ABSTRACT
Migratory polarity and epithelial polarity share many common regulatory mechanisms. Rho GTPases play a key role in modulating cell polarity, which in migrating cells has been conventionally studied along the solitary front-rear axis. In recent work, we discovered that Prickle1 (Pk1), a core com-ponent of planar cell polarity (PCP) signaling, mediates a novel lateral signaling pathway that coordinates multi-axial protrusive activities from the lateral cortex of migrating cancer cells. We identified that Arhgap21 and 23 are essential effectors of Pk1, and that lateral signaling regulates RhoA, actomyosin and focal adhesion dynamics. Interestingly, we showed that lateral signaling coordinates shape dynamics that are critical during cell migration and function orthogonally to front-rear directional polarity.
Cell migration is a complex process with significant relevance in health and diseases.Citation1 Polarity is a fundamental feature of migrating cells and is conventionally referred to as asymmetric signaling activities and cellular structures along the front-rear axis. Localized activation/inactivation of the Rho family of GTPases plays a central role in the signaling mechanisms that regulate this front-rear polarityCitation2 as are many of the pathways that orchestrate polarity in epithelial tissues and embryonic development.Citation3
Epithelial polarity is manifested in multiple dimensions. Planar cell polarity (PCP) organizes the asymmetry across the epithelial plane that is orthogonal to the apical-basal axis. Mechanistically, PCP depends on the asymmetric distribution of core signaling molecules that include transmembrane proteins Frizzled (Fzd) and Van Gogh-like (Vangl), in addition to cytoplasmic proteins Disheveled (Dvl), Diego and Prickle (Pk).Citation4 Extensive studies have revealed an essential role of PCP signaling in tissue organization and cell migration during morphogenesis and development.Citation5 Furthermore, research from our group and others have illustrated asymmetric distributions and functional significance of PCP components in metastatic cancer cells.Citation6,7 However, the mechanisms that underlie PCP signaling in cell migration and cancer metastasis remain elusive. Our recent work reveals that PCP signaling components Pk1 and Smurf2 coordinate a novel lateral signaling pathway, which functions orthogonally to the front-rear polarity in regulating cell migration.Citation8
The basic mechanisms of front-rear polarity are relatively well defined for cells that migrate in response to directional cues.Citation2,9 However, cells often migrate without a pre-determined direction, for example metastatic cancer cells must explore the microenvironment containing complex migrational cues, and regulation of cell polarity in such contexts is much less understood. We previously showed that fibroblast-derived exosomes mobilize Wnt-PCP signaling in breast cancer cells and promote cell migration and metastasis.Citation7 In response to activating exosomes, cancer cells undergo random migration and often display several active protrusions in multiple directions that are separated by non-protrusive cortex on the lateral sides (). As a result, it is usually hard to define a solitary front-rear axis in such migrating cancer cells. Intriguingly, immunolabeling experiments revealed that Pk1, a core PCP component, decorates the non-protrusive lateral cell cortex, which are also highlighted by actin bundles flanking active protrusions. Functionally, Pk1 silencing results in a disordered actin network and inhibition of cell migration with Pk1-deficient cells displaying flattened morphology with disrupted lateral cortex and diffuse protrusive activity that extends around the entire cell periphery (). Interestingly silencing of Smurf2, an E3 ubiquitin ligase that mediates the degradation of Pk1 in PCP signaling, also inhibits cell migration but leads to an elongated and spindle morphology that is opposite to Pk1-deficient cells (). Importantly, enhanced levels of Pk1 are observed along the lateral cortex of Smurf2-deficient cells, which is accompanied by intensified lateral actin bundles and restricted protrusive activity. These results support a model whereby Pk1 mediates a novel lateral signaling pathway that maintains the non-protrusive lateral cortex, thus confining the multi-directional protrusive activity in migrating cells (). We propose that this protrusive-lateral distinction is well suited to describe the asymmetry in cells with multiple active protrusions.
Figure 1. Interaction between lateral signaling and protrusive activity drives shape volatility during cell migration. (A) Migrating cells often extend multiple protrusions that lead to a multi-axial morphology. Pk1 and Arhgap21/23 function at the lateral cortex of the cell to confine protrusive activities. The activity of lateral signaling includes regulation of RhoA, cytoskeleton, actomyosin, focal adhesions, etc. Smurf2 mediates the negative regulation of lateral signaling. (B) Shape volatility of migrating cells depends on proper coordination of lateral signaling. Perturbation of lateral signaling leads to inhibited motility with distinct morphologies.
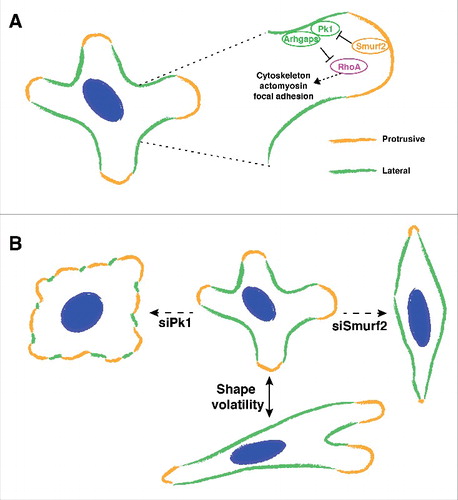
Cell polarity depends on the exquisite regulation of distinct members of the Rho family of small GTPases.Citation2,3 Activation and inactivation of Rho GTPases are under the regulation of guanine exchange factors (GEFs) and GTPase activating proteins (GAPs), respectively.Citation10 Although studies have identified a plethora of GEFs and GAPs that coordinate Rho GTPases along the front-rear axis as well as the apical-basal axis, little is known about such regulators in PCP signaling. Using immunoprecipitation (IP) and tandem mass spectrometry (MS/MS), we identified that Pk1 interacts with Arhgap21 and Arhgap23, two closely related proteins of the RhoGAP family. In biochemical assays, silencing of Pk1 and Arhgap21/23 both lead to increased levels of active RhoA, suggesting that lateral signaling negatively regulates RhoA. In the front-rear view of migratory polarity, activation of RhoA is key to promote the formation of contractile actomyosin networks and the detachment of the cell body at the rear.Citation2 However, active RhoA has also been observed at the very front region of lamellipodial protrusions.Citation11 Our results suggest that lateral signaling may play a role in confining active RhoA to the leading edges of protrusive regions.
The downstream effectors of Rho GTPases include the actomyosin network and focal adhesions, which are essential apparatus in migratory polarity.Citation12 Within the multiple lamellipodia of migrating cancer cells, we observed accumulation of phosphorylated myosin light chain (pMLC2), which is important in promoting the contractility of the actomyosin network. In addition, the majority of focal adhesions undergo dynamic turnover within the front region of protrusions. After knockdown of Pk1 or Arhgap21/23, pMLC2 expands throughout the cell and focal adhesions are stabilized around the entire cell periphery. Interestingly, a recent study reports that membranous Pk1 promotes microtubule-dependent disassembly of focal adhesions through interacting with CLASP and LL5β.Citation13 This suggests that Pk1-mediated lateral signaling regulates the organization of both the actin and microtubule cytoskeleton as well as focal adhesion dynamics in migrating cancer cells. An important area of future study is to investigate how lateral signaling integrates with other pathways to achieve the spatial and temporal regulation of small Rho GTPases in coordinating the asymmetry of different types of cytoskeleton. The fast advances in designing specific fluorescent resonance energy transfer (FRET)-probe reporters for small GTPases will provide invaluable tools for such studies in migrating cells.Citation14
Regulation of the actomyosin network and focal adhesions are fundamental to altering cell morphology.Citation15 In our work, we observed that exosome-stimulated cancer cells display dynamic changes in their multi-axial shape during migration (). We then analyzed morphological dynamics of cells using shape volatility, which measures the distribution of rate changes in cellular aspect ratios (AR). Interestingly, shape volatility has a positive correlation with migration speed, indicating a strong link between cell motility and the capacity to dynamically change cell shape. Mechanistically, we showed that the interplay between Pk1-mediated lateral signaling and Smurf-centered protrusive signaling is essential to drive shape volatility. Thus, when lateral signaling is inhibited (e.g. silencing of Pk1 or Arhgap21/23), protrusive activity expands around the entire cell periphery and the cells acquire a low AR morphology (). In contrast, when lateral signaling predominates (e.g., upon Smurf2 knockdown), protrusive activity is reduced and the cells accumulate a high AR shape (). In contrast to the starkly distinct geometry, the two contexts share disrupted cell motility, as both expanded and restricted protrusive activity fail to support functional protrusions. This highlights the importance of a coordinated lateral-protrusive balance in cell motility.
It is an important aspect to study how this lateral signaling integrates with the conventional front-rear polarity. An essential steering mechanism during directional migration is the inhibition of lateral pseudopods and restriction of new protrusions to pre-existing leading edges. Interestingly, we observed that the shape volatility of cells undergoing chemotaxis in response to serum also depends on Pk1 and Smurf2. In addition to inhibiting shape volatility, disruption of Pk1-mediated lateral signaling also dramatically reduced the speed of cell migration, similar to the phenotypes observed in exosome-stimulated random migration. Intriguingly, despite the reduced speed, cell movements were still steered along the chemotactic gradient, suggesting that the steering mechanism of “front-rear” orientation was not affected. These results indicate that lateral signaling mediated by planar cell polarity pathways coordinates cell shape volatility that we propose is a novel operative feature of moving cells. Moreover, we propose that analogous to polarized epithelia, PCP-mediated lateral signaling functions orthogonally to the conventional “front-rear” polarity that is mediated by apical-basal complexes during directional cell migration.
Our discovery of a lateral signaling pathway comprised of planar cell polarity components represents a novel mechanism that coordinates cell morphological dynamics in biological processes. The discovery of specific molecular pathways that regulate cell shape volatility and its importance to productive motility is an exciting new concept in cell biology that may have far reaching implications in understanding tissue morphogenesis.
Disclosure of potential conflicts of interest
No potential conflicts of interest were disclosed.
Funding
This work was supported by funds from CIHR (to J.L.W. grant #MOP14339) and Terry Fox Research Institute. J.L.W. is a CRC Chair in Systems Biology.
References
- Wedlich D. Cell Migration in Development and Disease. Wiley-Blackwell; 2006.
- Ridley AJ, Schwartz MA, Burridge K, Firtel RA, Ginsberg MH, Borisy G, Parsons JT, Horwitz AR. Cell migration: integrating signals from front to back. Science (New York, N.Y 2003; 302:1704-9; PMID:14657486; https://doi.org/10.1126/science.1092053
- Nelson WJ. Remodeling epithelial cell organization: transitions between front-rear and apical-basal polarity. Cold Spring Harb Perspect Biol 2009; 1:a000513; PMID:20066074; https://doi.org/10.1101/cshperspect.a000513
- Peng Y, Axelrod JD. Asymmetric protein localization in planar cell polarity: mechanisms, puzzles, and challenges. Curr Top Dev Biol 2012; 101:33-53; PMID:23140624; https://doi.org/10.1016/B978-0-12-394592-1.00002-8
- Wallingford JB. Planar cell polarity and the developmental control of cell behavior in vertebrate embryos. Annu Rev Cell Dev Biol 2012; 28:627-53; PMID:22905955; https://doi.org/10.1146/annurev-cellbio-092910-154208
- Anastas JN, Biechele TL, Robitaille M, Muster J, Allison KH, Angers S, Moon RT. A protein complex of SCRIB, NOS1AP and VANGL1 regulates cell polarity and migration, and is associated with breast cancer progression. Oncogene 2012; 31:3696-708; PMID:22179838; https://doi.org/10.1038/onc.2011.528
- Luga V, Zhang L, Viloria-Petit AM, Ogunjimi AA, Inanlou MR, Chiu E, Buchanan M, Hosein AN, Basik M, Wrana JL. Exosomes mediate stromal mobilization of autocrine Wnt-PCP signaling in breast cancer cell migration. Cell 2012; 151:1542-56; PMID:23260141; https://doi.org/10.1016/j.cell.2012.11.024
- Zhang L, Luga V, Armitage SK, Musiol M, Won A, Yip CM, Plotnikov SV, Wrana JL. A lateral signalling pathway coordinates shape volatility during cell migration. Nat Commun 2016; 7:11714; PMID:27226243; https://doi.org/10.1038/ncomms11714
- Petrie RJ, Doyle AD, Yamada KM. Random versus directionally persistent cell migration. Nat Rev 2009; 10:538-49; PMID:19603038; https://doi.org/10.1038/nrm2729
- Bos JL, Rehmann H, Wittinghofer A. GEFs and GAPs: critical elements in the control of small G proteins. Cell 2007; 129:865-77; PMID:17540168; https://doi.org/10.1016/j.cell.2007.05.018
- Machacek M, Hodgson L, Welch C, Elliott H, Pertz O, Nalbant P, Abell A, Johnson GL, Hahn KM, Danuser G. Coordination of Rho GTPase activities during cell protrusion. Nature 2009; 461:99-103; PMID:19693013; https://doi.org/10.1038/nature08242
- Gardel ML, Schneider IC, Aratyn-Schaus Y, Waterman CM. Mechanical integration of actin and adhesion dynamics in cell migration. Annu Rev Cell Dev Biol 2010; 26:315-33; PMID:19575647; https://doi.org/10.1146/annurev.cellbio.011209.122036
- Lim BC, Matsumoto S, Yamamoto H, Mizuno H, Kikuta J, Ishii M, Kikuchi A. Prickle1 promotes focal adhesion disassembly in cooperation with CLASP-LL5beta complex in migrating cells. J Cell Sci 2016; 16:3115-29; PMID:27378169; http://jcs.biologists.org/content/129/16/3115.long
- Schaefer A, Reinhard NR, Hordijk PL. Toward understanding RhoGTPase specificity: structure, function and local activation. Small GTPases 2014; 5:6; PMID:25483298; https://doi.org/10.4161/21541248.2014.968004
- Murrell M, Oakes PW, Lenz M, Gardel ML. Forcing cells into shape: the mechanics of actomyosin contractility. Nat Rev 2015; 16:486-98; PMID:26130009; https://doi.org/10.1038/nrm4012