ABSTRACT
Infectious bursal disease (IBD) is a widespread problem in the poultry industry, and vaccination is the primary preventive method. However, moderately virulent vaccines may damage the bursa, necessitating the development of a safe and effective vaccine. The Newcastle disease virus (NDV) has been explored as a vector for vaccine development. In this study, reverse genetic technology was used to obtain three recombinant viruses, namely, rClone30-VP2L (P/M)-chGM-CSF (NP), rClone30-chGM-CSF (P/M)-VP2L (NP), and rClone30-VP2L-chGM-CSF (P/M). Animal experiments showed that the three biological adjuvant bivalent vaccines effectively increased anti-NDV and anti-infectious bursal disease virus (IBDV) titres, enhancing both humoral and cellular immune responses in chickens without leading to any harm. Amongst the three biological adjuvant bivalent vaccines, the rClone30-chGM-CSF (P/M)-VP2L (NP) group had higher levels of anti-NDV antibodies at 14 days after the first immunization and stimulated a greater humoral immune response in 7–10 days. While, the rClone30-VP2L (P/M)-chGM-CSF (NP) group was the most effective in producing a higher level of IBDV antibody response. In conclusion, these three vaccines can induce immune responses more rapidly and effectively, streamline production processes, be cost-effective, and provide a new avenue for the development of Newcastle disease (ND) and IBD bivalent vaccines
Introduction
In many countries, Newcastle disease (ND) and infectious bursal disease (IBD) have seriously impacted poultry production and the social economy [Citation1,Citation2]. ND is a highly contagious disease that causes numerous deaths in chickens. ND is caused by an enveloped, non-segmented, and negative-stranded RNA virus, known as ND virus (NDV). It is also known as avian paramyxovirus type 1 (APMV-1), which belongs to the families Paramyxoviridae and Monoviridae [Citation3]. Further, based on their pathogenicity for birds, NDV strains are classified into three main pathogens: highly (velogenic), intermediate (mesogenic), and non-virulent (lentogenic). Furthermore, according to the sequencing of their F genes, these strains are categorized into two main classes (I and II). Class I viruses are isolated from wild birds, and the majority of them are low virulent. While, Class II viruses were basically divided into various genotypes, including more virulent and less virulent viruses. Some of low-virulence NDV strains, including B1, LaSota, and VG/GA are widely used as vaccines to prevent ND in poultry [Citation4]. Amongst, the LaSota strain has been used as a naturally occurring and attenuated live vaccine for more than half a century in China and worldwide with widely accepted safety and efficacy [Citation4]. Clone 30, a vaccine strain originating from LaSota, and there is no detectable genetic recombination with this strain that will make it safer and more stable [Citation5]. The NDV genome encodes six structural protein, includidng nucleocapsid protein (NP), phosphoprotein (P), matrix protein (M), fusion protein (F), haemagglutinin-neuraminidase protein (HN) and polymerase protein (L) [Citation6]. Studies have shown that genomic RNA, NP, P, and L proteins form the transcribed and replicated template (ribonucleoprotein complex RNP) [Citation4]. Recently, more modified RNA viruses have been made into vector vaccines due to advances in reverse genetics technology [Citation7]. NDV is a bivalent vaccine that has been developed as a vector in the poultry industry. This vector expresses exogenous proteins, thereby inducing a broad range of protective immune responses, including humoral and cellular immunity. The safety has been confirmed in many animal models [Citation8], and the expression of foreign genes is influenced by factors such as the size of their fragments and the position of their insertion into the NDV genome. Also, the expression of foreign genes significantly affects the effectiveness of the immune response [Citation9]. Studies have revealed that the non-coding regions between the P and M genes, as well as behind the open reading frame (ORF) of the NP gene in the NDV genome, are separated by independent transcription units (ITU). The internal ribosome entry site (IRES) approach can be used to identify optimal insertion sites for efficient exogenous gene expression [Citation10].
IBD is an intense, immunosuppressive viral epidemic that generally attacks the B-lymphocytes in the bursa of Fabricius (BF), leading to immunosuppression in chickens [Citation11]. IBD Virus (IBDV) is a non-enveloped RNA virus that belongs to the genus Avibirnavirus in the family Birnaviridae. Viral mature protein 2 (VP2) protein is the main component of IBDV capsid containing the main epitopes that induce neutralizing antibody production in the host [Citation12]. Generally, the VP2 protein of IBDV is used as a subunit vaccine to prevent IBD [Citation12]; however, it is composed of five neutralizing epitope antigen proteins [Citation13]. In the study, it was used as the foreign gene to insert into NDV recombinant virus vector at different sites. The VP2L gene is smaller than the VP2 gene; therefore, the expression level of VP2L may be higher in the NDV recombinant virus vector than VP2. Additionally, the VP2L protein induces higher neutralizing antibody levels than the VP2 whole protein [Citation13].
The application of adjuvants is vital to enhance the immune effects of vaccines. Granulocyte-macrophage colony-stimulating factor (GM-CSF) is a cytokine that stimulates the proliferation and differentiation of multifunctional haematopoietic stem cells. GM-CSF stimulates the proliferation, differentiation, and maturation of haematopoietic stem cells and metastasis to the peripheral bone marrow and activates macrophages, neutrophils, and other monocytes [Citation14]. Additionally, GM-CSF activates CD4+ and CD8+ T cells and enhances the ability of antigen-presenting cells (APCs) to present antigens, particularly dendritic cells (DC), stimulating their proliferation, differentiation, maturation, and local aggregation upon injection [Citation15]. Several studies have confirmed that GM-CSF is a safe and effective molecular adjuvant with multiple potentials to promote the body’s immune response to vaccines, thereby enhancing immune resistance against pathogens [Citation16–18]. Furthermore, the GM-CSF gene was added to the NDV recombinant virus vector as a biological adjuvant, which can improve the immune response level of the recombinant virus bivalent vaccine. Thus, the immune response level of the biological adjuvant bivalent vaccine may be better than that of the vaccine prepared with recombinant NDV expressing IBDV VP2.
In this study, three bio adjuvant vaccines utilized the NDV vector to express the neutralizing epitope antigen VP2L gene of IBDV and the immune adjuvant chGM-CSF. To explore the immune effects of the rClone30-VP2L (P/M)-chGM-CSF (NP) vaccine, rClone30-chGM-CSF (P/M)-VP2L (NP) vaccine, and rClone30-VP2L-chGM-CSF (P/M) vaccine, three different insertion methods were selected to insert two foreign genes into the NP gene and the P/M gene site of the NDV vector. This study focused on determining the optimal method to insert two foreign genes into the NDV.
Materials and methods
Procurement of plasmids, vectors, viruses, vaccines, cell lines, SPF embryos and animals
The plasmids pBrClone30-chGM-CSF (P/M), pBrClone30-VP2L (P/M), pTM-NP, pTM-P, and pTM-L were stored at −80°C. DF1 cells, BHK-21 cells, rClone30 virus, rabbit anti-GM-CSF polyclonal antibody and rabbit anti-VP2 polyclonal antibody were received from our laboratory. The pMD19-T simple vector was procured from TaKaRa (Japan). The E. coli DH5α was obtained from Tsingke Biotechnology Co., Ltd. The commercial vaccine (LaSota strain + M41 strain + YBF003 strain + S-VP2 protein) was received from Qingdao Yebio (China). Nine-day-old SPF chicken embryos and fourteen-day-old SPF chickens were obtained from the National Poultry Laboratory Animal Resource Center.
Recombinant NDV plasmids construction
pBrClone30-VP2L (P/M) plasmid was used as the template, and VP2L gene was sub-cloned with P1 and P2 or P3 and P4 primers using PCR. The PCR products of VP2L gene were ligated into the vector pBrClone30-chGM-CSF (P/M) using Sac II or Hpa I and Mlu I and named as pBrClone30-VP2L (P/M)-chGM-CSF (P/M) or pBrClone30-chGM-CSF (P/M)-VP2L (NP). The pBrClone30-chGM-CSF (P/M) plasmid was used as the template, and chGM-CSF gene was sub-cloned with P5 and P6 primers using PCR. The PCR products of chGM-CSF were ligated into the vector pBrClone30-VP2L (P/M) using Hpa I and Mlu I and designated as pBrClone30-VP2L (P/M)-chGM-CSF (NP).
Rescue of recombinant NDV viruses
Rescue of pBrClone30-VP2L (P/M)-chGM-CSF (NP), pBrClone30-chGM-CSF (P/M)-VP2L (NP), pBrClone30-VP2L (P/M)-chGM-CSF (P/M), pBrClone30-VP2L (P/M) and pBrClone30-chGM-CSF (P/M) was performed with BHK-21 cells as mentioned above [Citation17]. The cell lysate was collected by following the freeze and thaw cycle three times. The supernatants were collected and inoculated into the allantoic cavities of nine-day-old SPF chicken embryos. After 72 h of inoculation, allantoic fluids were collected for haemagglutination assay (HA) evaluation. Propagating the HA-positive viruses in allantoic fluids and storing at −80°C. The rescued recombinant viruses were specified rClone30-chGM-CSF (P/M)-VP2L (NP), rClone30-VP2L (P/M)-chGM-CSF (NP), rClone30-VP2L-chGM-CSF (P/M) or rClone30-VP2L (P/M) and rClone30-chGM-CSF (P/M), respectively.
RT-PCR
The recombinant viruses rClone30-chGM-CSF (P/M)-VP2L (NP), rClone30-VP2L (P/M)-chGM-CSF (NP), rClone30-VP2L-chGM-CSF (P/M), rClone30-chGM-CSF (P/M) and rClone30-VP2L (P/M), were inoculated in SPF chicken embryos three times separately and RNA of the recombinant viruses was extracted. The VP2L and chGM-CSF genes were detected by RT-PCR and both gene sequences were compared with the original sequence by DNAMAN.
Western blot analysis
The supernatant recombinant viruses-infected DF1 cell was obtained, and protein samples were prepared. The proteins were separated by sodium dodecyl sulphate-polyacrylamide gel electrophoresis (SDS-PAGE) and transferred onto the nitrocellulose membrane. Subsequently, the membrane was blocked by incubating with 5% skimmed milk in PBS at 37°C for 2 h and followed by incubation with rabbit anti-VP2 polyclonal antibody (1:2 500 dilution) or rabbit anti-chGM-CSF polyclonal antibody (1:2 000 dilution) at 4°C. The next day, the membrane was washed thrice with PBST and then incubated with HRP-goat anti-rabbit IgG antibody (1:7 500 dilution) at 37°C for 1 h. Lastly, the finalized membrane was detected using enhanced chemiluminescence (ECL) reagents.
HA, TCID50, EID50, ICPI and MDT assays
Using the HA test, 50% egg infectious dose (EID50) and 50% tissue infection dose (TCID50) test viral titre was determined, and TCID50 was implemented with DF1 cells on the basis of the Alexander method [Citation19]. The pathogenicity of recombinant viruses and parental viruses was determined by measuring the mean death time (MDT) in embryonated chicken eggs and the intracerebral pathogenicity index (ICPI) in one-day-old SPF chickens [Citation19]. The ICPI, MDT, TCID50, and EID50 were calculated as reported in previous research [Citation19].
The growth curve of recombinant viruses
The DF1 cells in the six-well plate were transfected with 0.1 MOI recombinant viruses. After 1 h of culture, the supernatant was harvested and the plates were supplemented with 2 mL DMEM medium containing 1% antibiotics and 2% FBS. The supernatants were harvested at 24, 48, 72 and 96 h after infection, respectively. The virus titre in DF1 cells was estimated by following an endpoint titration and calculated as infection dose per mL 50% tissue culture (log10 TCID50).
Ethics statement
The Experimental Animal Ethics Committee of Northeast Agricultural University reviewed and approved all the animal experiments (approval no. NEAUEC20210111). ARRIVE guidelines were followed for all the in vivo experiments.
Immunization of chicken
One hundred and sixty fourteen-day-old SPF chickens were randomly divided into eight groups of 20 chickens each. In the positive control group (group D), chickens were immunized intramuscularly with 250 µL of commercial vaccine (LaSota+M41+YBF003+S-VP2 protein strain). In other groups (A, B, C, E, F, and G), chickens were immunized intramuscularly with 106 EID50 dose of recombinant viruses; group A was treated with rClone30-VP2L (P/M)-chGM-CSF (NP); group B was treated with rClone30-chGM-CSF (P/M)-VP2L (NP); group C was treated with rClone30-VP2L-chGM-CSF (P/M); group E was treated with rClone30-chGM-CSF (P/M); group F was treated with rClone30-VP2L (P/M); group G treated with was rClone30. After 10 days of the first immunization, the chickens in all the groups were boosted in the same way as with the first immunization. At 3, 5, 7, 10, 14 and 21 days after the first immunization, blood samples were collected from the wing vein of the chickens. Three chickens from each group were euthanized and dissected to obtain the spleens on 3, 5, 7, and 14 days after the first immunization and to obtain the bursa, lungs, liver, thymus, and spleen on 35 days after the first immunization. For euthanasia, CO2 asphyxiation followed by a thoracotomy procedure was adopted as per American Veterinary Medical Association Guidelines (2013) [Citation20].
Anti-NDV and anti-IBDV antibody titers detection
At the 3, 5, 7, 14, and 21 days after the first immunization, blood samples of all chickens were collected and separated to obtain serum. The haemagglutination-inhibition (HI) test was used to detect the level of anti-NDV antibody [Citation18], while the ELISA technique was employed to detect the anti-IBDV antibody titre [Citation20].
Measurement of inflammatory cytokines in chicken’s serum by ELISA
Inflammatory cytokines levels in the serum samples were estimated using IL-4, GM-CSF, IL-1β and IFN-γ ELISA kits (Andy gene, China).
Flow cytometry (FCM) analysis
On the 3, 5, 7, 10, 14, and 21 days post immunization, all chicken blood samples were collected with anticoagulant tubes, followed by separation of the white blood cells. The leukocytes were incubated for 2 hours with the following antibodies: (1) FITC-labelled anti-chicken CD3 antibody and PE-labelled anti-chicken CD4 antibody, (2) FITC-labelled anti-chicken CD3 antibody and PE-labelled anti-chicken CD8a antibody, (3) PE-labelled anti-chicken Bu-1 antibody, and (4) PE-labelled anti-chicken MHC-II antibody, separately. Subsequently, the cells were analysed by FCM.
Analysis of the mRNA levels of cytokines by real-time PCR
At the 3, 5, 7, 10, 14, or 21 days post-immunization, spleen and white blood cells were collected. The total RNA of leukocytes and spleen was extracted using Trizol reagent (Invitrogen), and the cDNA was synthesized using M-MLV reverse transcriptase (Promega). The target gene was analysed using real-time PCR with iTaq SYBR Green SuperMix (Bio-Rad, Hercules, CA, USA). The primer sequences for IL-1β, IL-4, GM-CSF, IFN-γ, and β-actin are listed in .
Table 1. Primers used in the study.
Histopathological analysis
Three chickens were sacrificed in each group after 35 days of first immunization. Bursa, lung, liver, thymus, and spleen were collected and fixed in 4% paraformaldehyde solution for histopathological analysis. Thereafter, the tissues were embedded in paraffin, cut into thin sections after trimming, followed by staining with haematoxylin and eosin (HE) for gross and histological examination to evaluate the degree of tissue damage.
Statistical analysis
The multi-group one-way or two-way analysis of variance (ANOVA) was used to assess the significance of the differences between the experimental groups using GraphPad Prism 8.0 software. The significance value is reported at the p < 0.05 or p < 0.01 level.
Results
Rescue of recombinant viruses
Three recombinant plasmids pBrClone30-VP2L (P/M)-chGM-CSF (NP), pBrClone30-chGM-CSF (P/M)-VP2L (NP) and pBrClone30-VP2L (P/M)-chGM-CSF (P/M) were constructed successfully. Then, a reverse genetic operating system was utilized to rescue the infectious rClone30-VP2L (P/M)-chGM-CSF (NP), rClone30-chGM-CSF (P/M)-VP2L (NP), rClone30-VP2L-chGM-CSF (P/M), rClone30-chGM-CSF (P/M) and rClone30-VP2L (P/M) viruses. Later, these rescued viruses were passaged in embryonic eggs for two consecutive generations, resulting in HA values 27 or 28 for the recombinant viruses ().
Identification of recombinant viruses
The target fragments were amplified by RT-PCR using VP2L and chGM-CSF specific primers, respectively, and their sizes were consistent with expectations ()). The effective insertion of the VP2L and chGM-CSF genes into the NDV genome was confirmed by the DNAMAN comparison that revealed 100% nucleotide sequence homology with the original sequence.
Figure 2. Identification of recombinant viruses. (a) RT-pcr-based detection of VP2L gene inserted into recombinant viruses: lane M: DL2000 marker; lane 1: rClone30-VP2L (P/M) virus; lane 2: rClone30-chGM-csf (P/M)-VP2L (NP) virus; lane 3: rClone30-VP2L (p/m)-chGM-csf (NP) virus; lane 4: rClone30-VP2L-chGM-csf (P/M) virus; lane 5: negative control. (b) RT-pcr-based detection of GM-CSF gene inserted into recombinant viruses: lane M: DL2000 marker; lane 1: rClone30-VP2L-chGM-csf (P/M) virus; lane 2: rClone30-chGM-csf (P/M)-VP2L (NP) virus; lane 3: rClone30-VP2L (p/m)-chGM-csf (NP) virus; lane 4: rClone30-chGM-csf (P/M) virus; lane 5: negative control. (c) Western blot-based detection of VP2L gene expression in recombinant viruses: lane 1: rClone30-VP2L-chGM-csf (P/M) virus; lane 2: rClone30-VP2L (p/m)-chGM-csf (NP) virus; lane 3: rClone30-VP2L (p/m)-chGM-csf (NP); lane 4: rClone30-VP2L (P/M) virus; lane 5: rClone30 virus; lane 6: culture medium control. (d) Western blot-based detection of GM-CSF gene expression in recombinant viruses: lane 1: rClone30-VP2L-chGM-csf (P/M) virus; lane 2: rClone30- chGM-csf (P/M)-VP2L (NP) virus; lane 3: rClone30-VP2L (p/m)-chGM-csf (NP) virus; lane 4: rClone30-chGM-csf (P/M) virus; lane 5: rClone30 virus; lane 6: culture medium control.
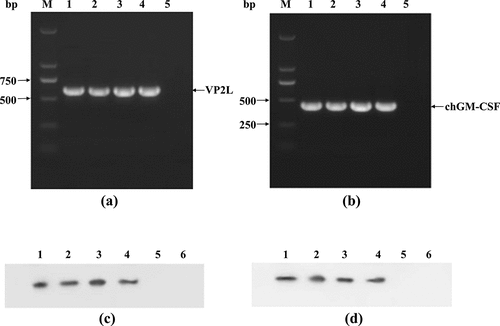
Western blot analysis results indicated that all the viruses with three different insertion sites, as well as rClone30-VP2L (P/M) virus, were able to express VP2L protein. While the viruses with three different insertion sites and rClone30-chGM-CSF (P/M) virus can express chGM-CSF protein ()). The findings demonstrated that the recombinant viruses can express VP2L or GM-CSF protein.
Comparison of viral titers and pathogenicity indexes between recombinant viruses and rClone30
The results showed that the HA of rClone30-VP2L (P/M)-chGM-CSF (NP), rClone30-VP2L (P/M), and rClone30 was found to be 28, whereas that of other viruses was 27. Moreover, the MDT of all viruses was greater than 120 h, and the ICPI results were 0.00, indicating that the pathogenicity of the recombinant viruses was not significantly different from that of the rClone30 virus. Further, the EID50 values of rClone30-VP2L (P/M)-chGM-CSF (NP), rClone30-chGM-CSF (P/M)-VP2L (NP), rClone30-VP2L-chGM-CSF (P/M), rClone30-chGM-CSF (P/M), rClone30-VP2L (P/M) and rClone30 were 10−7.5, 10−7.7, 10−7.7, 10−7.8, 10−8 and 10−8, respectively. Furthermore, the TCID50 values of rClone30-VP2L (P/M)-chGM-CSF (NP), rClone30-chGM-CSF (P/M)-VP2L (NP), rClone30-VP2L-chGM-CSF (P/M), rClone30-chGM-CSF (P/M), rClone30-VP2L (P/M), and rClone30 were 10−5.83, 10−5.9, 10−6.14, 10−5.36, 10−5.49 and 10−6.2, respectively.
Growth characteristics of recombinant viruses
The replication efficiency and growth curve of recombinant viruses was measured in DF1 cells with the parent rClone30 virus as control. The replication kinetics of recombinant viruses and the parent virus rClone30 were found to be similar to DF-1 cells ().
Immunization effect of biological adjuvant bivalent vaccines in SPF chickens
Measurement of anti-NDV and anti-IBDV antibody titer
The antibody levels of anti-NDV in the serum of each group were detected by HI test at 3, 5, 7, 10, 14, or 21 days after the first immunization. All the experimental groups reached to the peak level at 14 days and showed a downward trend at 21 days (). The levels of anti-NDV antibody in chickens of groups rClone30-VP2L (P/M)-chGM-CSF (NP), rClone30-chGM-CSF (P/M)-VP2L (NP), rClone30-VP2L-chGM-CSF (P/M), and rClone30-chGM-CSF (P/M) reached above the protective titre of 4 log2 at 7 days after the first immunization, while the chickens of groups commercial vaccine, rClone30-VP2L (P/M), rClone30 reached the protective titre above 4 log2 at 10 days after the first immunization. Further, the levels of anti-NDV antibody in the chickens of groups rClone30-VP2L (P/M)-chGM-CSF (NP), rClone30-chGM-CSF (P/M)-VP2L (NP), and rClone30-VP2L-chGM-CSF (P/M) were observed to be significantly higher than those of groups rClone30-chGM-CSF (P/M) (##p < 0.01 or #p < 0.05) or rClone30-VP2L (P/M) (**p < 0.01) on the 7, 14, or 21 days after the first immunization, which was significantly higher than those of group commercial vaccine (^^p < 0.01 or ^p < 0.05) at 7 days after the first immunization.
Figure 4. Detection of anti-ndv and anti-ibdv antibody titre. (a) The anti-ndv antibody in the serum of chickens at 3, 5, 7, 10, 14, and 21 days after the first immunization was determined by HI test. (b) The anti-ibdv antibody in the serum of chickens at 7, 10, 14, and 21 days after the first immunization was measured by ELISA. Values are expressed as mean ±SD; **p < 0.01 versus rClone30-VP2L (P/M) virus-treated SPF chickens; #p < 0.05, ##p < 0.01 versus rClone30-chGM-csf (P/M) virus-treated SPF chickens; ^p < 0.05, ^^p < 0.01 versus commercial vaccine-treated SPF chickens.
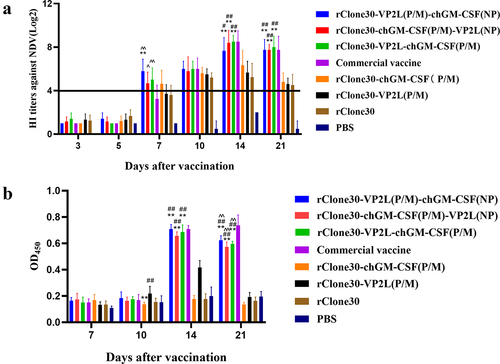
The serum levels of anti-IBDV antibodies in each group were detected by ELISA. Chickens in groups rClone30-chGM-CSF (P/M), rClone30, and PBS did not produce anti-IBDV antibodies. In contrast, antibody levels in chickens of the commercial vaccine group tended to rise 7–21 days after the first immunization, while in experimental groups, it showed an upward trend at 7–14 days, which started decreasing 21 days after the first immunization (). The antibody levels in chickens of groups rClone30-VP2L (P/M)-chGM-CSF (NP), rClone30-chGM-CSF (P/M)-VP2L (NP), and rClone30-VP2L-chGM-CSF (P/M) were significantly higher than those of group rClone30-VP2L (P/M) at 14 and 21 days (**p < 0.01). The antibody levels in the chickens of groups rClone30-VP2L (P/M)-chGM-CSF (NP), rClone30-chGM-CSF (P/M)-VP2L (NP), rClone30-VP2L-chGM-CSF (P/M) and commercial vaccine were found to be similar on 14 days, but the three experimental groups showed relatively lower value than the commercial vaccine group at 21 days (^^p < 0.01).
Detection of inflammatory cytokines in serum
At 3, 5, 7, 10, 14, and 21 days after the first immunization, IL-1β, IL-4, IFN-γ, and GM-CSF concentrations were measured in chicken serum samples using ELISA (). For 3–21 days after the first immunization, an increase in the levels of inflammatory cytokines was seen in the experimental groups. At 5 days after the first immunization, chickens in groups rClone30-VP2L (P/M)-chGM-CSF (NP) and rClone30-VP2L-chGM-CSF (P/M) exhibited significantly higher levels of IL-1β than the chickens in groups rClone30-VP2L (P/M) (**p < 0.01 or *p < 0.05) and commercial vaccine (^p < 0.05). At 7 days after the first immunization, chickens in groups rClone30-VP2L (P/M)-chGM-CSF (NP), rClone30-chGM-CSF (P/M)-VP2L (NP), and rClone30-VP2L-chGM-CSF (P/M) showed significantly higher concentrations of IL-1β, IL-4, or IFN-γ compared to chickens in groups (**p < 0.01 or *p < 0.05) or commercial vaccine (^p < 0.05). At 10, 14, and 21 days after the first immunization, chickens in groups rClone30-VP2L (P/M)-chGM-CSF (NP), rClone30-chGM-CSF (P/M)-VP2L (NP), and rClone30-VP2L-chGM-CSF (P/M) showed significantly higher concentrations of all four inflammatory cytokines than the chickens in group rClone30-VP2L (P/M) (**p < 0.01).
Figure 5. Detection of inflammatory cytokines in the serum. The levels of IL-1β, IL-4, ifn-γ, and GM-CSF in the serum at 3, 5, 7, 10, 14, and 21 days after the first immunization were determined by ELISA. The chickens of the rClone30-VP2L (P/M) group, rClone30-chGM-csf (P/M) group, and commercial vaccine group were used as control. Values are expressed as mean ±SD; *p < 0.05, **p < 0.01 versus rClone30-VP2L (P/M) virus-treated SPF chickens; #p < 0.05, ##p < 0.01 versus rClone30-chGM-csf (P/M) virus treated SPF chickens; ^p < 0.05 versus commercial vaccine-treated SPF chickens.
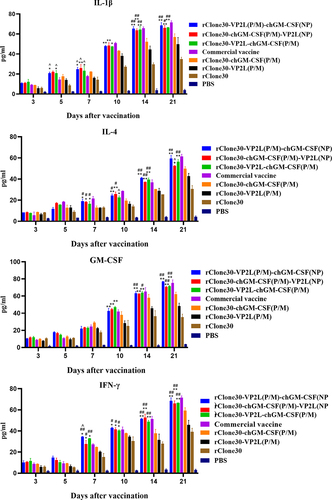
Immune response in immune cells
To assess the immune response, leukocytes were collected at 3, 5, 7, 10, 14, and 21 days after the first immunization, and the concentrations of CD4+ T, CD8+ T, B, and MHC-II+ cells in leukocytes were measured using FCM. The results showed that the percentages of CD4+ T, CD8+ T and MHC-II+ cells in each experimental group increased at 3–10 days and then showed a decreasing trend at 14–21 days after the first immunization (). Chickens in groups rClone30-VP2L (P/M)-chGM-CSF (NP), rClone30-chGM-CSF (P/M)-VP2L (NP), and rClone30-VP2L-chGM-CSF (P/M) showed significantly higher percentages of four immune cells than the chickens in rClone30-VP2L (P/M) group (**p < 0.01 or *p < 0.05). Moreover, at 7 days after the first immunization, chickens in groups rClone30-VP2L (P/M)-chGM-CSF (NP) and rClone30-VP2L-chGM-CSF (P/M) exhibited significantly higher percentages of B cells compared to the chickens in the group with the commercial vaccine (^p < 0.05). On 14 days after the first immunization, chickens in groups rClone30-VP2L-chGM-CSF (P/M) or rClone30-VP2L (P/M)-chGM-CSF (NP) displayed significantly higher percentages of CD4+ T, B or MHC-II+ cells than the chickens in group rClone30-chGM-CSF (P/M) (##p < 0.01 or ##p < 0.05). On 21 days after the first immunization, chickens in groups rClone30-VP2L (P/M)-chGM-CSF (NP) or rClone30-VP2L-chGM-CSF (P/M) demonstrated significantly higher percentages of B cells than the chickens in groups rClone30-chGM-CSF (P/M) (##p < 0.05) or rClone30-VP2L (P/M) (**p < 0.01).
Figure 6. Immune response in immune cells. The percentages of CD4+ T, CD8+ T, B, and MHC-II+ cells were determined by FCM at 3, 5, 7, 10, 14 and 21 days after the first immunization. The chickens of the rClone30-VP2L (P/M) group, rClone30-chGM-csf (P/M) group and commercial vaccine group were used as controls. Values are expressed as mean ±SD; *p < 0.05, **p < 0.01 versus rClone30-VP2L (P/M) virus-treated SPF chickens; #p < 0.05, ##p < 0.01 versus rClone30-chGM-csf (P/M) virus-treated SPF chickens; ^p < 0.05, ^^p < 0.01 versus commercial vaccine treated SPF chickens.
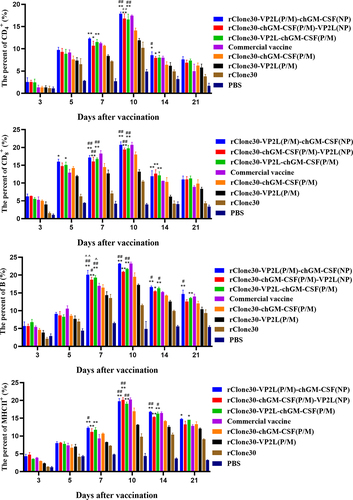
Inflammatory response of cells and tissues
At 3, 5, 7, 10, 14, and 21 days after the first immunization, inflammatory cytokines IL-1β, IL-4, IFN-γ, and GM-CSF in leukocytes were determined by real-time PCR. The levels of inflammatory factors of leukocytes increased in 3–7 days, which declined after 10 days in each group (). At 5, 7, or 10 days after the first immunization, chickens in groups rClone30-VP2L (P/M)-chGM-CSF (NP), rClone30-chGM-CSF (P/M)-VP2L (NP), and rClone30-VP2L-chGM-CSF (P/M) showed significantly higher levels of four inflammatory factors than the chickens in groups rClone30-chGM-CSF (P/M) (##p < 0.01 or #p < 0.05) or rClone30-VP2L (P/M) (**p < 0.01 or *p < 0.05). Further, at 14 or 21 days after the first immunization, chickens in groups rClone30-VP2L (P/M)-chGM-CSF (NP), rClone30-chGM-CSF (P/M)-VP2L (NP), and rClone30-VP2L-chGM-CSF (P/M) expressed significantly higher levels of IL-4, IL-1β or GM-CSF than the chickens in rClone30-VP2L (P/M) group (**p < 0.01 or *p < 0.05).
Figure 7. mRNA expression levels of inflammatory cytokines in white cells. The inflammatory cytokines IL-1β, ifn-γ, IL-4, and GM-CSF were analysed in the white cells by real-time PCR at 3, 5, 7, 10, 14, and 21 days post-first immunization. The chickens of the rClone30-VP2L (P/M) group, rClone30-chGM-csf (P/M) group, and commercial vaccine group were used as control. Values are expressed as mean ±SD; *p < 0.05, **p < 0.01 versus rClone30-VP2L (P/M) virus-treated SPF chickens; #p < 0.05, ##p < 0.01 versus rClone30-chGM-csf (P/M) virus-treated SPF chickens; ^p < 0.05 versus commercial vaccine-treated SPF chickens.
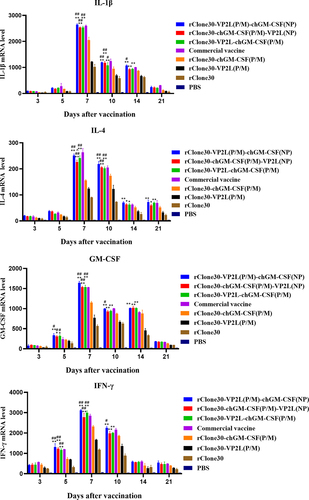
The inflammatory cytokines IL-1β, IL-4, IFN-γ, and GM-CSF were analysed in the spleen at 3, 5, 7, and 14 days after the first immunization by real-time PCR. Their levels were also found to be increased at 3–7 days, followed by a decline at 14 days in all groups (). At 5 or 7 days after the first immunization, chickens in groups rClone30-VP2L (P/M)-chGM-CSF (NP), rClone30-chGM-CSF (P/M)-VP2L (NP), and rClone30-VP2L-chGM-CSF (P/M) exhibited significantly higher levels of all the four inflammatory factors than the chickens in groups rClone30-chGM-CSF (P/M) (##p < 0.01 or #p < 0.05) and rClone30-VP2L (P/M) (**p < 0.01 or *p < 0.05). At 14 days after the first immunization, chickens in groups rClone30-VP2L (P/M)-chGM-CSF (NP), rClone30-chGM-CSF (P/M)-VP2L (NP), and rClone30-VP2L-chGM-CSF (P/M) showed significantly higher levels of GM-CSF or IL-1β than the chickens in rClone30-VP2L (P/M) group (*p < 0.05).
Figure 8. mRNA expression level of inflammatory cytokines in the spleen. The inflammatory cytokines IL-1β, IL-4, ifn-γ, and GM-CSF were analysed in the spleen by real-time PCR at 3, 5, 7, and 14 days post-first immunization. The chickens of the rClone30-VP2L (P/M) group, rClone30-chGM-csf (P/M) group, and commercial vaccine group were used as control. Values are expressed as mean ±SD; *p < 0.05, **p < 0.01 versus rClone30-VP2L (P/M) virus treated SPF chickens; #p < 0.05, ##p < 0.01 versus rClone30-chGM-csf (P/M) virus treated SPF chickens.
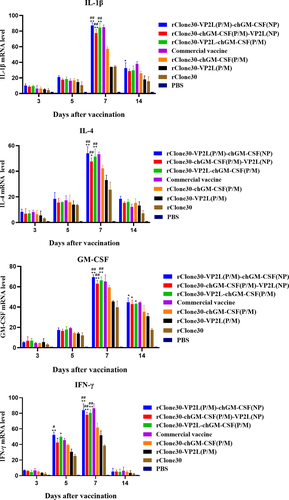
Histopathology
The histopathological analysis revealed no obvious pathological changes in the tissues of all group’s chickens at 35 days after the first immunization, indicating that these vaccines are safe ().
Figure 9. Histological structure of bursa, liver, lung, thymus, and spleen post immunization of SPF chickens. The bursa, liver, lung, thymus, and spleen samples were fixed by immersion in formalin, paraffin embedding, sectioned, and stained with haematoxylin and eosin. Histopathological changes were examined by light microscopy at a 200× field of view. Lane (a) PBS group; lane (b) rClone30 group; lane (c) rClone30-VP2L (P/M) group; lane (d) rClone30-chGM-csf (P/M) group; lane (e) rClone30-VP2L (p/m)-chGM-csf (NP) group; lane (f) rClone30-chGM-csf (P/M)-VP2L (NP) group; lane (g) rClone30-VP2L-chGM-csf (P/M) group; lane H: commercial vaccine group.
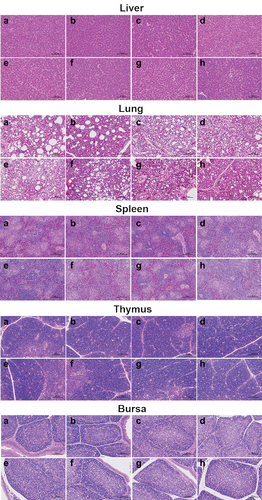
Discussion
NDV has been widely used as an effective vector system for transmitting other avian pathogens’ protective antigens as this vaccine expresses the foreign genes and induces robust immune responses at both local and systemic levels [Citation21]. Further, it has been demonstrated that NDV has great advantages as an exogenous gene expression vector [Citation22]. Since NDV can replicate in the cytoplasm without a DNA stage, it does not integrate into the host cell genome and thus provides enhanced safety as a vaccine [Citation23]. NDV live vector vaccine can choose chicken embryos to propagate viruses, thereby reducing the complicated steps of protein preparation, making it cost-effective and more suitable for industrial production. Some earlier studies have shown that different insertion sites greatly affect the expression of foreign genes [Citation9,Citation24,Citation25]. For example, HIV gag antigen gene insertion between the P and M sites of the NDV genome produced the best cellular immune response [Citation10,Citation26].
IBDV mainly infects young chickens of 3–6 weeks of age. The principal method to prevent IBDV is vaccination, and the live IBDV vaccines have been effective so far [Citation27]. However, these live attenuated IBDV vaccines may regain toxicity and change antigenicity [Citation28], and the moderately virulent vaccine can easily damage bursa in chickens leading to immunosuppression and thereby may affect the immunity of other vaccines such as ND and Marek’s disease [Citation29]. Therefore, there is an urgent need to develop a new vaccine that can effectively prevent IBD without causing excessive damage to the chickens.
The chimeric LaSota with genotype VII NDV F and HN genes expressing novel variant IBDV VP2, rLaS-VIIF/HN-VP2 was generated and provided complete protection against IBDV and virulent genotype VII NDV [Citation30]. The results showed that the NDV vector vaccine rLaS-VIIF/HN-VP2 was feasible and laid the foundation for this study. In this study, we compared the effects of recombination vaccines when the VP2L gene and the GM-CSF gene were inserted inside the NP gene, between the P/M genes, respectively, and when both were inserted between the P/M genes, followed by determination of the best insertion method of VP2L and GM-CSF genes. These genes were expressed in the NDV vector, which helps simplify the complicated steps of VP2L and GM-CSF protein preparation, purification, and metrology, and reducing the production cost and facilitating large-scale production. Furthermore, since the VP2L protein comprises only five neutralizing epitopes of VP2, it can be expressed more efficiently than the traditional VP2 protein. Moreover, it can avoid producing more IBDV variants because it doesn’t have the complete structure of IBDV. The biological adjuvant GM-CSF can stimulate the immune response strongly and rapidly in the vaccine and has no known side effects [Citation16,Citation31].
Recombinant viruses with different insertion sites were successfully rescued using reverse genetic technology, RT-PCR, and Western blot established that the VP2L and GM-CSF genes were successfully inserted and expressed. When recombinant viruses immunized the SPF chickens, compared with the rClone30-VP2L (P/M) vaccine group, the levels of anti-NDV antibody, anti-IBDV antibody, expression of four inflammatory factors, and the content of immune cells in white cells was found to be significantly increased in the biological adjuvant vaccines groups rClone30-VP2L (P/M)-chGM-CSF (NP), rClone30-chGM-CSF (P/M)-VP2L (NP) and rClone30-VP2L-chGM-CSF (P/M). These results indicate that biological adjuvant GM-CSF enhances the speed of immune response and efficacy of vaccines, which is consistent with previous research [Citation16–18]. The histopathological analysis reveals that the three biological adjuvants binary vaccine groups don’t illicit a strong tissue inflammatory response and tissue damage, which suggests their high safety profile.
Additionally, the IBDV antibody level analysis showed that the expression level of the rClone30-VP2L (P/M)-chGM-CSF (NP) vaccine is relatively higher than rClone30-chGM-CSF (P/M)-VP2L (NP) and rClone30-VP2L-chGM-CSF (P/M) vaccine. The results of HI revealed that the NDV antibody level of rClone30-VP2L (P/M)-chGM-CSF (NP) vaccine is higher than that of rClone30-chGM-CSF (P/M)-VP2L (NP) and rClone30-VP2L-chGM-CSF (P/M) vaccine at 5–10 days, however, after 14 days the rClone30-chGM-CSF (P/M)-VP2L (NP) vaccine stimulated a relatively higher NDV antibody level than those of rClone30-VP2L (P/M)-chGM-CSF (NP) vaccine. The analysis of inflammatory factors in spleen and leukocytes revealed that their levels of rClone30-VP2L (P/M)-chGM-CSF (NP) vaccine are higher than rClone30-chGM-CSF (P/M)-VP2L (NP) and rClone30-VP2L-chGM-CSF (P/M) vaccines. Serum inflammatory factors GM-CSF, IL-4, and IL-1β levels were also found to be higher for rClone30-chGM-CSF (P/M)-VP2L (NP) vaccine at 7–10 days and for rClone30-VP2L (P/M)-chGM-CSF (NP) vaccine at 14 days. Therefore, it can be concluded that insertion of a single foreign gene in the non-coding region between the P/M sites results in higher expression [Citation9], GM-CSF insertion at the P/M sites triggers a faster serum inflammatory response, while VP2L insertion at the P/M sites triggers a higher inflammatory and immune response. Thus, under the condition that the expression of the vector gene is not affected and the level of anti-NDV antibody reaches the protective titre, the three biological adjuvant binary vaccines may serve as candidate vaccines to respond to different needs. However, currently, NDV vector vaccine has not been applied on a large scale, probably because it takes too long to declare the drug number and how to improve its immunization effect is also important, and it will take some time for the NDV vector vaccine to be marketed.
In conclusion, the biological adjuvant bivalent vaccines significantly increase the level of NDV and IBDV antibodies, enabling SPF chickens to produce both humoral and cellular immune responses more quickly and effectively. Within 10 days after the first immunization, the levels of NDV antibodies, inflammatory factors, and immune cells were slightly higher in the three biological adjuvant bivalent vaccine group compared to the commercial vaccine group. This indicates that the three biological adjuvant combined vaccine could induce a faster immune response and NDV antibodies, thereby providing earlier protection for chickens. Additionally, the preparation process of the two vaccines with three types of biological adjuvants is simpler than that of commercial vaccines, reducing the preparation of VP2L and GM-CSF protein at a relatively lower cost; thus, facilitating large-scale factory production and use. Overall, the rClone30-VP2L (P/M)-chGM-CSF (NP) vaccine, which has an insertion of VP2L at the P/M site, is functionally better, and the developed three vaccines provide new options for the development of IBD and ND vaccines.
Author contributions statement
Wenying Sun, Xiaochen Guo and Shuang Li performed the experiments, analysed the results, and prepared the manuscript. Dun Niu, ZhiQiang Xue, Yunpeng Guo, Jinmiao Liu, Yijia Liu and Xinghao Jiang conducted the animal experiments. Ruihan Qin and Huimin Li improved the English grammar and language of the manuscript. Guiping Ren, Xiaochen Guo and Jiechao Yin designed the experiments. All the authors corrected and approved the final manuscript.
Disclosure statement
No potential conflict of interest was reported by the author(s).
Data availability statement
All data generated or analyzed during this study are deposited in figshare, DOI: https://doi.org/10.6084/m9.figshare.26134012.
Additional information
Funding
References
- Hu ZL, He XZ, Deng J, et al. Current situation and future direction of Newcastle disease vaccines. Vet Res. 2022;53(1):99. doi: 10.1186/s13567-022-01118-w
- Lupini C, Felice V, Silveira F, et al. Comparative in vivo pathogenicity study of an ITA genotype isolate (G6) of infectious bursal disease virus. Transbound Emerg Dis. 2020;67(2):1025–16. doi: 10.1111/tbed.13421
- Liu YQ, Sun CX, Chi MM, et al. Genetic characterization and phylogenetic analysis of Newcastle disease virus from China. Infect Genet Evol. 2019;75:103958. doi: 10.1016/j.meegid.2019.103958
- Choi KS. Newcastle disease virus vectored vaccines as bivalent or antigen delivery vaccines. Clin Exp Vaccine Res. 2017;6(2):72–82. doi: 10.7774/cevr.2017.6.2.72
- Duan Z, Xu H, Ji X, et al. Recombinant Newcastle disease virus-vectored vaccines against human and animal infectious diseases. Future Microbiol. 2015;10(8):1307–1323. doi: 10.2217/FMB.15.59
- De Swart RL, Belov GA. Advantages and challenges of Newcastle disease virus as a vector for respiratory mucosal vaccines. Curr Opin Virol. 2023;62:101348. doi: 10.1016/j.coviro.2023.101348
- Warner BM, Chan M, Tailor N, et al. Mucosal vaccination with a Newcastle disease virus-vectored vaccine reduces viral loads in SARS-CoV-2-infected cynomolgus macaques. Vaccines (Basel). 2024;12(4):404. doi: 10.3390/vaccines12040404
- Slamanig S, Gonzalez-Dominguez I, Chang LA, et al. Intranasal SARS-CoV-2 omicron variant vaccines elicit humoral and cellular mucosal immunity in female mice. EBioMedicine. 2024;105:105185. doi: 10.1016/j.ebiom.2024.105185
- Zhao W, Zhang ZY, Zsak L, et al. P and M gene junction is the optimal insertion site in Newcastle disease virus vaccine vector for foreign gene expression. J Gen Virol. 2015;96(1):40–45. doi: 10.1099/vir.0.068437-0
- Zhang ZY, Zhao W, Li DS, et al. Development of a Newcastle disease virus vector expressing a foreign gene through an internal ribosomal entry site provides direct proof for a sequential transcription mechanism. J Gen Virol. 2015;96(8):2028–2035. doi: 10.1099/vir.0.000142
- Li GP, Kuang HY, Guo HX, et al. Development of a recombinant VP2 vaccine for the prevention of novel variant strains of infectious bursal disease virus. Avian Pathol. 2020;49(6):557–571. doi: 10.1080/03079457.2020.1791314
- Huang ZH, Elankumaran S, Yunus AS, et al. A recombinant Newcastle disease virus (NDV) expressing VP2 protein of infectious bursal disease virus (IBDV) protects against NDV and IBDV. J Virol. 2004;78(18):10054–10063. doi: 10.1128/JVI.78.18.10054-10063.2004
- Guo XC, Sun WY, Wei L, et al. Development and evaluation of a recombinant VP2 neutralizing epitope antigen vaccine candidate for infectious bursal disease virus. Transbound Emerg Dis. 2021;68(6):3658–3675. doi: 10.1111/tbed.13974
- Hamilton JA. GM-CSF in inflammation. J Exp Med. 2020;217(1):e20190945. doi: 10.1084/jem.20190945
- Petrina M, Martin J, Basta S. Granulocyte macrophage colony-stimulating factor has come of age: from a vaccine adjuvant to antiviral immunotherapy. Cytokine Growth F R. 2021;59:101–110. doi: 10.1016/j.cytogfr.2021.01.001
- Guo XC, Zheng Q, Jiang XH, et al. The composite biological adjuvants enhance immune response of porcine circovirus type 2 vaccine. Vet Microbiol. 2019;228:69–76. doi: 10.1016/j.vetmic.2018.11.015
- Guo XC, Zhang T, Wang XX, et al. The immune enhancement effects of recombinant NDV expressing chicken granulocyte-macrophage colony stimulating factor on the different avian influenza vaccine subtypes. Transbound Emerg Dis. 2020;67(5):2108–2117. doi: 10.1111/tbed.13559
- Zhang TY, Liu YY, Wang H, et al. Recombinant NDV expressing cytokines or fliC confers a quick immune response against NDV challenge and resistance to maternal antibody. Vet Microbiol. 2016;196:107–117. doi: 10.1016/j.vetmic.2016.10.008
- Shirvani E, Paldurai A, Manoharan VK, et al. A recombinant Newcastle disease virus (NDV) expressing S protein of infectious bronchitis virus (IBV) protects chickens against IBV and NDV. Sci Rep. 2018;8(1):11951. doi: 10.1038/s41598-018-30356-2
- Sun WY, Cao XL, Wang YX, et al. Development and evaluation of a bivalent vaccine based on recombinant Newcastle disease virus expressing infectious bursal disease virus VP2L-CH3-CH4 in SPF chickens. Vet Microbiol. 2024;288:109950. doi: 10.1016/j.vetmic.2023.109950
- Fulber JPC, Kamen AA. Development and scalable production of Newcastle disease virus-vectored vaccines for human and veterinary use. Viruses-Basel. 2022;14(5):975. doi: 10.3390/v14050975
- Yan Y, Liu S, Li M, et al. Recombinant Newcastle disease virus expressing human ifn-λ1 (rL-hIFN-λ1)-induced apoptosis of A549 cells is connected to endoplasmic reticulum stress pathways. Thorac Cancer. 2018;9(11):1437–1452. doi: 10.1111/1759-7714.12857
- Olszewska-Tomczyk M, Dolka I, Swieton E, et al. Genetic changes in pigeon paramyxovirus type-1 induced by serial passages in chickens and microscopic lesions caused by the virus in various avian hosts. J Vet Res. 2018;62(4):447–455. doi: 10.2478/jvetres-2018-0059
- He L, Zhang ZY, Yu QZ. Expression of two foreign genes by a Newcastle disease virus vector from the optimal insertion sites through a combination of the ITU and IRES-dependent expression approaches. Front Microbiol. 2020;11:769. doi: 10.3389/fmicb.2020.00769
- Wang Y, Ge JY, Xie XD, et al. Impact of modification of cleavage site of fusion protein and foreign gene insertion on the virulence of Newcastle disease virus LaSota vaccine strain. Acta Microbiologica Sin. 2008;48(3):362–368.
- Carnero E, Li W, Borderia AV, et al. Optimization of human immunodeficiency virus gag expression by Newcastle disease virus vectors for the induction of potent immune responses. J Virol. 2009;83(2):584–597. doi: 10.1128/JVI.01443-08
- Müller H, Mundt E, Eterradossi N, et al. Current status of vaccines against infectious bursal disease. Avian Pathol. 2012;41(2):133–139. doi: 10.1080/03079457.2012.661403
- S DEY, Pathak DC, Ramamurthy N, et al. Infectious bursal disease virus in chickens: prevalence, impact, and management strategies. Vet Med-Res Rep. 2019;10:85–97. doi: 10.2147/VMRR.S185159
- Gimeno IM, Schat KA. Virus-induced immunosuppression in chickens. Avian Dis. 2018;62(3):272–285. doi: 10.1637/11841-041318-Review.1
- Qiao QL, Song MZ, Song CC, et al. Single-dose vaccination of recombinant chimeric Newcastle disease virus (NDV) LaSota vaccine strain expressing infectious bursal disease virus (IBDV) VP2 gene provides full protection against genotype VII NDV and IBDV challenge. Vaccines (Basel). 2021;9(12):1483. doi: 10.3390/vaccines9121483
- Zeshan B, Mushtaq MH, Wang XL, et al. Protective immune responses induced by in ovo immunization with recombinant adenoviruses expressing spike (S1) glycoprotein of infectious bronchitis virus fused/co-administered with granulocyte-macrophage colony stimulating factor. Vet Microbiol. 2010;148(1):8–17. doi: 10.1016/j.vetmic.2010.08.003