ABSTRACT
The mechanistic target of rapamycin (mTOR) is an evolutionary conserved protein with a serine/threonine kinase activity that regulates cell growth, proliferation, motility, survival, protein synthesis, autophagy and transcription. It is embedded in 2 large protein complexes: mTORC1 and mTORC2. Regulation of specific mTOR pathway functions depends on multiple GTPases, that act either as regulators of mTOR protein complexes, coupling energy availability with mTORC1 activity, or as downstream effectors of both mTORC1 and mTORC2. In this commentary, we highlight the advantages of studying the mTOR pathway in C. elegans, including the subcellular localization of the signaling pathway components and the animal phenotypes following tissue specific protein over-expression or knockdown. One important regulator that is not limited to the mTOR pathway is RHEB. We discuss in vitro and in vivo data suggesting that RHEB can function as an inhibitor of mTOR when not bound to GTP. RHEB-1 itself is regulated by Rab GDP dissociation inhibitor β, which directly binds to ATX-2. We also highlight the roles of these proteins in dietary restriction-depended reduction in animal size and fat content.
GTPases in C. elegans
Caenorhabditis elegans is a model organism useful for studying GTPases in the context of a whole organism. The C. elegans genome contains 56 genes of various small GTPase subfamilies.Citation1 These include multiple members of the Rho, Rab, Ran, Arf/Sar and Ras/Ral/Rap families. Additionally, multiple regulators for the different families have been identified. For example, 19 Rho GTP exchange factors (GEFs) of the Dbl-homology family have been identified in C. elegans.Citation1 However, extensive identification of protein-protein interactions regulating all small GTPases is lacking. Studying GTPases in C. elegans has unique advantages such as the large gene knockout resources,Citation2 knockdown librariesCitation3 and extensively annotated and detailed genome-wide high-throughput knockdown experiments. C. elegans are translucent, and are currently the only multicellular organism with a fully mapped cell lineage. It is thus possible to track and measure protein expression levels in a tissue and cell specific manner, as well as protein dynamics in live animals. Moreover, comprehensive understanding of the way systemic RNAi works in nematodes enabled the development and distribution of strains where gene knockdown can be easily done in a tissue specific manner.Citation4
These tools were leveraged to study the roles of small GTPases as key regulators of C. elegans biology. For example, after Sakiko Honjoh et al. established intermittent fasting as a lifespan extending metabolic intervention in C. elegans, they were able to map RHEB-1 as a signaling protein mediating this effect.Citation5 RHEB-1 is the ortholog of human RHEB (Ras homolog enriched in brain; see below) and RHEBL1 GTPases, upstream regulators of mTOR. Downregulation of rheb-1 in C. elegans was sufficient to completely abolish the effect of intermittent fasting on lifespan, while having no effect on the lifespan of well-fed animals.Citation5 However, downregulation of let-363 (paralog of mTOR) only partially blocked lifespan extension by intermittent fasting, suggesting additional roles for RHEB.Citation5 Mutations in daf-16, the sole C. elegans forkhead box O (FOXO) transcription factor and master regulator of insulin/IGF-1-mediated signaling, suppressed most of the lifespan extension conveyed by intermittent fasting.Citation5 DAF-16 is known to translocate into the nucleus in intestinal cells under fasting conditions.Citation6 This nuclear translocation is essential for activating its targets. Imaging DAF-16 fused to GFP showed that the translocation is regulated by RHEB-1, mapping RHEB-1 to yet another lifespan regulating pathway.Citation5
Other examples of well-studied and tissue specific roles of GTPases in C. elegans include the K-ras homolog let-60 and its roles in vulval cell fate induction, and RHO-1 and CDC-42 control of embryonic development. An extensive review of small GTPase studies in C. elegans can be found in small GTPase chapter of wormbook.Citation1
GTPases in mTOR pathway
Mechanistic target of rapamycin (mTOR; formally mammalian target of rapamycin), first discovered as the molecular target of the lifespan extending drug rapamycin, is a phosphatidylinositol 3-kinase-related kinase.Citation7 It functions as the catalytic core of 2 protein complexes, mTORC1 and mTORC2. Together, the mTOR pathway integrates inputs about nutrient availability and cellular state in order to regulate multiple aspects of cell biology, including transcription, translation, autophagy and growth. mTORC1 and mTORC2 have distinct targets and functions, although in some cells prolonged inhibition of mTORC1 will inhibit mTORC2 assembly in an AKT/PKB dependent manner.Citation8
First discovered and better studied, mTORC1 has multiple key functions in cell growth and proliferation. It regulates protein synthesis via S6 Kinase (S6K) and translation initiation factor 4E-BP1, autophagy via ULK1 and ATG13 and microtubule organization via CLIP-170.Citation9,10 mTOR regulation of downstream GTPases is often indirect. For example, the yeast protein TOR2 (phosphatidylinositol kinase homolog and member of TORC1) activates RHO1/2 through ROM2, a Guanine nucleotide exchange factor.Citation11 mTORC1 is regulated by AMP kinase (AMPK), whose activity is dictated by the ATP/ADP ratio, as well as several small GTPases.
One of the well-studied regulators of the mTOR pathway is RHEB. This small GTPase localizes to membranes, in part via farnesylation and processing of its CAAX motif. Localization of RHEB to the surface of lysosomes is essential for its role in activating mTORC1, and inhibiting its farnesylation with farnesyltransferase inhibitors (FTIs) inhibits mTOR and S6 kinase phosphorylation.Citation12 This is of particular importance in treatment of patients with Hutchinson–Gilford progeria syndrome (HGPS). Both FTIs and mTOR inhibitors convey a positive effect in various HGPS disease models and in the clinic. However, the effects of FTIs on the mTOR pathway in these cases have not been well studied. Also, the effects of statins and amino bisphosphonates, which reduce the amount of farnesyl groups in cells, on mTOR signaling, have not been studied. RHEB favors the biologically active GTP-bound state due to its low intrinsic GTPase activity.Citation13 The hydrolysis of the GTP in GTP-bound RHEB is stimulated by the Tuberous sclerosis complex (TSC). Surprisingly, C. elegans has no clear homologs for TSC1, and TSC2 has only limited homology to SIPA-1. Localization of RHEB and mTORC1 to the surface of lysosomes facilitates RHEB-mTOR binding. This binding does not require GTP and nucleotide-deficient RHEB mutants can bind mTOR in vivo and in vitro.Citation14 Interestingly, these mutants show an inhibitory effect on mTOR activity. By contrast, mTOR bound to the RHEB mutant Rheb(Gln64Leu), which is nearly 90% GTP charged, is found predominantly in a GTP bound state and shows higher activity levels.Citation14 These results expand the classical view of GTPases as being active in a GTP bound state and passive in a GDP bound state and suggest that RHEB may act both as an activator and a repressor.
Another set of small GTPases regulating mTOR is RAS-related GTP-binding proteins (RAGs). When activated by specific amino acids such as Leucine, RAGA and RAGB are loaded with GTP and RAGC and RAGD with GDP.Citation15-17 The heterodimer of GTP and GDP bound small GTPases facilitates the translocation of mTORC1 to the lysosome surface, where it can be activated by GTP bound RHEB. Thus, spatial control of small GTPases and their target proteins appears to be a common mechanism for GTPase regulation.
The roles of mTORC2 were less studied. This complex is regulated by growth factors and insulin. Known functions of mTORC2 include regulation of cell metabolism and cytoskeleton via actin organization. Of note, mTORC2 regulation requires several Rho family GTPases, including RhoA, Rac1 and CDC42 to regulate cytoskeletal organization.Citation10,18,19 This is achieved by direct phosphorylation of protein kinase Cζ by mTORC2, which in turn controls the level of active Rho GTPases by mechanism not completely understood.Citation19
Ataxin-2/GDI2 complex regulates mTOR
When subjected to dietary restriction (DR), C. elegans grow to a smaller size, show slower rate of development, accumulate less fat, have fewer offspring and live longer. We noticed ATX-2 is required for many of these DR-induced phenotypes. ATX-2 is the ortholog of human Ataxin-2 proteins.Citation20 Expansion of the polyglutamine tract in Ataxin-2 in humans causes spinocerebellar ataxia type 2Citation21-24 and is associated with an elevated risk for amyotrophic lateral sclerosis (ALS),Citation25 however, the cellular functions of Ataxin-2 are largely unknown. Overexpression of Pbp1, the yeast ortholog of the human Ataxin-2 gene, represses mTORC1,Citation26 while low energy levels it causes PBP1 to sequester mTORC1 at stress granules.Citation27 In C. elegans, ATX-2 forms a complex with cytoplasmic polyA-binding protein (PAB-1) to regulate translation in the germline.Citation28 Knockdown of atx-2 in DR animals resulted in larger animals that developed at a normal pace and accumulated more fat.Citation29 This was confirmed in 3 different genetic models of DR, as well as under bacterial dilution conditions. Reciprocally, overexpression of ATX-2 was sufficient to reduce animal size in wild-type animals. Genetic mapping, using gene knockdown and null alleles, identified ATX-2 to function upstream of RAPTOR (daf-15; essential protein of mTORC1) and its target S6 kinase and downstream of AMPK (). Downregulation of RHEB-1 yielded results that looked initially counter intuitive; It increased the size of the small DR animals, but mildly decreased the size of well-fed animals. These results can be explained if we consider the possibility of RHEB-1 acting as a GTP-bound activator in well-fed animals and GDP-bound repressor in DR animals. Downregulation of RHEB-1 removes a repressor in DR animals, making them larger or removes an activator, making well-fed animals smaller. ATX-2 binds GDI-1, the homolog of human Rab GDP dissociation inhibitor β (GDI2). Indeed, downregulation of gdi-1 mimicked the effect of atx-2 knockdown, rescuing the size of DR animals. These results suggested that ATX-2 and GDI-1 regulate mTORC1 by locking RHEB-1 in a GDP bound state.Citation29 While ATX-2 regulates multiple aspects of DR, including cell and animal size, fat accumulation and developmental rate, there was no effect for ATX-2 on lifespan. DR, mTOR and S6 kinase are extensively studied regulators of aging in C. elegans. Thus uncoupling lifespan from other metabolic targets of mTOR is surprising and potentially important. However, these results need to be interpreted carefully. The extensive study of DR in C. elegans has identified multiple regulators of aging, however, different DR methods result in different dependencies. Genes essential for DR lifespan extension in one DR regime may not be needed in another. For example, daf-16 and aak-2 (AMPK catalytic subunit) are required for lifespan extension in “solid DR,” partially required for lifespan extension under bacterial dilution in liquid and not required when using eat-2 mutant animals, a common genetic model of DR.Citation30 It is thus possible that ATX-2 downregulation will abolish the lifespan extension under different DR conditions. The finding that ATX-2/GDI-1 regulates size and fat accumulation via mTORC1 may be relevant for mammals as well. Mice lacking Ataxin-2 show adult onset obesity, hepatosteatosis, dyslipidemia , elevated insulin levels, reduced insulin receptor proteins levels and enhanced phosphorylation of S6 kinase and 4E-BP1.Citation31-33 Moreover, stress causes Ataxin-2 to sequester mTORC1 in stress granulesCitation34 and recent work has independently suggested Ataxin-2 to regulate mTOR under nutritional stress.Citation31 Finally, the SH2B3 and ATNX2 containing human locus is associated with type 1 diabetes.Citation35
Figure 1. A schematic model of the mTOR pathway in C. elegans. The small GPTAses RHEB-1 and RAGA/C-1 are also shown.
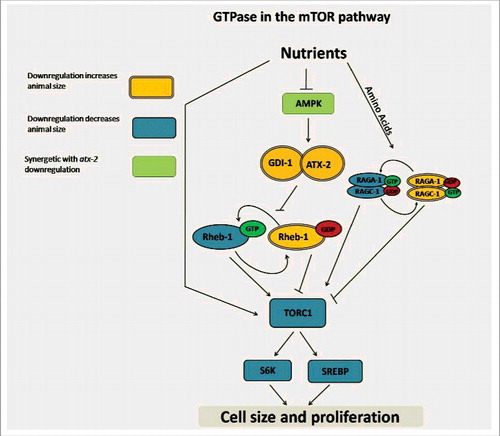
In conclusion, small GTPases play key regulatory roles in the mTOR pathway. They both couple mTORC1 to nutrient levels and regulate key functions downstream of mTORC1 and mTORC2.Citation9,10 Interestingly, this regulatory role is often facilitated by changes in protein localization.Citation5 RHEB-1, known for regulating mTORC1, is essential for intermittent fasting dependent increase in lifespan. Moreover, under these conditions RHEB-1 regulates the insulin-like signaling pathway, presumably independently of its regulation of mTOR.Citation5 While additional data is needed, the in vitro and in vivo data suggest RHEB has a dual function, both as an activator and as a repressor.Citation14,29 When subjected to DR, RHEB-1 is locked in a GDP bound state by ATX-2 and GDI-1. Knockdown of a specific regulator, atx-2, uncoupled multiple metabolic effects from lifespan regulation.Citation29 Rapamycin, while prolonging the life of mice, has limited use in humans due to side effects that include hyperglycemia, hyperlipidemia, insulin resistance and increased risk for type-2 diabetes.Citation36 These results suggest it may be possible to uncouple the lifespan effects of RHEB/mTOR from its control over fat accumulation and cell size.Citation29
Acknowledgments
We thank Noam Zuela for critical reading of the manuscript.
Funding
This work was supported by grants from the Niedersachsen-Israeli Research Cooperation, The German Israel Foundation (GIF 1-223-2014) and Israel Science Foundation (785/15).
References
- Reiner DJ, Lundquist EA. Small GTPases. WormBook 2016:1-99; PMID:27218782; https://doi.org/10.1895/wormbook.1.67.2
- Consortium CeDM. large-scale screening for targeted knockouts in the Caenorhabditis elegans genome. G3 2012; 2:1415-25; PMID:23173093; https://doi.org/10.1534/g3.112.003830
- Kamath RS, Ahringer J. Genome-wide RNAi screening in Caenorhabditis elegans. Methods 2003; 30:313-21; PMID:12828945; https://doi.org/10.1016/S1046-2023(03)00050-1
- Qadota H, Inoue M, Hikita T, Koppen M, Hardin JD, Amano M, Moerman DG, Kaibuchi K. Establishment of a tissue-specific RNAi system in C. elegans. Gene 2007; 400:166-73; PMID:17681718; https://doi.org/10.1016/j.gene.2007.06.020
- Honjoh S, Yamamoto T, Uno M, Nishida E. Signalling through RHEB-1 mediates intermittent fasting-induced longevity in C. elegans. Nature 2009; 457:726-30; PMID:19079239; https://doi.org/10.1038/nature07583
- Oh SW, Mukhopadhyay A, Svrzikapa N, Jiang F, Davis RJ, Tissenbaum HA. JNK regulates lifespan in Caenorhabditis elegans by modulating nuclear translocation of forkhead transcription factor/DAF-16. Proc Natl Acad Sci U S A 2005; 102:4494-9; PMID:15767565; https://doi.org/10.1073/pnas.0500749102
- Heitman J, Movva NR, Hall MN. Targets for cell cycle arrest by the immunosuppressant rapamycin in yeast. Science 1991; 253:905-9; PMID:1715094; https://doi.org/10.1126/science.1715094
- Sarbassov DD, Ali SM, Sengupta S, Sheen JH, Hsu PP, Bagley AF, Markhard AL, Sabatini DM. Prolonged rapamycin treatment inhibits mTORC2 assembly and Akt/PKB. Mol Cell 2006; 22:159-68; PMID:16603397; https://doi.org/10.1016/j.molcel.2006.03.029
- Hall MN. mTOR-what does it do? Transplant Proc 2008; 40:S5-8; PMID:19100909; https://doi.org/10.1016/j.transproceed.2008.10.009
- Laplante M, Sabatini DM. mTOR signaling at a glance. J Cell Sci 2009; 122:3589-94; PMID:19812304; https://doi.org/10.1242/jcs.051011
- Schmidt A, Bickle M, Beck T, Hall MN. The yeast phosphatidylinositol kinase homolog TOR2 activates RHO1 and RHO2 via the exchange factor ROM2. Cell 1997; 88:531-42; PMID:9038344; https://doi.org/10.1016/S0092-8674(00)81893-0
- Basso AD, Mirza A, Liu G, Long BJ, Bishop WR, Kirschmeier P. The farnesyl transferase inhibitor (FTI) SCH66336 (lonafarnib) inhibits Rheb farnesylation and mTOR signaling. Role in FTI enhancement of taxane and tamoxifen anti-tumor activity. J Biol Chem 2005; 280:31101-8; PMID:16006564; https://doi.org/10.1074/jbc.M503763200
- Mazhab-Jafari MT, Marshall CB, Ishiyama N, Ho J, Di Palma V, Stambolic V, Ikura M. An autoinhibited noncanonical mechanism of GTP hydrolysis by Rheb maintains mTORC1 homeostasis. Structure 2012; 20:1528-39; PMID:22819219; https://doi.org/10.1016/j.str.2012.06.013
- Long X, Lin Y, Ortiz-Vega S, Yonezawa K, Avruch J. Rheb binds and regulates the mTOR kinase. Curr Biol 2005; 15:702-13; PMID:15854902; https://doi.org/10.1016/j.cub.2005.02.053
- Shimobayashi M, Hall MN. Making new contacts: the mTOR network in metabolism and signalling crosstalk. Nat Rev Mol Cell Biol 2014; 15:155-62; PMID:24556838; https://doi.org/10.1038/nrm3757
- Sancak Y, Peterson TR, Shaul YD, Lindquist RA, Thoreen CC, Bar-Peled L, Sabatini DM. The Rag GTPases bind raptor and mediate amino acid signaling to mTORC1. Science 2008; 320:1496-501; PMID:18497260; https://doi.org/10.1126/science.1157535
- Kim E, Goraksha-Hicks P, Li L, Neufeld TP, Guan KL. Regulation of TORC1 by Rag GTPases in nutrient response. Nat Cell Biol 2008; 10:935-45; PMID:18604198; https://doi.org/10.1038/ncb1753
- He Y, Li D, Cook SL, Yoon MS, Kapoor A, Rao CV, Kenis PJ, Chen J, Wang F. Mammalian target of rapamycin and Rictor control neutrophil chemotaxis by regulating Rac/Cdc42 activity and the actin cytoskeleton. Mol Biol Cell 2013; 24:3369-80; PMID:24006489; https://doi.org/10.1091/mbc.E13-07-0405
- Li X, Gao T. mTORC2 phosphorylates protein kinase Czeta to regulate its stability and activity. EMBO Rep 2014; 15:191-8; PMID:24375676
- Kiehl TR, Shibata H, Pulst SM. The ortholog of human ataxin-2 is essential for early embryonic patterning in C. elegans. J Mol Neurosci 2000; 15:231-41; PMID:11303786; https://doi.org/10.1385/JMN:15:3:231
- Imbert G, Saudou F, Yvert G, Devys D, Trottier Y, Garnier JM, Weber C, Mandel JL, Cancel G, Abbas N, et al. Cloning of the gene for spinocerebellar ataxia 2 reveals a locus with high sensitivity to expanded CAG/glutamine repeats. Nat Genet 1996; 14:285-91; PMID:8896557; https://doi.org/10.1038/ng1196-285
- Pulst SM, Nechiporuk A, Nechiporuk T, Gispert S, Chen XN, Lopes-Cendes I, Pearlman S, Starkman S, Orozco-Diaz G, Lunkes A, et al. Moderate expansion of a normally biallelic trinucleotide repeat in spinocerebellar ataxia type 2. Nat Genet 1996; 14:269-76; PMID:8896555; https://doi.org/10.1038/ng1196-269
- Sanpei K, Takano H, Igarashi S, Sato T, Oyake M, Sasaki H, Wakisaka A, Tashiro K, Ishida Y, Ikeuchi T, et al. Identification of the spinocerebellar ataxia type 2 gene using a direct identification of repeat expansion and cloning technique, DIRECT. Nat Genet 1996; 14:277-84; PMID:8896556; https://doi.org/10.1038/ng1196-277
- Lorenzetti D, Bohlega S, Zoghbi HY. The expansion of the CAG repeat in ataxin-2 is a frequent cause of autosomal dominant spinocerebellar ataxia. Neurology 1997; 49:1009-13; PMID:9339681; https://doi.org/10.1212/WNL.49.4.1009
- Elden AC, Kim HJ, Hart MP, Chen-Plotkin AS, Johnson BS, Fang X, Armakola M, Geser F, Greene R, Lu MM, et al. Ataxin-2 intermediate-length polyglutamine expansions are associated with increased risk for ALS. Nature 2010; 466:1069-75; PMID:20740007; https://doi.org/10.1038/nature09320
- Takahara T, Maeda T. Transient sequestration of TORC1 into stress granules during heat stress. Mol Cell 2012; 47:242-52; PMID:22727621; https://doi.org/10.1016/j.molcel.2012.05.019
- DeMille D, Badal BD, Evans JB, Mathis AD, Anderson JF, Grose JH. PAS kinase is activated by direct SNF1-dependent phosphorylation and mediates inhibition of TORC1 through the phosphorylation and activation of Pbp1. Mol Biol Cell 2015; 26:569-82; PMID:25428989; https://doi.org/10.1091/mbc.E14-06-1088
- Ciosk R, DePalma M, Priess JR. ATX-2, the C. elegans ortholog of ataxin 2, functions in translational regulation in the germline. Development 2004; 131:4831-41; PMID:15342467; https://doi.org/10.1242/dev.01352
- Bar DZ, Charar C, Dorfman J, Yadid T, Tafforeau L, Lafontaine DL, Gruenbaum Y. Cell size and fat content of dietary-restricted Caenorhabditis elegans are regulated by ATX-2, an mTOR repressor. Proc Natl Acad Sci U S A 2016; 113:E4620-9; PMID:27457958; https://doi.org/10.1073/pnas.1512156113
- Greer EL, Brunet A. Different dietary restriction regimens extend lifespan by both independent and overlapping genetic pathways in C. elegans. Aging Cell 2009; 8:113-27; PMID:19239417; https://doi.org/10.1111/j.1474-9726.2009.00459.x
- Lastres-Becker I, Nonis D, Eich F, Klinkenberg M, Gorospe M, Kotter P, Klein FA, Kedersha N, Auburger G. Mammalian ataxin-2 modulates translation control at the pre-initiation complex via PI3K/mTOR and is induced by starvation. Biochim Biophys Acta 2016; 1862:1558-69; PMID:27240544; https://doi.org/10.1016/j.bbadis.2016.05.017
- Kiehl TR, Nechiporuk A, Figueroa KP, Keating MT, Huynh DP, Pulst SM. Generation and characterization of Sca2 (ataxin-2) knockout mice. Biochem Biophys Res Commun 2006; 339:17-24; PMID:16293225; https://doi.org/10.1016/j.bbrc.2005.10.186
- Fittschen M, Lastres-Becker I, Halbach MV, Damrath E, Gispert S, Azizov M, Walter M, Müller S, Auburger G. Genetic ablation of ataxin-2 increases several global translation factors in their transcript abundance but decreases translation rate. Neurogenetics 2015; 16:181-92; PMID:25721894; https://doi.org/10.1007/s10048-015-0441-5
- Meierhofer D, Halbach M, Sen NE, Gispert S, Auburger G. Ataxin-2 (Atxn2)-Knock-Out Mice Show Branched Chain Amino Acids and Fatty Acids Pathway Alterations. Mol Cell Proteomics 2016; 15:1728-39; https://doi.org/10.1074/mcp.M115.056770
- Auburger G, Gispert S, Lahut S, Omur O, Damrath E, Heck M, Başak N. 12q24 locus association with type 1 diabetes: SH2B3 or ATXN2? World J Diabetes 2014; 5:316-27; PMID:24936253; https://doi.org/10.4239/wjd.v5.i3.316
- Salmon AB. About-face on the metabolic side effects of rapamycin. Oncotarget 2015; 6:2585-6; PMID:25691064; https://doi.org/10.18632/oncotarget.3354