Abstract
The small GTPase ADP-ribosylation factor 6 (Arf6) plays important roles in membrane dynamics-based neuronal cell events such as neurite outgrowth and spine formation. However, physiological functions of Arf6 in the nervous system at whole animal level have not yet been explored. We have recently generated conditional knockout mice lacking Arf6 in neurons or oligodendrocytes of central nervous system (CNS) or both cell lineages, and analyzed them. We found that ablation of Arf6 gene from neurons, but not from oligodendrocytes, caused the defect in axon myelination at the fimbria of hippocampus (Fim) and corpus callosum (CC). We also found that migration of oligodendrocyte precursor cells (OPCs) from the subventricular zone to the Fim and CC in mice lacking Arf6 in neurons was impaired. Finally, it was found that secretion of fibroblast growth factor-2 (FGF-2), a guidance factor for OPC migration, from hippocampi lacking Arf6 was impaired. Collectively, these findings demonstrate that Arf6 in neurons of the CNS plays an important role in OPC migration by regulating secretion of FGF-2 from neurons, thereby contributing to the axon myelination. Here, we discuss our current understanding of physiological functions of Arf6 in the nervous system.
ADP-ribosylation factors (Arfs) are Arf subfamily members in the small GTPase Ras superfamily. Like other small GTPases, they functions as molecular switches in a wide variety of signal transduction systems by cycling between GDP-bound inactive and GTP-bound active forms, which is precisely regulated by the guanine nucleotide exchange factors (GEFs) and GTPase-activating proteins (GAPs). Arf subfamily is consisted of 6 isoforms, Arf1-6, which are classified into 3 classes based on their sequence similarity.Citation1 Class I includes Arf1, Arf2 and Arf3, class II Arf4 and Arf5, and class III Arf6. Class I and II of Arfs localize at the Golgi and endoplasmic reticulum, and primarily regulate vesicular trafficking between these intracellular organelles.Citation2,3 On the other hand, Arf6, the sole member of class III, predominantly localizes to the plasma membrane and endosomal compartments, and is involved in the membrane dynamics-related cellular events, including actin cytoskeleton reorganization, adherence junction formation, clathrin-mediated and -independent endocytosis, phagocytosis, exocytosis, and post-endocytic vesicle trafficking.Citation4-6 These cellular events regulated by Arf6 are closely related to physiological cell functions such as transcytosis in primary cultured kidney proximal tubule cells,Citation7 gastric acid secretion from parietal cells of the stomachCitation8 and insulin secretion from pancreatic β-cells.Citation9 Arf6 has also been implicated in pathogenesis with the invasion and metastasis of tumor cells.Citation10 Although these reports demonstrate that Arf6 is essential for a wide variety of physiological and pathophysiological cell functions, it is important to clarify which cellular functions regulated by Arf6 link to which physiological and pathophysiological functions at whole animal level. To address this question, we had prepared Arf6 knockout mice. However, full loss of Arf6 in mice results in embryonic lethality,Citation11 thereby preventing further analyses of the physiological and pathophysiological functions of Arf6. To overcome this problem, it is required to generate tissue- and cell type-specific Arf6 conditional knockout mice (Arf6-CKO mice).
Arf6 has been reported to play an important role in axonal outgrowth, dendritic branching and spine formation in primary cultured cortical and hippocampal neurons,Citation12-15 suggesting that Arf6 is essential for neuronal development in the central nervous system (CNS). However, in vivo roles of Arf6 in the CNS are still unclear. To address this issue, we have recently generated neural stem cell (NSC)-specific Arf6-CKO mice (NSC-Arf6-CKO mice), which did not show any obvious behavioral abnormality, by mating Arf6-flox/flox mice with Nestin-Cre mice.Citation16 In these mice, the fimbria of hippocampus (Fim) and corpus callosum (CC), which contain highly myelinated axon fibers, were smaller in their size than those of wild type mice. The transmission electron microscopy analyses revealed that the smaller size of these tissues in the NSC-Arf6-CKO mice were due to the defect in the myelination of axons. Myelination in other regions of the CNS, such as the fornix, cerebral peduncle, and in cerebellum and the sciatic nerve of the peripheral nervous system (PNS) was not affected by ablation of Arf6 from the brain. Thus, axon myelination regulated by Arf6 is dependent upon regions in the brain.
Myelin sheaths in the CNS are formed by oligodendrocytes which are derived from neural stem cells through oligodendrocyte precursor cells (OPCs): neuronal stem cells differentiate into OPCs at the ventricular zone of the lateral ventricles, then OPCs migrate to the target tissues such as the Fim and CC where they further differentiate into mature oligodendrocytes to form myelin sheaths. We found that the migration of OPCs from the ventricular zone to the Fim and CC was impaired in NSC-Arf6-CKO mice, suggesting that Arf6 is crucial for the OPC migration and the defect in axonal myelination in NSC-Arf6-CKO mice is attributable to the suppression of OPC migration.
In NSC-Arf6-CKO mice, Arf6 in both neurons and oligodendrocytes could be knocked-out. To determine in which type of cells Arf6 is required for OPC migration, we generated the neuron- and oligodendrocyte-specific Arf6-CKO mice (N-Arf6-CKO and O-Arf6-CKO mice, respectively), all of which did not show any obvious behavioral abnormality, by mating Arf6-flox/flox mice with Tau-Cre and CNP-Cre mice, respectively.Citation17,18 Surprisingly, N-Arf6-CKO mice, but not O-Arf6-CKO mice, showed the same phenotype as NSC-Arf6-CKO mice, demonstrating that Arf6 in neurons plays an important role in the myelin sheath formation by regulating OPC migration in the brain.
The migration of OPCs could be accurately regulated by guidance factors secreted from the target tissues of OPCs. Platelet-derived growth factor (PDGF), fibroblast growth factor-2 (FGF-2), Semaphorin 3A, epidermal growth factor (EGF), hepatocyte growth factor (HGF) and glutamate have been shown to promote OPC migration in in vitro systems,Citation19,20 which led us to speculate that some of these molecules would be released from neurons in an Arf6-dependent manner to regulate OPC migration in vivo. Supporting this hypothesis, OPC migration, which was elicited by co-culture with the hippocampal tissue in a Boyden chamber, was reduced when the hippocampi were prepared from N-Arf6-CKO mice. Thus, Arf6 appears to regulate production and/or secretion of a guidance molecule(s) for OPC migration in hippocampal neurons. Furthermore, we found that FGF-2, which is highly expressed in the hippocampus,Citation19,21 was expressed in N-Arf6-CKO hippocampal tissues at the comparable level to controls, but its secretion from cultured N-Arf6-CKO hippocampal tissues was significantly lower than that from control tissues. Thus, Arf6 at least in part plays an important role in the FGF-2 secretion from hippocampal neurons. Collectively, these results suggest that Arf6 regulates OPC migration through FGF-2 secretion during neuronal development of the CNS, thereby contributing to myelination of axons in the Fim and CC ().Citation22
Figure 1. Schematic model for trans-regulation of OPC migration and axon myelination by neuronal Arf6. OPCs derived from the neural stem cells in the ventricular zone migrate to the target tissues, the Fim and CC, in response to FGF-2 secreted from neurons in an Arf6-dependent manner. In the Fim and CC, OPCs mature into oligodendrocytes which in turn form myelin sheaths.
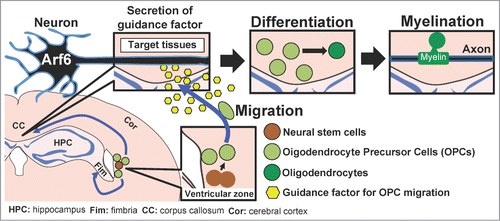
FGF-2 is secreted through the unconventional secretion pathway in which FGF-2 is directly translocated across the plasma membrane. In this pathway, phosphatidylinositol 4,5-bisphosphate (PI4,5P2) locally synthesized at the plasma membrane directly binds to FGF-2 to trigger its secretion.Citation23 Considering the critical function of Arf6 in the activation of PI4,5P2-synthesising enzyme phosphatidylinositol 4-phosphate 5-kinase (PIP5K),Citation6 Arf6 may control FGF-2 secretion through the PIP5K-PI4,5P2 pathway. Since Arf6 is also known to regulates dense core vesicle secretion, membrane vesicle shedding and membrane trafficking along the axon,Citation24-26 all of which are important for neural secretion of biologically active substances,Citation27-29 it is plausible that Arf6 is also involved in secretion of other guidance factors as well as FGF-2. The finding that the defect in myelination caused by Arf6 ablation was limited to the Fim and CC, however, indicates that different guidance factors utilize distinct molecular mechanisms for their secretion.
The myelinated axon fiber bundle at the Fim connects the hippocampus and subcortical brain regions. Because Fim lesion in rodents induces defects in the radial arm maze learning, the Morris water maze learning and homing behavior on the Barnes maze, Fim is thought to be involved in spatial working memory.Citation30-32 Myelinated axon fiber bundle at the CC connects 2 halves of the brain. Callosotomy, a surgical bisection of the CC in human, results in strange-hand syndrome, decrease in attention and difficulties in acquiring new interdependent bimanual skills.Citation33 In addition, it is well known that the myelin sheath is important in conducting signals in the complex neural network, and abnormal myelination causes psychotic symptoms such as schizophrenia and bipolar disorders.Citation34 Based on these reports, loss of Arf6 function in neuron may link to higher function disorders such as memory impairment and mental disorder. This assumption should be proved by behavioral experiments in future studies.
Our recent study demonstrated a new role of Arf6 in the axon myelination during neuronal development.Citation22 In that study, we employed CNP-Cre mice to produce O-Arf6-CKO mice which did not show any abnormality in axon myelination, suggesting that Arf6 in OPCs/oligodendrocytes is dispensable for myelination. Considering that efficiency of Cre-mediated recombination in OPCs is about 50%,Citation35 however, we cannot totally rule out the possibility that Arf6 expressed in OPCs/oligodendrocytes is also involved in myelination: if Arf6 in OPCs/oligodendrocytes is completely knocked out, defect in axon myelination may be induced. The developmental process of myelination including OPC migration, oligodendrocyte maturation and myelin sheath formation is closely related to dynamic morphological changes of these cells driven by actin cytoskeletal rearrangements.Citation36-38 Arf6 is well known to control actin cytoskeletal rearrangements by regulating PIP5K and Rac1 activity, thereby being involved in cell morphological changes.Citation4,39,40 It has been reported that Rac1 plays a key role in actin cytoskeletal rearrangements required for OPC migration and oligodendrocyte myelination.Citation41-43 In addition, knockdown of Arf6 in primary cultured OPCs inhibits morphological changes and expression of marker molecules for differentiated oligodendrocyte such as myelin basic protein.Citation44 Astrocytes have also been reported to be implicated in myelination by promoting OPC migration, proliferation, and differentiation through secretion of growth factors such as platelet-derived growth factor (PDGF), leukemia inhibitory factor (LIF) and FGF-2.Citation45 FGF-2 is expressed in both neurons and astrocytes during development, but explosively produced by astrocytes when the myelin of adult CNS is injoredCitation45. Thus, astrocytes may have an important role in re-myelination as well as myelin development, although it has not yet been clarified whether Arf6 is involved in FGF-2 secretion from astrocytes. To clarify functions of Arf6 in oligodendrocytes and astrocytes, further experiments are required.
In the PNS, myelin sheath is formed by Schwann cells, which change dynamically their membrane morphology during the process of myelination. In the PNS as well as CNS, Arf6 seems to be involved in myelination. This notion is supported by the report that knockdown of Arf6 in Schwann cells inhibits migration of Schwan cells as assessed by the Boyden chamber migration assay.Citation46 In addition, knockout mice of cytohesin-1, the guanine-nucleotide exchange factor (GEF) specific to Arf1 and Arf6, exhibit decrease in the thickness of myelin in sciatic nerves, accompanied by decreased levels of the active form of Arf6, but not that of Arf1.Citation47 Consistent with these reports, in vivo expression of the cytohesin-1 in Schwann cells of mice increases the thickness of the sciatic nerve myelin.Citation48 These reports strongly suggest the possibility that Arf6 in Schwann cells also play an important role in the developmental process of myelination during development of the PNS.
The myelinating cells such as oligodendrocytes and Schwann cells are involved in myelin growth (thickening myelin sheath) and maintenance (replenishing the myelin components that slowly turn over) throughout adult life. Interestingly, the FGF receptor signaling in oligodendrocytes is closely related to the regulation of growth and the maintenance of myelin.Citation49 Since N-Arf6-CKO mice show that the myelin sheath thickness is thinner than controls, Arf6 in neurons may be also involved in myelin growth and maintenance through secretion of FGF-2.
In conclusion, we demonstrated that Arf6 in hippocampal neurons regulates OPC migration by controlling the secretion of FGF-2, a guidance molecule for OPC migration, thereby being involved in myelination in the Fim and CC during development of the CNS. This finding provides insights into physiological functions of Arf6 in the CNS. In addition, we have reported that Arf6 is ubiquitously expressed in the tissues of adult mice.Citation50 To clarify the physiological functions of Arf6 in various tissues and organs at whole animal level, in vivo experiments in addition to in vitro experiments are required. To address this issue, we have generated several tissue- and cell type-specific Arf6 CKO mice, and revealed that Arf6 is involved in various physiological and pathophysiological processes such as tumor angiogenesis and growth, wound healing and spermatogenesis (unpublished data). Thus, Arf6 would be involved in various physiological and pathophysiological processes in a wide variety of organs and tissues. Such multiple Arf6 functions are due to the existence of various Arf6-specific GEFs (Arf6-GEFs) and -GAPs (Arf6-GAPs), which regulate cycling between the active and inactive forms of Arf6. It is likely that Arf6-GAPs as well as Arf6-GEFs are involved in Arf6-regulated cellular functions: the Arf6-GAP, TBC1D24, regulates neuronal migration and maturation through modulation of the Arf6-dependent pathway.Citation51 Further analyses are required to clarify whether existence of various Arf6-GEFs and -GAPs is attributable to the multi-functions of Arf6.
Disclosure of Potential Conflicts of Interest
No potential conflicts of interest were disclosed.
References
- Tsuchiya M, Price SR, Tsai SC, Moss J, Vaughan M. Molecular identification of ADP-ribosylation factor mRNAs and their expression in mammalian cells. J Biol Chem 1991; 266:2772-7; PMID:1993656
- Wieland F, Harter C. Mechanisms of vesicle formation: insights from the COP system. Curr Opin Cell Biol 1999; 11:440-6; PMID:10449336; http://dx.doi.org/10.1016/s0955-0674(99)80063-5
- Volpicelli-Daley LA, Li Y, Zhang CJ, Kahn RA. Isoform-selective effects of the depletion of ADP-ribosylation factors 1–5 on membrane traffic. Mol Biol Cell 2005; 16:4495-508; PMID: 16030262; http://dx.doi.org/10.1091/mbc.e04-12-1042
- D'Souza-Schorey C, Chavrier P. ARF proteins: roles in membrane traffic and beyond. Nat Rev Mol Cell Biol 2006; 7:347-58; PMID:16633337; http://dx.doi.org/10.1038/nrm1910
- Song J, Khachikian Z, Radhakrishna H, Donaldson JG. Localization of endogenous ARF6 to sites of cortical actin rearrangement and involvement of ARF6 in cell spreading. J Cell Sci 1998; 111(Pt 15):2257-67; PMID:9664047
- Honda A, Nogami M, Yokozeki T, Yamazaki M, Nakamura H, Watanabe H, Kawamoto K, Nakayama K, Morris AJ, Frohman MA, et al. Phosphatidylinositol 4-phosphate 5-kinase alpha is a downstream effector of the small G protein ARF6 in membrane ruffle formation. Cell 1999; 99:521-32; PMID:10589680; http://dx.doi.org/10.1016/s0092-8674(00)81540-8
- Wolff NA, Lee WK, Abouhamed M, Thevenod F. Role of ARF6 in internalization of metal-binding proteins, metallothionein and transferrin, and cadmium-metallothionein toxicity in kidney proximal tubule cells. Toxicol Appl Pharmacol 2008; 230:78-85; PMID:18353411; http://dx.doi.org/10.1016/j.taap.2008.02.008
- Matsukawa J, Nakayama K, Nagao T, Ichijo H, Urushidani T. Role of ADP-ribosylation factor 6 (ARF6) in gastric acid secretion. J Biol Chem 2003; 278:36470-5; PMID:12860984; http://dx.doi.org/10.1074/jbc.m305444200
- Lawrence JT, Birnbaum MJ. ADP-ribosylation factor 6 regulates insulin secretion through plasma membrane phosphatidylinositol 4,5-bisphosphate. Proc Natl Acad Sci U S A 2003; 100:13320-5; PMID:14585928; http://dx.doi.org/10.1073/pnas.2232129100
- Hashimoto S, Onodera Y, Hashimoto A, Tanaka M, Hamaguchi M, Yamada A, Sabe H. Requirement for Arf6 in breast cancer invasive activities. Proc Natl Acad Sci U S A 2004; 101:6647-52; PMID:15087504; http://dx.doi.org/10.1073/pnas.0401753101
- Suzuki T, Kanai Y, Hara T, Sasaki J, Sasaki T, Kohara M, Maehama T, Taya C, Shitara H, Yonekawa H, et al. Crucial role of the small GTPase ARF6 in hepatic cord formation during liver development. Mol Cell Biol 2006; 26:6149-56; PMID:16880525; http://dx.doi.org/10.1128/mcb.00298-06
- Krauss M, Kinuta M, Wenk MR, De Camilli P, Takei K, Haucke V. ARF6 stimulates clathrin/AP-2 recruitment to synaptic membranes by activating phosphatidylinositol phosphate kinase type Igamma. J Cell Biol 2003; 162:113-24; PMID:12847086; http://dx.doi.org/10.1083/jcb.200301006
- Hernandez-Deviez DJ, Roth MG, Casanova JE, Wilson JM. ARNO and ARF6 regulate axonal elongation and branching through downstream activation of phosphatidylinositol 4-phosphate 5-kinase alpha. Molecular biology of the cell 2004; 15:111-20; PMID:14565977; http://dx.doi.org/10.1091/mbc.e03-06-0410
- Choi S, Ko J, Lee JR, Lee HW, Kim K, Chung HS, Kim H, Kim E. ARF6 and EFA6A regulate the development and maintenance of dendritic spines. J Neurosc 2006; 26:4811-9; PMID:16672654; http://dx.doi.org/10.1523/jneurosci.4182-05.2006
- Jaworski J. ARF6 in the nervous system. Eur J Cell Biol 2007; 86:513-24; PMID:17559968; http://dx.doi.org/10.1016/j.ejcb.2007.04.007
- Isaka F, Ishibashi M, Taki W, Hashimoto N, Nakanishi S, Kageyama R. Ectopic expression of the bHLH gene Math1 disturbs neural development. Eur J Neurosci 1999; 11:2582-8; PMID:10383648; http://dx.doi.org/10.1046/j.1460-9568.1999.00699.x
- Muramatsu K, Hashimoto Y, Uemura T, Kunii M, Harada R, Sato T, Morikawa A, Harada A. Neuron-specific recombination by Cre recombinase inserted into the murine tau locus. Biochem Biophys Res Commun 2008; 370:419-23; PMID:18381201; http://dx.doi.org/10.1016/j.bbrc.2008.03.103
- Lappe-Siefke C, Goebbels S, Gravel M, Nicksch E, Lee J, Braun PE, Griffiths IR, Nave KA. Disruption of Cnp1 uncouples oligodendroglial functions in axonal support and myelination. Nat Genet 2003; 33:366-74; PMID:12590258; http://dx.doi.org/10.1038/ng1095
- de Castro F, Bribian A. The molecular orchestra of the migration of oligodendrocyte precursors during development. Brain Res Brain Res Rev 2005; 49:227-41; PMID:16111552; http://dx.doi.org/10.1038/ng1095
- Gudz TI, Komuro H, Macklin WB. Glutamate stimulates oligodendrocyte progenitor migration mediated via an alphav integrin/myelin proteolipid protein complex. J Neurosci 2006; 26:2458-66; PMID:16510724; http://dx.doi.org/10.1523/jneurosci.4054-05.2006
- Monfils MH, Driscoll I, Melvin NR, Kolb B. Differential expression of basic fibroblast growth factor-2 in the developing rat brain. Neuroscience 2006; 141:213-21; PMID:16677765; http://dx.doi.org/10.1523/jneurosci.4054-05.2006
- Akiyama M, Hasegawa H, Hongu T, Frohman MA, Harada A, Sakagami H, Kanaho Y. Trans-regulation of oligodendrocyte myelination by neurons through small GTPase Arf6-regulated secretion of fibroblast growth factor-2. Nat Commun 2014; 5:4744; PMID:25144208; http://dx.doi.org/10.1038/ncomms5744
- Steringer JP, Bleicken S, Andreas H, Zacherl S, Laussmann M, Temmerman K, Contreras FX, Bharat TA, Lechner J, Muller HM, et al. Phosphatidylinositol 4,5-bisphosphate (PI(4,5)P2)-dependent oligomerization of fibroblast growth factor 2 (FGF2) triggers the formation of a lipidic membrane pore implicated in unconventional secretion. J Biol Chem 2012; 287:27659-69; PMID:22730382; http://dx.doi.org/10.1074/jbc.m112.381939
- Aikawa Y, Martin TF. ARF6 regulates a plasma membrane pool of phosphatidylinositol(4,5)bisphosphate required for regulated exocytosis. J Cell Biol 2003; 162:647-59; PMID:12925709; http://dx.doi.org/10.1083/jcb.200212142
- Muralidharan-Chari V, Clancy J, Plou C, Romao M, Chavrier P, Raposo G, D'Souza-Schorey C. ARF6-regulated shedding of tumor cell-derived plasma membrane microvesicles. Curr Biol 2009; 19:1875-85; PMID:19896381; http://dx.doi.org/10.1016/j.cub.2009.09.059
- Eva R, Crisp S, Marland JR, Norman JC, Kanamarlapudi V, ffrench-Constant C, Fawcett JW. ARF6 directs axon transport and traffic of integrins and regulates axon growth in adult DRG neurons. J Neurosci 2012; 32:10352-64; PMID:22836268; http://dx.doi.org/10.1523/jneurosci.1409-12.2012
- Zhang X, Bao L, Ma GQ. Sorting of neuropeptides and neuropeptide receptors into secretory pathways. Progr Neurobiol 2010; 90:276-83; PMID:19853638; http://dx.doi.org/10.1016/j.pneurobio.2009.10.011
- Fruhbeis C, Frohlich D, Kuo WP, Kramer-Albers EM. Extracellular vesicles as mediators of neuron-glia communication. Front Cell Neurosci 2013; 7:182; PMID:24194697; http://dx.doi.org/10.3389/fncel.2013.00182
- de Wit J, Toonen RF, Verhaagen J, Verhage M. Vesicular trafficking of semaphorin 3A is activity-dependent and differs between axons and dendrites. Traffic 2006; 7:1060-77; PMID:16734664; http://dx.doi.org/10.1111/j.1600-0854.2006.00442.x
- Cassel JC, Duconseille E, Jeltsch H, Will B. The fimbria-fornix/cingular bundle pathways: a review of neurochemical and behavioural approaches using lesions and transplantation techniques. Prog Neurobiol 1997; 51:663-716; PMID:9175161; http://dx.doi.org/10.1016/s0301-0082(97)00009-9
- Gorny JH, Gorny B, Wallace DG, Whishaw IQ. Fimbria-fornix lesions disrupt the dead reckoning (homing) component of exploratory behavior in mice. Learn Mem 2002; 9:387-94; PMID:12464698; http://dx.doi.org/10.1101/lm.53002
- Cassel JC, Cassel S, Galani R, Kelche C, Will B, Jarrard L. Fimbria-fornix vs selective hippocampal lesions in rats: effects on locomotor activity and spatial learning and memory. Neurobiol Learn Mem 1998; 69:22-45; PMID:9521808; http://dx.doi.org/10.1006/nlme.1997.3807
- Wong CW. Corpus callosum and cerebral laterality in a modular brain model. Med Hypotheses 2000; 55:177-82; PMID:10904437; http://dx.doi.org/10.1054/mehy.1999.0934
- Mighdoll MI, Tao R, Kleinman JE, Hyde TM. Myelin, myelin-related disorders, and psychosis. Schizophr Res 2014; PMID:25449713; http://dx.doi.org/10.1016/j.schres.2014.09.040
- Ishii A, Furusho M, Bansal R. Sustained activation of ERK1/2 MAPK in oligodendrocytes and schwann cells enhances myelin growth and stimulates oligodendrocyte progenitor expansion. J Neurosci 2013; 33:175-86; PMID:23283332; http://dx.doi.org/10.1523/jneurosci.4403-12.2013
- Bacon C, Lakics V, Machesky L, Rumsby M. N-WASP regulates extension of filopodia and processes by oligodendrocyte progenitors, oligodendrocytes, and Schwann cells-implications for axon ensheathment at myelination. Glia 2007; 55:844-58; PMID:17405146; http://dx.doi.org/10.1002/glia.20505
- Miller RH. Regulation of oligodendrocyte development in the vertebrate CNS. Prog Neurobiol 2002; 67:451-67; PMID:12385864; http://dx.doi.org/10.1016/s0301-0082(02)00058-8
- Klambt C. Modes and regulation of glial migration in vertebrates and invertebrates. Nat Rev Neurosci 2009; 10:769-79; PMID:19773781; http://dx.doi.org/10.1038/nrn2720
- Kahn RA, Cherfils J, Elias M, Lovering RC, Munro S, Schurmann A. Nomenclature for the human Arf family of GTP-binding proteins: ARF, ARL, and SAR proteins. J Cell Biol 2006; 172:645-50; PMID:16505163; http://dx.doi.org/10.1083/jcb.200512057
- Donaldson JG, Jackson CL. ARF family G proteins and their regulators: roles in membrane transport, development and disease. Nat Rev Mol Cell Biol 2011; 12:362-75; PMID:21587297; http://dx.doi.org/10.1038/nrm3159
- Xiao L, Hu C, Yang W, Guo D, Li C, Shen W, Liu X, Aijun H, Dan W, He C. NMDA receptor couples Rac1-GEF Tiam1 to direct oligodendrocyte precursor cell migration. Glia 2013; 61:2078-99; PMID:24123220; http://dx.doi.org/10.1002/glia.22578
- Liang X, Draghi NA, Resh MD. Signaling from integrins to Fyn to Rho family GTPases regulates morphologic differentiation of oligodendrocytes. J Neurosci 2004; 24:7140-9; PMID:15306647; http://dx.doi.org/10.1523/jneurosci.5319-03.2004
- Thurnherr T, Benninger Y, Wu X, Chrostek A, Krause SM, Nave KA, Franklin RJ, Brakebusch C, Suter U, Relvas JB. Cdc42 and Rac1 signaling are both required for and act synergistically in the correct formation of myelin sheaths in the CNS. J Neurosci 2006; 26:10110-9; PMID:17021167; http://dx.doi.org/10.1523/jneurosci.2158-06.2006
- Miyamoto Y, Yamamori N, Torii T, Tanoue A, Yamauchi J. Rab35, acting through ACAP2 switching off Arf6, negatively regulates oligodendrocyte differentiation and myelination. Mol Biol Cell 2014; 25:1532-42; PMID:24600047; http://dx.doi.org/10.1091/mbc.e13-10-0600
- Moore CS, Abdullah SL, Brown A, Arulpragasam A, Crocker SJ. How factors secreted from astrocytes impact myelin repair. J Neurosci Res 2011; 89:13-21; PMID: 20857501; http://dx.doi.org/10.1002/jnr.22482
- Miyamoto Y, Torii T, Nakamura K, Takashima S, Sanbe A, Tanoue A, Yamauchi J. Signaling through Arf6 guanine-nucleotide exchange factor cytohesin-1 regulates migration in Schwann cells. Cell Signal 2013; 25:1379-87; PMID:23517829; http://dx.doi.org/10.1016/j.cellsig.2013.03.008
- Yamauchi J, Miyamoto Y, Torii T, Takashima S, Kondo K, Kawahara K, Nemoto N, Chan JR, Tsujimoto G, Tanoue A. Phosphorylation of cytohesin-1 by Fyn is required for initiation of myelination and the extent of myelination during development. Sci Signal 2012; 5:ra69; PMID:23012656; http://dx.doi.org/10.1126/scisignal.2002802
- Torii T, Miyamoto Y, Onami N, Tsumura H, Nemoto N, Kawahara K, Kato M, Kotera J, Nakamura K, Tanoue A, et al. In vivo expression of the Arf6 Guanine-nucleotide exchange factor cytohesin-1 in mice exhibits enhanced myelin thickness in nerves. J Mol Neurosci 2013; 51:522-31; PMID:23636892; http://dx.doi.org/10.1007/s12031-013-0018-4
- Furusho M, Dupree JL, Nave KA, Bansal R. Fibroblast growth factor receptor signaling in oligodendrocytes regulates myelin sheath thickness. J Neurosci 2012; 32:6631-41; PMID:22573685; http://dx.doi.org/ 10.1523/jneurosci.6005-11.2012
- Akiyama M, Zhou M, Sugimoto R, Hongu T, Furuya M, Funakoshi Y, Kato M, Hasegawa H, Kanaho Y. Tissue- and development-dependent expression of the small GTPase Arf6 in mice. Dev Dyn 2010; 239:3416-35; PMID:21069828; http://dx.doi.org/10.1002/dvdy.22481
- Falace A, Buhler E, Fadda M, Watrin F, Lippiello P, Pallesi-Pocachard E, Baldelli P, Benfenati F, Zara F, Represa A, et al. TBC1D24 regulates neuronal migration and maturation through modulation of the ARF6-dependent pathway. Proc Natl Acad Sci U S A 2014; 111:2337-42; PMID:24469796; http://dx.doi.org/10.1073/pnas.1316294111