C, Mitchell HD, et al. Persistent transmission of shigellosis in England is associated with a recently emerged multidrug-resistant strain of Shigella sonnei. J Clin Microbiol. 2020;58(4):1692.
- Lefèvre S, Njamkepo E, Feldman S, Ruckly C, Carle I, Lejay-Collin M, et al. Rapid emergence of extensively drug-resistant Shigella sonnei in France. Nat Commun. 2023;14(1):462.
- Qu M, Zhang X, Liu G, Huang Y, Jia L, Liang W, et al. An eight-year study of Shigella species in Beijing, China: serodiversity, virulence genes, and antimicrobial resistance. J Infect Developing Countries. 2014;8(7):904–908.
- Vinh H, Baker S, Campbell J, et al. Rapid emergence of third generation cephalosporin resistant Shigella spp. In southern Vietnam. J Med Microbiol. 2009;58(2):281–283.
- Nuzhat S, Das R, Das S, et al. Antimicrobial resistance in shigellosis: a surveillance study among urban and rural children over 20 years in Bangladesh. PLoS One. 2022;17(11):e0277574.
- Sousa MÂB, Mendes EN, Collares GB, et al. Shigella in Brazilian children with acute diarrhoea: prevalence, antimicrobial resistance and virulence genes. Mem Inst Oswaldo Cruz. 2013;108(1):108 30–35. doi: 10.1590/S0074-02762013000100005
- Das A, Natarajan M, Mandal J, et al. The emergence of quinolone resistant Shigella sonnei, Pondicherry, India. PLoS One. 2016;11(8):e0160290. doi: 10.1371/journal.pone.0160290
- Moez-Ardalan K, Zali MR, Dallal MM, et al. Prevalence and pattern of antimicrobial resistance of Shigella species among patients with acute diarrhea in Karaj Tehran. Iran J Health Popul Nutr. 2003;21:96–102.
- Ram PK, Crump JA, Gupta SK, Miller MA, Mintz EP II. Part II. Analysis of data gaps pertaining to Shigella infections in low and medium human development index countries, 1984–2005. Epidemiol Infect. 2008;136(5):577–603. doi: 10.1017/S0950268807009351
- Wang H, Edwards M, Falkinham IJ, et al. Molecular survey of the occurrence of legionella spp., mycobacterium spp., Pseudomonas aeruginosa, and amoeba hosts in two chloraminated drinking water distribution systems. Appl Environ Microbiol. 2012;78(17):6285–6294. doi: 10.1128/AEM.01492-12
- Shi Y, Queller DC, Tian Y, Zhang S, Yan Q, He Z, Liu S-J, et al. The ecology and evolution of amoeba-bacterium interactions. Appl environ microbiol. 2021;87(2):1866.
- Rowbotham TJ. Preliminary report on the pathogenicity of legionella pneumophila for freshwater and soil amoebae. J Clin Pathol. 1980;33(12):1179–1183. doi: 10.1136/jcp.33.12.1179
- Hoffmann C, Harrison CF, Hilbi H. The natural alternative: protozoa as cellular models for L egionella infection. Cell Microbiol. 2014;16(1):15–26. doi: 10.1111/cmi.12235
- Strassmann JE, Shu L. Ancient bacteria–amoeba relationships and pathogenic animal bacteria. PLoS Biol. 2017;15(5):e2002460. doi: 10.1371/journal.pbio.2002460
- Watson J, Jenkins C, Clements A, Schottel JL. Shigella sonnei does not use amoebae as protective hosts. Appl environ microbiol. 2018;84(9):2679. doi: 10.1128/AEM.02679-17
- Shepherd JG, Wang L, Reeves PR, et al. Comparison of O-antigen gene clusters of Escherichia coli (Shigella) sonnei and Plesiomonas shigelloides O17: sonnei gained its current plasmid-borne O-antigen genes from P. shigelloides in a recent event. Infect Immun. 2000;68(10):6056–6061. doi: 10.1128/IAI.68.10.6056-6061.2000
- Ascari A, Tran ENH, Eijkelkamp BA, Morona R, Comstock LE. Identification of the Shigella flexneri Wzy domain modulating WzzpHS-2 interaction and detection of the Wzy/Wzz/Oag complex. J Bacteriol. 2022;204(9):224. doi: 10.1128/jb.00224-22
- Sack DA, Hoque S, Etheridge M, Huq A. Is protection against shigellosis induced by natural infection with Plesiomonas shigelloides? Lancet. 1994;343(8910):1413–1415. doi: 10.1016/S0140-6736(94)92531-3
- Jiang Y, Yang F, Zhang X, et al. The complete sequence and analysis of the large virulence plasmid pSS of Shigella sonnei. Plasmid. 2005;54(2):149–159.
- Van De Verg L,L, Herrington DA, Boslego J, et al. Age-specific prevalence of serum antibodies to the invasion plasmid and lipopolysaccharide antigens of Shigella species in Chilean and North American populations. J Infect Dis. 1992;166(1):158–161. doi: 10.1093/infdis/166.1.158
- Pupo GM, Lan R, Reeves PR. Multiple independent origins of Shigella clones of Escherichia coli and convergent evolution of many of their characteristics. Proc Natl Acad Sci, USA. 2000;97(19):10567–10572. doi: 10.1073/pnas.180094797
- Sahl JW, Morris CR, Emberger J, et al. Defining the phylogenomics of Shigella species: a pathway to diagnostics. J Clin Microbiol. 2015;53(3):951–960.
- Yang F, Yang J, Zhang X, et al. Genome dynamics and diversity of Shigella species, the etiologic agents of bacillary dysentery. Nucleic Acids Res. 2005;33(19):6445–6458.
- Feng Y, Chen Z, Liu S, et al. Gene decay in Shigella as an incipient stage of host-adaptation. PLoS One. 2011;6(11):e27754. doi: 10.1371/journal.pone.0027754
- Cole ST, Eiglmeier K, Parkhill J, James KD, Thomson NR, Wheeler PR, et al. Massive gene decay in the leprosy bacillus. Nature. 2001;409(6823):1007–1011.
- Parkhill J, Sebaihia M, Preston A, Murphy LD, Thomson N, Harris DE, et al. Comparative analysis of the genome sequences of Bordetella pertussis, Bordetella parapertussis and Bordetella bronchiseptica. Nature Genet. 2003;35(1):32–40.
- Holt KE, Parkhill J, Mazzoni CJ, Roumagnac P, Weill F, Goodhead I, et al. High-throughput sequencing provides insights into genome variation and evolution in Salmonella Typhi. Nature Genet. 2008;40(8):987–993.
- Sentausa E, Basso P, Berry A, et al. Insertion sequences drive the emergence of a highly adapted human pathogen. Microb Genom. 2020;6(9). doi: 10.1099/mgen.0.000265
- Wagner A, De la Chaux N. Distant horizontal gene transfer is rare for multiple families of prokaryotic insertion sequences. Mol Genet Genomic. 2008;280(5):280 397–408. doi: 10.1007/s00438-008-0373-y
- Hawkey J, Monk JM, Billman-Jacobe H, et al. Impact of insertion sequences on convergent evolution of Shigella species. PLoS Genet. 2020;16(7):e1008931. doi: 10.1371/journal.pgen.1008931
- Ito H, Kido N, Arakawa Y, Ohta M, Sugiyama T, Kato N. Possible mechanisms underlying the slow lactose fermentation phenotype in Shigella spp. Appl environ microbiol. 1991;57(10):2912–2917. doi: 10.1128/aem.57.10.2912-2917.1991
- Rezwan F, Lan R, Reeves PR. Molecular basis of the indole-negative reaction in Shigella strains: extensive damages to the tna operon by insertion sequences. J Bacteriol. 2004;186(21):7460–7465. doi: 10.1128/JB.186.21.7460-7465.2004
- Day W,A Jr, Fernández R,E, Maurelli AT, et al. Pathoadaptive mutations that enhance virulence: genetic organization of the cadA regions of Shigella spp. Infect Immun. 2001;69(12):7471–7480. doi: 10.1128/IAI.69.12.7471-7480.2001
- Bliven KA, Maurelli AT, Andrews-Polymenis HL. Antivirulence genes: insights into pathogen evolution through gene loss. Infect Immun. 2012;80(12):4061–4070. doi: 10.1128/IAI.00740-12
- Prosseda G, Di Martino ML, Campilongo R, et al. Shedding of genes that interfere with the pathogenic lifestyle: the Shigella model. Res Microbiol. 2012;163(6–7):399–406. doi: 10.1016/j.resmic.2012.07.004
- Chung the H, Boinett C, Pham Thanh D, Jenkins C, Weill F, Howden BP, et al. Dissecting the molecular evolution of fluoroquinolone-resistant Shigella sonnei. Nat Commun. 2019;10(1):4828.
- Venkatesan MM, Goldberg MB, Rose DJ, et al. Complete DNA sequence and analysis of the large virulence plasmid of Shigella flexneri. Infect Immun. 2001;69(5):3271–3285. doi: 10.1128/iai.69.5.3271-3285.2001
- Ruiting L, Brad L, David R, et al. Molecular Evolution of Large Virulence Plasmid inShigella Clones and EnteroinvasiveEscherichia coli. Infect Immun. 2001;69(10):6303–6309. doi: 10.1128/iai.69.10.6303-6309.2001
- Makino S, Sasakawa C, Yoshikawa M. Genetic relatedness of the basic replicon of the virulence plasmid in shigellae and enteroinvasive Escherichia coli. Microbial Pathogenesis. 1988;5(4):267–274. doi: 10.1016/0882-4010(88)90099-X
- Lan R, Alles MC, Donohoe K, Martinez MB, Reeves PR. Molecular evolutionary relationships of enteroinvasive Escherichia coli and Shigella spp. Infect Immun. 2004;72(9):5080–5088. doi: 10.1128/IAI.72.9.5080-5088.2004
- Martyn JE, Pilla G, Hollingshead S, Winther KS, Lea S, McVicker G, Comstock LE, et al. Maintenance of the Shigella sonnei virulence plasmid is dependent on its repertoire and amino acid sequence of toxin-antitoxin systems. J Bacteriol. 2022;204(3):519.
- McVicker G, Tang CM. Deletion of toxin–antitoxin systems in the evolution of Shigella sonnei as a host-adapted pathogen. Nat Microbiol. 2016;2(2):1–8. doi: 10.1038/nmicrobiol.2016.204
- Baker KS, Campos J, Pichel M, Della Gaspera A, Duarte-Martínez F, Campos-Chacón E, et al. Whole genome sequencing of Shigella sonnei through PulseNet Latin America and Caribbean: advancing global surveillance of foodborne illnesses. Clin Microbiol Infect. 2017;23(11):845–853. doi: 10.1016/j.cmi.2017.03.021
- Hawkey J, Paranagama K, Baker KS, Bengtsson RJ, Weill F, Thomson NR, et al. Global population structure and genotyping framework for genomic surveillance of the major dysentery pathogen, Shigella sonnei. Nat Commun. 2021;12(1):2684.
- Pakbin B, Didban A, Brück W,M, et al. Phylogenetic analysis and antibiotic resistance of Shigella sonnei isolates. FEMS Microbiol Lett. 2022;369(1):fnac042. doi: 10.1093/femsle/fnac042
- DuPont HL, Levine MM, Hornick RB, Formal SB. Inoculum size in shigellosis and implications for expected mode of transmission. J Infect Dis. 1989;159(6):1126–1128. http://www.jstor.org/stable/30137443
- ALAMANOS Y, MAIPA V, LEVIDIOTOU S, GESSOULI E. A community waterborne outbreak of gastro-enteritis attributed to Shigella sonnei. Epidemiol Infect. 2000;125(3):499–503. doi: 10.1017/S0950268800004866
- Vanden Esschert K, L Mattioli, Hilborn ED, et al. Outbreaks associated with untreated recreational water — California, Maine, and Minnesota, 2018–2019. MMWR Morb Mortal Wkly Rep. 2020;69(25):781–783.
- Qiu S, Liu K, Yang C, Xiang Y, Min K, Zhu K, et al. A Shigella sonnei clone with extensive drug resistance associated with waterborne outbreaks in China. Nat Commun. 2022;13(1):7365.
- Smith JL. Shigella as a food borne pathogen. J Food Prot. 1987;50(9):788–801. doi: 10.4315/0362-028X-50.9.788
- NYGREN BL, SCHILLING KA, BLANTON EM, SILK BJ, COLE DJ, MINTZ ED. Foodborne outbreaks of shigellosis in the USA, 1998–2008. Epidemiol Infect. 2013;141(2):233–241. doi: 10.1017/S0950268812000222
- Lee LA, Ostroff SM, McGee HB, et al. An outbreak of shigellosis at an outdoor music festival. Am J Epidemiol. 1991;133(6):608–615.
- Farag TH, Faruque AS, Wu Y, Das SK, Hossain A, Ahmed S, Kosek M, et al. Housefly population density correlates with shigellosis among children in Mirzapur, Bangladesh: a time series analysis. PLoS negl trop dis. 2013;7(6):e2280.
- Simms I, Field N, Jenkins C, Childs T, Gilbart VL, Dallman TJ, et al. Intensified shigellosis epidemic associated with sexual transmission in men who have sex with men-Shigella flexneri and S. sonnei in England, 2004 to end of February 2015. Eurosurveillance. 2015;20(15). doi: 10.2807/1560-7917.ES2015.20.15.21097
- Ingle DJ, Easton M, Valcanis M, Seemann T, Kwong JC, Stephens N, et al. Co-circulation of multidrug-resistant Shigella among men who have sex with men in Australia. Clinl Infect Dis. 2019;69(9):1535–1544.
- Khan WA, Griffiths JK, Bennish ML, et al. Gastrointestinal and extra-intestinal manifestations of childhood shigellosis in a region where all four species of Shigella are endemic. PLoS One. 2013;8(5):e64097. doi: 10.1371/journal.pone.0064097
- Hamer DH, Gill CJ, Chilengi R. Intestinal infections: overview. In: Quah SRBT ed. International Encyclopedia of Public Health. 2nd ed. Oxford:Academic Press;2017. pp. 322–335
- Thompson CN, Thieu NTV, Vinh PV, Duc AN, Wolbers M, Vinh H, et al. Clinical implications of reduced susceptibility to fluoroquinolones in paediatric Shigella sonnei and Shigella flexneri infections. J Antimicrob Chemother. 2016;71(3):807–815.
- Tansarli GS, Long DR, Waalkes A, Bourassa LA, Libby SJ, Penewit K, et al. Genomic reconstruction and directed interventions in a multidrug-resistant shigellosis outbreak in Seattle, WA, USA: a genomic surveillance study. Lancet Infect Dis. 2023;23(6):740–750.
- Levine MM, DuPont HL, Formal SB, Hornick RB, Takeuchi A, Gangarosa EJ, et al. Pathogenesis of Shigella dysenteriae 1 (Shiga) dysentery. J Infect Dis. 1973;127(3):261–270.
- Ghosh S, Pazhani GP, Niyogi SK, et al. Genetic characterization of Shigella spp. isolated from diarrhoeal and asymptomatic children. J Med Microbiol. 2014;63(7):903–910. doi: 10.1099/jmm.0.070912-0
- Khalil I, Troeger CE, Blacker BF, Reiner RC. Capturing the true burden of Shigella and ETEC: the way forward. Vaccine. 2019;37(34):4784–4786. doi: 10.1016/j.vaccine.2019.01.031
- Kotloff KL. Bacterial diarrhoea. Curr Opin Pediatr. 2022;34(2):147–155. doi: 10.1097/MOP.0000000000001107
- Afroze F, Ahmed T, Sarmin M, Shahid SA, Shahunja KM, Shahrin L, et al. Risk factors and outcome of Shigella encephalopathy in Bangladeshi children. PLoS neglected tropical diseases. 2017; 11 (4): e0005561.
- Grondin C, Imbert P, Ficko C, et al. Shigella flexneri Bacteremia in Two Immune‐Competent Adult Travelers. J Travel Med. 2012;19(4):258–260.
- Miti S, Chilyabanyama ON, Chisenga CC, et al. Sensitivity and predictive value of dysentery in diagnosing shigellosis among under five children in Zambia. PLoS One. 2023;18(2):e0279012.
- Liu J, Platts-Mills J, Juma J, Kabir F, Nkeze J, Okoi C, et al. Use of quantitative molecular diagnostic methods to identify causes of diarrhoea in children: a reanalysis of the GEMS case-control study. Lancet. 2016;388(10051):1291–1301. doi: 10.1016/S0140-6736(16)31529-X
- Tickell KD, Brander RL, Atlas HE, Pernica JM, Walson JL, Pavlinac PB. Identification and management of Shigella infection in children with diarrhoea: a systematic review and meta-analysis. Lancet Glob Health. 2017;5(12):e1235–e1248. doi: 10.1016/S2214-109X(17)30392-3
- Platts-Mills J, Houpt ER, Liu J, et al. Etiology and incidence of moderate-to-severe diarrhea in Young children in Niger. J Pediatric Infect Dis Soc. 2021;10(12):1062–1070. doi: 10.1093/jpids/piab080
- Mohle-Boetani J, Stapleton M, Finger R, Bean NH, Poundstone J, Blake PA, et al. Communitywide shigellosis: control of an outbreak and risk factors in child day-care centers. Am J Public Health. 1995;85(6):812–816. doi: 10.2105/AJPH.85.6.812
- Arvelo W, Hinkle CJ, Nguyen TA, Weiser T, Steinmuller N, Khan F, et al. Transmission risk factors and treatment of pediatric shigellosis during a large daycare center-associated outbreak of multidrug resistant Shigella sonnei: implications for the management of shigellosis outbreaks among children. Pediatr Infect Dis J. 2009;28(11):976–980. doi: 10.1097/INF.0b013e3181a76eab
- Scallan E, Hoekstra RM, Angulo FJ, Tauxe RV, Widdowson M, Roy SL, et al. Foodborne illness acquired in the United States—major pathogens. Emerg Infect Dis. 2011;17(1):7–15.
- Cohen D, Korin H, Bassal R, Perry Markovich M, Sivan Y, Goren S, et al. Burden and risk factors of Shigella sonnei shigellosis among children aged 0–59 months in hyperendemic communities in Israel. Inter J Infect Dis. 2019;82:117–123. doi: 10.1016/j.ijid.2019.02.031
- Siddiq M, O’Flanagan H, Richardson D, et al. Factors associated with sexually transmitted shigella in men who have sex with men: a systematic review. sexually transmitted infections. Sex Transm Infect. 2023;99(1):58 LP–63. doi: 10.1136/sextrans-2022-055583
- Zayet S, Klopfenstein T, Pierron A, et al. Shigella sonnei, an emerging multidrug-resistant sexually transmitted pathogen in Franche-Comté, France. Emerg Microbes Infect. 2021;10(1):1702–1705.
- Fischer N, Maex M, Mattheus W, Van den Bossche A, Van Cauteren D, Laisnez V, et al. Genomic epidemiology of persistently circulating MDR Shigella sonnei strains associated with men who have sex with men (MSM) in Belgium (2013–19). J Antimicrob Chemother. 2021;77(1):89–97. doi: 10.1093/JAC/DKAB377
- Braam JF, Bruisten SM, Hoogeland M, et al. Shigella is common in symptomatic and asymptomatic men who have sex with men visiting a sexual health clinic in Amsterdam. sexually transmitted infections. Sex Transm Infect. 2022;98(8):564 LP–569. doi: 10.1136/sextrans-2021-055274
- Moreno-Mingorance A, Mir-Cros A, Goterris L, Rodriguez-Garrido V, Sulleiro E, Barberà MJ, et al. Increasing trend of antimicrobial resistance in Shigella associated with MSM transmission in Barcelona, 2020–21: outbreak of XRD Shigella sonnei and dissemination of ESBL-producing Shigella flexneri. J Antimicrob Chemother. 2023;78(4):975–982.
- Mitchell HD, Whitlock G, Zdravkov J, Olsson J, Silalang P, Bardsley M, et al. Prevalence and risk factors of bacterial enteric pathogens in men who have sex with men: a cross-sectional study at the UK’s largest sexual health service. J Infect. 2023;86(1):33–40. doi: 10.1016/j.jinf.2022.10.033
- Gaufin T, Blumenthal J, Ramirez-Sanchez C, et al. Antimicrobial-Resistant Shigella spp. in San Diego, California, USA, 2017–2020. Emerg Infect Dis. 2022;28(6):1110–1116.
- UK Health Security Agency. Sexually transmitted Shigella spp. In England – data up to quarter 2. 2022. https://www.gov.uk/government/publications/non-travel-associated-shigella-infections/sexually-transmitted-shigella-spp-in-england-data-up-to-quarter-2-2022.
- Allen H, Mitchell HD, Simms I, et al. Evidence for re-infection and persistent carriage of Shigella species in adult males reporting domestically acquired infection in England. Clin Microbiol Infect. 2021;27(1):.e126.7–.e126.13. doi: 10.1016/j.cmi.2020.03.036
- Stefanovic A, Matic N, Ritchie G, et al. Multidrug resistant Shigella sonnei bacteremia among persons experiencing homelessness, Vancouver, British Columbia, Canada. Emerg Infect Dis. 2023;29(8):1668.
- Hines JZ. Notes from the field: shigellosis outbreak among men who have sex with men and homeless persons—Oregon, 2015–2016. MMWR Morbidity And Mortality Weekly Report. 2016;65.
- Centers for Disease Control and Prevention, (CDC). Shigellosis among people experiencing homelessness. https://www.cdc.gov/shigella/specific_groups/people-experiencing-homelessness.html.
- Hines JZ, Jagger MA, Jeanne TL, West N, Winquist A, Robinson BF, et al. Heavy precipitation as a risk factor for shigellosis among homeless persons during an outbreak — Oregon, 2015–2016. J Infect. 2018;76(3):280–285. doi: 10.1016/j.jinf.2017.11.010
- Kozyreva VK, Jospin G, Greninger AL, et al. Recent outbreaks of shigellosis in California caused by two distinct populations of Shigella sonnei with either increased virulence or fluoroquinolone resistance. mSphere. 2016;1(6):00344–16. doi: 10.1128/mSphere.00344-16
- Murti M, Louie K, Bigham M, et al. Outbreak of shigellosis in a homeless shelter with healthcare worker transmission—British Columbia, April 2015. Infect Control Hosp Epidemiol. 2015;36(11):1372–1373.
- Riddle MS, Chen WH, Kirkwood CD, MacLennan CA. Update on vaccines for enteric pathogens. Clin Microbiol Infect. 2018;24(10):1039–1045. doi: 10.1016/j.cmi.2018.06.023
- Adler AV, Ciccotti HR, Trivitt SJH, et al. What’s new in travellers’ diarrhoea: updates on epidemiology, diagnostics, treatment and long-term consequences. J Travel Med. 2022;29(1):taab099. doi: 10.1093/jtm/taab099
- Cohen D, Sela T, Slepon R, et al. Prospective cohort studies of shigellosis during military field training. Eur J Clin Microbiol Infect Dis. 2001;20(2):123–126. doi: 10.1007/s100960000428
- Porter CK, Olson S, Hall A, et al. Travelers’ diarrhea: an update on the incidence, Etiology, and risk in military deployments and similar travel populations. Mil Med. 2017;182(suppl_2):4–10. doi: 10.7205/MILMED-D-17-00064
- Chandani Y, Ghosh V, Suresh V, et al. An outbreak of shigellosis in European travellers returning from Cape Verde. New Microbes New Infect. 2023;52:101091. doi: 10.1016/j.nmni.2023.101091
- Bowen A, Hurd J, Hoover C, et al. Importation and domestic transmission of Shigella sonnei resistant to ciprofloxacin—United States, May 2014–February 2015. Morbidity Mortality Weekly Rep. 2015;64(12):318.
- Medeiro QP, Ledwaba SE, Bolick DT, et al. A murine model of diarrhea, growth impairment and metabolic disturbances with Shigella flexneri infection and the role of zinc deficiency. Gut Microbes. 2019;10(5):615–630.
- Sansonetti PJ, Arondel J, Cantey JR, et al. Infection of rabbit Peyer’s patches by Shigella flexneri: effect of adhesive or invasive bacterial phenotypes on follicle-associated epithelium. Infect Immun. 1996;64(7):2752–2764. doi: 10.1128/iai.64.7.2752-2764.1996
- Fernandez MI, Sansonetti PJ. Shigella interaction with intestinal epithelial cells determines the innate immune response in shigellosis. Int J Med Microbiol. 2003;293(1):55–67. doi: 10.1078/1438-4221-00244
- Shim D, Suzuki T, Chang S, Park S, Sansonetti PJ, Sasakawa C, et al. New animal model of shigellosis in the Guinea Pig: its usefulness for protective efficacy studies. J Immunol. 2007;178(4):2476–2482. doi: 10.4049/JIMMUNOL.178.4.2476
- Barman S, Saha DR, Ramamurthy T, Koley H. Development of a new guinea-pig model of shigellosis. FEMS Immunol Med Microbiol. 2011;62(3):304–314. doi: 10.1111/j.1574-695X.2011.00810.x
- Yum LK, Byndloss MX, Feldman SH, Agaisse H. Critical role of bacterial dissemination in an infant rabbit model of bacillary dysentery. Nat Commun. 2019;10(1):1826. doi: 10.1038/s41467-019-09808-4
- Duggan GM, Mostowy S. Use of zebrafish to study Shigella infection. Dis Models Mech. 2018;11(2):dmm032151. doi: 10.1242/dmm.032151
- Torraca V, Mostowy S. Zebrafish infection: from pathogenesis to cell biology. Trends Cell Biol. 2018;28(2):143–156. doi: 10.1016/j.tcb.2017.10.002
- Torraca V, Kaforou M, Watson J, Duggan GM, Guerrero-Gutierrez H, Krokowski S, Mulvey MA, et al. Shigella sonnei infection of zebrafish reveals that O-antigen mediates neutrophil tolerance and dysentery incidence. PLOS Pathogens. 2019;15(12):e1008006.
- Koestler BJ, Ward CM, Fisher CR, Rajan A, Maresso AW, Payne SM, Young VB. Human intestinal enteroids as a model system of Shigella pathogenesis. Infect Immun. 2019;87(4). doi: 10.1128/IAI.00733-18
- Hu Y, Huang H, Cheng X, et al. A global survey of bacterial type III secretion systems and their effectors. Environ Microbiol. 2017;19(10):3879–3895.
- Bajunaid W, Haidar-Ahmad N, Kottarampatel AH, et al. The T3SS of Shigella: expression, structure, function, and role in vacuole escape. Microorganisms. 2020;8(12):1933.
- Sansonetti PJ, Kopecko DJ, Formal SB. Involvement of a plasmid in the invasive ability of Shigella flexneri. Infect Immun. 1982;35(3):852–860. doi: 10.1128/iai.35.3.852-860.1982
- Muthuramalingam M, Whittier SK, Picking WL, et al. The Shigella type III secretion system: an overview from top to bottom. Microorganisms. 2021;9(2). doi: 10.3390/microorganisms9020451
- Blocker A, Gounon P, Larquet E, Niebuhr K, Cabiaux V, Parsot C, et al. The tripartite type III secreton of Shigella flexneri inserts IpaB and IpaC into host membranes. J Cell Bio. 1999;147(3):683–693.
- Picking WL, Nishioka H, Hearn PD, Baxter MA, Harrington AT, Blocker A, et al. IpaD of Shigella flexneri is independently required for regulation of ipa protein secretion and efficient insertion of IpaB and IpaC into host membranes. Infect Immun. 2005;73(3):1432–1440.
- Dickenson NE, Zhang L, Epler CR, Adam PR, Picking WL, Picking WD. Conformational changes in IpaD from Shigella flexneri upon binding bile salts provide insight into the second step of type III secretion. Biochemistry. 2011;50(2):172–180. doi: 10.1021/bi101365f
- Weatherspoon-Griffin N, Picker MA, Wing HJ. The genetic organization and transcriptional regulation of Shigella virulence genes. Mol Cel Biol Wd Picking & WL Picking (Eds) UK: caister Academic Press. 2016;65–107.
- Tobe T, Yoshikawa M, Mizuno T, Sasakawa C. Transcriptional control of the invasion regulatory gene virB of Shigella flexneri: activation by virF and repression by H-NS. J Bacteriol. 1993;175(19):6142–6149. doi: 10.1128/jb.175.19.6142-6149.1993
- Falconi M, Colonna B, Prosseda G, et al. Thermoregulation of Shigella and Escherichia coli EIEC pathogenicity. A temperature-dependent structural transition of DNA modulates accessibility of virF promoter to transcriptional repressor H-NS. EMBO J. 1998;17(23):7033–7043.
- Falconi M, Prosseda G, Giangrossi M, et al. Involvement of FIS in the H‐NS‐mediated regulation of virF gene of Shigella and enteroinvasive Escherichia coli. Mol Microbiol. 2001;42(2):439–452. doi: 10.1046/j.1365-2958.2001.02646.x
- Tran CN, Giangrossi M, Prosseda G, Brandi A, Di Martino ML, Colonna B, et al. A multifactor regulatory circuit involving H-NS, VirF and an antisense RNA modulates transcription of the virulence gene icsA of Shigella flexneri. Nucleic Acids Res. 2011;39(18):8122–8134.
- Wing HJ, Yan AW, Goldman SR, Goldberg MB. Regulation of IcsP, the outer membrane protease of the Shigella actin tail assembly protein IcsA, by virulence plasmid regulators VirF and VirB. J Bacteriol. 2004;186(3):699–705. doi: 10.1128/JB.186.3.699-705.2004
- Gall TL, Mavris M, Martino MC, Bernardini ML, Denamur E, Parsot C. Analysis of virulence plasmid gene expression defines three classes of effectors in the type III secretion system of Shigella flexneri. Microbiology. 2005;151(3):951–962. doi: 10.1099/mic.0.27639-0
- Hall CP, Jadeja NB, Sebeck N, et al. Characterization of MxiE-and H-NS-Dependent expression of ipaH7. 8, ospC1, yccE, and yfdF in Shigella flexneri. mSphere. 2022;7(6):485. doi: 10.1128/msphere.00485-22
- Simonian HP, Vo L, Doma S, Fisher RS, Parkman HP. Regional postprandial differences in pH within the stomach and gastroesophageal junction. Dig Dis Sci. 2005;50(12):50 2276–2285. doi: 10.1007/s10620-005-3048-0
- Evans DF, Pye G, Bramley R, et al. Measurement of gastrointestinal pH profiles in normal ambulant human subjects. Gut. 1988;29(8):1035–1041. doi: 10.1136/gut.29.8.1035
- Gorden J, Small P. Acid resistance in enteric bacteria. Infect Immun. 1993;61(1):364–367.
- Waterman SR, Small PLC. Identification of sigma S-dependent genes associated with the stationary-phase acid-resistance phenotype of Shigella flexneri. Mol Microbiol. 1996;21(5):925–940. doi: 10.1046/j.1365-2958.1996.00058.x
- Chiang I, Wang Y, Fujii S, et al. Biofilm formation and virulence of Shigella flexneri are modulated by pH of gastrointestinal tract. Infect Immun. 2021;89(11):387.
- Lin J, Lee IS, Frey J, Slonczewski JL, Foster JW. Comparative analysis of extreme acid survival in Salmonella typhimurium, Shigella flexneri, and Escherichia coli. J Bacteriol. 1995;177(14):4097–4104. doi: 10.1128/jb.177.14.4097-4104.1995
- Gajiwala KS, Burley SK. HDEA, a periplasmic protein that supports acid resistance in pathogenic enteric bacteria 1 1Edited by P. E. Wright. J Mol Biol. 2000;295(3):605–612. doi: 10.1006/jmbi.1999.3347
- Yang G, Wang L, Wang Y, et al. Hfq regulates acid tolerance and virulence by responding to acid stress in Shigella flexneri. Res Microbiol. 2015;166(6):476–485.
- Zhang B, Ran L, Wu M, et al. Shigella flexneri regulator SlyA controls bacterial acid resistance by directly activating the glutamate decarboxylation system. Front Microbiol. 2018;9:2071. doi: 10.3389/fmicb.2018.02071
- Mitobe J, Morita-Ishihara T, Ishihama A, et al. Involvement of RNA-binding protein Hfq in the osmotic-response regulation of invE gene expression in Shigella sonnei. BMC Microbiol. 2009;9(1):1–13. doi: 10.1186/1471-2180-9-110
- Wang Y, Teng Y, Geng J, et al. Involvement of RNA chaperone Hfq in the regulation of antibiotic resistance and virulence in Shigella sonnei. Res Microbiol. 2023;174(5):104047.
- Begley M, Gahan CGM, Hill C. The interaction between bacteria and bile. FEMS Microbiol Rev. 2005;29(4):625–651. doi: 10.1016/j.femsre.2004.09.003
- Nickerson KP, Chanin RB, Sistrunk JR, Rasko DA, Fink PJ, Barry EM, McCormick B, et al. Analysis of Shigella flexneri resistance, biofilm formation, and transcriptional profile in response to bile salts. Infect Immun. 2017;85(6):1067.
- Faherty CS, Redman JC, Rasko DA, et al. Shigella flexneri effectors OspE1 and OspE2 mediate induced adherence to the colonic epithelium following bile salts exposure. Mol Microbiol. 2012;85(1):107–121. doi: 10.1111/j.1365-2958.2012.08092.x
- Chanin RB, Nickerson KP, Llanos-Chea A, et al. Shigella flexneri adherence factor expression in in vivo-like conditions. mSphere. 2019;4(6) 10.1128/msphere.00751-19.
- Bernard AR, Jessop TC, Kumar P, Dickenson NE. Deoxycholate-enhanced Shigella virulence is regulated by a rare π-helix in the type three secretion system tip protein IpaD. Biochemistry. 2017;56(49):6503–6514. doi: 10.1021/acs.biochem.7b00836
- Zumsteg AB, Goosmann C, Brinkmann V, Morona R, Zychlinsky A. IcsA is a Shigella flexneri adhesin regulated by the type III secretion system and required for pathogenesis. Cell Host Microbe. 2014;15(4):435–445. doi: 10.1016/j.chom.2014.03.001
- Köseoğlu VK, Hall CP, Rodríguez-López EM, Agaisse H, Roy CR. The autotransporter IcsA promotes Shigella flexneri biofilm formation in the presence of bile salts. Infect Immun. 2019;87(7). doi: 10.1128/IAI.00861-18
- Mahmoud RY, Stones DH, Li W, et al. The multivalent adhesion molecule SSO1327 plays a key role in Shigella sonnei pathogenesis. Mol Microbiol. 2016;99(4):658–673.
- Bansil R, Turner BS. Mucin structure, aggregation, physiological functions and biomedical applications. Curr Opin Colloid Interface Sci. 2006;11(2–3):164–170. doi: 10.1016/j.cocis.2005.11.001
- Nutten S, Sansonetti P, Huet G, et al. Epithelial inflammation response induced by Shigella flexneri depends on mucin gene expression. Microbes Infect. 2002;4(11):1121–1124.
- Navarro-Garcia F, Gutierrez-Jimenez J, Garcia-Tovar C, et al. Pic, an autotransporter protein secreted by different pathogens in the Enterobacteriaceae family, is a potent mucus secretagogue. Infect Immun. 2010;78(10):4101–4109. doi: 10.1128/IAI.00523-10
- Prakash R, Bharathi Raja S, Devaraj H, et al. Up-regulation of MUC2 and IL-1β expression in human colonic epithelial cells by Shigella and its interaction with mucins. PLoS One. 2011;6(11):e27046. doi: 10.1371/journal.pone.0027046
- Ranganathan S, Doucet M, Grassel CL, Delaine-Elias B, Zachos NC, Barry EM, Young VB. Evaluating Shigella flexneri pathogenesis in the human enteroid model. Infect Immun. 2019;87(4):740. doi: 10.1128/IAI.00740-18
- Haider K, Hossain A, Wanke C, et al. Production of mucinase and neuraminidase and binding of Shigella to intestinal mucin. J Diarrhoeal Dis Res. 1993;11:88–92.
- Prizont R. Degradation of intestinal glycoproteins by pathogenic Shigella flexneri. Infect Immun. 1982;36(2):615–620. doi: 10.1128/iai.36.2.615-620.1982
- Henderson IR, Czeczulin J, Eslava C, Noriega F, Nataro JP, Orndorff PE. Characterization of Pic, a secreted protease of Shigella flexneri and enteroaggregative Escherichia coli. Infect Immun. 1999;67(11):5587–5596. doi: 10.1128/IAI.67.11.5587-5596.1999
- Marteyn B, West NP, Browning DF, Cole JA, Shaw JG, Palm F, et al. Modulation of Shigella virulence in response to available oxygen in vivo. Nature. 2010;465(7296):355–358.
- Nickerson KP, Llanos-Chea A, Ingano L, et al. A versatile human intestinal organoid-derived epithelial monolayer model for the study of enteric pathogens. Microbiol Spectr. 2021;9(1):3.
- Mounier J, Vasselon T, Hellio R, et al. Shigella flexneri enters human colonic caco-2 epithelial cells through the basolateral pole. Infect Immun. 1992;60(1):237–248. doi: 10.1128/iai.60.1.237-248.1992
- Rey C, Chang Y, Latour-Lambert P, Varet H, Proux C, Legendre R, Knodler L, et al. Transcytosis subversion by M cell-to-enterocyte spread promotes Shigella flexneri and Listeria monocytogenes intracellular bacterial dissemination. PLOS Pathogens. 2020;16(4):e1008446.
- Mabbott NA, Donaldson DS, Ohno H, et al. Microfold (M) cells: important immunosurveillance posts in the intestinal epithelium. Mucosal Immunol. 2013;6(4):666–677. doi: 10.1038/mi.2013.30
- Ashida H, Sasakawa C. Shigella hacks host immune responses by reprogramming the host epigenome. EMBO J. 2014;33(22):2598–2600. doi: 10.15252/embj.201489934
- High N, Mounier J, Prevost MC, Sansonetti PJ. IpaB of Shigella flexneri causes entry into epithelial cells and escape from the phagocytic vacuole. EMBO J. 1992;11(5):1991–1999. doi: 10.1002/j.1460-2075.1992.tb05253.x
- Zychlinsky A, Kenny B, Ménard R, et al. IpaB mediates macrophage apoptosis induced by Shigella flexneri. Mol Microbiol. 1994;11(4):619–627. doi: 10.1111/j.1365-2958.1994.tb00341.x
- Broz P, Monack DM. Molecular mechanisms of inflammasome activation during microbial infections. Immunol Rev. 2011;243(1):174–190. doi: 10.1111/j.1600-065X.2011.01041.x
- Broz P, Dixit VM. Inflammasomes: mechanism of assembly, regulation and signalling. Nat Rev Immunol. 2016;16(7):407–420. doi: 10.1038/nri.2016.58
- Ashida H, Mimuro H, Sasakawa C. Shigella manipulates host immune responses by delivering effector proteins with specific roles. Front Immunol. 2015;6:6 219. doi: 10.3389/fimmu.2015.00219
- Strowig T, Henao-Mejia J, Elinav E, Flavell R. Inflammasomes in health and disease. Nature. 2012;481(7381):278–286. doi: 10.1038/nature10759
- Kayagaki N, Stowe IB, Lee BL, O’Rourke K, Anderson K, Warming S, et al. Caspase-11 cleaves gasdermin D for non-canonical inflammasome signalling. Nature. 2015;526(7575):666–671.
- Shi J, Zhao Y, Wang K, Shi X, Wang Y, Huang H, et al. Cleavage of GSDMD by inflammatory caspases determines pyroptotic cell death. Nature. 2015;526(7575):660–665.
- Sborgi L, Rühl S, Mulvihill E, Pipercevic J, Heilig R, Stahlberg H, et al. GSDMD membrane pore formation constitutes the mechanism of pyroptotic cell death. EMBO J. 2016;35(16):1766–1778.
- Miao EA, Mao DP, Yudkovsky N, et al. Innate immune detection of the type III secretion apparatus through the NLRC4 inflammasome. Proc Nat Acad Sci. 2010;107(7):3076–3080.
- Yang J, Zhao Y, Shi J, et al. Human NAIP and mouse NAIP1 recognize bacterial type III secretion needle protein for inflammasome activation. Proc Natl Acad Sci, USA. 2013;110(35):14408–14413. doi: 10.1073/pnas.1306376110
- Senerovic L, Tsunoda SP, Goosmann C, Brinkmann V, Zychlinsky A, Meissner F, et al. Spontaneous formation of IpaB ion channels in host cell membranes reveals how Shigella induces pyroptosis in macrophages. Cell Death Dis. 2012;3(9):e384.
- Muñoz-Planillo R, Kuffa P, Martínez-Colón G, Smith BL, Rajendiran TM, Núñez G. K+ efflux is the common trigger of NLRP3 inflammasome activation by bacterial toxins and particulate matter. Immunity. 2013;38(6):1142–1153. doi: 10.1016/j.immuni.2013.05.016
- Tang D, Kang R, Berghe TV, et al. The molecular machinery of regulated cell death. Cell Res. 2019;29(5):347–364. doi: 10.1038/s41422-019-0164-5
- Guichon A, Zychlinsky A. Clinical isolates of Shigella species induce apoptosis in macrophages. J Infect Dis. 1997;175(2):470–473. doi: 10.1093/infdis/175.2.470
- Watson JL, Sanchez-Garrido J, Goddard PJ, et al. Shigella sonnei O-antigen inhibits internalization, vacuole escape, and inflammasome activation. MBio. 2019;10(6):2654.
- Raqib R, Ekberg C, Sharkar P, et al. Apoptosis in acute shigellosis is associated with increased production of Fas/Fas ligand, perforin, caspase-1, and caspase-3 but reduced production of bcl-2 and interleukin-2. Infect Immun. 2002;70(6):3199–3207.
- Weinrauch Y, Drujan D, Shapiro SD, Weiss J, Zychlinsky A. Neutrophil elastase targets virulence factors of enterobacteria. Nature. 2002;417(6884):91–94. doi: 10.1038/417091a
- Brinkmann V, Reichard U, Goosmann C, Fauler B, Uhlemann Y, Weiss DS, et al. Neutrophil extracellular traps kill bacteria. Science. 2004;303(5663):1532–1535.
- Mostowy S, Boucontet L, Mazon Moya M,J, et al. The zebrafish as a new model for the in vivo study of Shigella flexneri interaction with phagocytes and bacterial autophagy. PLOS Pathogens. 2013;9(9):e1003588.
- Sansonetti PJ, Van Nhieu GT, Égile C. Rupture of the intestinal epithelial barrier and mucosal invasion by Shigella flexneri. Clinl Infect Dis. 1999;28(3):466–475. doi: 10.1086/515150
- Mandic-Mulec I, Weiss J, Zychlinsky A. Shigella flexneri is trapped in polymorphonuclear leukocyte vacuoles and efficiently killed. Infect Immun. 1997;65(1):110–115. doi: 10.1128/iai.65.1.110-115.1997
- Schroeder GN, Hilbi H. Molecular pathogenesis of Shigella spp.: controlling host cell signaling, invasion, and death by type III secretion. Clinical Microbiology Reviews. 2008;21(1):134–156. doi: 10.1128/CMR.00032-07
- François M, Le Cabec V, Dupont M, Sansonetti PJ, Maridonneau-Parini I, Tuomanen EI. Induction of necrosis in human neutrophils by Shigella flexneri requires type III secretion, IpaB and IpaC invasins, and actin polymerization. Infect Immun. 2000;68(3):1289–1296. doi: 10.1128/IAI.68.3.1289-1296.2000
- Watarai M, Funato S, Sasakawa C. Interaction of ipa proteins of Shigella flexneri with alpha5beta1 integrin promotes entry of the bacteria into mammalian cells. J Exp Med. 1996;183(3):991–999. doi: 10.1084/jem.183.3.991
- Skoudy A, Mounier J, Aruffo A, et al. CD44 binds to the Shigella IpaB protein and participates in bacterial invasion of epithelial cells. Cell Microbiol. 2000;2(1):19–33.
- Lafont F, Van Nhieu GT, Hanada K, et al. Initial steps of Shigella infection depend on the cholesterol/sphingolipid raft-mediated CD44–IpaB interaction. EMBO J. 2002;21(17):4449–4457. doi: 10.1093/emboj/cdf457
- Köhler H, Rodrigues SP, McCormick BA. Shigella flexneri interactions with the basolateral membrane domain of polarized model intestinal epithelium: role of lipopolysaccharide in cell invasion and in activation of the mitogen-activated protein kinase ERK. Infect Immun. 2002;70(3):1150–1158. doi: 10.1128/IAI.70.3.1150-1158.2002
- Marteyn B, Gazi A, Sansonetti P. Shigella: a model of virulence regulation in vivo. Gut Microbes. 2012;3(2):104–120. doi: 10.4161/gmic.19325
- Dickenson NE, Choudhari SP, Adam PR, Kramer RM, Joshi SB, Middaugh CR, et al. Oligomeric states of the Shigella translocator protein IpaB provide structural insights into formation of the type III secretion translocon. Protein Sci. 2013;22(5):614–627.
- Russo BC, Stamm LM, Raaben M, et al. Intermediate filaments enable pathogen docking to trigger type 3 effector translocation. Nat Microbiol. 2016;1(4):16025.
- Van Nhieu GT, Caron E, Hall A, et al. IpaC induces actin polymerization and filopodia formation during Shigella entry into epithelial cells. EMBO J. 1999;18(12):3249–3262. doi: 10.1093/emboj/18.12.3249
- DeMali KA, Jue AL, Burridge K. IpaA targets β1 integrins and rho to promote actin cytoskeleton rearrangements necessary for Shigella entry*. J Biol Chem. 2006;281(51):39534–39541. doi: 10.1074/jbc.M605939200
- Mellouk N, Enninga J. Cytosolic access of intracellular bacterial pathogens: the Shigella paradigm. Front Cell Infect Microbiol. 2016;6:35. doi: 10.3389/fcimb.2016.00035
- Deretic V. Autophagy in inflammation, infection, and immunometabolism. Immunity. 2021;54(3):437–453. doi: 10.1016/j.immuni.2021.01.018
- Keestra-Gounder A, Tsolis RM. NOD1 and NOD2: beyond peptidoglycan sensing. Trends Immunol. 2017;38(10):758–767. doi: 10.1016/j.it.2017.07.004
- Ogawa M, Yoshimori T, Suzuki T, Sagara H, Mizushima N, Sasakawa C. Escape of intracellular Shigella from autophagy. Science. 2005;307(5710):727–731. doi: 10.1126/science.1106036
- Campbell-Valois F, Sachse M, Sansonetti PJ, et al. Escape of actively secreting Shigella flexneri from ATG8/LC3-positive vacuoles formed during cell-to-cell spread is facilitated by IcsB and VirA. MBio. 2015;6(3):10–1128. doi: 10.1128/mBio.02567-14
- Pieper R, Fisher CR, Suh M, et al. Analysis of the proteome of intracellular Shigella flexneri reveals pathways important for intracellular growth. Infect Immun. 2013;81(12):4635–4648.
- Egile C, Loisel TP, Laurent V, Li R, Pantaloni D, Sansonetti PJ, et al. Activation of the Cdc42 effector N-Wasp by the Shigella flexneri Icsa protein promotes actin nucleation by Arp2/3 complex and bacterial actin-based motility. J Cell Bio. 1999;146(6):1319–1332.
- Fukumatsu M, Ogawa M, Arakawa S, Suzuki M, Nakayama K, Shimizu S, et al. Shigella targets epithelial tricellular junctions and uses a noncanonical clathrin-dependent endocytic pathway to spread between cells. Cell Host Microbe. 2012;11(4):325–336.
- Weddle EA, Köseoğlu V,K, DeVasure BA, et al. The type three secretion system effector protein IpgB1 promotes Shigella flexneri cell-to-cell spread through double-membrane vacuole escape. PLOS Pathogens. 2022;18(2):e1010380. doi: 10.1371/journal.ppat.1010380
- Caboni M, Pedron T, Rossi O, Goulding D, Pickard D, Citiulo F, Koehler TM, et al. An O antigen capsule modulates bacterial pathogenesis in Shigella sonnei. PLOS Pathogens. 2015;11(3):e1004749.
- Ashida H, Suzuki T, Sasakawa C. Shigella infection and host cell death: a double-edged sword for the host and pathogen survival. Curr Opin Microbiol. 2021;59:1–7. doi: 10.1016/j.mib.2020.07.007
- Faherty CS, Merrell DS, Semino-Mora C, Dubois A, Ramaswamy AV, Maurelli AT. Microarray analysis of Shigella flexneri-infected epithelial cells identifies host factors important for apoptosis inhibition. BMC Genomics. 2010;11(1):1–18. doi: 10.1186/1471-2164-11-272
- Clark CS, Maurelli AT. Shigella flexneri inhibits staurosporine-induced apoptosis in epithelial cells. Infect Immun. 2007;75(5):2531–2539. doi: 10.1128/IAI.01866-06
- Pendaries C, Tronchère H, Arbibe L, et al. PtdIns (5) P activates the host cell PI3‐kinase/Akt pathway during Shigella flexneri infection. EMBO J. 2006;25(5):1024–1034.
- Bergounioux J, Elisee R, Prunier A, et al. Calpain activation by the Shigella flexneri effector VirA regulates key steps in the formation and life of the bacterium’s epithelial niche. Cell Host Microbe. 2012;11(3):240–252.
- Faherty CS, Maurelli AT. Spa15 of Shigella flexneri is secreted through the type III secretion system and prevents staurosporine-induced apoptosis. Infect Immun. 2009;77(12):5281–5290. doi: 10.1128/IAI.00800-09
- Carneiro LAM, Travassos LH, Soares F, Tattoli I, Magalhaes JG, Bozza MT, et al. Shigella induces mitochondrial dysfunction and cell death in nonmyleoid cells. Cell Host Microbe. 2009;5(2):123–136.
- Paciello I, Silipo A, Lembo-Fazio L, et al. Intracellular Shigella remodels its LPS to dampen the innate immune recognition and evade inflammasome activation. Proceedings of the National Academy of Sciences. 2013;110(46):E4345–E4354.
- Dong N, Zhu Y, Lu Q, Hu L, Zheng Y, Shao F. Structurally distinct bacterial TBC-like GAPs link arf GTPase to Rab1 inactivation to counteract host defenses. Cell. 2012;150(5):1029–1041. doi: 10.1016/j.cell.2012.06.050
- Kobayashi T, Ogawa M, Sanada T, Mimuro H, Kim M, Ashida H, et al. The Shigella OspC3 effector inhibits caspase-4, antagonizes inflammatory cell death, and promotes epithelial infection. Cell Host Microbe. 2013;13(5):570–583.
- Mounier J, Boncompain G, Senerovic L, Lagache T, Chrétien F, Perez F, et al. Shigella effector IpaB-induced cholesterol relocation disrupts the golgi complex and recycling network to inhibit host cell secretion. Cell Host Microbe. 2012;12(3):381–389.
- Burnaevskiy N, Fox TG, Plymire DA, Ertelt JM, Weigele BA, Selyunin AS, et al. Proteolytic elimination of N-myristoyl modifications by the Shigella virulence factor IpaJ. Nature. 2013;496(7443):106–109.
- Burnaevskiy N, Peng T, Reddick LE, Hang HC, Alto NM. Myristoylome profiling reveals a concerted mechanism of ARF GTPase deacylation by the bacterial protease IpaJ. Molecular Cell. 2015;58(1):110–122. doi: 10.1016/j.molcel.2015.01.040
- Krachler AM, Ham H, Orth K. Outer membrane adhesion factor multivalent adhesion molecule 7 initiates host cell binding during infection by Gram-negative pathogens. Proc Nat Acad Sci. 2011;108(28):11614–11619. doi: 10.1073/pnas.1102360108
- Pope LM, Reed KE, Payne SM. Increased protein secretion and adherence to HeLa cells by Shigella spp. following growth in the presence of bile salts. Infect Immun. 1995;63(9):3642–3648. doi: 10.1128/iai.63.9.3642-3648.1995
- Liu B, Knirel YA, Feng L, Perepelov AV, Senchenkova SN, Wang Q, et al. Structure and genetics of Shigella O antigens. FEMS Microbiol Rev. 2008;32(4):627–653.
- Whitfield C, Wear SS, Sande C. Assembly of bacterial capsular polysaccharides and exopolysaccharides. Annu Rev Microbiol. 2020;74(1):521–543. doi: 10.1146/annurev-micro-011420-075607
- Sandlin RC, Lampel KA, Keasler SP, et al. Avirulence of rough mutants of Shigella flexneri: requirement of O antigen for correct unipolar localization of IcsA in the bacterial outer membrane. Infect Immun. 1995;63(1):229–237. doi: 10.1128/iai.63.1.229-237.1995
- Hong M, Payne SM. Effect of mutations in Shigella flexneri chromosomal and plasmid‐encoded lipopolysaccharide genes on invasion and serum resistance. Mol Microbiol. 1997;24(4):779–791.
- West NP, Sansonetti P, Mounier J, Exley RM, Parsot C, Guadagnini S, et al. Optimization of virulence functions through glucosylation of Shigella LPS. Science. 2005;307(5713):1313–1317.
- Qin J, Li R, Raes J, Arumugam M, Burgdorf KS, Manichanh C, et al. A human gut microbial gene catalogue established by metagenomic sequencing. Nature. 2010;464(7285):59–65. doi: 10.1038/nature08821
- Sender R, Fuchs S, Milo R. Revised estimates for the number of human and bacteria cells in the body. PLoS Biol. 2016;14(8):e1002533. doi: 10.1371/journal.pbio.1002533
- Sana TG, Lugo KA, Monack DM. T6SS: the bacterial“fight club” in the host gut. PLOS Pathogens. 2017;13(6):e1006325.
- Abby SS, Cury J, Guglielmini J, Néron B, Touchon M, Rocha EPC. Identification of protein secretion systems in bacterial genomes. Sci Rep. 2016;6(1):23080. doi: 10.1038/srep23080
- Chen C, Yang X, Shen X. Confirmed and potential roles of bacterial T6SSs in the intestinal ecosystem. Front Microbiol. 2019;10:1484. doi: 10.3389/fmicb.2019.01484
- Anderson MC, Vonaesch P, Saffarian A, Marteyn BS, Sansonetti PJ. Shigella sonnei encodes a functional T6SS used for interbacterial competition and niche occupancy. Cell Host Microbe. 2017;21(6):769–776. doi: 10.1016/j.chom.2017.05.004
- Riley MA, Wertz JE. Bacteriocin diversity: ecological and evolutionary perspectives. Biochimie. 2002;84(5–6):357–364. doi: 10.1016/S0300-9084(02)01421-9
- Cascales E, Buchanan SK, Duché D, et al. Colicin biology. Microbiol Mol Biol Rev. 2007;71(1):158–229.
- De Silva PM, Bennett RJ, Kuhn L, et al. Escherichia Coli killing by epidemiologically successful sublineages of Shigella sonnei is mediated by Colicins. EBioMedicine. 2023;97:104822. doi: 10.2139/SSRN.4318406
- MacLennan CA, Grow S, Ma L, Steele AD. The Shigella vaccines pipeline. Vaccines. 2022;10(9):1376. doi: 10.3390/vaccines10091376
- World Health Organization. Guidelines for the control of shigellosis, including epidemics due to Shigella dysenteriae type 1. https://apps.who.int/iris/handle/10665/43252.
- Centers for Disease Control and Prevention. Shigella - shigellosis. Information for healthcare professionals. https://www.cdc.gov/shigella/audience-medical-professionals.html.
- Rashid H, Rahman M. Possible transfer of plasmid mediated third generation cephalosporin resistance between Escherichia coli and Shigella sonnei in the human gut. Infect Genet Evol. 2015;30:15–18. doi: 10.1016/j.meegid.2014.11.023
- Puzari M, Sharma M, Chetia P. Emergence of antibiotic resistant Shigella species: a matter of concern. J Infect Public Health. 2018;11(4):451–454. doi: 10.1016/j.jiph.2017.09.025
- Gaudreau C, Bernaquez I, Pilon PA, et al. Clinical and genomic investigation of an International ceftriaxone- and azithromycin-resistant Shigella sonnei cluster among men who have sex with men, Montréal, Canada 2017–2019. Microbiol Spectr. 2022;10(3):2337. doi: 10.1128/spectrum.02337-21
- Mason LCE, Greig DR, Cowley LA, Partridge SR, Martinez E, Blackwell GA, et al. The evolution and international spread of extensively drug resistant Shigella sonnei. Nat Commun. 2023;14(1):1983.
- Tacconelli E, Carrara E, Savoldi A, et al. Discovery, research, and development of new antibiotics: the WHO priority list of antibiotic-resistant bacteria and tuberculosis. Lancet Infect Dis. 2018;18(3):318–327. doi: 10.1016/S1473-3099(17)30753-3
- Centers for Disease Control and Prevention. 2019. AR Threats report. https://www.cdc.gov/drugresistance/Biggest-Threats.html.
- Thanh Duy P, Thi Nguyen TN, Vu Thuy D, et al. Commensal Escherichia coli are a reservoir for the transfer of XDR plasmids into epidemic fluoroquinolone-resistant Shigella sonnei. Nat Microbiol. 2020;5(2):256–264.
- Gu B, Qin T, Fan W, Bi R, Chen Y, Li Y, et al. Novel mutations in gyrA and parC among Shigella sonnei strains from Jiangsu Province of China, 2002-2011. Inter J Infect Dis. 2017;59:44–49. doi: 10.1016/j.ijid.2017.03.023
- Baker S, Scott TA. Antimicrobial-resistant Shigella: where do we go next? Nat Rev Microbiol. 2023;21(7):1–2. doi: 10.1038/s41579-023-00906-1
- Centers for Disease Control and Prevention, (CDC). Increase in extensively drug-resistant shigellosis in the United States. https://emergency.cdc.gov/han/2023/pdf/CDC_HAN_486.pdf