ABSTRACT
RhoH is a haematopoietic -specific, GTPase-deficient Rho GTPase that plays an essential role in T lymphocyte development and haematopoietic cell migration. RhoH is known to interact with ZAP70 in T cell receptor (TCR) signaling and antagonize Rac GTPase activity. To further elucidate the molecular mechanisms of RhoH in T cell function, we carried out in vivo biotinylation and mass spectrometry analysis to identify new RhoH-interacting proteins in Jurkat T cells. We indentified Kaiso by streptavidin capture and confirmed the interaction with RhoH by co-immunoprecipitation. Kaiso is a 95 kDa dual-specific Broad complex, Trantrak, Bric-a-brac/Pox virus, Zinc finger (POZ-ZF) transcription factor that has been shown to regulate both gene expression and p120 catenin-associated cell-cell adhesions. We further showed that RhoH, Kaiso and p120 catenin all co-localize at chemokine-induced actin-containing cell protrusion sites. Using RhoH knockdown we demonstrated that Kaiso localization depends on RhoH function. Similar to the effect of RhoH deficiency, Kaiso down-regulation led to altered cell migration and actin-polymerization in chemokine stimulated Jurkat cells. Interestingly, RhoH and Kaiso also co-localized to the nucleus in a time-dependent fashion after chemokine stimulation and with T cell receptor activation where RhoH is required for Kaiso localization. Based on these results and previous studies, we propose that extracellular microenvironment signals regulate RhoH and Kaiso to modulate actin-cytoskeleton structure and transcriptional activity during T cell migration.
Introduction
RhoH is a haematopoietic-specific atypical Rho GTPase that was initially identified as an oncogene fusion partner and target for somatic hypermutation in various B cell malignancies.Citation1-3 The 5′-noncoding region and first intron of the RHOH gene is mutated in a variety of B-lymphoid malignancies such as diffuse large B-cell lymphomas, AIDS-related non-Hodgkin's lymphoma and primary central nervous system lymphomas. The biological relevance of these mutations is yet unknown. However, a recent report strongly implicates a loss-of-function mutation in the coding sequence of RHOH in Epidermodysplasia verruciformis, a disease associated with disseminated cutaneous warts and non-melanotic skin cancer.Citation4 In those patients RhoH deficiency was associated with decreased skin-homing T lymphocytes. RhoH is also upregulated in chronic lymphocytic leukemia (CLL) in humans and deficiency of RhoH attenuates the disease phenotype of a CLL-like murine model.Citation5,6 In contrast, low RhoH expression has been shown to be a negative prognostic indicator in acute myeloid leukemia (AML),Citation7 which may be related to its role in regulating the expression of p21 and p27 as observed in THP-1 cells, a model of AML.Citation8
RhoH, harboring amino acid substitutions at codons 12, 13, 59 and 61 (based on Ras amino acid sequence numbering), which are analogous to mutations in oncogenic Ras proteins, is a constitutively active, GTP-bound proteinCitation9-12 and its activity is regulated in part by the level of protein expression and posttranslational modifications. Due to the pivotal role of RhoH in T cell receptor (TCR) signaling and thymocyte selection, Rhoh−/− mice are immunodeficient.Citation13,14 RhoH has been shown to directly interact with ZAP70 regulating its phosphorylation and recruitment to the immune synapse.Citation15 In addition, recent large scale proteomic analysis of TCR- and BCR-induced tyrosine phosphorylation has revealed that interactions between RhoH and α- and β-tubulin and/or PLCγ2 may direct the translocation of both the immunoreceptor-associated tyrosine kinases SYK and ZAP70.Citation16,17 RhoH function is also required for chemokine induced cell migration.Citation18 On a molecular level, RhoH modulates actin cytoskeleton structures influencing Rac activity and membrane localization in haematopoietic stem / progenitor cells (HSC / P) and leukemia cellsCitation6,19 and RhoH mutated Jurkat cells show enhanced adhesion via activation of LFA-1.Citation20 Thus, it is possible that RhoH regulates the balance of Rac and RhoA function during actin cytoskeleton rearrangement in the context of chemokine induced cell migration, immune synapse formation, and T cell maturation.Citation21 However, the molecular mechanism of these processes is still not fully understood.
Kaiso is a 95 kDa dual-specific Broad complex, Trantrak, Bric-a-brac/Pox virus, Zinc finger (POZ-ZF) transcription factor that associates with p120 catenin. Both Kaiso and p120 catenin are associated with E-cadherin and this complex is required for regulation of cell adhesion by modulating Rho GTPase activity and actin cytoskeleton structures downstream of extracellular signals. Due to its interaction with p120 catenin, Kaiso has also been implicated in regulation of tumor cell invasion.Citation22 In addition, Kaiso is recruited into the nucleus where it represses gene expression by binding to methylated CpG island motifsCitation23 and to the histone deacetylase (HDAC)-nuclear receptor co-repressor complex.Citation24 TCR-induced β-catenin activation results in WNT-dependent gene expression and based on promoter sequences WNT is considered a potential candidate gene for regulation by Kaiso. In summary, in response to extracellular environment signals, Kaiso is considered to both regulate the actin cytoskeleton at the cell membrane and in the cytosol and modulate gene expression in the nucleus.
To further delineate possible mechanisms of RhoH function in T cells, we carried out in vivo biotinylation and mass spectrometry analysis to identify RhoH associated molecules in the Jurkat T cell line. We identified Kaiso as a novel RhoH interacting protein and observed a multiprotein complex that regulates nuclear and cytosolic localization of both Kaiso and RhoH after chemokine stimulation in these cells. We further demonstrate that Kaiso is required for RhoH-associated Rac attenuation and modulation of chemokine-induced Jurkat cell migration. In addition, we show that TCR activation-induced nuclear localization of Kaiso requires RhoH function. Thus, we demonstrate that RhoH participates in a multi-protein complex that regulates both T cell cytoskeletal structures modulating cell migration, cell shape and adhesion via Rac GTPases, p120 catenin and Kaiso as well as nuclear localization of Kaiso.
Results
Isolation of kaiso as rhoh-interacting protein
To investigate new molecular mechanisms by which RhoH regulates T cell functions, we performed experiments to isolate RhoH-interacting proteins in Jurkat cells. To this end we utilized metabolic biotin tagging of recombinant RhoH protein by co-transducing Jurkat cells with lentivirus vectors expressing E. coli biotin ligase BirA (LeGO-iV2-Bir) and the RhoH cDNA containing a 23-amino-acid BirA recognition motif fused to its N terminus (LeGO-iG2-RhoH) or a lentivirus expressing the BirA alone (LeGO-iV2-Bir) as a control (Figure S1). Streptavidin affinity purification was performed on cell lysates harvested from stably transduced and sorted cells and subsequently co-purified proteins were separated by one-dimensional SDS-PAGE and applied to mass spectrometry analysis. The resulting SDS-PAGE lane was analyzed by microcapillary liquid chromatography/tandem mass spectrometry and RhoH-associated proteins were identified using SEQUEST software. We categorized identified proteins from 3 independent experiments that were specifically isolated in BirA/RhoH co-expressing Jurkat cells in all experiments as positive hits. Ten peptides derived from Kaiso (ZBTB33) were identified as interacting molecules with RhoH by mass spectrometry (Figure S2). ZAP70 and LCK were identified as interacting proteins (data not shown) confirming previous reports and validating this approach to isolating RhoH associated molecules from Jurkat cells.Citation15,18
We first confirmed the interaction of RhoH and Kaiso in immunoprecipitation (IP) experiments. Jurkat cells transduced with a retroviral vector expressing HA-RhoH (Figure S3) were subjected to IP with anti-HA monoclonal antibody or IgG at identical concentrations and analyzed by western blotting using anti-Kaiso monoclonal antibody. Kaiso was co-immunoprecipitated with RhoH using an anti-HA monoclonal antibody but not with control IgG (). No protein was immunoprecipitated from Jurkat cells transduced with the MIEG3 control vector (data not shown). This result showed that RhoH and Kaiso form a complex in Jurkat cells.
Figure 1. RhoH and Kaiso interact and co-localize in Jurkat T cells. (A) Interaction of HA-RhoH and the Kaiso complex were confirmed by co-immunoprecipitation. Jurkat cells transduced with retroviral vectors expressing HA-RhoH-IRES-YFP were subjected to IP with anti-HA monoclonal antibody and analyzed by immunoblotting with anti-Kaiso monoclonal antibody. Position of interacting Kaiso is indicated by arrowhead. IgG control is shown in left lane. Size marker is shown on right. A representative immunoblot of 3 independent experiments that gave similar results is shown. (B) Co-localization of RhoH and Kaiso in Jurkat cells. Jurkat cells were stained with polyclonal anti-RhoH antibody (green), monoclonal anti-Kaiso antibody (red) and nuclear staining with DAPI (blue). Arrowheads indicate co-localization readily apparent in cytosol and areas of cell protrusion. Cell Images were captured by Zeiss LSM 700 Laser Scanning Confocal-Microscope with 63X magnification and processed by ZEN software (Zeiss). Panels on the right show higher magnification (126X) of cell outlined by box in lower magnification veiw. This result represents 3 independent experiments. Mouse IgG and rabbit IgG were used as negative controls respectively and showed no signals (data not shown).
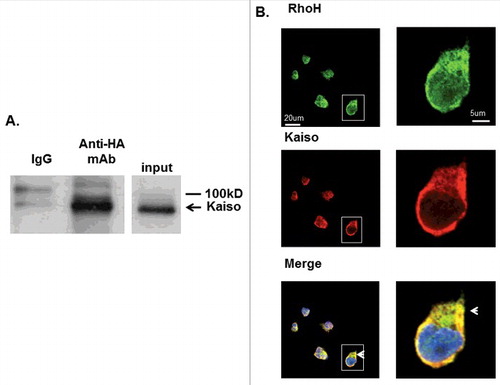
RhoH and kaiso co-localize in jurkat cells
To further investigate the co-localization of RhoH and Kaiso in Jurkat cells the cells were stained with anti-Kaiso monoclonal antibody and RhoH polyclonal antibody using fluorescence-coupled secondary antibodies and resulting images were analyzed using confocal microscopy on a single confocal plane. We observed that RhoH and Kaiso co-localized in the nucleus, cytosol, and particularly at the cell membrane in Jurkat cells (), suggesting that RhoH and Kaiso could regulate cell functions in multiple cellular domains. We detected no signals with mouse IgG, rabbit IgG or secondary antibodies alone (data not shown).
We have previously implicated RhoH in modulation of Rac GTPase activation and chemokine-induced cell migration in haematopoietic cells. To address the functional significance of RhoH and Kaiso interaction in Jurkat cells, we next examined the effect of chemokine stimulation on RhoH and Kaiso co-localization. Jurkat cells were stimulated with SDF-1 for up to 15 minutes, stained with anti-RhoH polyclonal antibody and anti-Kaiso monoclonal antibody and examined by confocal microscopy in a single confocal plane (). The RhoH antibody was validated by the decrease of RhoH signal in cells transdued with RhoHshRNAs (Figure S5C). As early as 30 seconds after SDF-1 stimulation we found Kaiso and RhoH co-localized at surface protrusion sites (). By 2 minutes after SDF-1 stimulation RhoH and Kaiso started to co-localize in the nucleus. This nuclear co-localization increased up to 15 minutes after stimulation (). To confirm RhoH localization, Jurkat cells were transduced with a vector expressing EGFP-RhoH (Figure S3) and then stained with anti-Kaiso. EGFP-RhoH and Kaiso localization were examined by confocal microscopy in a single confocal plane. We confirmed EGFP-RhoH and Kaiso co-localization at chemokine induced cell protrusion sites at 2 minutes after SDF-1 stimulation and EGFP-RhoH and Kaiso co-localization at 15 minutes after chemokine stimulation in the nucleus further supporting the observation in (Figure S4). We noted significant staining of RhoH that remained at the cell periphery at 15 minutes post stimulation.
Figure 2. SDF-induced Kaiso co-localization in cell protrusions is RhoH dependent. (A) Co-localization of RhoH and Kaiso at cell protrusion sites and nucleus after SDF-1 stimulation. Jurkat cells were stimulated with SDF-1 (200 ng/ml) for the indicated time points, fixed and stained with polyclonal anti-RhoH antibody (green), monoclonal anti-Kaiso antibody (red) and nuclear staining with DAPI (blue). Cell images were captured by Zeiss LSM 700 Laser Scanning Confocal-Microscope with 63X magnification and processed by ZEN software (Zeiss). RhoH and Kaiso co-localized at cell protrusion sites 30 sec. after SDF-1 stimulation (indicated by arrow) then started to co-localize in the cytosol and nucleus by 2 min. This shift in localization increased over 15 min (indicated by arrowhead) with a concomitant reduction in membrane localization. The higher magnification images of 15 minutes stimulation are shown at bottom column. Represenative images of 3 independent experiments are shown. (B) Cell fractionation and immunoprecipitation of HA-RhoH and Kaiso complex after SDF-1 stimulation. Jurkat cells transduced with a retrovirus expressing HA-RhoH-IRES-YFP were stimulated with SDF-1 (200 ng/ml) for the indicated time points. Subsequently cells were separated into nuclear, cytosolic fractions. The cell lysates from each fraction were subjected to IP with anti-HA monoclonal antibody and analyzed by immunoblotting with anti-Kaiso monoclonal antibody. HA-RhoH transduced Jurkat cells were stimulated with SDF-1 (200ng/ml) for the times indicated and fractionated into nuclear and cytosolic fractions. The HA-immunoprecipitated fractions (20ug) were analyzed for purity by immune blotting with anti-GAPDH antibody (cytosol) and anti-Histone polyclonal antibody (nuclear). A representative example of 3 independent experiments is shown. Abbreviations: c, unstimulated control. (C) RhoH knockdown is associated with the loss of Kaiso localization at SDF-1-induced cell protrusion sites. Jurkat cells transduced with scrambled control or RhoH shRNA were stimulated with SDF-1 (200 ng/ml) for 2 minutes. Stimulated cells were stained with monoclonal anti-Kaiso antibody (purple), rhodamine-phalloidin (red) to detect F-actin and nuclear DAPI staining (blue). Cell images were captured by Zeiss LSM 700 Laser Scanning Confocal-Microscope with 63X magnification and processed by ZEN software (Zeiss). Note decreased intensity of cortical Kaiso staining and increase in F-action at cell protrusion sites (indicated by arrowheads) following SDF-stimulation in RhoH knockdown cells.
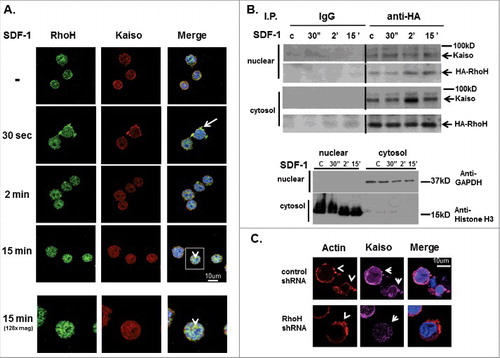
Next, to confirm the time-dependent co-localization of RhoH and Kaiso observed by confocal microscopy, we carried out IP experiments using subcellular fractions of RhoH-expressing Jurkat cells. Jurkat cells transduced with retrovirus expressing HA-RhoH were stimulated with SDF-1 for up to 15 minutes and subsequently separated into nuclear and cytosolic fractions. The purity of each subcellular fraction was confirmed by immunoblotting using specific nuclear and cytoplasmic markers (). Equal amounts of cell lysates from each fraction obtained at the indicated time points were subjected to IP with anti-HA monoclonal antibody or IgG as control and analyzed by immunoblotting with anti-Kaiso monoclonal antibody. At 30 seconds, 2 minutes and 15 minutes after SDF-1 stimulation, RhoH and Kaiso form a complex in cytosolic and nuclear fractions (). These results confirm confocal-microscopy studies showing co-localization of RhoH and Kaiso in the cytosol and nucleus with SDF-1 stimulation.
RhoH knockdown is associated with loss of kaiso localization at SDF-1 induced cell protrusions
We next studied the effect of RhoH knockdown on actin-containing surface protrusions induced by SDF-1 in Jurkat cells. Effective RhoH knockdown using RhoH targeting shRNA was confirmed by qPCR (Figure S5B) and immunofluorescence microscopy (Figure S5C). SDF-1 treated Jurkat cells transduced with control or RhoH shRNA vectors were stained with anti-Kaiso monoclonal antibody and rhodamine-phalloidin to detect F-actin and then analyzed by confocal microscopy in a single confocal plane. We observed an increase of cells exhibiting actin-containing surface protrusions after RhoH knockdown compared with control cells (). Next, the localization of Kaiso to actin-containing surface protrusions was observed in these cells. We noticed decreased intensity of cortical Kaiso staining () that was associated with an increase in F-actin after RhoH knockdown. To further investigate the requirement of RhoH for SDF-1 induced Kaiso localization at cell protrusion sites in more physiological conditions, we isolated T cells from spleens of wildtype and Rhoh−/− mice and stimulated them with SDF-1. Subsequently, the cells were stained with anti-Kaiso monoclonal antibody and DAPI and analyzed by confocal microscopy in a single confocal plane. By 30 seconds after SDF-1 stimulation, we observed polarized Kaiso localization at SDF-1 induced cell protrusion sites in T cells isolated from wildtype mouse spleens () but non-polarized diffuse Kaiso localization at the membrane () in T cells isolated from Rhoh−/− mouse spleens. We further noted nuclear localization of Kaiso 15 minutes after SDF-1 stimulation in T cells isolated from wildtype mice () that appeared less pronounced in T cells isolated from Rhoh−/− mice (). These data confirm that Kaiso localization at chemokine-induced actin-containing surface protrusion sites and translocation to the nucleus requires RhoH.
Figure 3. Sequential SDF-1 induced Kaiso localization at cell protrusion sites and in nucleus requires RhoH function in primary T cells of wildtype and Rhoh−/− mouse spleens. (A) T cells isolated from wildtype (WT) and Rhoh−/− mouse spleens were stimulated with SDF-1 (200ng/ml) for 30 seconds and stained with anti-Kaiso monoclonal antibody (red) and nuclear DAPI (blue). (B) Isolated T cells were stimulated with SDF-1 (200ng/ml) for 15 minutes and stained with anti-Kaiso monoclonal antibody (red) and nuclear DAPI (blue). Cell images were captured by Zeiss LSM 700 Laser Scanning Confocal-Microscope with 63X magnification and processed by ZEN software (Zeiss). Top row is WT and lower row is Rhoh−/− T cells.
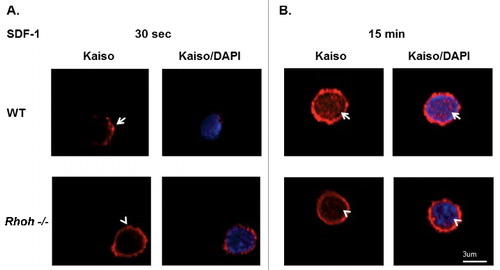
Knockdown of kaiso is associated with increased rac activation
RhoH is known to antagonize Rac signaling in Jurkat cells and Rac activation and membrane localization in haematopoietic stem and progenitor cells.Citation11,19 We next studied the effect of Kaiso knockdown on Rac activation. Jurkat cells transduced with control or Kaiso shRNA were stimulated with SDF-1 for 30 seconds and GTP-bound (active) Rac in cell lysates was determined by PBD-pull down assay. Kaiso knockdown was confirmed by qPCR (Figure S7B) and immunoblot (Figure S7C). Kaiso knockdown did not affect RhoH expression as assessed by qPCR (data not shown). As seen in , knockdown of Kaiso leads to dramatically increased levels of GTP-bound Rac at baseline (). However, in contrast to control cells, an additional increase in GTP-bound Rac was not seen with SDF-1 stimulation after Kaiso knockdown (). These data suggest that Kaiso plays a role in the regulation of Rac activation.
Figure 4. Knockdown of Kaiso is associated with constitutive activation of Rac. GTP-bound (active) Rac is increased at baseline and fails to respond to SDF-1 stimulation after knockdown of Kaiso in Jurkat cells. Rac activity was assessed by PBD pull-down assays and compared to total Rac protein in lysates from Jurkat cells transduced with control or Kaiso shRNA lentivirus and stimulated with SDF-1 (200 ng/ml) for 2 minutes. A representative immunoblot of 3 independent experiments is shown.
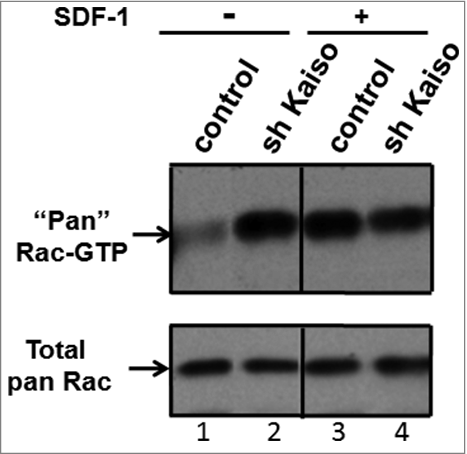
RhoH and kaiso down-regulate f-actin assembly and cell migration
We previously reported that RhoH regulates F-actin polymerization by antagonizing membrane localization of Rac GTPases in haematopoietic cells.Citation19 To address the potential involvement of Kaiso in regulation of F-actin polymerization, we determined the F-actin content in Jurkat cells with and without knockdown of Kaiso. Control or Kaiso shRNA transduced Jurkat cells were stained with rhodamine-phalloidin to detect F-actin and then captured by confocal microscopy and analyzed using ImageJ software. Similar to what we have reported previously in RhoH-deficient haematopoietic cells, we observed enhanced F-actin staining intensity () and a significant increase of lamellipodia formation () in Kaiso shRNA transduced Jurkat cells within 2 minutes SDF stimulation. These results suggest that RhoH and Kaiso may function in the same pathway to regulate SDF-1 induced F-actin polymerization most likely through the attenuation of Rac activation.
Figure 5. Kaiso regulates F-actin assembly and cell migration. (A) Kaiso knockdown in Jurkat cells leads to enhanced SDF-1 induced actin-polymerization. Control and Kaiso shRNA transduced Jurkat cells were stained with rhodamine-phalloidin (red) to detect F-actin and nuclear DAPI staining (blue). Cell images were captured by Zeiss LSM 700 Laser Scanning Confocal-Microscope with 63X magnification and processed by ZEN software (Zeiss). (B) Quantification confirmed increased polymerization of F-actin in Kaiso knockdown Jurkat cells. F-actin staining intensities in Kaiso compared with control shRNA transduced Jurkat cells after 2 min. SDF-1 (200 ng/ml) stimulation were quantified using ImageJ software. Y-axis represents the intensity of actin staining per cell. Data represent mean+/− SD (N = 10), **P < 0.01. (C) Enhanced SDF-1 induced cell migration of Jurkat cells after Kaiso knockdown. Jurkat cells transduced with lentivirus expressing control and Kaiso shRNA were subjected to transwell migration assays without (left)/with (right) 100 ng/ml SDF-1 as chemoattractant in the lower chamber. Cells in the lower chambers were counted after 3 hours incubation. Ratios of migrating cells/ input cells are shown as percentage migrating cells. Data represent mean +/− SD (N = 10), **P<0.01. Experiments were repeated 3 times for each condition.
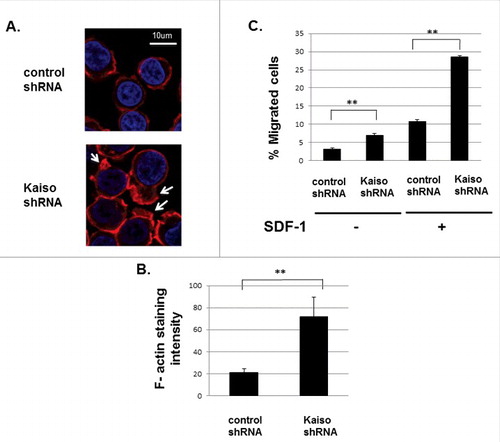
Next, to assess the potential functional consequence of this change in F-actin, we examined the effect of Kaiso downregulation on SDF-1 induced Jurkat cell migration. Jurkat cells transduced with lentivirus expressing control or Kaiso shRNA were subjected to transwell migration assays with or without a SDF-1 gradient. Cells that had migrated to the lower chamber were counted after 3 hours of incubation and the percentage of migrating cells in relation to input cells was calculated. We observed enhanced migration of Jurkat cells after Kaiso down regulation without SDF-1 stimulation, which was further increased with SDF-1 stimulation (). Taken together, these results indicate that the basal increase of Rac activation induced by Kaiso knockdown induces both increased F-actin assembly and enhanced cell migration that is further increased with SDF-1 stimulation. As we have previously reported that RhoH downregulation induced increased Rac activation in Rhoh−/− primary haematopoietic progenitor cells,Citation15,19 these results suggest Kaiso down-regulation, similar to loss of RhoH, enhances both random and SDF-1-directed cell migration in Jurkat cells via Rac activation.
RhoH and p120 catenin form a complex and co-localize in jurkat cells
Kaiso is reported to associate with p120 catenin which associates with E-cadherin to modulate adhesion in areas of cell-cell contact. After induction of WNT signaling, p120 catenin dissociates from E-cadherin and recruits Kaiso into the nucleusCitation23 and activates Rac via Vav2.Citation25 Our results showing RhoH and Kaiso association and both cytosolic and nuclear co-localization in Jurkat cells after chemokine stimulation suggest that RhoH may form a complex with Kaiso and p120 catenin. To investigate this possibility, we determined the association of RhoH with p120 catenin in Jurkat cells. Jurkat cells transduced with retroviral vectors expressing HA-RhoH were subjected to IP with anti-HA monoclonal antibody or IgG as control and analyzed by immunoblotting with an anti-p120 catenin monoclonal antibody. As indicated in , we observed that p120 catenin co-immunoprecipitated with RhoH. We next investigated the possible co-localization of RhoH and p120 catenin in Jurkat cells. Jurkat cells were stained with p120 catenin monoclonal and RhoH polyclonal antibody using fluorescence-coupled secondary antibodies and subsequently analyzed by confocal microscopy in a single confocal plane. We observed that RhoH and p120 catenin co-localized in the cytosol and at the cell membrane in Jurkat cells (). To address the functional significance of RhoH and p120 catenin interaction in T cells, we next examined the effect of chemokine stimulation on RhoH and p120 catenin co-localization. Jurkat cells were stimulated with SDF-1 for 2 minutes, stained with anti-RhoH polyclonal antibody and anti-p120 catenin monoclonal antibody and examined by confocal microscopy. Similar to the localization of Kaiso with RhoH at SDF-1-induced cell protrusions, we found p120 catenin and RhoH co-localized at SDF-1 induced surface protrusion structures (). These structures were observed in SDF-1 stimulated Jurkat cells but not in non-stimulated Jurkat cells.
Figure 6. RhoH and P120 catenin co-localize in Jurkat cells. (A) Immunoprecipitation of HA-RhoH and the p120 catenin complex. Jurkat cells transduced with retroviral vectors expressing HA-RhoH-IRES-YFP were subjected to immunoprecipitation with anti-HA monoclonal antibody and analyzed by immune blotting with anti-p120 catenin monoclonal antibody. IgG control is shown in left lane. Position of p120 catenin in right lane is noted by arrowhead. Size marker is shown on the left. This result is representative of 3 independent experiments. (B) Co-localization of RhoH and p120 catenin in Jurkat cells. Jurkat cells were fixed and stained with polyclonal anti-RhoH antibody (green), monoclonal anti-p120 catenin antibody (red) and nuclear staining with DAPI (blue). Arrowheads indicate co-localization readily apparent in the cytosol and at the membrane. Cell Images were captured by Zeiss LSM 700 Laser Scanning Confocal-Microscope with 63X magnification and processed by ZEN software (Zeiss). Mouse IgG and rabbit IgG were used as negative controls respectively and showed no signals (data not shown). (C) Co-localization of RhoH and p120 catenin at cell protrusion sites after SDF-1 stimulation in Jurkat cells. Jurkat cells were stimulated with SDF-1 (200 ng/ml) for 2 minutes, fixed and stained with polyclonal anti-RhoH antibody (green), monoclonal anti-p120 catenin antibody (red) and nuclear staining with DAPI (blue). Arrowheads indicate co-localization of RhoH and p120 catenin in cytosol and at areas of cell protrusion. SDF-1 induced cell protrusion stractures (arrowhead) were observed in that is not observed in . Cell Images were captured by Zeiss LSM 700 Laser Scanning Confocal-Microscope with 63X magnification and processed by ZEN software (Zeiss). Mouse IgG and rabbit IgG were used as negative controls respectively and showed no signals (data not shown).
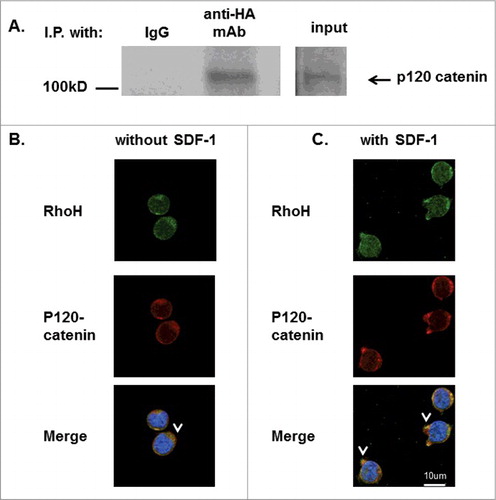
RhoH and kaiso co-localize in the nucleus after TCR activation and RhoH is required for TCR activation-induced kaiso nuclear localization
Chemokine induced T cell migration is required for T cell localization and T cell receptor activation after formation of an immunological synapse (IS) and both are reported to be regulated by RhoH via modulating Rap activity.Citation26 Rac activation is also required for inhibition of TCR dependent cell migration.Citation27 These previously reported results suggest a possible role of the RhoH and Kaiso complex in TCR activation by modulating Rac1 and Rap1 activity. To address the possible function of RhoH in modulating Kaiso during T cell receptor activation, Jurkat cells were treated with anti-CD3 antibody for 15 minutes to induce TCR activation and then stained with RhoH polyclonal and Kaiso monoclonal antibody using fluorescence-coupled secondary antibodies and subsequently analyzed by confocal microscopy. We found RhoH and Kaiso co-localized in the nucleus of TCR activated Jurkat cells () but to a lesser extent in unstimulated Jurkat cells ().
Figure 7. RhoH is required for TCR activation-induced Kaiso nuclear localization. (A) Co-localization of RhoH and Kaiso in nucleus after TCR activation. Jurkat cells were plated on anti-CD3 monoclonal antibody coated wells for 15min, fixed and stained with polyclonal anti-RhoH antibody (green), monoclonal anti-Kaiso antibody (red) and nuclear staining with DAPI (blue). Cell Images were captured by Zeiss LSM 700 Laser Scanning Confocal-Microscope with 63X magnification and processed by ZEN software (Zeiss). RhoH and Kaiso co-localized in the nucleus indicated by arrowhead vs. arrow. Represenative images of 3 independent experiments are shown. (B) RhoH knockdown is associated with the loss of Kaiso nuclear localization after TCR activation. Jurkat cells transduced with control or RhoH shRNA were plated on anti-CD3 monoclobnal antibody coated wells for 15min and then stained with monoclonal anti-Kaiso antibody (red), RhoH (purple) and nuclear DAPI staining (blue). Cell images were captured by Zeiss LSM 700 Laser Scanning Confocal-Microscope with 63X magnification and processed by ZEN software (Zeiss). The loss of RhoH and Kaiso co-localization in the nucleus indicated by arrowhead vs. arrow. The images were analyzed in a Z axis single plane with 0.9um thickness to address Kaiso nuclear localization.
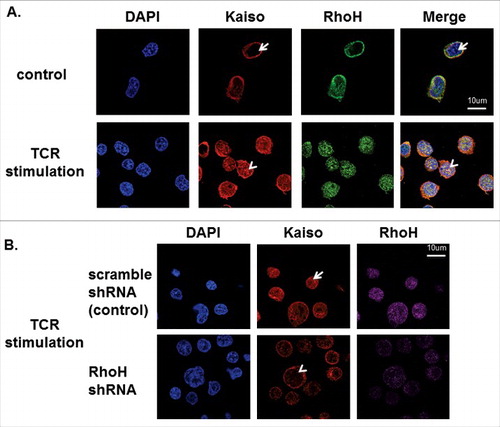
To further evaluate RhoH involvement in TCR activation-induced Kaiso nuclear localization, Jurkat cells were transduced with RhoH shRNA and stained with RhoH polyclonal and Kaiso monoclonal antibody using fluorescence-coupled secondary antibodies and subsequently analyzed by confocal microscopy. We observed the loss of nuclear localization of Kaiso with RhoH down regulation in Jurkat cells after TCR stimulation () compared to control (scrambled) shRNA transduced Jurkat cells (). These results indicate RhoH function is also required for TCR activation-induced nuclear localization of Kaiso.
Discussion
RhoH is a haematopoietic-specific Rho GTPase that lacks intrinsic GTPase activity and remains in a constitutively active, GTP-bound state.Citation21 Thus, RhoH cellular activity is likely related to its expression level, intracellular localization and phosphorylation state. RhoH is known to be critical for T cell development in the thymus and deficiency of RhoH leads to T cell lymphopenia in mice and a milder T cell immunodeficiency associated with Epidermodysplasia verruciformis in man.Citation4,13 In this regard, unlike other Rho GTPases, RhoH contains a functional ITAM-like motif which appears critical for its function in T cell receptor signaling.Citation13 RhoH has previously been shown to modulate SDF-1 induced cell migration by regulating Rac activity and subsequent actin polymerization.Citation19 However, the exact mechanism of RhoH function in T cells, apart from its role in ZAP70 activation and its functional relevance in other haematopoietic cells remains largely unknown.
Here we confirmed RhoH regulation of actin polymerization and T cell migration in Jurkat T cells. Furthermore, we identified Kaiso as a RhoH-associated molecule in these cells. Kaiso is a multifunctional protein that is critical for cell-cell adhesion, acts as a transcription modulator and accordingly is reported to localize to the nucleus, cytosol and at the cell membrane.Citation22-24 Cell membrane-localized Kaiso is associated with p120 catenin bound to E-cadherin and previous studies have shown this complex regulates actin cytoskeletal structures.Citation28 In this study, we show that RhoH and Kaiso interact and co-localize after SDF-1 stimulation at actin-rich cell protrusion sites in Jurkat cells. SDF-1 is a chemokine known to induce directed cell migration in several haematopoietic cell populations, including lymphocytes and haematopoietic stem cells. Confirming the functional relationship of Kaiso and RhoH, we show that Kaiso knockdown induces Rac membrane localization and activation and enhances actin polymeryzation and cell migration, a phenotype analogous to the one seen in Rhoh−/− cells. Additionally we showed that RhoH function is required for Kaiso localization at chemokine induced cell protrusion sites in Jurkat cells and isolated primary murine T cells. Based on these observations, we propose that the RhoH and Kaiso complex coordinately regulates chemokine induced cell migration by modulating actin cytoskeleton structures via modulation of Rac activation. We also isolated p120 catenin with the RhoH-Kaiso complex and demonstrated that p120 catenin co-localizes with RhoH at SDF-1 induced cell protrusion sites in Jurkat cells. Previous studies have implicated p120 catenin in Rho GTPase biology. p120 catenin is phosphorylated during WNT signaling and complexes with Rac and the guanine exchange factor Vav2 in fibroblasts and colonic cells.Citation25 p120 catenin also associates with p190 RhoGAP and downregulates RhoA activity thus inhibiting cell polarization and chemokine induced cell migration in melanoma cells.Citation29 However, in preliminary studies, we observed that RhoH downregulation does not affect SDF-1 induced p120 catenin localization at surface protrusion sites (data not shown). This suggests that RhoH may regulate p120 catenin function by mechanisms other than affecting its subcellular localization, perhaps by modulating its activation or phosphorylation state.
In addition, we demonstrate that RhoH and Kaiso co-localize in the nucleus after SDF-1 stimulation and TCR activation and this Kaiso nuclear localization requires RhoH. The role of Rho GTPases and actin polymerization in the nucleus has been increasingly defined.Citation30 For instance, RhoA and Rac are recruited to the nucleus after irradiation.Citation31 RhoA may affect gene expression via modulation of nuclear membrane stiffness and nuclear actin may be involved in nucleosome reassembly and regulate gene expression in association with the Ino80 chromatin remodeling complex. Therefore, it is possible that Rac and RhoA regulated nuclear actin polymerization modulates the translocation of specific, transcription regulating molecules to the nuclear envelope and nuclear pore complexes at specific positions of the genome depending on the microenvironment.Citation32,33 Given the cross-talk between RhoH and Rac in the cytoplasm and at the cell membrane, our data suggest the possibility that Rac regulated nuclear actin polymerization may be modulated by a protein complex containing RhoH and Kaiso and that this complex may link cytoskeletal changes occurring during directed cell migration with subsequent changes in gene expression in T cell maturation.
The RhoH-Kaiso complex could also be directly involved in nuclear protein trafficking. Kaiso is recruited into the nucleus and regulates gene expression in response to extracellular signals where it binds the sequence TCCTGCnA and to methylated CpG island motifs of target gene promoters. In the nucleus, Kaiso forms complexes with subfamilies of the HDAC-NcoR complex regulating the expression of different genes depending on the biological context.Citation24 Interestingly, we identified Lamin A/C and a number of other peptides of nuclear proteins in association with RhoH in our screening (data not shown), suggesting that the RhoH-Kaiso complex may also be involved in nuclear protein trafficking. Thus, the RhoH and Kaiso complex may regulate both the actin cytoskeleton and influence gene expression in a coordinated fashion during the process of chemokine induced cell migration and T cell maturation in response to microenvironment signals.
In summary, we propose that RhoH and Kaiso function as a complex together with p120 catenin to modulate SDF-1 induced cell migration via regulation of Rac activity controlling the actin cystoskekleton rearrangement and possibly genes expression in a coordinated fashion.
Material and methods
Identification of RhoH interacting proteins by mass spectrometry
Flag-Biotin tagged RhoH containing a 23-amino-acid BirA recognition motif fused to its N-terminus was cloned into a lentiviral LeGO-iG2 vector (LeGO-iG2-RhoH) (Figure S1). In addition, E. coli biotin ligase BirA was cloned into a LeGO-iV2 vector (LeGO-iV2-Bir). Jurkat cells were then transduced with the lentiviral vector supernatants of either the E. coli biotin ligase BirA expressing LeGO-iV2 vector (LeGO-iV2-Bir) or both LeGO-iV2-Bir and the tagged RhoH expressing LeGO-iG2-RhoH vector on fibronectin (CH296 provided by Takara Bio, Otsu, Japan) as previously described.Citation34 Single or double transduced Jurkat cells were then selected by fluorescence-activated cell sorting (FACS) for eGFP (LeGO-iG2) and/ or Venus (LeGO-iV2) fluorescence signals. Stably transduced cells were expanded, centrifuged and cell pellets lysed in ice-cold Mg2+ lysis/wash buffer (Upstate Biotechnology, Lake Placid, NY) containing a mixture of protease and phosphatase inhibitors (1 mM Na3VO4, 10 mM NaF, complete protease inhibitor cocktail). For streptavidin affinity purification of RhoH and associated proteins, lysates were then incubated with streptavidin beads, washed and RhoH protein complexes eluted with Laemmli SDS sample buffer and boiling. Subsequently, associated proteins were separated by SDS-page and the respective lanes analyzed using tandem mass spectrometry with Velos LTQ Linear ion traps. Finally resulting peptide sequences were analyzed using SEQUEST software at the Taplin Mass Spectrometry Facility at Harvard Medical School. Only peptide sequences identified in BirA and tagged RhoH-co-expressing but not BirA single positive Jurkat cells in 3 separate experiments were considered positive hits.
Antibodies
The following primary antibodies were used in immunoblot analyses at 1:500 dilution: anti-Kaiso (Abcam, Cambridge, MA) and anti-RhoH,Citation35 anti-p120 catenin (Invitrogen, Carlsbad, CA) and anti-HA (3F10) (Covance, Dedham, MA) antibody. For detection of Rac a “pan”-Rac antibody (BD Biosciences Transduction Laboratories, San Diego, CA) recognizing all 3 Rac isforms was used. Primary antibodies were detected using horseradish peroxidase-conjugated goat anti-mouse (Cell Signaling Technology, Danvers, MA), or goat anti-rabbit antibodies (Cell Signaling Technology, Danvers, MA) as well as enhanced chemiluminescence detection assays (Cell Signaling Technology, Danvers, MA). Kaiso monoclonal antibody (Abcam, Cambridge, MA), p120 catenin antibody (Invitrogen, Carlsbad, CA), RhoH polyclonal antibody and anti-HA monoclonal antibodies (Covance, Dedham, MA) were also used for immunofluorecence staining at a dilution of 1:500. Alexa488/594/647 conjugated anti-mouse or anti-rabbit IgG were used as secondary detection antibodies (Invitrogen, Carlsbad, CA).
Retroviral vector construction and retrovirus supernatant generation
EGFP-fused and HA-tagged RhoH were each subcloned in the murine stem cell virus–based vector MIEG3.Citation36 High-titer retroviral supernatants were generated by transfection of ecotropic Phoenix-gp cells using a calcium phosphate precipitation kit (Invitrogen, Carlsbad, CA) as described previously.Citation36
Confocal microscopy
For immunofluorescence staining, cells were layered onto poly-L-lysine coated chamber slides and subsequently stimulated or not, with 100 ng/mL SDF-1 at 37°C for the indicated time points. Cells were subsequently fixed using BD Cytofix/Cytoperm (BD Bioscience, San Jose, CA) solution and stained with Rhodamine (TRITC)–labeled phalloidin, anti-Rac1 (BD Bioscience, San Jose, CA), anti-Kaiso (Abcam, Cambridge, MA), anti-RhoH antibody or anti-HA antibody followed by Alexa 488/594/647 conjugated anti-mouse or anti-rabbit IgG monoclonal antibodies (Invitrogen, Carlsbad, CA). Images were captured by Zeiss LSM 700 Laser Scanning Confocal-Microscope using red, green and blue laser channels with 63X magnification with single confocal plane and thereafter processed by ZEN software (Zeiss, Jena, Germany).
Subcellular fractionation
Pellets of HA-RhoH expressing Jurkat cells were solubilized in ice-cold lysis buffer (250mM sucrose, 20mM HEPES (pH 7.4), 10mM KCl, 1.5mM MgCl2, 1mM EDTA, 1mM EGTA), homogenized and subsequently centrifuged 5 min at 800xg. The resulting pellet was resuspended in Mg2+ lysis/wash buffer (Upstate Biotechnology, Lake Placid, NY) with 10% glycerol and 0.1% SDS and centrifuged at 800xg. The pellet containing the nuclear fraction was then solubilized again in Mg2+ lysis/wash buffer containing 10% glycerol and 0.1% SDS and subsequently briefly (3 sec) sonicated on ice at a power setting of 2. Supernatants were subjected to ultracentrifugation (100,000xg, 30 min). The supernatant contained the cytosolic fraction. Nuclear fractions were diluted 5-fold with Mg2+ lysis/wash buffer and equal volumes of each fraction were used for immunoprecipitation.
RhoH/kaiso knockdown
Kaiso-specific shRNA (TRCN0000232208) and luciferase-specific shRNA expressing pLKO5lentiviral vectors were obtained from the Broad Institute (Cambridge, MA). RhoH shRNAs (Figure S5A) and scrambled control shRNA were cloned into the lentiviral vector LeGO-iV2. Jurkat cells were transduced with the supernatants of the respective lentiviruses on fibronectin (fragment CH296; provided by Takara Bio, Otsu, Japan). Cells transduced with pLKO5 lentivirus were selected by puromycin (pLKO5); cells transduced with Venus co-expressing LeGO virus were purified using FACS. The efficiency of Kaiso and RhoH knockdown was confirmed by quantitative RT-PCR with primers chosen for each gene. RhoH (Forward: ATGCTGAGTTCCATCAAGTGC, Reverse: TCTGCCTGCTGGTAGGACA), Kaiso (Forward: TGCTGAACTCCTTGAATGAGC, Reverse: CGGAATTTTCGGTCTTCCACAA) using Applied Biosystems 7500 Real-Time PCR System. The real-time PCR profile for the assay was: 50°C for 2 min, followed by activation at 72°C for 10 min and 40 cycles of denaturation at 95°C for 15 s and annealing/elongation at 60°C for 1 min.
Immunoprecipitation and immunoblotting
As previously described, Jurkat cells transduced with HA-RhoH expressing retrovirusCitation6,13,15 were sorted and lysed in ice-cold Mg2+ lysis/wash buffer containing a mixture of protease and phosphatase inhibitors (1 mM Na3VO4, 10 mM NaF, complete protease inhibitor cocktail). The cell lysates were subjected to immunoprecipitation with anti-HA monoclonal antibody (Covance, Dedham, MA, 1:500 dilution) using Protein A/G PLUS-Agarose (Santa Cruz Biotech, Dallas, TX). Finally, Kaiso or p120 catenin were detected with anti-Kaiso monoclonal antibody (Abcam, Cambridge, MA 1:500 dilution) or anti-p120 catenin antibody (BD Biosciences Transduction Laboratories, San Diego, CA) and HRP-coupled anti-mouse IgG antibody (1:2000, Cell Signaling Technology, Danvers, MA) using chemiluminescence (Cell Signaling Technology, Danvers, MA).
Measurement of the GTP-bound rac protein
Rac activity was determined by glutathione-S-transferase (GST)–fusion pull-down assay using p21 activated kinase (PAK) effector domain (PBD) (Cell Signaling Technology, Danvers, MA) as described previously.Citation37 Control or Kaiso shRNA transduced Jurkat cells were treated with 100 ng/ml SDF-1α for 2 min and then immediately lysed in ice cold Mg2+ lysis/wash buffer. The cellular extracts were incubated with the GST–p21-activated kinase 1 (PAK1) beads following the manufacturer instructions. Levels of bead-bound GTP-Rac and total Rac proteins were analyzed by immunoblot with anti-Rac antibody (1:1000; BD Biosciences Transduction Laboratories, San Diego, CA) and HRP-coupled anti-mouse IgG antibody (1:2000, Cell Signaling Technology) using chemiluminescence (Cell Signaling Technology, Danvers, MA).
Transwell cell migration assay
Jurkat cells transduced with lentivirus vectors expressing control, RhoH shRNA or Kaiso shRNA were subjected to transwell migration assays. Equal numbers of transduced cells were applied to the upper chamber of 5-μm pore filter transwell assay chambers (Corning, Corning, NY) and SDF-1 (100 ng/ml) was added as chemoattractant into the lower chamber as previously described.Citation37 After 3 hours of incubation at 37° in 5% CO2 cells that had migrated into the lower chambers were counted. Data represent the ratio of migrated cells/total input cells and is shown as percentage.
Isolation of T cells from spleen
Rhoh−/− mice were generated by standard DNA homologous recombination and backcrossed in a C57BL/6J background as described previously.Citation37 T cells were purified from wildtype and Rhoh−/− mouse spleens by negative selection using the Pan T Cell Isolation Kit (Miltenyi Biotec, Auburn, CA). The purity of isolated T cells in all experiments was greater than 90%, as assessed by flow cytometry with anti-mouse CD3ε Ab (BD Biosciences Transduction Laboratories, San Diego, CA). All animal procedures and experiments were approved by the Institutional Animal Care and Use Committee of Boston Children's Hospital (Boston, MA).
TCR activation induction
For Jurkat cell receptor activation, wells were coated with monoclonal antibodies against CD3 (OKT3, 10 µg/ml) (BD Biosciences Transduction Laboratories, San Diego, CA), or control (mouse IgG, 10 µg/ml) (BD Biosciences Transduction Laboratories, San Diego, CA). Jurkat cells were incubated on coated wells for indicated time.
Statistical analysis
All experiments were performed 3 times with representative data shown in figures. Statistical significance was determined by a Student t-Test and data with p-value less than 0.05 were considered as significantly different.
Disclosure of potential conflicts of interest
No potential conflicts of interest were disclosed.
Acknowledgments
We thank members of the Williams and Cantor laboratories for helpful discussions and Maria Suarez and Natasha Rossi for administrative assistance. We also thank Ross Tomaino at the Taplin Mass Spectrometry Facility at Harvard Medical School for his advice, Anthony Hill at the Imaging Core Facility at Boston Children's Hospital (BCH) for use of their Confocal microcope, Ronald Mathieu and Mahnaz Paktinat at the Flow Lab HSCI Core at BCH, and expert animal husbandry and support from Meaghan McGuinness, Jenna Wood, Heedon Chae, and Chad Harris.
Additional information
Funding
References
- Dallery E, Galiegue-Zouitina S, Collyn-d'Hooghe M, Quief S, Denis C, Hildebrand MP, Lantoine D, Deweindt C, Tilly H, Bastard C, et al. TTF, a gene encoding a novel small G protein, fuses to the lymphoma- associated LAZ3 gene by t(3;4) chromosomal translocation. Oncogene 1995; 10:2171-8; PMID:7784061
- Preudhomme C, Roumier C, Hildebrand MP, Dallery-Prudhomme E, Lantoine D, Lai JL, Daudignon A, Adenis C, Bauters F, Fenaux P, et al. Nonrandom 4p13 rearrangements of the RhoH/TTF gene, encoding a GTP- binding protein, in non-Hodgkin's lymphoma and multiple myeloma. Oncogene 2000; 19:2023-32; PMID:10803463; https://doi.org/10.1038/sj.onc.1203521
- Pasqualucci L, Neumeister P, Goossens T, Nanjangud G, Chaganti RS, Kuppers R, Dalla-Favera R. Hypermutation of multiple proto-oncogenes in B-cell diffuse large-cell lymphomas. Nature 2001; 412:341-6; PMID:11460166; https://doi.org/10.1038/35085588
- Crequer A, Troeger A, Patin E, Ma CS, Picard C, Pedergnana V, Fieschi C, Lim A, Abhyankar A, Gineau L, et al. Human RHOH deficiency causes T cell defects and susceptibility to EV-HPV infections. J Clin Invest 2012; 122:3239-47; PMID:22850876; https://doi.org/10.1172/JCI62949
- Sanchez-Aguilera A, Rattmann I, Drew DZ, Muller LU, Summey V, Lucas DM, Byrd JC, Croce CM, Gu Y, Cancelas JA, et al. Involvement of RhoH GTPase in the development of B-cell chronic lymphocytic leukemia. Leukemia 2010; 24:97-104; PMID:19847197; https://doi.org/10.1038/leu.2009.217
- Troeger A, Johnson AJ, Wood J, Blum WG, Andritsos LA, Byrd JC, Williams DA. RhoH is critical for cell-microenvironment interactions in chronic lymphocytic leukemia in mice and humans. Blood 2012; 119:4708-18; PMID:22474251; https://doi.org/10.1182/blood-2011-12-395939
- Iwasaki T, Katsumi A, Kiyoi H, Tanizaki R, Ishikawa Y, Ozeki K, Kobayashi M, Abe A, Matsushita T, Watanabe T, et al. Prognostic implication and biological roles of RhoH in acute myeloid leukaemia. Eur J Haematol 2008; 81:454-60; PMID:18691253; https://doi.org/10.1111/j.1600-0609.2008.01132.x
- Gundogdu MS, Liu H, Metzdorf D, Hildebrand D, Aigner M, Aktories K, Heeg K, Kubatzky KF. The haematopoietic GTPase RhoH modulates IL3 signalling through regulation of STAT activity and IL3 receptor expression. Molecular cancer 2010; 9:225; PMID:20738848; https://doi.org/10.1186/1476-4598-9-225
- Dallery-Prudhomme E, Roumier C, Denis C, Preudhomme C, Kerckaert JP, Galiegue-Zouitina S. Genomic structure and assignment of the RhoH/TTF small GTPase gene (ARHH) to 4p13 by in situ hybridization. Genomics 1997; 43:89-94; PMID:9226377; https://doi.org/10.1006/geno.1997.4788
- Crul M, de Klerk GJ, Beijnen JH, Schellens JH. Ras biochemistry and farnesyl transferase inhibitors: a literature survey. Anticancer Drugs 2001; 12:163-84; PMID:11290863; https://doi.org/10.1097/00001813-200103000-00001
- Li X, Bu X, Lu B, Avraham H, Flavell RA, Lim B. The hematopoiesis-specific GTP-binding protein RhoH is GTPase deficient and modulates activities of other Rho GTPases by an inhibitory function. Mol Cell Biol 2002; 22:1158-71; PMID:11809807; https://doi.org/10.1128/MCB.22.4.1158-1171.2002
- Fueller F, Kubatzky KF. The small GTPase RhoH is an atypical regulator of haematopoietic cells. Cell Commun Signal 2008; 6:6; PMID:18823547; https://doi.org/10.1186/1478-811X-6-6
- Gu Y, Chae H, Siefring J, Jast IJ, Hildeman D, Williams DA. RhoH, a GTPase recruits and activates Zap70 required for T cell receptor signaling and thymocyte development. Nat Immunol 2006; 7:1182-90; PMID:17028588; https://doi.org/10.1038/ni1396
- Dorn T, Kuhn U, Bungartz G, Stiller S, Bauer M, Ellwart J, Peters T, Scharffetter-Kochanek K, Semmrich M, Laschinger M, et al. RhoH is important for positive thymocyte selection and T-cell receptor signaling. Blood 2007; 109:2346-55; PMID:17119112; https://doi.org/10.1182/blood-2006-04-019034
- Chae HD, Siefring JE, Hildeman DA, Gu Y, Williams DA. RhoH regulates subcellular localization of ZAP-70 and Lck in T cell receptor signaling. PloS one 2010; 5:e13970; PMID:21103055; https://doi.org/10.1371/journal.pone.0013970
- Matsumoto M, Oyamada K, Takahashi H, Sato T, Hatakeyama S, Nakayama KI. Large-scale proteomic analysis of tyrosine-phosphorylation induced by T-cell receptor or B-cell receptor activation reveals new signaling pathways. Proteomics 2009; 9:3549-63; PMID:19609962; https://doi.org/10.1002/pmic.200900011
- Oda H, Fujimoto M, Patrick MS, Chida D, Sato Y, Azuma Y, Aoki H, Abe T, Suzuki H, Shirai M. RhoH plays critical roles in Fc epsilon RI-dependent signal transduction in mast cells. J Immunol 2009; 182:957-62; PMID:19124738; https://doi.org/10.4049/jimmunol.182.2.957
- Wang H, Zeng X, Fan Z, Lim B. RhoH modulates pre-TCR and TCR signalling by regulating LCK. Cell Signal 2011; 23:249-58; PMID:20851766; https://doi.org/10.1016/j.cellsig.2010.09.009
- Chae HD, Lee KE, Williams DA, Gu Y. Cross-talk between RhoH and Rac1 in regulation of actin cytoskeleton and chemotaxis of hematopoietic progenitor cells. Blood 2008; 111:2597-605; PMID:18089848; https://doi.org/10.1182/blood-2007-06-093237
- Cherry LK, Li X, Schwab P, Lim B, Klickstein LB. RhoH is required to maintain the integrin LFA-1 in a nonadhesive state on lymphocytes. Nat Immunol 2004; 5:961-7; PMID:15300248; https://doi.org/10.1038/ni1103
- Troeger A, Williams DA. Hematopoietic-specific Rho GTPases Rac2 and RhoH and human blood disorders. Exp Cell Res 2013; 319:2375-83; PMID:23850828; https://doi.org/10.1016/j.yexcr.2013.07.002
- Zhang PX, Wang Y, Liu Y, Jiang GY, Li QC, Wang EH. p120-catenin isoform 3 regulates subcellular localization of Kaiso and promotes invasion in lung cancer cells via a phosphorylation-dependent mechanism. Int J Oncol 2011; 38:1625-35; PMID:21468542
- Del Valle-Perez B, Casagolda D, Lugilde E, Valls G, Codina M, Dave N, de Herreros AG, Dunach M. Wnt controls the transcriptional activity of Kaiso through CK1epsilon-dependent phosphorylation of p120-catenin. J Cell Sci 2011; 124:2298-309; PMID:21670201; https://doi.org/10.1242/jcs.082693
- Yoon HG, Chan DW, Reynolds AB, Qin J, Wong J. N-CoR mediates DNA methylation-dependent repression through a methyl CpG binding protein Kaiso. Mol Cell 2003; 12:723-34; PMID:14527417; https://doi.org/10.1016/j.molcel.2003.08.008
- Valls G, Codina M, Miller RK, Del Valle-Perez B, Vinyoles M, Caelles C, McCrea PD, Garcia de Herreros A, Dunach M. Upon Wnt stimulation, Rac1 activation requires Rac1 and Vav2 binding to p120-catenin. J Cell Sci 2012; 125:5288-301; PMID:22946057; https://doi.org/10.1242/jcs.101030
- Baker CM, Comrie WA, Hyun YM, Chung HL, Fedorchuk CA, Lim K, Brakebusch C, McGrath JL, Waugh RE, Meier-Schellersheim M, et al. Opposing roles for RhoH GTPase during T-cell migration and activation. Proc Natl Acad Sci U S A 2012; 109:10474-9; PMID:22689994; https://doi.org/10.1073/pnas.1114214109
- Cernuda-Morollon E, Millan J, Shipman M, Marelli-Berg FM, Ridley AJ. Rac activation by the T-cell receptor inhibits T cell migration. PloS one 2010; 5:e12393; PMID:20811629; https://doi.org/10.1371/journal.pone.0012393
- Schackmann RC, Tenhagen M, van de Ven RA, Derksen PW. p120-catenin in cancer - mechanisms, models and opportunities for intervention. J Cell Sci 2013; 126:3515-25; PMID:23950111; https://doi.org/10.1242/jcs.134411
- Molina-Ortiz I, Bartolome RA, Hernandez-Varas P, Colo GP, Teixido J. Overexpression of E-cadherin on melanoma cells inhibits chemokine-promoted invasion involving p190RhoGAP/p120ctn-dependent inactivation of RhoA. J Biol Chem 2009; 284:15147-57; PMID:19293150; https://doi.org/10.1074/jbc.M807834200
- Guilluy C, Osborne LD, Van Landeghem L, Sharek L, Superfine R, Garcia-Mata R, Burridge K. Isolated nuclei adapt to force and reveal a mechanotransduction pathway in the nucleus. Nat Cell Biol 2014; 16:376-81; PMID:24609268; https://doi.org/10.1038/ncb2927
- Dubash AD, Guilluy C, Srougi MC, Boulter E, Burridge K, Garcia-Mata R. The small GTPase RhoA localizes to the nucleus and is activated by Net1 and DNA damage signals. PloS one 2011; 6:e17380; PMID:21390328; https://doi.org/10.1371/journal.pone.0017380
- Dion V, Shimada K, Gasser SM. Actin-related proteins in the nucleus: life beyond chromatin remodelers. Curr Opin Cell Biol 2010; 22:383-91; PMID:20303249; https://doi.org/10.1016/j.ceb.2010.02.006
- Yoshida T, Shimada K, Oma Y, Kalck V, Akimura K, Taddei A, Iwahashi H, Kugou K, Ohta K, Gasser SM, et al. Actin-related protein Arp6 influences H2A.Z-dependent and -independent gene expression and links ribosomal protein genes to nuclear pores. PLoS genetics 2010; 6:e1000910; PMID:20419146; https://doi.org/10.1371/journal.pgen.1000910
- Woo AJ, Moran TB, Schindler YL, Choe SK, Langer NB, Sullivan MR, Fujiwara Y, Paw BH, Cantor AB. Identification of ZBP-89 as a novel GATA-1-associated transcription factor involved in megakaryocytic and erythroid development. Mol Cell Biol 2008; 28:2675-89; PMID:18250154; https://doi.org/10.1128/MCB.01945-07
- Gu Y, Jasti AC, Jansen M, Siefring JE. RhoH, a hematopoietic-specific Rho GTPase, regulates proliferation, survival, migration, and engraftment of hematopoietic progenitor cells. Blood 2005; 105:1467-75; PMID:15494435; https://doi.org/10.1182/blood-2004-04-1604
- Williams DA, Tao W, Yang F, Kim C, Gu Y, Mansfield P, Levine JE, Petryniak B, Derrow CW, Harris C, et al. Dominant negative mutation of the hematopoietic-specific Rho GTPase, Rac2, is associated with a human phagocyte immunodeficiency. Blood 2000; 96:1646-54; PMID:10961859
- Gu Y, Filippi MD, Cancelas JA, Siefring JE, Williams EP, Jasti AC, Harris CE, Lee AW, Prabhakar R, Atkinson SJ, et al. Hematopoietic cell regulation by Rac1 and Rac2 guanosine triphosphatases. Science 2003; 302:445-9; PMID:14564009; https://doi.org/10.1126/science.1088485