ABSTRACT
The Eaf6 protein, a conserved component of the NuA4 and NuA3 complexes in yeast and MOZ/MORF complexes in humans, plays crucial roles in transcriptional activation, gene regulation, and cell cycle control. Despite its significance in other organisms, the functional role of Eaf6 in entomopathogenic fungi (EPF) remained unexplored. Here, we investigate the function of BbEaf6, the Eaf6 homolog in the entomopathogenic fungus Beauveria bassiana. We demonstrate that BbEaf6 is predominantly localized in nuclei, similar to its counterpart in other fungi. Deletion of BbEaf6 resulted in delayed conidiation, reduced conidial yield, and altered conidial properties. Transcriptomic analysis revealed dysregulation of genes involved in asexual development and cell cycle progression in the ΔBbEaf6 mutant. Furthermore, the ΔBbEaf6 mutant exhibited decreased tolerance to various stresses, including ionic stress, cell wall perturbation, and DNA damage stress. Notably, the ΔBbEaf6 mutant displayed attenuated virulence in insect bioassays, accompanied by dysregulation of genes associated with cuticle penetration and haemocoel infection. Overall, our study elucidates the multifaceted role of BbEaf6 in stress response, development, and virulence in B. bassiana, providing valuable insights into the molecular mechanisms governing fungal pathogenesis and potential targets for pest management strategies.
Disclaimer
As a service to authors and researchers we are providing this version of an accepted manuscript (AM). Copyediting, typesetting, and review of the resulting proofs will be undertaken on this manuscript before final publication of the Version of Record (VoR). During production and pre-press, errors may be discovered which could affect the content, and all legal disclaimers that apply to the journal relate to these versions also.Introduction
Histone acetylation stands as one of the most prevalent histone posttranslational modifications (PTMs),which is mediated by histone acetyltransferases (HATs) [1]. Typically, HATs are capable of adding acetyl groups to specific histone residues, which results in chromatin decondensation and gene activing [2,3]. The NuA4 complex, a highly conserved histone acetyltransferase (HAT) complex spanning from yeast to humans, demonstrates a preference for acetylating histones H4 and H2A [4,5]. The NuA4 complex plays a pivotal role in various genomic processes, including ensuring chromosome stability, facilitating gene transcription, participating in DNA damage repair, and promoting cell cycle progression [6,7,8]. Beyond its established role in modifying histones, the NuA4 complex showcases remarkable versatility by acetylating over 250 non-histone substrates, allowing NuA4 to exert critical control over cellular metabolism, autophagy, and homeostasis [9,10]. Another similar histone acetyltransferase complex, called NuA3 HAT complex, mainly promotes acetylation of histone H3 lysine 14 (H3K14) and mediates transcription of a subset of downstream genes in yeast [11].
Eaf6 homologs are found across various organisms, serving as integral components of NuA4 and NuA3 complexes in yeast, as well as MOZ and MORF complexes in humans [12,13,14]. Notably, the deletion or mutation of yeast Eaf6 does not lead to lethal phenotypes, suggesting that this NuA4 subunit is not indispensable for yeast [15]. However, in NuA3 complex, yeast Eaf6 contributes to transcriptional activation and cell cycle regulation through facilitating H3 acetylation [11,16]. In humans, Eaf6 forms trimeric complexes with ING5 and BRPF, playing crucial roles in cell proliferation, disease development, and interactions with MOZ and MORF complexes [14,17]. In Arabidopsis thaliana, Eaf6 functions as a characteristic subunit of the NuA4 complex and is essential for chlorophyll biosynthesis and photosynthesis [18]. Moreover, the deletion of Eaf6 in Oryza sativa can lead to hybrid breakdown in intersubspecific rice crosses [19]. Additionally, Eaf6 homolog has been identified in the fungal pathogen Fusarium graminearum, which causes Fusarium head blight (FHB) or scab on wheat [20]. In this pathogen, FgEaf6 has been shown to positively regulate virulence, asexual/sexual reproduction, and conidial septation, the deletion of FgEaf6 significantly impairs the sporulation process and pathogenicity on wheat [20].
Despite its significance in other organisms, however, the functions of Eaf6 homologs in other fungi, particularly entomopathogenic fungi, remain largely unexplored. One such example is the insect pathogenic fungus, Beauveria bassiana, widely recognized as a fungal biological pest control agent globally [21]. In B. bassiana, the efficacy of biological control hinges on critical aspects such as conidial production, tolerance to various stresses, and pathogenicity against insect hosts [22,23]. With the the completion of genome sequencing of B. bassiana, a expanding number of related activators have been characterized, including asexual developmental activators, antioxidant enzymes, cuticle-degrading enzymes, etc. [24,25]. Moreover, in this fungus, we also investigated the global acetylation network previously, which not only emphasized the importance of lysine acetylation, but also provided more genetic targets to explore [26]. So far, in B. bassiana, five histone acetyltransferases have been characterized, comprising three GNAT superfamily members (Gcn5, Spt10, Elp3), one MYST superfamily member (Mst2), and one P300/CBP family member (Rtt109), showcasing diverse impacts on developmental and virulence pathways within the fungus [27,28,29,30,31]. Here, we elucidate that the B. bassiana NuA4 subunit Eaf6 is vital for fungal sporulation, stress responses, and pathogenicity. The deletion of BbEaf6 resulted in decreased conidiation, conidial resistance to muiti-stress and attenuated virulence upon insect hosts. Correspondingly, the comparative transcriptomic analyses also revealed a large array of related genes, including asexual developmental activators, cuticle-degrading enzymes and many different kinds of cellular transporters.
Materials and methods
Blast search and identification of Eaf6 homolog in B. bassiana
The BLAST research using the amino acid sequence of Saccharomyces cerevisiae Eaf6 protein (ScEaf6, NP_012615) was conducted to identify homologs in the genomic database of B. bassiana ARSEF 2860 (NCBI accession: NZ_ADAH00000000) [24], as well as in other representative fungi, including fungal pathogens of plants, insects, and humans (http://blast.ncbi.nlm. nih.gov/). Subsequently, the identified sequences were aligned using the SMART program (http://smart.embl-heidelberg.de) to facilitate structural comparison. Phylogenetic analysis was then conducted using MEGA7 software (http://www.megasoftware.net) to assess evolutionary relationships. Additionally, the molecular weight and isoelectric point (pI) of BbEaf6 were predicted using the ExPASy-Compute pI/Mw tool (https://web.expasy.org/compute_pi/).
Subcellular localization of BbEaf6 protein
The subcellular localization of the Eaf6 homolog in B. bassiana (BbEaf6) was investigated using a fusion protein approach. The coding region of BbEaf6 was amplified from wild type cDNA using specific primers (Eaf6-LC-F/R, see Table S1) and fused to the green fluorescent protein (GFP) at the C-terminus of BbEaf6. This fusion gene, BbEaf6:GFP, was then cloned into plasmid pAN52-bar, which confers resistance to phosphinothricin. The BbEaf6:GFP fusion construct was integrated into the genome of the wild type B. bassiana strain via blastospore transformation, following a protocol described elsewhere [32]. Transgenic colonies expressing the fusion protein were identified by their resistance to phosphinothricin (200 μg/ml). The localization of the fusion protein was visualized using confocal microscopy. For visualization, conidia were cultured in SDB (4% glucose, 1% peptone, and 1% yeast extract) at 25°C for 3 days with aeration at 150 rpm. Hyphal cells were then collected and counter-stained with DAPI (nuclei-specific dye, Sigma) for 30 minutes at room temperature before microscopic examination.
Construction of B. bassiana Eaf6 deleted and complemented strains
To generate a targeted gene deletion strain of BbEaf6 (ΔBbEaf6) in B. bassiana, homologous recombination was employed using 5‘and 3’ coding fragments (1509 and 1442 bp, respectively) flanking the bar selection marker followed a strategy previously described [27]. Subsequently, a complemented strain (ΔBbEaf6:BbEaf6) was constructed through ectopic integration of the full-length BbEaf6 sequence, along with approximately 1500 bp of the 5’-flanking (promoter) region (totaling 3528 bp), cloned into a vector containing the sur sulfonylurea resistance marker. DNA fragments required for these manipulations were amplified from B. bassiana genomic DNA using paired primers (Table S1) and LaTaq DNA polymerase. Putative ΔBbEaf6 mutant colonies were screened either by bar-mediated resistance to phosphinothricin (200 μg/ml) or by sur resistance to chlorimuron ethyl (10 μg/ml) for the ΔBbEaf6:BbEaf6 strain. Corrected integration events were verified through PCR and quantitative PCR (qPCR), employing the primers and procedures outlined in Table S1.
Investigation of fungal tolerance to multi-stresses
To evaluate responses to various stressors, 1 μl aliquots of 1×106 conidia/ml suspensions from each strain were spotted onto different agar media plates. The base medium used was Czapek Dox Agar (CDA, comprising 3% sucrose, 0.3% NaNO3, 0.1% K2HPO4, 0.05% KCl, 0.05% MgSO4, and 0.001% FeSO4, supplemented with 1.5% agar). Additionally, 11 CDA-derived media were prepared, each supplemented with different stress-inducing agents: (i) Osmotic Stresses: 0.4 M NaCl or KCl; (ii) Ionic Stresses: 2 mM CuCl2, 4 mM MnCl2 or FeCl2; (iii) Oxidative Stresses: H2O2 (2 mM) or menadione (0.02 mM); (iv) SDS (100 μg ml–1) or Congo red (10 μg ml–1) for cell wall perturbing stresses; (v) Cell Wall Perturbing Stresses: hydroxyurea (HU, 10mM) or methyl methanesulfonate (MMS, 0.05%). After incubation for 8 days at 25°C, the diameter of each colony was measured. Relative growth inhibition of each strain by each chemical was calculated using the formula: (Sc–St)/Sc×100, where Sc represents the area of the control colony and St represents the area of the stressed colony.
Examination of fungal conidiation and conidial activity
To assess conidiation capacity, aliquots of 100 μl from a 1×107 conidia/ml suspension were spread on SDAY plates (9 cm diameter) and incubated for 9 d at 25°C under a 12:12-hour light/dark cycle. Beginning from day 4 of incubation, three plugs (5 mm diameter) were extracted daily from each plate, and the conidia from each plug were harvested into 1 ml of 0.02% Tween 80 using ultrasonication. The conidial suspensions were then counted using a hemocytometer, and the conidial concentrations were converted to the number of conidia per square centimeter (conidia/cm2). In addition, the sporophore structures on SDAY plates from days 3 to 6 post-inoculation were morphologically examined under microscopy. The size and density (complexity) of 7-day-old aerial conidia (2 × 104 conidia per sample) were quantified according to the forward scatter (FSc) and side scatter (SSc) readings in the flow cytometry. Conidial viability was assessed by determining the time for 50% germination of conidia (GT50) at 25°C.
Insect bioassays to test the fungal virulence
The insect bioassays were conducted using Galleria mellonella larvae with two distinct methods of infection. (i) Topical Bioassays: Approximately 30 larvae were immersed for 10 seconds in 30 ml of a suspension containing 1×107 conidia/ml for cuticular infection. (ii) Intra-hemocoel Assays: For each larva, 5 μl of a suspension containing 1×105 conidia/ml was injected directly into the hemocoel. Following immersion or injection, the larvae were transferred to individual containers and maintained at 25°C for a duration of 10 days. Survival of the larvae was monitored at regular intervals, either every 12 or 24 hours. The bioassay experiments were repeated three times to ensure reliability and consistency. Probit analysis was employed to estimate the mean lethal time (LT50) required to kill 50% of the hosts based on the time-mortality trends. Additionally, after 4 days post-death, fungal hyphae growing on the surfaces of dead larvae were observed and examined.
To investigate the fungal growth and proliferation dynamics, biomass levels (comprising blastospores and hyphae) and blastospore concentrations were quantified from 3-day-old submerged cultures initiated with 50 ml aliquots of a 106 conidia/ml suspension in two different media: CZB (standard medium) and TPB (an amended CZB mimic to insect hemolymph, containing 3% trehalose as the sole carbon source and 0.5% peptone as the sole nitrogen source). The obtained biomass levels and blastospore concentrations were utilized to estimate the dimorphic transition rate (N blastospores/mg), which serves as a reference to the speed of fungal proliferation within the host hemocoel.
Examination of cell cycle and hyphal septation pattern
Hyphae were harvested from cultures inoculated with 50 ml of 1×106 conidia/ml suspension in Sabouraud Dextrose Broth (SDB) and shaken at 150 rpm for 3 days at 25°C. Subsequently, the hyphae were stained with calcofluor white (Sigma) for 15 minutes and examined for septation pattern and cell morphology under a fluorescent microscope. The mean unicellular cell length and width were examined from approximately 30 stained multicellular hyphal cells of each strain using ImageJ software. To facilitate the production of unicellular blastospores for cell cycle analysis, aliquots of 50 ml of a 1×106 conidia/ml suspension were inoculated into Nutrient Limited Broth (NLB) containing 4% glucose, 0.4% NH4NO3, 0.3% KH2PO4 and 0.3% MgSO4. These cultures were then incubated by shaking at 150 rpm for 3 days at 25°C. Blastospores collected from NLB cultures were used to determine the G0/G1, G2/M, and S phases of the cell cycle based on the respective readings of unduplicated (1C), duplicated (2C), and intermediate DNA concentrations obtained from flow cytometry analysis (FACS) of three samples per strain. Additionally, blastospore size and density were assessed using the FSc and SSc readings from the flow cytometry, respectively.
All phenotypic data above were subjected to one-way ANOVA analysis. Post hoc comparisons were performed using Tukey’s honestly significant difference (HSD, p < 0.05) test to determine significant differences between experimental groups.
Genome wide transcriptome analysis
To investigate the potential gene targets and downstream process regulated by BbEaf6, RNA-Seq analyses were conducted using three replicates of 4-day cultures of the ΔBbEaf6 strain and wild type strain (WT) grown on cellophane-overlaid SDAY plates. The cells were harvested using sterilized spoons, and frozen cells were sent to Personal Biotechnology Co., Ltd. (Shanghai, China) for the construction and analysis of transcriptomes. Total RNA extraction was performed using RNA Trizol (Sigma), and mRNAs were isolated from total RNAs using magnetic oligo(dT) beads. The isolated mRNAs were fragmented into segments using the ionic disruption method, and the mRNA fragments served as templates for synthesizing the first-strand cDNAs using random hexamer primers. Subsequently, second-strand cDNAs were synthesized using a cDNA Synthesis Kit (Sigma) with the first-strand cDNAs as templates. Each double-stranded cDNA was purified and end-repaired, with single adenines added to the ends of the cDNA molecules. Finally, a cDNA library was constructed by adding appropriate adaptors to the cDNA. The samples were sequenced on an Illumina HiSeq platform. All raw reads obtained from sequencing the cDNA samples were filtered to generate clean tags, which were then mapped to the genome of B. bassiana [32] with significant levels of log2(ΔBbEaf6/WT ratio) < −1 (down-regulated) or > 1 (up-regulated), and false discovery rate (FDR) < 0.01. All identified Differentially Expressed Genes (DEGs) were functionally annotated with known or putative gene information from the non-redundant NCBI protein databases and subjected to Funcat category classification (https://elbe.hki-jena.de/fungifun/). Additionally, Gene Ontology (GO) enrichment analyses (https://geneontology.org) were performed to enrich the DEGs into various GO categories at a significant level of p < 0.05.
Results
Characterization of BbEaf6, and construction of deletion and complemented strains
Based on BLAST research with yeast Eaf6 (NP_012615) as a query, the Eaf6 homolog was identified in B. bassiana ARSEF 2860 (XP_008601226). In the B. bassiana genomic dataset, BbEaf6 is comprised by a nucleotide sequence of 706 bp containing two introns. The open reading frame (ORF) of BbEaf6 can be translated into a protein of 182 amino acids, with a predicted molecular mass as 19.4 kDa (isoelectric point: 10.03). In terms of the conserved domains, BbEaf6 has only one called NuA4 superfamily domain (residues 28–102), which typically presented in NuA4 subunit superfamily members. Through protein alignment analysis, this conserved domain was also founded in Eaf6 homologs in F. graminearum, Candida albicans, and S. cerevisiae (Figure S1A). Phylogenetic relationship of BbEaf6 with other homologs in representative fungi was also conducted. As showed in the phylogenetic tree (Figure S1B), BbEaf6 shared high sequence identities (~60%–83%) with homologs found in filamentous fungi, while displaying relatively lower identities (~25%–35%) with yeasts, indicating specific roles for Eaf6 homologs in filamentous fungi.
To assess the subcellular localization of BbEaf6, the full coding region of BbEaf6 protein was amplified and fused to the green fluorescent protein (GFP) at the C-terminus of BbEaf6, then a transgenic strain expressing a BbEaf6:GFP fusion protein was generated. After 3-day growth in SDB, the hyphal cells of the transgenic strain were collected from 72-hour Sabouraud dextrose broth (SDB) cultures and stained with the nuclei-specific dye DAPI. As showed in the fluorescence images in , the green fluorescence of BbEaf6 was mainly observed in the nuclei of hyphal cells, which overlap well with the blue fluorescence of the nuclei-specific dye DAPI, indicating predominant nuclear localization of BbEaf6 ().
Figure 1. Subcellular localization of BbEaf6 and its role on conidiation and conidial properties in B. bassiana.
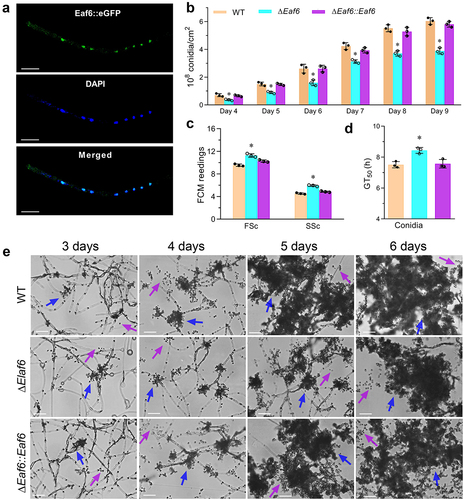
In order to further investigate the function of BbEaf6, the BbEaf6 single gene deletion (ΔBbEaf6) and complemented (ΔBbEaf6:BbEaf6) strains were constructed and verified via PCR and quantitative PCR analyses (Figure S2, primers listed in Table S1).
Impact of Eaf6 deletion on sporulation and conidial yield
To elucidate the role of BbEaf6 in sporulation processes, conidial suspensions of all strains, including wild type, deletion mutant (ΔBbEaf6), and complemented strain (ΔBbEaf6:BbEaf6), were spread on SDAY plates and incubated for 9 days. As showed in , the ΔBbEaf6 strain exhibited significantly decreased conidial production, with reduced conidial yields by ~39%–48% after 4–6 days post-inoculation and ~26%–36% after 7–9 days post-inoculation. Microscopic examination also revealed significantly fewer sporophores (pointed by blue arrows) and conidia (pointed by purple arrows) in the ΔBbEaf6 mutant compared to control strains ().
In terms of the conidial properties, as indicated in the results of FACS (Fluorescence activated Cell Sorting) analyses (), compared to wild type, the conidial size was significantly affected in the ΔBbEaf6 mutant, with an ~18% increase in conidial size and a ~31% increase in conidial density. However, the conidial germiantion was significantly impaired, and the time required for 50% of the conidia to germinate (GT50) was prolonged by ~12% for ΔBbEaf6 cells (). All of these results indicated the important role of BbEaf6 in sporulation and conidial germiantion.
Contribution of BbEaf6 in fungal tolerance to multi-stress
To investigate the role of BbEaf6 in multi-stress tolerances, wild type, ΔBbEaf6, and complemented strain (ΔBbEaf6:BbEaf6) were grown for 8 days in various media, including Czapek-Dox agar (CDA), and CDA modified with different stressful agents. The dual images of fungal colonies and relative growth inhibition index (RGI) calculated based on the diameters were presented in . As indicated in the results, compared to wild type, the deletion of BbEaf6 resulted in reduced fungal tolerances to osmotic stresses, cell wall perturbing agents, and DNA damage stresses. For instance, although the ΔBbEaf6 mutant showed no obvious difference on NaCl, MnCl2 and FeCl2, the loss of BbEaf6 resulted in decreased tolerances to two different ionic stress agents (KCl and CuCl2) by ~11% and ~21%, respectively. Moreover, in the ΔBbEaf6 strains, the fungal sensitivity to two different cell wall perturbing agents, SDS and Congo Red, were significantly increased by ~14% and ~12%. Additionally, the ΔBbEaf6 mutant exhibited increased (~12%) sensitivity to the DNA damage-causing agent, methyl methanesulfonate (MMS), although unaffected sensitivity to another DNA synthesize inhibitor (HU, hydroxyurea). However, the deletion of BbEaf6 exerted on insignificant impact on the fungal tolerance to two different oxidant agents (H2O2 and menadione), as indicated in .
Fig. 2. Impact of BbEaf6 deletion on multi-stress tolerance in B. bassiana.
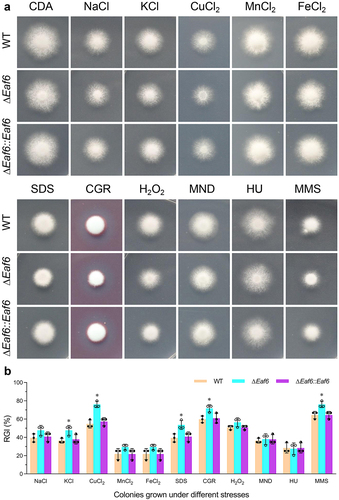
Role of BbEaf6 in hyphal septation and cell cycle control
To determine the role of BbEaf6 in submerged hyphal development, all strains including wild type, ΔBbEaf6, and complemented strain were inoculated in Sabouraud dextrose broth (SDB) for 72-hour. Hyphal cells were collected and stained with the cell wall-specific dye calcofluor white, followed by microscopic examination. As revealed in , the fungal cells of ΔBbEaf6 mutant strain showed obviously altered hyphal septation patterns compared to control strains. The average length of unicellular cells from ΔBbEaf6 hyphae were significantly increased by ~26% than wild type strain, while with no dramatic alteration in cell width ().
Fig. 3. Contributions of Eaf6 in hyphal septation and cell cycle control in B. bassiana.
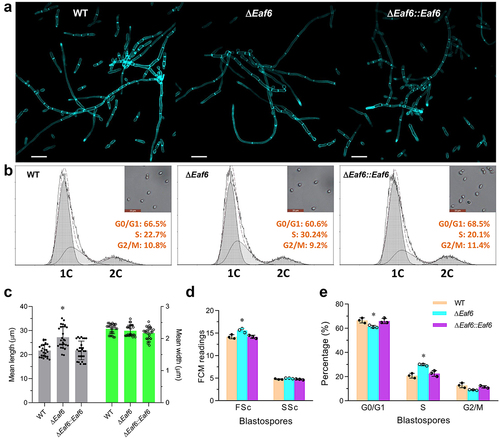
To examine potential effects of BbEaf6 on cell cycle progression, the ΔBbEaf6 mutant and control strains were incubated for 3 days at 25°C in nitrogen-limited broth (NLB) used to generate unicellular blastospores. The resulting cells were analyzed by flow cytometry after staining with the DNA-specific dye propidium iodide. Compared to the wild type, blastospores derived from the ΔBbEaf6 mutant strain showed a slight increase (~10%) in size but no change in cell density (). The ΔBbEaf6-derived cells exhibited significantly shorter (by 8%) G0/G1 phase (~60.6%), but longer (by 30%) S phase (~30.24%), while unaffected G2/M phase times (~9.2%) (), indicating pronounced S phase. These results revealed the vital role of BbEaf6 in cell cycle control and hyphal septation.
BbEaf6 is indispensable for fungal virulence
To assess the role of BbEaf6 in B. bassiana virulence, insect bioassays using Greater wax moth, Galleria mellonella, larvae as hosts were conducted via both topical application bioassays (immersion) and intra-hemocoel injection bioassays (injection), with the median lethal time for killing 50% of the target hosts (LT50) calculated (). Overall, compared to wild type, the ΔBbEaf6 strains exhibited decreased efficiency in killing insect host both in topical bioassays and in intra-hemocoel injection assays. In topical bioassays, the average of LT50 for the ΔBbEaf6 strains was ~5.19 days, which was significantly prolonged by ~23% compared to the wild type strain. While in intra-hemocoel injection assays, the average of LT50 for the ΔBbEaf6 strains was ~4.79 days, which was prolonged by ~12%. Additionally, after 4 days post-death, the fungal outgrowths observed on the cadavers of theΔBbEaf6 strains were noticeably reduced compared to the control strains, indicating the attenuated ability to penetrate insect cuticle ().
Fig. 4. Impact of Eaf6 deletion on fungal virulence upon insect host in B. bassiana.
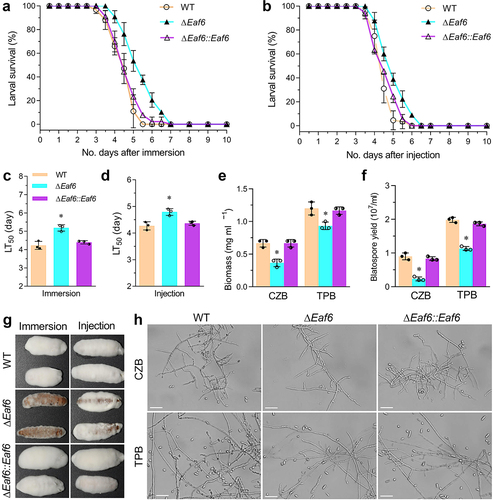
The attenuated intra-hemocoel virulence suggested that the transition of penetrating hyphae to unicellular blastospores was delayed or impeded in the ΔBbEaf6 mutant. To test this hypothesis, we quantified the biomass and blastspore production after 3 days of growth in CZB and trehalose-peptone broth (TPB), a modified CZB that mimics insect hemolymph. Correspondingly, the ΔBbEaf6 mutant showed significantly decreased total biomass in CZB (~45%) and TPB (~22%) media compared to the wild type and complemented mutants (). In terms of blastospore production, the ΔBbEaf6 mutant was even more affected, showing decreased blastospores in CZB (~64%) and TPB (~43%) cultures (). Based on the biomass and blastospore yield, the dimorphic transition rate in the ΔBbEaf6 mutant was decreased in CZB (~53%) and TPB (~26%) cultures compared to wild type. Under microscopy, the capacity of blastospores in the ΔBbEaf6 mutant was obviously much less than in the control strains inoculated in the two different media above (). These results suggested that the deletion of BbEaf6 affected the fungal growth in the insect body.
Role of BbEaf6 in global gene transcription
To investigate the potential gene targets regulated by BbEaf6, comparative transcriptomic analyses was conducted to compare ΔBbEaf6 and wild type cells using triplicate biological samples, as detailed in the Materials and Methods section. A total of 810 differentially expressed genes (DEGs) were identified between the ΔBbEaf6 mutant and wild type (Table S2). Among these DEGs, 224 DEGs were down-regulated [(Log2 (ratio): −4.55 to −1.00)], while 586 DEGs were up-regulated [(Log2 (ratio): +1.00 to +8.75)] in the ΔBbEaf6 mutant compared to the wild type (, Table S2).
Fig. 5. The global regulatory role of BbEaf6 in gene transcription B. bassiana.
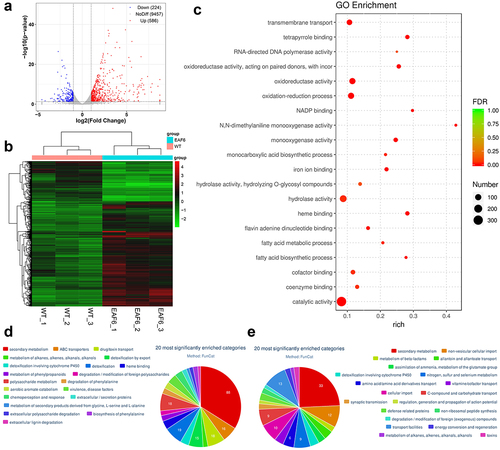
Gene Ontology (GO) enrichment analyses were performed to identify gene functions that are significantly regulated in the ΔBbEaf6 mutant relative to the wild type (Table S3). Among the Top 20 enriched GO terms (), 314 DEGs were involved in catalytic activity, 125 DEGs were in hydrolase activity, 96 DEGs were in oxidoreductase activity and oxidation-reduction processes, and 71 DEGs were in transmembrane transport. Followed were those involved in cofactor binding (37), monooxygenase activity (35), heme binding (31), tetrapyrrole binding (31), iron ion binding (28), coenzyme binding (27), and flavin adenine dinucleotide binding (21). Moreover, the other DEGs were related to NADP binding (11), monocarboxylic acid biosynthetic process (11), fatty acid metabolic process (11), fatty acid biosynthetic process (10), N,N-dimethylaniline monooxygenase activity (9), and RNA-directed DNA polymerase activity (7), etc.
FunCat category classification was also performed to classify the up-regulated genes and down-regulated genes in the ΔBbEaf6 mutant relative to the wild type (Table S4 & S5). There were 178 up-regulated genes annotated in 37 different categories (). Among the 20 most significantly enriched categories, 88 DEGs were involved in secondary metabolism, followed by 19 DEGs in detoxification; 18 DEGs in drug/toxin transport; 18 DEGs in polysaccharide metabolism; 16 DEGs in ABC transporters; and 15 DEGs in detoxification by export. Moreover, the other DEGs were classified in detoxification involving cytochrome P450 (9), heme binding (9), chemoperception and response (8), aerobic aromate catabolism (7), and metabolism of phenylpropanoids (6), degradation of phenylalanine (6), etc. On the contrary, there were 71 down-regulated genes annotated in 21 different categories (). Among the 20 most significantly enriched categories, 33 DEGs were involved in secondary metabolism, followed by 13 DEGs in transport facilities; 12 DEGs in non-vesicular cellular import; and 10 DEGs in cellular import. Moreover, the other DEGs were classified in nitrogen, sulfur, and selenium metabolism (9), C-compound and carbohydrate transport (9), amino acid/amino acid derivatives transport (6), allantoin and allantoate transport (5), detoxification involving cytochrome P450 (5), and vitamin/cofactor transport (5), etc. These trancriptional changes indicating a global regulatory role of BbEaf6 in gene regulation in B. bassiana.
Discussion
Protein acetylation has been regarded as a crucial regulatory mechanism in fungal infection process [33]. Histone acetyltransferases (HATs), which are responsible for lysine acetylation, have been proved to play vital roles in various pathogenic fungi, including different kinds of animal/human pathogens and plant pathogens [34,35,36,37,38]. In B. bassiana, five HATs, including members of the GNAT and MYST superfamily, have been studied regarding their roles in growth and virulence. Notably, Gcn5, Elp3, Spt10, Mst2, and Rtt109 have demonstrated varying contributions to cell morphogenesis and virulence [27,28,29,30,31]. However, the function of the NuA4 HAT complex, particularly its effects on filamentous fungi development and virulence, remains poorly understood. Attempts to delete the catalytic subunit Esa1 in B. bassiana, similar to yeast cells, were unsuccessful, suggesting its essentiality in filamentous fungi. Likewise, efforts to knock out the NuA4 subunit Elp1 also failed. However, successful knockout of Eaf6 in B. bassiana provided insight into its function in this fungus, shedding light on the role of the NuA4 HAT complex in fungal biology and pathogenicity.
The homologs of Eaf6 are integral components of the NuA4 and NuA3 complexes in yeast, as well as the MOZ/MORF complexes in humans, where they participate in transcriptional activation, gene regulation, and cell cycle control [4,6].In this study, we identified a single Eaf6 homolog in B. bassiana, which, as anticipated, exhibited predominant nuclear localization, a characteristic shared with the plant pathogen F. graminearum [20]. Notably, the deletion of BbEaf6 in B. bassiana resulted in differential regulation of a moderate number of genes (810), indicating its involvement in transcriptional regulation. Interestingly, the overall growth defect observed in the ΔBbEaf6 mutant was less severe compared to that reported in yeast [15]. Specifically, we observed a higher number of upregulated genes (586) compared to downregulated genes (224) in the ΔBbEaf6 mutant strain, suggesting a potential suppressor role for BbEaf6 in gene regulation.
Conidia serve as the primary active ingredient in insect biological control applications utilizing B. bassiana, and maintaining their viability is crucial for effective pest control [22]. In the ΔBbEaf6 mutant, we observed delayed conidiation and reduced conidial yield, likely attributable to the significantly prolonged S-phase observed in ΔBbEaf6 cells. Proper cell cycle regulation and hyphal septation are known to be essential for both vegetative growth and spore development [39]. Furthermore, RNA-Seq analysis of the ΔBbEaf6 mutant revealed a wide range of differentially expressed genes (DEGs) involved in asexual development and cell cycle progression. For instance, the expression of two crucial factors for asexual development, AbaA and FlbC [40,41], was significantly regulated in the ΔBbEaf6 mutant, likely contributing to the impaired sporulation observed. More specifically, the transcription of AbaA was downregulated duo to the deletion of BbEaf6, while FlbC was upregulated. Additionally, the expression of several protein kinases implicated in DNA processes and cell cycle regulation were differentially regulted in the ΔBbEaf6 mutant. These included members of the CAMK family, elongation factor-2 kinase EFK-1B, and ribose-phosphate pyrophosphokinase [42,43]. These findings highlight the intricate regulatory network orchestrated by BbEaf6 in governing fungal development and cellular processes.
Conidial tolerance to various stresses and complex environmental conditions is crucial for the viability of fungal conidia [23]. In the ΔBbEaf6 mutant, we observed decreased conidial tolerance across different types of stressors, particularly ionic stress, cell wall-disturbing stress, and DNA damage stress. This reduced tolerance correlated with significantly downregulated expression of numerous transporter genes in the ΔBbEaf6 mutant, including 24 MFS transporters, twelve ABC transporters, four OPT oligopeptide transporters, and two ion transporters. However, two genes associated with cell wall integrity and ion homeostasis was significantly upregulated, including the cell wall stress-responsive component WSC4D and P-type IIC/NK Na+/K±ATPases (NK1) [44,45]. Furthermore, several genes involved in DNA replication and repair were significantly downregulated in the ΔBbEaf6 mutant, including DNA repair protein Rad57 [46], elongation factor-2 kinase, and RNase H domain containing protein. These transcriptional alterations likely contribute to the impaired conidial tolerance to various stressful conditions observed in the ΔBbEaf6 mutant. The comprehensive understanding of these molecular mechanisms sheds light on the role of BbEaf6 in orchestrating fungal responses to environmental stressors, thereby influencing conidial viability and fungal fitness.
Our findings also underscore the essential role of BbEaf6 in the full virulence of B. bassiana, encompassing both the ability to breach the insect cuticle and subsequent growth and evasion of the host immune response within the hemocoel. The deletion of BbEaf6 resulted in significantly differential regulation of numerous genes crucial for cuticle penetration and hemocoel infection processes in B. bassiana. For instance, some protein/peptide degrading enzymes were found to be downregulated, including four peptidases, one aspartic protease, a subtilase-like protein and lipase 2 precursor. Also, the expression of two subtilisin-like proteases (Pr1A and Pr1C), which are pivotal for cuticle degradation and invasion [47], were differentially regulated in the ΔBbEaf6 mutant. Additionally, the expression of hydrophobins, essential for fungal conidial hydrophobicity and adhesion to the host cuticle [48], and LysM domain proteins, known to suppress chitin-induced immunity and contribute to fungal virulence in B. bassiana [49], was also found to be differentially regulated in the ΔBbEaf6 mutant.
In summary, our study elucidates functional aspects of BbEaf6, highlighting its role in multi-stress sensitivity, delayed cell cycle progression and conidiation, and impaired virulence as major consequences of BbEaf6 loss. These insights provide valuable knowledge for understanding the molecular mechanisms underlying fungal pathogenesis and contribute to the development of novel strategies for insect pest management.
Author contributions
Conception and design & Data analysis and interpretation: Q.C. and J.-J. W.; the Drafting of the paper: Q.C.; Revision and the final approval: J.-T.-X. and D.-H. J.; All authors agree to be accountable for all aspects of the work.
Declaration of interest statement
The authors declare no conflict of interest.
Data Availibility Statement
All transcriptomics data have been deposited in the Sequence Read Archive (SRA) on NCBI (https://www.ncbi.nlm.nih.gov/) and are openly available with the dataset identifiers PRJNA721731.
Supporting information20240429.xlsx
Download MS Excel (105 KB)Eaf6 Supporting Information20240428.docx
Download MS Word (1.9 MB)Supplementary material
Supplemental data for this article can be accessed online at https://doi.org/10.1080/21505594.2024.2387172
Additional information
Funding
References
- Millar CB, Grunstein M. Genome-wide patterns of histone modifications in yeast. Nat Rev Mol Cell Biol. 2006;7(9):657–666. doi: 10.1038/nrm1986
- Li B, Carey M, Workman JL. The role of chromatin during transcription. Cell. 2007;128(4):707–719. doi: 10.1016/j.cell.2007.01.015
- Wang Z, Zang C, Cui K, et al. Genome-wide mapping of HATs and HDACs reveals distinct functions in active and inactive genes. Cell. 2009;138(5):1019–1031. doi: 10.1016/j.cell.2009.06.049
- Doyon Y, Selleck W, Lane WS, et al. Structural and functional conservation of the NuA4 histone acetyltransferase complex from yeast to humans. Mol Cell Biol. 2004;24(5):1884–1896. doi: 10.1128/MCB.24.5.1884-1896.2004
- Keogh MC, Mennella TA, Sawa C, et al. The Saccharomyces cerevisiae histone H2A variant Htz1 is acetylated by NuA4. Genes Dev. 2006;20(5):660–665. doi: 10.1101/gad.1388106
- Doyon Y, Cote J. The highly conserved and multifunctional NuA4 HAT complex. Curr Opin Genet Dev. 2004;14(2):147–154. doi: 10.1016/j.gde.2004.02.009
- Squatrito M, Gorrini C, Amati B. Tip60 in DNA damage response and growth control: many tricks in one HAT. Trends Cell Biol. 2006;16(8):433–442.
- Krogan NJ, Baetz K, Keogh MC, et al. Regulation of chromosome stability by the histone H2A variant Htz1, the Swr1 chromatin remodeling complex, and the histone acetyltransferase NuA4. Proc Natl Acad Sci USA. 2004;101(37):13513–13518.
- Lin YY, Lu JY, Zhang J, et al. Protein acetylation microarray reveals that NuA4 controls key metabolic target regulating gluconeogenesis. Cell. 2009;136(6):1073–1084. doi: 10.1016/j.cell.2009.01.033
- Downey M, Knight B, Vashisht AA, et al. Acetylome profiling reveals overlap in the regulation of diverse processes by sirtuins, GCN5, and ESA1. Mol Cell Proteomics. 2015;14(1):162–176.
- Taverna SD, Ilin S, Rogers RS, et al. Yng1 PHD finger binding to H3 trimethylated at K4 promotes NuA3 HAT activity at K14 of H3 and transcription at a subset of targeted ORFs. Mol Cell. 2006;24(5):785–796. doi: 10.1016/j.molcel.2006.10.026
- Wang X, Chang P, Ding J, Chen J. Architecture of the Saccharomyces cerevisiae NuA4/TIP60 complex. Nat Commun. 2018;9:1147.
- Ullah M, Pelletier N, Xiao L, et al. Molecular architecture of quartet MOZ/MORF histone acetyltransferase complexes. Mol Cell Biol. 2008;28(20):6828–6843.
- Yang XJ. MOZ and MORF acetyltransferases: molecular interaction, animal development and human disease. Biochim Biophys Acta. 2015;1853(8):1818–1826. doi: 10.1016/j.bbamcr.2015.04.014
- Mitchell L, Lambert JP, Gerdes M, et al. Functional dissection of the NuA4 histone acetyltransferase reveals its role as a genetic hub and that Eaf1 is essential for complex integrity. Mol Cell Biol. 2008;28(7):2244–2256. doi: 10.1128/MCB.01653-07
- Lafon A, Chang CS, Scott EM, Jacobson SJ, Pillus L. MYST opportunities for growth control: yeast genes illuminate human cancer gene functions. Oncogene. 2007;26(38):5373–5384.
- Panagopoulos I, Micci F, Thorsen J, et al. Novel fusion of MYST/Esa1-associated factor 6 and PHF1 in endometrial stromal sarcoma. PLoS One. 2012;7(6):e39354. doi: 10.1371/journal.pone.0039354
- Zhou JX, Su XM, Zheng SY, et al. The Arabidopsis NuA4 histone acetyltransferase complex is required for chlorophyll biosynthesis and photosynthesis. J Integr Plant Biol. 2022;64(4):901–914. doi: 10.1111/jipb.13227
- Kubo T, Yoshimura A, Kurata N Loss of OsEAF6, a Subunit of the Histone Acetyltransferase Complex, Causes Hybrid Breakdown in Intersubspecific Rice Crosses. Front Plant Sci. 2022;13:866404. 10.3389/fpls.2022.866404
- Qin J, Wu M, Zhou S FgEaf6 regulates virulence, asexual/sexual development and conidial septation in Fusarium graminearum. Curr Genet. 2020 Jun;66(3):517–529.10.1007/s00294-019-01043-0
- Mascarin GM, Jaronski ST. The production and uses of Beauveria bassiana as a microbial insecticide. World J Microbiol Biotechnol. 2016;32(11):177. doi: 10.1007/s11274-016-2131-3
- Ortiz-Urquiza A, Luo ZB, Keyhani NO. Improving mycoinsecticides for insect biological control. Appl Microbiol Biotechnol. 2015;99(3):1057–1068. doi: 10.1007/s00253-014-6270-x
- Ortiz-Urquiza A, Keyhani NO. Stress response signaling and virulence: insights from entomopathogenic fungi. Curr Genet. 2015;61(3):239–249. doi: 10.1007/s00294-014-0439-9
- Xiao GH, Ying SH, Zheng P, et al. Genomic perspectives on the evolution of fungal entomopathogenicity in Beauveria bassiana. Sci Rep. 2012;2(1):483. doi: 10.1038/srep00483
- Ortiz-Urquiza A, Keyhani NO. Molecular genetics of Beauveria bassiana infection of insects. Adv Genet. 2016;94:165249.
- Cai Q. Wang ZK, Shao W, et al. Essential role of Rpd3-dependent lysine modification in the growth, development and virulence of Beauveria bassiana. Environ Microbiol. 2018;20:1590–1606.
- Cai Q, Wang JJ, Fu B, Ying SH, Feng MG. Gcn5-dependent histone H3 acetylation and gene activity are required for the asexual development and virulence of Beauveria bassiana. Environ Microbiol. 2018;20:1484–1497.
- Cai Q, Wang JJ, Shao W, et al. Rtt109-dependent histone H3 K56 acetylation and gene activity are essential for the biological control potential of Beauveria bassiana. Pest Manag Sci. 2018;74(11):2626–2635. doi: 10.1002/ps.5054
- Cai Q, Wang JJ, Xie JT, Jiang DH, Keyhani NO. The Spt10 GNAT Superfamily Protein Modulates Development, Cell Cycle Progression and Virulence in the Fungal Insect Pathogen, Beauveria bassiana J Fungi (Basel). 2021;7(11):905.
- Cai Q, Wang J, Xie J, et al. The elongator subunit Elp3 regulates development, stress tolerance, cell cycle, and virulence in the entomopathogenic fungus beauveria bassiana. J Fungi (Basel). 2022;8(8):834. doi: 10.3390/jof8080834
- Wang JJ, Cai Q, Qiu L, et al. The histone acetyltransferase Mst2 sustains the biological control potential of a fungal insect pathogen through transcriptional regulation. Appl Microbiol Biotechnol. 2018;102(3):1343–1355. doi: 10.1007/s00253-017-8703-9
- Ying SH, Feng MG. Novel blastospore-based transformation system for easy integration of phosphinothricin resistance and green fluorescence protein genes into Beauveria bassiana. Appl Microbiol Biotechnol. 2006;72:206–210.
- Chen J, Liu Q, Zeng L, et al. Protein Acetylation/Deacetylation: A Potential Strategy for Fungal Infection Control. Front Microbiol. 2020;11:574736. 10.3389/fmicb.2020.574736
- Wang X, Chang P, Ding J, Chen J. Distinct and redundant roles of the two MYST histone acetyltransferases Esa1 and Sas2 in cell growth and morphogenesis of Candida albicans. Eukaryot Cell. 2013;12:43–449.
- Chen X, Wu L, Lan H, et al. Histone acetyltransferases MystA and MystB contribute to morphogenesis and aflatoxin biosynthesis by regulating acetylation in fungus aspergillus flavus. Environ Microbiol. 2022;24(3):1340–1361. doi: 10.1111/1462-2920.15856
- O’Meara TR, Hay C, Price MS, et al. Cryptococcus neoformans histone acetyltransferase Gcn5 regulates fungal adaptation to the host. Eukaryot Cell. 2010;9(8):1193–1202. doi: 10.1128/EC.00098-10
- Kwon S, Lee J, Jeon J, et al. Role of the histone acetyltransferase Rtt109 in development and pathogenicity of the rice blast fungus. Mol Plant Microbe Interact. 2018;31(11):1200–1210. doi: 10.1094/MPMI-01-18-0015-R
- González-Prieto JM, Rosas-Quijano R, Domínguez A, et al. The UmGcn5 gene encoding histone acetyltransferase from Ustilago maydis is involved in dimorphism and virulence. Fungal Genet Biol. 2014;71:86–95. 10.1016/j.fgb.2014.09.002
- Qiu L, Wang JJ, Ying SH, et al. Wee1 and Cdc25 control morphogenesis, virulence and multistress tolerance of Beauveria bassiana by balancing cell cycle-required cyclin-dependent kinase 1 activity. Environ Microbiol. 2015;17(4):1119–1133. doi: 10.1111/1462-2920.12530
- Zhang AX, Mouhoumed AZ, Tong SM, et al. BrlA and AbaA Govern Virulence-Required Dimorphic Switch, Conidiation, and Pathogenicity in a Fungal Insect Pathogen. mSystems. 2019;4(4):e00140–19. doi: 10.1128/mSystems.00140-19
- Guo CT, Luo XC, Ying SH, Feng MG. Differential Roles of Five Fluffy Genes (flbA-flbE) in the Lifecycle In Vitro and In Vivo of the Insect-Pathogenic Fungus Beauveria bassiana. J Fungi (Basel). 2022 Mar 23;8(4):334.
- Skelding KA, Rostas JA, Verrills NM. Controlling the cell cycle: the role of calcium/calmodulin-stimulated protein kinases I and II. Cell Cycle. 2011 Feb 15;10(4):631–9.
- Bertoli C, Skotheim JM, de Bruin RA. Control of cell cycle transcription during G1 and S phases. Nat Rev Mol Cell Biol. 2013 Aug;14(8):518–28.
- Tong SM, Chen Y, Zhu J, et al. Subcellular localization of five singular WSC domain-containing proteins and their roles in Beauveria bassiana responses to stress cues and metal ions. Environ Microbiol Rep. 2016 Apr;8(2):295–304.10.1111/1758-2229.12380
- Mou YN, Gao BJ, Ren K, et al. P-type Na+/K+ ATPases essential and nonessential for cellular homeostasis and insect pathogenicity of Beauveria bassiana. Virulence. 2020;11(1):1415–1431. doi: 10.1080/21505594.2020.1836903
- Roy U, Kwon Y, Marie L, Symington L, Sung P, Lisby M, Greene EC. The Rad51 paralog complex Rad55-Rad57 acts as a molecular chaperone during homologous recombination. Mol Cell. 2021 Mar 4;81(5):1043–1057.e8.
- Gao BJ, Mou YN, Tong SM, et al. Subtilisin-like Pr1 proteases marking the evolution of pathogenicity in a wide-spectrum insect-pathogenic fungus. Virulence. 2020;11(1):365–380. doi: 10.1080/21505594.2020.1749487
- Zhang SZ, Xia YX, Kim B, Keyhani NO. Two hydrophobins are involved in fungal spore coat rodlet layer assembly and each play distinct roles in surface interactions, development and pathogenesis in the entomopathogenic fungus, Beauveria bassiana Mol Microbiol. 2011;80:811–826.
- Cen K, Li B, Lu Y, et al. Divergent LysM effectors contribute to the virulence of Beauveria bassiana by evasion of insect immune defenses. PloS Pathog. 2017;13(9):e1006604. doi: 10.1371/journal.ppat.1006604