ABSTRACT
Modes of cancer invasion interchange between the mesenchymal type and amoeboid type in response to the microenvironment, in which RhoA and Rac1 are selectively required to perform different modes of actin-cytoskeletal remodeling. Membrane remodeling is another integral part of invasion. Arf6 regulates the recycling of molecules at the cell periphery, and is often overexpressed in malignant cancers together with its effector AMAP1/ASAP1/DDEF1. This pathway promotes mesenchymal-type invasion when AMAP1 binds to EPB41L5, a mesenchymal-specific protein induced by ZEB1. Here we show that the Arf6-AMAP1-EPB41L5 pathway, and ZEB1, are also crucial for amoeboid-type invasion, via receptor tyrosine kinase and G-protein-coupled receptor signaling. Thus, Arf6 appears to be necessary for both RhoA- and Rac1-driven cancer invasion. Moreover, amoeboid-type cancer invasion may require the activation of some type of mesenchymal program within the cancer cells.
More than 2 decades ago, Ridley and Hall found that lysophosphatidic acid (LPA) activates the small GTPase RhoA; and that RhoA and other small-GTPases, such as Rac1, play pivotal roles in the remodeling of actin-based cytoskeletal architecture.Citation1,2 Prompted by this finding, a substantial number of studies have been performed on the molecular bases of cell migration, that is, understanding the precise roles of small GTPases, as well as extracellular stimulations regulating small GTPases, in cytoskeletal remodeling and cell migration, as well as in cancer invasion and metastasis. LPA is a lipid mediator that is produced extracellularly from lysophosphatidic choline, which is abundant in body fluids, by the ectoenzyme autotaxin. Autotaxin was originally identified as a factor that promotes tumor cell motility,Citation3 and is also called ectonucleotide pyrophosphatase/phosphodiesterase family member 2, produced by different tissues and different types of cancers. This enzyme has a hydrophobic pocket containing the catalytic domain, which also functions as a hydrophobic channel to transfer de novo synthesized LPA molecules to their cognate G-protein coupled receptors (GPCRs).Citation4 This feature of autotaxin appears to ensure the characteristic of LPA to only act locally upon its production. We have recently demonstrated that LPA also directly activates Arf6 (see below).Citation5
RhoA, Rac1, and Arf6, are all critical to the 2-dimensional cell migration of adherent cells, such as epithelial cells and fibroblasts.Citation6,7 In 2-dimensional migration, RhoA is primarily involved in the formation of robust bundled structures of actin fibers (i.e., actin stress fibers) to generate a contractile force particularly at the rear ends,Citation8 whereas Rac1 is primarily involved in the formation of actin-meshwork structures to promote membrane ruffling and protrusions at the front ends.Citation9 On the other hand, cell migration may also require the active supplementation of membrane components to the leading edges.Citation10 Arf6 primarily regulates the recycling of plasma membrane components at the cell periphery.Citation11 Arf6 was demonstrated to be a prerequisite for Rac1-mediated membrane ruffling and protrusion, which thus emphasized the importance of membrane remodeling in migration.Citation12
Invasion (i.e., 3-dimensional cell migration penetrating into extracellular environments) is a very different mode of cell motility compared with 2-dimensional migration. Two different types of cancer cell invasion have been described: mesenchymal-type invasion and amoeboid-type invasion.Citation13,14 Mesenchymal-type invasion requires integrins and their binding to the cognate extracellular matrixes (ECMs), as well as the degradation of ECMs by specific matrix metalloproteinases, whereas amoeboid-type invasion does not require either of these activities. RhoA and Rac1 have been shown to be differently required between mesenchymal invasion and amoeboid invasion. It was demonstrated that a ROCK inhibitor blocks the amoeboid invasion of HT1080 fibrosarcoma cells, which is induced by the treatment of cells with protease inhibitors, although the ROCK inhibitor does not block mesenchymal invasion of the same cells.Citation15 Thus, RhoA appears to be preferentially required for amoeboid invasion rather than mesenchymal invasion.Citation15 It should be noted, however, that RhoA may also participate in mesenchymal invasion to some extent.Citation16 Mechanistically, it was reported that RhoA and ROCK-dependent myosin light chain phosphorylation is crucial for promoting amoeboid invasion.Citation14,17,18 More recently, it was shown that the increased hydrostatic pressure induced at the moving ends by RhoA-ROCK-mediated contractile forces drives the blebbing in membrane protrusion (i.e., bleb-driven migration).Citation7,19,20 On the other hand, Rac1 and its guanine nucleotide exchange factor DOCK3 may drive cancer mesenchymal invasion via WAVE2, as has been demonstrated in melanomas.Citation21 Interestingly, Rac1-WAVE2 signaling suppresses actomyosin-based cell contractility and hence blocks RhoA-mediated amoeboid invasion.Citation21 On the other hand, RhoA-ROCK signaling activates a GTPase-activating protein for Rac-GTPases, namely ARHGAP22, and thereby suppresses Rac1-mediated mesenchymal invasion.Citation21 Therefore, RhoA and Rac1 appear to alternately drive the 2 modes of invasion, by reciprocally blocking each other. Recent studies have shown that not only these intrinsic factors but also extracellular factors, such as matrix stiffness and cell-ECM adhesion, may trigger mesenchymal-amoeboid transition.Citation22,23
The small GTPase Arf6 is essential for cell motility.Citation6 Arf6 and its signaling proteins are often overexpressed in different types of cancers, including those of the breast, kidney, head and neck, and lung, and drive their mesenchymal invasion and metastasis.Citation5,24-29 In breast cancer, the overexpression of receptor tyrosine kinases (RTKs), such as epidermal growth factor receptor (EGFR) and hepatocyte growth factor receptor (HGFR/c-Met), are risk factors of malignancy.Citation30-32 We showed that these RTKs activate Arf6, in which ligand-activated RTKs directly recruit a guanine nucleotide exchanger, namely guanine nucleotide-exchange protein 100 (GEP100).Citation27 GTP-Arf6 then recruits a multiple-domain arf-GAP protein 1 (AMAP1), which is also called ASAP1/DDEF1, to transmit the downstream signals.Citation26 In invasion and metastasis, AMAP1 binds to protein kinase D2 (PRKD2) to promote the recycling of integrins,Citation33 and also binds to cortactin and paxillin to remodel the actin cytoskeleton and focal adhesions.Citation34 AMAP1 may also bind to erythrocyte membrane protein band 4.1-like 5 (EPB41L5), which is a mesenchymal-specifc protein that is normally induced during epithelial-mesenchymal transition (EMT).Citation35 EPB41L5 then binds to p120 catenin to sequester this molecule from binding to E-cadherin, thus leading to E-cadherin internalization.Citation35 EPB41L5 also binds to paxillin to promote focal adhesion dynamics.Citation35
Enhanced RTK signaling does not appear to be a strong risk factor for renal cancer. In renal cancer cells, we found that LPA is a potent activator of Arf6, and thereby promotes invasion and metastasis.Citation5 In this process, GTP-Gα12, which is released from LPA-activated GPCRs, binds EFA6 to activate Arf6 and its downstream signaling involving AMAP1 and EPB41L5.Citation5 We have demonstrated that the Arf6-based pathway, activated by either ligand, is crucial not only for invasion and metastasis, but also for promoting the drug resistance of cancer cells.Citation5,36 On the other hand, there were reports that EMT might not be necessary for cancer invasion and metastasis, although it is crucial for drug resistance. Contrary to these reports, our results clearly illustrate that EMT is crucial for both invasion/metastasis and drug resistance.Citation37,38
Mesenchymal invasion and amoeboid invasion are interchangeable within a single cancer cell (i.e., mesenchymal-amoeboid transition), depending on the environmental conditions. For example, the relatively low usage of integrins with regard to their binding to ECMs (i.e., low adhesion) may induce amoeboid-type invasion of cancer cells.Citation13 Moreover, the treatment of cancer cells with protease inhibitors may switch cells from using mesenchymal invasion to using amoeboid invasion.Citation13 On the other hand, certain types of cancers, such as small cell lung carcinomas, which demonstrate undifferentiated, stem-cell like phenotypes rather than being mesenchymal-like, have been shown to utilize amoeboid-type invasion even in the absence of protease inhibitors.Citation39 Furthermore, it should be noted that cancer cells might require the membrane-anchored metalloproteinase MT1-MMP, even for their ‘classical’ amoeboid-type invasion (i.e., invasiveness that is largely independent of protease activity).Citation40
We here examined whether the Arf6-based pathway also drives amoeboid invasion. MDA-MB-231 cells exhibit amoeboid invasion in the presence of protease inhibitors (also see ).Citation13 We found that the silencing of Arf6 and AMAP1 each effectively blocks EGF-induced invasion of MDA-MB-231 cells into collagen matrices, in the presence of protease inhibitors (). Moreover, the mesenchymal-specific EPB41L5 was also found to be critically involved in the protease-independent invasion of MDA-MB-231 cells (). ZEB1, which is a EMT-related transcriptional factor, is primarily responsible to induce the EPB41L5 gene in human breast cancer, including MDA-MB-231 cells.Citation41 Silencing of ZEB1 also blocked the EGF-induced invasion in the presence of protease inhibitors (). We have shown recently that LPA activates Arf6 to promote the mesenchymal invasion of renal cancer cells, in which RhoA, but not Rac1, is dispensable.Citation5 In zebrafish germline cells, LPA induces the cell polarization of bleb-driven cell migration.Citation23 We found that LPA also promotes the invasion of MDA-MB-231 cells in the presence of protease inhibitors, in which the silencing of EPB41L5 and ZEB1, as well as Arf6 and AMAP1, each blocks invasion (). As a control, we confirmed that silencing GEP100, a product of which activates Arf6 by binding to RTKs, did not at all block the LPA-induced invasion, conducted in the presence of protease inhibitors (). Therefore, collectively, these results demonstrated that the Arf6-based mesenchymal program, which involves ZEB1, is also pivotal to drive amoeboid-type invasion of some cancer cells in response to RTK and GPCR signaling.
Figure 1. Arf6-based mesenchymal pathway and ZEB1 promote amoeboid invasion of MDA-MB-231 cells in response to EGF or LPA. (A) Morphologies of cells cultured on fibrillar collagen in the absence or presence of a protease inhibitor mix (PtdIns mix). Bars, 20 µm. Ratios of MDA-MB-231 cells with an elongated or round shape, in the absence or presence of a PI mix, are shown in the graph on the right. More than 300 cells were examined in each assay. (B) Invasion through fibrillar collagen in the presence of a PtdIns mix, in response to EGF or LPA. Cells were pretreated with the indicated siRNAs. (C) Protein expression in the siRNA-treated cells was detected by immunoblotting. (D) Cell viabilities of siRNA-treated cells.
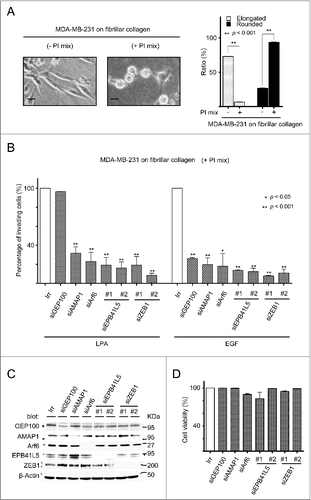
Our results described above and reports in the literature collectively indicated that Arf6 and its signaling pathway promote both mesenchymal-type invasion and amoeboid-type invasion, in which Arf6 can be activated by RTKs or by LPA (). Our results moreover demonstrate that amoeboid-type cancer invasion may require the Arf6-based mesenchymal program. Therefore, it is likely that EMT-like change is a prerequisite for both mesenchymal-type invasion and amoeboid-type invasion of some cancer cells. Amoeboid-type invasion may require the anchorage-independent survival of cancer cells. Closely associated with this, the acquisition of mesenchymal phenotypes by epithelial cells is coupled with their resistance to anoikis to a certain extent.Citation42,43 Moreover, resistance to anoikis might help to maintain cell survival during the process of distant metastasis of cancer cells. We have shown that EPB41L5 is also necessary for the distant metastasis of different cancer cells.Citation5,41 Molecular mechanisms by which EPB41L5 plays a crucial role in amoeboid-type invasion, as well as in anoikis resistance, require further experimental scrutiny.
Figure 2. Requirement of the Arf6-based mesenchymal pathway in both mesenchymal-type and amoeboid-type cancer invasion. Several receptor tyrosine kinases (RTKs), including those for epidermal growth factor (EGF) and hepatocyte growth factor (HGF), as well as G-protein-coupled receptors (GPCRs) for lysophosphatidic acid (LPA), are known to activate RhoA and Rac1 to promote cancer invasiveness; in which RhoA is preferentially required for amoeboid-type invasion, whereas Rac1 is required for mesenchymal-type invasion. We show that the Arf6-AMAP1-EPB41L5 pathway, activated either by EGF or by LPA, is essential for both mesenchymal-type and amoeboid-type invasion. EPB41L5 is a mesenchymal-specific protein primarily induced by ZEB1 in breast cancer. ZEB1, as well as EPB41L5, are also necessary for both mesenchymal-type and amoeboid-type invasion. We propose that, for cancer therapeutics, blocking Arf6, such as by inhibition of the mevalonate pathway, might be more effective than blocking RhoA or Rac1. It should be noted that blocking Arf6 may also effectively decrease the drug resistance of cancer cells, if cells overexpress the Arf6-based pathway (see text).
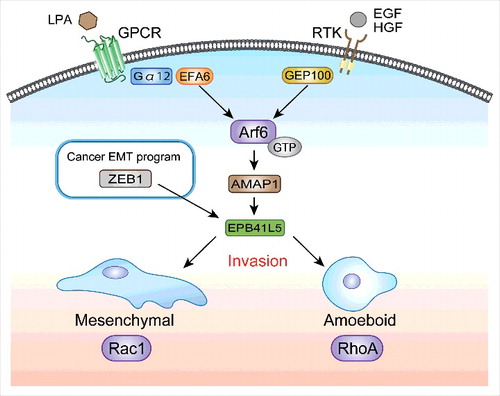
Different types of drugs, including protease inhibitors, have been extensively developed with an aim to block tumor invasion and metastasis. However, metalloproteinase inhibitors have turned out to be ineffective and not beneficial in cancer treatment.Citation44 The plasticity of cancer cells during tumor invasion between the mesenchymal mode and the amoeboid mode appears to be the major cause of the ineffectiveness of protease inhibitors. Moreover, each tumor might not express only a single type of proteinase for invasion.Citation45 We have shown recently that enhancement of the cellular mevalonate pathway is crucial for efficient activation of overexpressed Arf6 by overexpressed RTKs, and demonstrated that inhibition of this lipid metabolism, such as by statins, which inhibit the rate-limiting enzyme HMG-CoA reductase, is very effective for blocking the invasion and metastasis of breast cancer cells, if cells overexpress Arf6 and its signaling proteins.Citation36 Moreover, in our in vitro experimental system, 1 nM of statins was found to be sufficient to improve the drug resistance of cancer cells by about 100-1000-fold, if cells overexpress the Arf6-based pathway.Citation36 We are currently investigating whether the use of statins coupled with certain anti-cancer drugs in the clinical setting is beneficial for patients with breast cancers that overexpress Arf6 and its signaling pathway. We are also investigating methods by which Arf6 activation by GPCR-signaling can be blocked ().
Materials and methods
Cells
MDA-MB-231 cells were purchased from American Type Cell Culture. The cells were cultured at 37°C in a 1:1 mixture of DMEM (Invitrogen) and RPMI 1640 (Invitrogen), with 10% fetal calf serum (HyClone) and 5% NU serum (BD Biosciences).
Cell viabilities were measured using a cell counting kit (CCK-8; Dojindo) following the manufacturer's instructions. The protein concentration of cell lysates was measured using a coomassie protein assay kit (Thermo Fisher Scientific).
Reagents
Bovine collagen solution, type-I, 3 mg/ml (PureCol) was purchased from Advanced BioMatrix. The amoeboid invasion assay was performed using the following protease inhibitor mix (PI mix)Citation13: GM6001 (50 mM; sc-203979, Santa Cruz Biotechnology), E64 (250 mM; E3132, Sigma-Aldrich), pepstatin A (100 mM; P5318, Sigma-Aldrich), leupeptin (2 mM; L9783, Sigma-Aldrich), and aprotinin (2.2 mM; A4529, Sigma-Aldrich). Lysophosphatidic acid was purchased from Santa Crus and epithelial growth factor was from PeproTech.
Antibodies and immunoblotting
Rabbit polyclonal antibodies against AMAP1, GEP100, and EPB41L5 were described previously.Citation5,26,27 Other antibodies were obtained from commercial sources, as follows: ZEB1 (#3396, Cell Signaling Technology), and β-actin (#A5441, Sigma-Aldrich). Donkey antibodies against rabbit and mouse IgGs, each conjugated with horseradish peroxidase, were from Jackson ImmunoResearch Laboratories. Immunoblotting analysis was performed using ECL Western Detection Reagents (GE Healthcare) as described previously.Citation29
siRNA
Gene silencing by siRNA was performed as described previously.Citation36 In brief, cells were transfected with 50 nM of each siRNA duplex using Lipofectamine 2000 or Lipofectamine RNAiMax (Invitrogen) according to the manufacturer's instructions, and incubated for 2 d before the amoeboid invasion assay. Duplex oligonucleotides were chemically synthesized and purified by Japan BioService. Two different sequences were used for EPB41L5 and ZEB1. For the silencing of ZEB1, ZEB1 stealth siRNAs (ZEB1-HSS110548 and ZEB1-HSS110549) were used, together with a negative control Stealth RNAi duplex with medium GC content (Invitrogen).
Amoeboid invasion assay and quantification
The amoeboid invasion assay was performed according to a previously described method.Citation15 Briefly, 1 mg/ml fibrillar type-I collagen gels were prepared using a 3 mg/ml collagen solution, 4 × DMEM, 0.1 N NaOH, and double-distilled water. For the morphology assay, MDA-MB-231 cells were cultured on the top of fibrillar collagen gels with or without a PI mix. After incubation for 2 days, cells were observed using a microscope (EVOS FL Cell Imaging System). MDA-MB-231 cells (1.0 × 105), pretreated with the indicated siRNAs, were seeded onto the top of 3-dimensional fibrillar collagen gels, which were set within the upper chamber of a 24-well Transwell plate (Corning) in the presence of a PI mix. The lower chamber was filled with medium containing LPA (2 ng/ml) or EGF (10 ng/ml). After incubation for 3 days, cells were stained with 4 μM calcein-AM (Dojindo), and z-section images (5 μm intervals) from the surface of fibrillar collagen gels down to 100 µm were acquired using a confocal microscope equipped with a Plan Fluor 10 objective, and analyzed by the attached software (Nikon A1R with NIS-Elements). Cell invasion activities were quantified as the percentage of cells invading between 40 µm and 100 µm of the collagen gels, in which 2 different fields were counted in 2 independent experiments. All data were analyzed by the unpaired t-test, and p < 0.05 was considered to indicate a statistically significant difference between 2 groups. All graphs were created using Prism 6.0 (GraphPad Software).
Disclosure of potential conflicts of interest
No potential conflicts of interest were disclosed
Acknowledgments
We thank Y. Kado and E. Hayashi for their assistance, and H. A. Popiel for her critical reading of the manuscript.
Additional information
Funding
References
- Ridley AJ, Hall A. The small GTP-binding protein rho regulates the assembly of focal adhesions and actin stress fibers in response to growth factors. Cell 1992; 70:389-99; PMID:1643657; http://dx.doi.org/10.1016/0092-8674(92)90163-7
- Ridley AJ, Paterson HF, Johnston CL, Diekmann D, Hall A. The small GTP-binding protein rac regulates growth factor-induced membrane ruffling. Cell 1992; 70:401-10; PMID:1643658; http://dx.doi.org/10.1016/0092-8674(92)90164-8
- Stracke ML, Krutzsch HC, Unsworth EJ, Arestad A, Cioce V, Schiffmann E, Liotta LA. Identification, purification, and partial sequence analysis of autotaxin, a novel motility-stimulating protein. J Biol Chem 1992; 267:2524-9; PMID:1733949
- Nishimasu H, Okudaira S, Hama K, Mihara E, Dohmae N, Inoue A, Ishitani R, Takagi J, Aoki J, Nureki O. Crystal structure of autotaxin and insight into GPCR activation by lipid mediators. Nat Struct Mol Biol 2011; 18:205-12; PMID:21240269; http://dx.doi.org/10.1038/nsmb.1998
- Hashimoto S, Mikami S, Sugino H, Yoshikawa A, Hashimoto A, Onodera Y, Furukawa S, Handa H, Oikawa T, Okada Y, et al. Lysophosphatidic acid activates Arf6 to promote the mesenchymal malignancy of renal cancer. Nat Commun 2016; 7:10656; PMID:26854204; http://dx.doi.org/10.1038/ncomms10656
- Sabe H. Requirement for Arf6 in cell adhesion, migration, and cancer cell invasion. J Biochem 2003; 134:485-9; PMID:14607973; http://dx.doi.org/10.1093/jb/mvg181
- Ridley AJ. Rho GTPase signalling in cell migration. Curr Opin Cell Biol 2015; 36:103-12; PMID:26363959; http://dx.doi.org/10.1016/j.ceb.2015.08.005
- Raftopoulou M, Hall A. Cell migration: Rho GTPases lead the way. Dev Biol 2004; 265:23-32; PMID:14697350; http://dx.doi.org/10.1016/j.ydbio.2003.06.003
- Ridley AJ, Schwartz MA, Burridge K, Firtel RA, Ginsberg MH, Borisy G, Parsons JT, Horwitz AR. Cell migration: integrating signals from front to back. Science 2003; 302:1704-9; PMID:14657486; http://dx.doi.org/10.1126/science.1092053
- Ridley AJ. Life at the leading edge. Cell 2011; 145:1012-22; PMID:21703446; http://dx.doi.org/10.1016/j.cell.2011.06.010
- D'Souza-Schorey C, van Donselaar E, Hsu VW, Yang C, Stahl PD, Peters PJ. ARF6 targets recycling vesicles to the plasma membrane: insights from an ultrastructural investigation. J Cell Biol 1998; 140:603-16; PMID:9456320; http://dx.doi.org/10.1083/jcb.140.3.603
- Radhakrishna H, Al-Awar O, Khachikian Z, Donaldson JG. ARF6 requirement for Rac ruffling suggests a role for membrane trafficking in cortical actin rearrangements. J Cell Sci 1999; 112(Pt 6):855-66; PMID:10036235
- Wolf K, Mazo I, Leung H, Engelke K, von Andrian UH, Deryugina EI, Strongin AY, Bröcker E-B, Friedl P. Compensation mechanism in tumor cell migration: mesenchymal–amoeboid transition after blocking of pericellular proteolysis. J Cell Biol 2003; 160:267-77; PMID:12527751; http://dx.doi.org/10.1083/jcb.200209006
- Sahai E, Marshall CJ. Differing modes of tumour cell invasion have distinct requirements for Rho/ROCK signalling and extracellular proteolysis. Nat Cell Biol 2003; 5:711-9; PMID:12844144; http://dx.doi.org/10.1038/ncb1019
- Carragher NO, Walker SM, Scott Carragher LA, Harris F, Sawyer TK, Brunton VG, Ozanne BW, Frame MC. Calpain 2 and Src dependence distinguishes mesenchymal and amoeboid modes of tumour cell invasion: a link to integrin function. Oncogene 2006; 25:5726-40; PMID:16652152; http://dx.doi.org/10.1038/sj.onc.1209582
- Lamouille S, Xu J, Derynck R. Molecular mechanisms of epithelial-mesenchymal transition. Nat Rev Mol Cell Biol 2014; 15:178-96; PMID:24556840; http://dx.doi.org/10.1038/nrm3758
- Sedgwick AE, Clancy JW, Olivia Balmert M, D'Souza-Schorey C. Extracellular microvesicles and invadopodia mediate non-overlapping modes of tumor cell invasion. Scientific reports 2015; 5:14748; PMID:26458510; http://dx.doi.org/10.1038/srep14748
- Schlienger S, Campbell S, Claing A. ARF1 regulates the Rho/MLC pathway to control EGF-dependent breast cancer cell invasion. Mol Biol Cell 2014; 25:17-29; PMID:24196838; http://dx.doi.org/10.1091/mbc.E13-06-0335
- Charras G, Paluch E. Blebs lead the way: how to migrate without lamellipodia. Nat Rev Mol Cell Biol 2008; 9:730-6; PMID:18628785; http://dx.doi.org/10.1038/nrm2453
- Pinner S, Sahai E. Imaging amoeboid cancer cell motility in vivo. J Microsc 2008; 231:441-5; PMID:18754999; http://dx.doi.org/10.1111/j.1365-2818.2008.02056.x
- Sanz-Moreno V, Gadea G, Ahn J, Paterson H, Marra P, Pinner S, Sahai E, Marshall CJ. Rac activation and inactivation control plasticity of tumor cell movement. Cell 2008; 135:510-23; PMID:18984162; http://dx.doi.org/10.1016/j.cell.2008.09.043
- Liu YJ, Le Berre M, Lautenschlaeger F, Maiuri P, Callan-Jones A, Heuze M, Takaki T, Voituriez R, Piel M. Confinement and low adhesion induce fast amoeboid migration of slow mesenchymal cells. Cell 2015; 160:659-72; PMID:25679760; http://dx.doi.org/10.1016/j.cell.2015.01.007
- Ruprecht V, Wieser S, Callan-Jones A, Smutny M, Morita H, Sako K, Barone V, Ritsch-Marte M, Sixt M, Voituriez R, et al. Cortical contractility triggers a stochastic switch to fast amoeboid cell motility. Cell 2015; 160:673-85; PMID:25679761; http://dx.doi.org/10.1016/j.cell.2015.01.008
- Menju T, Hashimoto S, Hashimoto A, Otsuka Y, Handa H, Ogawa E, Toda Y, Wada H, Date H, Sabe H. Engagement of overexpressed Her2 with GEP100 induces autonomous invasive activities and provides a biomarker for metastases of lung adenocarcinoma. PloS one 2011; 6:e25301; PMID:21966491; http://dx.doi.org/10.1371/journal.pone.0025301
- Sato H, Hatanaka KC, Hatanaka Y, Hatakeyama H, Hashimoto A, Matsuno Y, Fukuda S, Sabe H. High level expression of AMAP1 protein correlates with poor prognosis and survival after surgery of head and neck squamous cell carcinoma patients. Cell Commun Signal 2014; 12:17; PMID:24621372; http://dx.doi.org/10.1186/1478-811X-12-17
- Onodera Y, Hashimoto S, Hashimoto A, Morishige M, Mazaki Y, Yamada A, Ogawa E, Adachi M, Sakurai T, Manabe T, et al. Expression of AMAP1, an ArfGAP, provides novel targets to inhibit breast cancer invasive activities. EMBO J 2005; 24:963-73; PMID:15719014; http://dx.doi.org/10.1038/sj.emboj.7600588
- Morishige M, Hashimoto S, Ogawa E, Toda Y, Kotani H, Hirose M, Wei S, Hashimoto A, Yamada A, Yano H, et al. GEP100 links epidermal growth factor receptor signalling to Arf6 activation to induce breast cancer invasion. Nat Cell Biol 2008; 10:85-92; PMID:18084281; http://dx.doi.org/10.1038/ncb1672
- Sabe H, Hashimoto S, Morishige M, Ogawa E, Hashimoto A, Nam JM, Miura K, Yano H, Onodera Y. The EGFR-GEP100-Arf6-AMAP1 signaling pathway specific to breast cancer invasion and metastasis. Traffic 2009; 10:982-93; PMID:19416474; http://dx.doi.org/10.1111/j.1600-0854.2009.00917.x
- Hashimoto S, Onodera Y, Hashimoto A, Tanaka M, Hamaguchi M, Yamada A, Sabe H. Requirement for Arf6 in breast cancer invasive activities. Proc Natl Acad Sci USA 2004; 101:6647-52; PMID:15087504; http://dx.doi.org/10.1073/pnas.0401753101
- Birchmeier C, Birchmeier W, Gherardi E, Vande Woude GF. Met, metastasis, motility and more. Nat Rev Mol Cell Biol 2003; 4:915-25; PMID:14685170; http://dx.doi.org/10.1038/nrm1261
- Blume-Jensen P, Hunter T. Oncogenic kinase signalling. Nature 2001; 411:355-65; PMID:11357143; http://dx.doi.org/10.1038/35077225
- Hynes NE, Lane HA. ERBB receptors and cancer: the complexity of targeted inhibitors. Nat Rev Cancer 2005; 5:341-54; PMID:15864276; http://dx.doi.org/10.1038/nrc1609
- Onodera Y, Nam JM, Hashimoto A, Norman JC, Shirato H, Hashimoto S, Sabe H. Rab5c promotes AMAP1-PRKD2 complex formation to enhance beta1 integrin recycling in EGF-induced cancer invasion. J Cell Biol 2012; 197:983-96; PMID:22734003; http://dx.doi.org/10.1083/jcb.201201065
- Hashimoto S, Hirose M, Hashimoto A, Morishige M, Yamada A, Hosaka H, Akagi K-i, Ogawa E, Oneyama C, Agatsuma T, et al. Targeting AMAP1 and cortactin binding bearing an atypical src homology 3/proline interface for prevention of breast cancer invasion and metastasis. Proc Natl Acad Sci USA 2006; 103:7036-41; PMID:16636290; http://dx.doi.org/10.1073/pnas.0509166103
- Hirano M, Hashimoto S, Yonemura S, Sabe H, Aizawa S. EPB41L5 functions to post-transcriptionally regulate cadherin and integrin during epithelial-mesenchymal transition. J Cell Biol 2008; 182:1217-30; PMID:18794329; http://dx.doi.org/10.1083/jcb.200712086
- Hashimoto A, Oikawa T, Hashimoto S, Sugino H, Yoshikawa A, Otsuka Y, Handa H, Onodera Y, Nam J-M, Oneyama C, et al. P53- and mevalonate pathway–driven malignancies require Arf6 for metastasis and drug resistance. J Cell Biol 2016; 213(1):81-95; PMID:27044891
- Fischer KR, Durrans A, Lee S, Sheng J, Li F, Wong ST, Choi H, El Rayes T, Ryu S, Troeger J, et al. Epithelial-to-mesenchymal transition is not required for lung metastasis but contributes to chemoresistance. Nature 2015; 527:472-6; PMID:26560033; http://dx.doi.org/10.1038/nature15748
- Zheng X, Carstens JL, Kim J, Scheible M, Kaye J, Sugimoto H, Wu CC, LeBleu VS, Kalluri R. Epithelial-to-mesenchymal transition is dispensable for metastasis but induces chemoresistance in pancreatic cancer. Nature 2015; 527:525-30; PMID:26560028; http://dx.doi.org/10.1038/nature16064
- Rintoul RC, Sethi T. The role of extracellular matrix in small-cell lung cancer. Lancet Oncol 2001; 2:437-42; PMID:11905738; http://dx.doi.org/10.1016/S1470-2045(00)00421-6
- Sabeh F, Shimizu-Hirota R, Weiss SJ. Protease-dependent versus -independent cancer cell invasion programs: three-dimensional amoeboid movement revisited. J Cell Biol 2009; 185:11-9; PMID:19332889; http://dx.doi.org/10.1083/jcb.200807195
- Hashimoto A, Hashimoto S, Sugino H, Yoshikawa A, Onodera Y, Handa H, Oikawa T, Sabe H. ZEB1 induces EPB41L5 in the cancer mesenchymal program that drives ARF6-based invasion, metastasis and drug resistance. Oncogenesis 2016; 5:e259; PMID:27617643; http://dx.doi.org/10.1038/oncsis.2016.60
- Frisch SM, Francis H. Disruption of epithelial cell-matrix interactions induces apoptosis. J Cell Biol 1994; 124:619-26; PMID:8106557; http://dx.doi.org/10.1083/jcb.124.4.619
- Meredith JE, Jr., Fazeli B, Schwartz MA. The extracellular matrix as a cell survival factor. Mol Biol Cell 1993; 4:953-61; PMID:8257797; http://dx.doi.org/10.1091/mbc.4.9.953
- Coussens LM, Fingleton B, Matrisian LM. Matrix metalloproteinase inhibitors and cancer: trials and tribulations. Science 2002; 295:2387-92; PMID:11923519; http://dx.doi.org/10.1126/science.1067100
- Kessenbrock K, Plaks V, Werb Z. Matrix metalloproteinases: regulators of the tumor microenvironment. Cell 2010; 141:52-67; PMID:20371345; http://dx.doi.org/10.1016/j.cell.2010.03.015