ABSTRACT
ADP-ribosylation factors (Arfs) are members of the Ras GTPase superfamily. The function of Arfs is dependent on GTPase-activating proteins (GAPs) and guanine nucleotide exchange factors (GEFs), which allow Arfs to cycle between the GDP-bound and GTP-bound forms. Arf GAPs have been shown to be present in integrin adhesion complexes, which include focal adhesions. Integrin adhesion complexes are composed of integrins, scaffolding proteins and signaling proteins and regulate cell proliferation, survival, differentiation and migration. Understanding the role of Arf GAPs in the regulation of integrin adhesion complexes is relevant to understanding normal physiology and cancer. In this review, we will discuss the contribution of the Arf GAP family members to the regulation of integrin adhesion complexes, examining the diverse mechanisms by which they control integrin adhesion complex formation, maturation and dissolution. GIT1 and ARAP2 serve as GAPs for Arf6, regulating Rac1 and other effectors by mechanisms still being defined. In contrast, GIT2 regulates Rac1 independent of Arf6. AGAP2 binds to and regulates focal adhesion kinase (FAK). ARAP2 and ACAP1, both Arf6 GAPs, regulate membrane trafficking of integrins through different endocytic pathways, exerting opposite effects on focal adhesions. ASAP1 not only regulates actin cytoskeleton remodeling through its interaction with nonmuscle myosin 2A, but is also important in integrin recycling. These examples illustrate the diversity and versatility of Arf GAPs as regulators of integrin adhesion complex structure and function.
Introduction
Integrin adhesion complexes, including focal adhesions, and the associated actin cytoskeleton control cell proliferation, survival, differentiation and migration.Citation1-Citation3 The structures are essential for normal physiology including development, maintenance of tissue and organ structure and wound healing. Integrin adhesion complex-dependent functions also contribute to diseases such as cancer.Citation05,Citation2 Integrin adhesion complexes are composed of a variety of proteins that can be considered to function in 3 capacities: i) structural ii) signaling and iii) regulation of the integrin adhesion complexes. The 3 functions are integrated to control the assembly, maturation and dissolution of integrin adhesion complexes. Multiple members of one family of proteins, Arf GTPase-activating proteins (Arf GAPs) (), are found in integrin adhesion complexes and have been discovered to function in all 3 capacities and, in some cases, a single Arf GAP may have more than one role. Following a brief introduction to integrin adhesion complexes, we will discuss some of the molecular mechanisms by which Arf GAPs control integrin adhesion complex structure and function.
Figure 1. A. Schematic of Arf GAPs involved in regulation of integrin adhesion complexes. Domain structures of GIT1, GIT2, ASAP1, ASAP3, ACAP1, ARAP2 and AGAP2. A, Ankyrin repeats; ArfGAP, Arf GTPase activating domain; BAR, Bin/Amphiphysin/Rvs domain; CC, coiled-coiled motif; (D/ELPPKP)8, 8 tandem proline-rich (D/ELPPKP) motifs; GLD, GTP-binding protein-like domain; PBS, paxillin binding site; PH, pleckstrin homology domain; PPXPP, cluster of 3 proline-rich motifs; Rho GAP, Rho GTPase activating domain; S, serine rich; SAM, sterile α-motif; SH3, Src homology 3 domain; SHD, Spa homology domain.
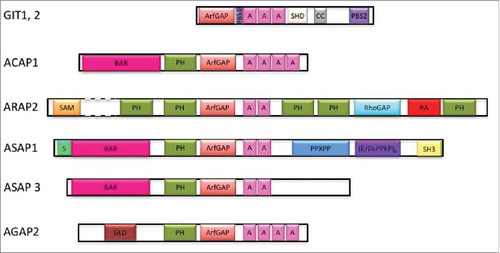
Integrin adhesion complexes are dynamic and under continuous control
Integrins are heterodimeric transmembrane receptors comprised of an α and β subunit. Humans have 18 α chains and 8 β chains, which combine to form 24 different integrins.Citation6-Citation8 Once activated in the plasma membrane, integrins cluster to initiate formation of integrin adhesion complexes. Activation of integrins involves binding of the integrin extracellular domain to the extracellular matrix (ECM) and binding of the integrin intracellular tail to focal adhesion proteins. Hundreds of focal adhesion proteins constitute the plaque component of integrin adhesion complexes that connect integrins to the actin cytoskeleton.Citation08,Citation1 Integrin adhesion complexes are dynamic and can rapidly transition among several forms. Nascent adhesions are formed immediately after integrin receptor binding to ECM at the leading edge of a migrating cell within the lamellipodium, which is a membrane protrusion containing a mesh of actin filaments.Citation1,Citation7-Citation10 Nascent adhesions are transient structures that either rapidly disassemble or, with nonmuscle myosin 2 activity, mature into focal complexes. Focal complexes can continue to mature and elongate to form focal adhesion s that localize at the ends of actomyosin stress fibers ().Citation9,Citation11
Figure 2. Roles of Arf GAPs in regulation of integrin adhesion complexes. The cartoon illustrates 3 mechanisms by which Arf GAPs can affect integrin adhesion complexes and the potential sites of action of the Arf GAPs in cells. First is by signaling through Rac1 and through phosphorylation (red arrows). Rac1•GTP blocks the transition of nascent adhesions and focal complexes to focal adhesions. Arf6•GTP facilitates Rac1 activation. GIT1 and ARAP2 reduce Rac1•GTP levels by reducing Arf6•GTP levels through GAP activity. GIT2 decreases Rac1•GTP levels by a Crk-dependent mechanism not yet defined (indicated by a question mark) that does not involve Arf6. Phosphorylation of FAK and FAK kinase activity also control integrin adhesion dynamics. Change in phosphorylation of FAK consequent to overexpression of AGAP2 has been found to reduce stability of integrin adhesions. The second mechanism by which Arf GAPs affect integrin adhesion complexes is by control of membrane trafficking of integrins (blue). This mechanism is used by at least 4 Arf GAPs: ACAP1, ARAP2, ASAP1 and ASAP3. ACAP1 functions on a tubulovesicular recycling compartment containing Arf6•GTP and Arf6•GTP is required for integrin recycling from this compartment. ARAP2 functions with Arf6 at a distinct endocytic compartment involved in the trafficking of integrins. ASAP1 and ASAP3 affect recycling of integrins but the specific compartment has not been defined. Furthermore, a role for GAP activity of ASAP1 and ASAP3 in integrin recycling has not been established nor whether ASAP3 is inhibitory or stimulatory. The third mechanism is by control of actomyosin, used by ASAP1, which directly binds to nonmuscle myosin 2A (NM2A, green).
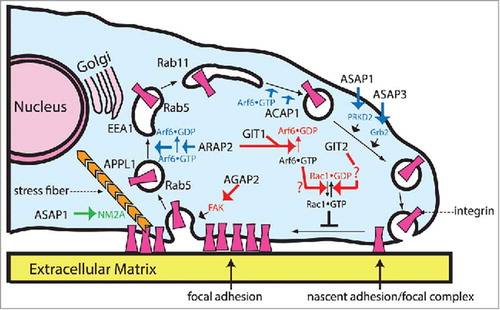
Formation of integrin adhesion complexes and transitioning between different forms of integrin adhesion complexes involves several signaling cascades. Tyrosine kinases, including focal adhesion kinase (FAK) and Src, phosphorylate several adhesion components such as p130CAS and paxillin, resulting in recruitment of additional proteins with consequent activation of several signaling pathways, including Ras, Rap1 and Rho family GTP-binding proteins.Citation8,Citation12-Citation16 Signaling changes with maturation of the adhesions and, at the same time, drives maturation and maintenance of the adhesions. For instance, tyrosine kinase activity of FAK increases when adhesions assemble but is also necessary for adhesion disassembly.Citation17-Citation21 Rho family proteins function sequentially in the maturation of nascent adhesions to focal adhesions. Rac1 is activated with assembly of nascent adhesions and drives the formation of focal complexes. Inactivation of Rac1 and activation of RhoA is required for formation of focal adhesions. Expression of a constitutively active form of Rac1 prevents the maturation of focal complexes to focal adhesions.Citation16,Citation9
Formation, maturation and maintenance of adhesions also require coordinated changes in the actin cytoskeleton and membrane traffic.Citation22,Citation10 Tension on adhesions is critical for the maturation of integrin adhesion complexes as it unmasks binding sites for multiple focal adhesion proteins, resulting in the growth of integrin adhesion complexes. This tension is largely generated by nonmuscle myosin2, which binds to actin filaments forming stress fibers.Citation23 When nascent adhesions engage with the actomyosin stress fibers in the lamella, a membrane region further back to the lamellipodia, they grow in size and change in composition to become focal adhesions (). Disassembly of integrin adhesion complexes involves endocytosis of integrins and degradation of focal adhesion proteins, by calpain and by targeting to autophagosomes.Citation22,Citation24-Citation27 However, the effects of membrane traffic and the endocytic paths taken by integrins on integrin adhesion complexes are complex as internalization of integrins can be an effective way of redistributing integrins to assemble new adhesions or to existing focal adhesions to maintain the structures.Citation10,Citation25,Citation27,Citation28 Recycling of integrins affects adhesions dependent on the particular endocytic path and on the specific integrin being recycled.Citation11,Citation22,Citation26,Citation27,Citation29 For instance, rapid recycling of α5β1 through a syndecan-dependent pathway results in smaller adhesions whereas αVβ3 results in larger adhesions.Citation30 Integrins are also reported to transit through several different endocytic paths including the fast and slow recycling, autophagic, and recycling lysosome paths.Citation22,Citation27-Citation29 Determinants targeting integrins to specific endocytic compartments continue to be discovered. ADP-ribosylation factor (Arf) family GTP-binding proteins are being investigated as part of the regulatory mechanism for membrane trafficking of integrins and actin remodeling.
ADP Ribosylation factors (Arfs)
Five genes encode Arfs in humans. The Arf proteins are categorized into 3 classes: Arf1 and 3 are class 1; Arf4 and 5 are class 2 and Arf6 is class 3.Citation31 Members of each class have been implicated in adhesion regulation. Arf1, Arf5 and Arf6 have been found to affect adhesion morphology by affecting membrane traffic and signaling that modulate actin cytoskeleton.Citation32 Arf5 and Arf6 regulate endocytosis of β1 integrin. Arf6 also regulates recycling of β1 integrin and affects integrin adhesion complexes by facilitating Rac1 activation.31,33-35 The function of Arfs, like other Ras superfamily members, depends on cycling between GDP and GTP bound forms. The conversion from Arf•GDP to Arf•GTP depends on exchange of GDP for GTP while the conversion from GTP to GDP depends on hydrolysis of GTP. Arf has very slow intrinsic exchange and no detectable intrinsic GTPase activity. Consequently, the cycling between the GTP and GDP bound Arfs is dependent on accessory proteins called guanine nucleotide exchange factors (GEFs) and GTPase-activating proteins (GAPs). Because Arfs affect integrin adhesion complexes, regulators of Arfs, Arf GEFs and GAPs, were anticipated to affect the structure and function of integrin adhesion complexes by controlling Arf•GTP levels. However, regulation of Arf•GTP levels only accounts for some of Arf GAPs effects on integrin adhesion complexes. Diverse molecular mechanisms are used by Arf GAPs to affect signaling, membrane traffic and actin cytoskeleton resulting in changes in integrin adhesion complexes.
The Arf GAP family
Arf GAPs are defined by the Arf GAP domain, comprised of a zinc binding motif, which mediates catalysis of GTP bound to Arf.Citation36 Arf GAP proteins are encoded by 31 genes and many have multiple splice variants. Based on structural and phylogenetic analyses, Arf GAPs have been classified into 10 groups. In 6 groups, the zinc-finger motif is at the N-terminus of the protein. Four groups contain a tandem of PH, Arf GAP and Ankyrin repeat domains. These latter 4 groups include 21 of the 31 Arf GAP genes. Arf GAPs often have complex domain structures. Most are named according to their domain composition.Citation29 For example, ASAP stands for: Arf GAP, SH3, Ank repeat and PH domains; ARAP: Arf GAP, Rho GAP, Ank repeat and PH domains; ACAP: Arf GAP, coiled-coil (BAR domain), Ank repeat and PH domain; and AGAP: Arf GAP, GTP-binding protein-like, Ank repeat and PH domain.
At least 7 Arf GAPs are found in integrin adhesion complexes (GIT1, GIT2, ASAP1, ASAP2, ASAP3, ARAP2 and AGAP2).Citation37-Citation45 Six of these as well as an Arf6 GAP ACAP146 have been examined as regulators of integrin adhesion complexes. These 7, whose domain structures are schematized in , are the subject of this review. Studies of these Arf GAPs indicate distinct molecular mechanisms contributing to integrin adhesion complex regulation. GIT1 and ARAP2 provide examples of the catalytic GAP activity having a role in regulating Arf6•GTP levels with consequences to signaling controlling integrin adhesion complexes. In addition, studies with GIT2 and ARAP2 illustrate that the inactivation of Arf is not sufficient to explain all of the effects of the Arf GAPs in integrin adhesion complexes.Citation46,Citation39 GIT1, GIT2, ARAP2 and AGAP2 form complexes with adaptor proteins, exchange factors for Rac1 and kinases to affect signaling that impinges on integrin adhesion complexes. ACAP1, ARAP2, ASAP1 and ASAP3 are examples of Arf GAPs that affect membrane traffic to control integrin adhesion complexes. ASAP1 is an example of an Arf GAP that has direct effects on the actin cytoskeleton, which associates with and affects integrin adhesion complexes ().
Arf GAPs that affect integrin adhesion complexes
Arf GAPs affect cell signaling related to integrin adhesion complex dynamics
As described above, Rho family protein signaling is crucial for control of integrin adhesion complex dynamics. Rac1•GTP maintains integrin adhesion complexes in a dynamic state and prevents maturation toward more stable adhesions. Arfs have been implicated in control of Rho family proteins. Arf6•GTP facilitates activation of Rac1.Citation47-Citation49 GITs and ARAP2 are Arf6 GAPs that affect signaling through Rac1 to control integrin adhesion complexes ().
Two genes encode GIT proteins in mammals, GIT1 and GIT2. The gene products include multiple splice variants of GIT2. The GIT proteins are composed of an N-terminal Arf GAP domain, followed by Ankyrin repeat, paxillin binding site 1 (PBS1), Spa homology, coiled coil and paxillin binding site 2 domains (PBS2)().Citation16,Citation37,Citation50,Citation51 Although early studies suggested that GIT1 destabilized integrin adhesion complexes, the results should be reevaluated with the recent report that the recombinant GIT1 used in the work was rendered catalytically inactive because of the N-terminal epitope tagCitation37; the epitope tagged proteins may have functioned as dominant negative proteins. This interpretation is consistent with later work in which GIT1 and GIT2 were found to stabilize adhesions. The effect of both GIT1 and GIT2 was mediated by reducing Rac1•GTP in cells () but differed in Arf6 dependency.
GIT1 was studied in CHO cells exogenously expressing α4 integrin. GIT1 binds paxillin and the complex inhibited Rac1 activity, which stabilized integrin adhesion complexes. Paxillin directly binds α4 integrin but not phosphorylated α4 integrin. Therefore, paxillin/GIT1 complex could be differentially recruited to adhesions depending on the phosphorylation state of α4 integrin. In migrating cells, the paxillin/GIT1 complex was excluded from the leading edge where phosphorylated α4 integrin was present. As a result, Rac1•GTP levels were high, leading to more dynamic adhesions in the leading edge whereas adhesions at the sides and the trailing edge were more stable due to the inhibition of Rac1 by the paxillin/GIT1 complex. The effect on adhesions depended on the Arf6 GAP activity of GIT1. The adhesions were less stable when wild type GIT1 was replaced with a GAP dead mutant. The results are consistent with the idea that converting Arf6•GTP to Arf6•GDP by GIT1 reduced Rac1•GTP levels,Citation38 which stabilized integrin adhesion complexes ().
Like GIT1 in CHO cells, GIT2 stabilized integrin adhesion complexes in HeLa cells by reducing Rac1•GTP levels but, interestingly did not appear to involve Arf6 ().Citation39 Also interesting, in HeLa cells GIT1 did not appear to stabilize integrin adhesion complexes. Knockdown of GIT2 increased adhesion turnover and the effect was reversed by expressing dominant negative Rac1 and dominant negative Cdc42, indicating that GIT2 functions by reducing Rac1•GTP and Cdc42•GTP levels. However, expression of dominant negative Arf6 did not reverse the effect of GIT2 knockdown, indicating that, in this case, the control of Rac1 and Cdc42 was not mediated by Arf GAP activity and Arf6. This result is in contrast to GIT1 in CHO cellsCitation38 and, as described below, ARAP2 in HeLa cells,Citation41 in which the Arf GAP activity is necessary for the effect to reduce Rac1•GTP and to stabilize adhesions. The idea that the GAP activity of GIT2 is dispensable for effects on the adhesions remains to be thoroughly tested. Based on these results, GITs may affect Rac1 by more than one pathway. The particular pathway may be dependent on cell type.
ARAP2 has also been found to stabilize integrin adhesion complexes through effects on Rac1.Citation40,Citation41 ARAP2, composed of a SAM, 5 PH, Arf GAP, Rho GAP and Ras association domains, localizes to focal adhesions (). The Rho GAP domain lacks catalytic activity but binds to Rho•GTP, which is necessary for ARAP2 function in cells to stabilize focal adhesions and actin stress fibers. Overexpression of ARAP2 increases the size of focal adhesions whereas knockdown of ARAP2 reduces the size and number of focal adhesions.Citation46,Citation40 The effects are mediated in part by regulation of Arf6 and Rac1 (). Knockdown of ARAP2 increased both Arf6•GTP and Rac1•GTP levels. The effect of ARAP2 knockdown could be reversed by expression of either dominant negative Arf6 or dominant negative Rac1 but not a GAP dead mutant, indicating control of Arf6 and Rac1 activity through the Arf GAP activity is required for ARAP2 function in integrin adhesion complexes. However, the effects on Arf6 and Rac1 were not sufficient for the effect of ARAP2. Dominant negative Rac1 by itself did not recapitulate the effect of ARAP2 overexpression, suggesting ARAP2 requires mechanisms in addition to reducing Rac1•GTP levels to regulate integrin adhesion complexes.Citation41,Citation46
Signaling events controlled by GITs and ARAP2 converge on modulating Rac1 activity but the mechanism is not yet defined. For GIT1 and ARAP2, the effect on Rac1 is mediated by changes in Arf6 activity. Arf6 may affect Rac1 activation by several mechanisms such by 1) affecting phosphoinositide metabolismCitation52,Citation53 which can regulate exchange factors for Rac1, 2) by releasing cytoplasmic Rac1 from Arfaptin,Citation54,Citation55 3) by recruiting a Rac1 exchange factor (GEF),Citation56 or 4) by affecting a Rac1GEF that associated with the Arf GAPs.Citation57-Citation59 In contrast, the effect of GIT2 on Rac1, Cdc42 and adhesion stability was independent of Arf6. The GIT-binding partner, β-PIX, is a Rac1GEF known to regulate adhesion dynamics and was considered as a potential mechanism by which GIT2 controlled Rac1•GTP levels. However, knockdown of β-PIX did not reverse the increased Rac1 and Cdc42•GTP levels by GIT2 knockdown. Crk knockdown, on the other hand, did reverse the effect of GIT2 knockdown. GIT2 can form a complex with Crk through paxillin.Citation60 Given that Crk binds DOCK180 members of Rac1GEFs,Citation61 GIT2 could potentially regulate DOCK180 to affect Rac1 activity. Identification of Rac1GEFs acting downstream from each of these Arf GAPs and the effect of Arf6 and Arf GAP activity on the Rac1GEFs will clarify the mechanism.
Studies on AGAP2 revealed another potential category of signaling regulation by Arf GAPs in integrin adhesion complexes, that is by modulating phosphorylation of focal adhesion proteins. Phosphorylation of focal adhesion proteins is central to the transitions among different forms of integrin adhesion complexes as it creates docking sites for different focal adhesion components. FAK can promote integrin adhesion complex maturation by phosphorylating paxillin on tyrosines 31 and 118. Phospho-paxillin recruits vinculin, a key event in focal adhesion maturation.Citation62 FAK can also promote destabilization of focal adhesions by at least 2 mechanisms. First is by increasing Rac1•GTP levels. FAK activity results in the phosphorylation of p130CAS. P130CAS recruits Crk and the associated Rac1GEF, DOCK180, leading to the activation of Rac1. The second mechanism is through promoting endocytosis of integrins. FAK enhances recruitment of dynamin to integrin adhesion complexes, necessary for endocytosis of integrins and consequent dissolution of integrin adhesion complexes.Citation17 AGAP2 has been shown to destabilize integrin adhesion complexes by affecting FAK activity. In HEK293 and U87 glioblastoma cells, AGAP2 was reported to bind FAK directly and increase FAK phosphorylation.Citation63 In PC12 cells, AGAP2 bound FAK indirectly through RACK1 and inhibited FAK phosphorylation.Citation64 Curiously, the opposite biochemical effects on FAK led to the same biologic effect of destabilizing the integrin adhesion complexes. Given that FAK phosphorylation could promote both integrin adhesion complex maturation and dissolution, one plausible explanation for the conflicting results is that in the case of FAK inhibition in PC12 cells, adhesions were not able to mature due to decreased recruitment of vinculin. In the case of FAK activation in HEK293 and U87 glioblastoma cells, adhesions were destabilized due to either high Rac1 activity or increased endocytosis of integrins.
Arf GAPs affect integrin adhesion complexes by control of membrane trafficking and protein translocation
Integrin adhesion complexes are dynamic which involves continuous exchange of protein components including soluble cytosolic proteins and transmembrane integrins. Arf GAPs have been found to regulate exchange of proteins in and out of integrin adhesion complexes.
ARAP2 and ACAP1 are 2 Arf6 GAPs with different domain structures that have distinct effects on trafficking of β1 integrin () and integrin adhesion complexes.Citation46 ARAP2 bound to Adaptor Protein containing a Pleckstrin homology domain, phosphotyrosine binding domain and Leucine zipper motif (APPL1) and localized to endosomes containing APPL1 and Arf6. ARAP2 knockdown increased the association of β1 integrin with APPL1 endosomes and decreased association with early endosome antigen 1 (EEA1) endosomes. The effect on integrin distribution required Arf GAP activity of ARAP2. ACAP1, on the other hand, associated with and regulated recycling tubular endosomes containing Arf6. The tubular endosomes are one path through which integrins transit. With the regulation of distinct endosomal compartments, ARAP2 and ACAP1 also had different effects on integrin trafficking. ARAP2 knockdown accelerated β1 integrin recycling whereas ACAP1 knockdown slowed integrin recycling.Citation46 Overexpression of ACAP1 also blocked β1 integrin recycling. The apparently conflicting results were explained by the finding that overexpression of ACAP1 interfered with clathrin coat assembly, thereby blocking its normal function in promoting β1 integrin recycling.Citation65 ACAP1 and ARAP2 overexpression also had opposing effects on integrin adhesion complexes, which were reduced in size and number with ACAP1 and increased in size with ARAP2.Citation46,Citation66 In summary, in regulation of integrin traffic, ACAP1 and ARAP2 may function with Arf6 to control specific endocytic trafficking routes of integrins resulting in opposing effects on integrin adhesion complexes.
ASAP1 and ASAP3 have been reported to regulate integrin recycling although the specific compartments in which they act remain to be determined ().Citation67,Citation68 ASAP1 and ASAP3 are both ASAP subtype Arf GAPs but have some important differences in structure (). Both contain BAR, PH, Arf GAP, Ankyrin repeat and proline rich domains. ASAP1 has a N-terminal poly serine extension from the BAR domain, a motif in the C-terminus comprised of E/DLPPKP repeated 8 times in tandem, and an SH3 domain, which are not present in ASAP3 ().Citation36,Citation69 ASAP1 is necessary for epidermal growth factor receptor (EGFR) dependent integrin recycling.Citation68 ASAP1 binds β1 integrin tail through its interaction with protein kinase D2 (PRKD2), a member in the polycystin/PKD1/PKCμ family. Their association is facilitated by Rab5C, which is activated by EGFR, and promotes recycling of integrin. Different than ASAP1, ASAP3/ β1 integrin interaction is mediated by growth factor receptor bound protein 2 (Grb2).Citation67 Grb2 is recruited to integrin upon EGFR activation. ASAP3 binding to Grb2 was also promoted by EGFR activation and the resulting phosphorylation of tyrosine 733 of ASAP3. Unexpectedly, both overexpression and knockdown of ASAP3 reduced β1 integrin recycling. Based on the precedent of ACAP1, we speculated that ASAP3 promotes β1 integrin recycling, with overexpression interfering with a coat assembly step that ASAP3 would normally promote. A role for Arf in the trafficking effects of ASAP1 and ASAP3 has not been investigated.
GIT1 was found to be one of the components that cycle in and out of integrin adhesion complexes, although mechanistic details and significance to integrin adhesion complex dynamics is not yet well defined. A complex of GIT1 with its binding partners, β-PIX and PAK, arose from the trailing edge of the cell where adhesions were being disassembled and translocated to the leading edge and adhesions. The nature of the GIT1 cytoplasmic structures is not certain. In one study, they were not membrane delimited, suggesting they are not membrane traffic intermediates.Citation70 In other reports, the structures were found to have the features of membrane traffic intermediates: delimited by membranes and associated with Rab11 and SNX27.Citation71-Citation74 Furthermore, neither a potential regulatory role of GITs on membrane traffic through these compartments nor a role of GITs in integrin traffic has been examined.
Arf GAPs may affect integrin adhesion complexes through control of actin-myosin dynamics
In addition to affecting integrin adhesion complexes by integrating cell signals and controlling membrane traffic, Arf GAPs may directly control the mechanical machinery of the actin cytoskeleton that associates with integrin adhesion complexes. Recently, The BAR-PH tandem of ASAP1 was discovered to bind to and regulate nonmuscle myosin 2A ().Citation75 Nonmuscle myosin 2A is an F-actin binding molecular motor that contributes to cellular events that involve force or translocation, including regulation of integrin adhesion complexes, cell spreading and cell migration.Citation23 ASAP1 and nonmuscle myosin 2A coimmunoprecipitated and colocalized in cells.Citation75 Reducing expression of either ASAP1 or nonmuscle myosin 2A had similar effects on actin-associated structures. Integrin adhesion complexes were reduced in size, consistent with a defect in adhesion maturation previously reported with nonmuscle myosin 2A knockdown. Knockdown of either ASAP1 or nonmuscle myosin 2A resulted in increased formation of circular dorsal ruffles (CDRs) in cells treated with growth factors. Overexpression of nonmuscle myosin 2A rescued the effect of ASAP1 knockdown on CDRs but ASAP1 did not rescue the effect of nonmuscle myosin 2A knockdown on CDRs, supporting the idea that nonmuscle myosin 2A functions downstream of ASAP1, mediating the effects of ASAP1 on actin remodeling that is involved, for instance, in the maturation of focal adhesions (). Other Arf GAPs with the BAR-PH tandem, including ASAP2, ASAP3, ACAP1, 2, 3 may also bind and regulate nonmuscle myosin 2 activity.
Conclusions
Of the 31 known Arf GAPs, at least 7 are found in integrin adhesion complexes. The integrin adhesion complex-associated Arf GAPs have diverse structures and, anticipated based on the structural differences, have functional differences. Arf GAPs can control integrin adhesion complexes in 3 ways: 1) by affecting signaling through Rac1 or through FAK (, red arrows), 2) by regulating membrane trafficking of integrins (blue arrows) and, most recently discovered, 3) by affecting actin-myosin dynamics (green arrows). The potential sites of action of the Arf GAPs discussed in this review are summarized in . Understanding how the assembly of various Arf GAP complexes is regulated in time and space will be key to understanding the control of integrin adhesion complex dynamics and functions. Only part of the Arf GAP function can be attributed to the catalytic Arf GAP activity and in most cases, the relationship of the GAP domain and its catalytic function to the effects on integrin adhesion complexes remain to be explored. One hypothesis currently being tested is that some of the Arf GAPs are self-limiting effectors of Arf; that is, by stimulating the GTP hydrolysis on Arf, they turn off the signal (Arf•GTP) that activates them, much like PLC-β functions as both an effector and a GAP for Gq.Citation76,Citation77 This hypothesis, the extent to which the mechanisms described for GITs, ARAP2, AGAP2 and ASAP1/3 apply to similar Arf GAPs and the molecular basis for the action of Arf GAPs with different domain structures are exciting directions for continued work.
Disclosure of potential conflicts of interest
No potential conflicts of interest were disclosed.
Acknowledgments
We thank Roberto Weigert for insightful discussions and Marielle E. Yohe for insightful discussions and critical review of the manuscript.
Funding
The work was supported by the intramural program of the National Institutes of Health, project BC 007365.
References
- Huttenlocher A, Horwitz AR. Integrins in Cell Migration. Cold Spring Harb Perspect Biol 2011; 3:a005074; PMID:21885598; http://dx.doi.org/10.1101/cshperspect.a005074
- Moreno-Layseca P, Streuli CH. Signalling pathways linking integrins with cell cycle progression. Matrix Biol 2014; 34:144-53; PMID:24184828; http://dx.doi.org/10.1016/j.matbio.2013.10.011
- Streuli CH. Integrins and cell-fate determination. J Cell Sci 2009; 122:171-7; PMID:19118209; http://dx.doi.org/10.1242/jcs.018945
- Guo WJ, Giancotti FG. Integrin signalling during tumour progression. Nat Rev Mol Cell Biol 2004; 5:816-26; PMID:15459662; http://dx.doi.org/10.1038/nrm1490
- Hehlgans S, Haase M, Cordes N. Signalling via integrins: Implications for cell survival and anticancer strategies. Biochim Biophys Acta 2007; 1775:163-80; http://dx.doi.org/10.1016/j.bbcan.2006.09.001
- Humphries JD, Paul NR, Humphries MJ, Morgan MR. Emerging properties of adhesion complexes: what are they and what do they do? Trends Cell Biol 2015; 25:388-97; PMID:25824971; http://dx.doi.org/10.1016/j.tcb.2015.02.008
- Hynes RO. Integrins: Bidirectional, allosteric signaling machines. Cell 2002; 110:673-87; PMID:12297042; http://dx.doi.org/10.1016/S0092-8674(02)00971-6
- Schoenwaelder SM, Burridge K. Bidirectional signaling between the cytoskeleton and integrins. Curr Opin Cell Biol 1999; 11:274-86; PMID:10209151; http://dx.doi.org/10.1016/S0955-0674(99)80037-4
- Choi CK, Vicente-Manzanares M, Zareno J, Whitmore LA, Mogilner A, Horwitz AR. Actin and alpha-actinin orchestrate the assembly and maturation of nascent adhesions in a myosin II motor-independent manner. Nat Cell Biol 2008; 10:1039-U36; PMID:19160484; http://dx.doi.org/10.1038/ncb1763
- Parsons JT, Horwitz AR, Schwartz MA. Cell adhesion: integrating cytoskeletal dynamics and cellular tension. Nat Rev Mol Cell Biol 2010; 11:633-43; PMID:20729930; http://dx.doi.org/10.1038/nrm2957
- Kuo JC. Mechanotransduction at focal adhesions: integrating cytoskeletal mechanics in migrating cells. J Cell Mol Med 2013; 17:704-12; PMID:23551528; http://dx.doi.org/10.1111/jcmm.12054
- Brown MC, Cary LA, Jamieson JS, Cooper JA, Turner CE. Src and FAK kinases cooperate to phosphorylate paxillin kinase linker, stimulate its focal adhesion localization, and regulate cell spreading and protrusiveness. Mol Biol Cell 2005; 16:4316-28; PMID:16000375; http://dx.doi.org/10.1091/mbc.E05-02-0131
- Webb DJ, Donais K, Whitmore LA, Thomas SM, Turner CE, Parsons JT, Horwitz AF. FAK-Src signalling through paxillin, ERK and MLCK regulates adhesion disassembly. Nat Cell Biol 2004; 6:154-67; PMID:14743221; http://dx.doi.org/10.1038/ncb1094
- Parsons SJ, Parsons JT. Src family kinases, key regulators of signal transduction. Oncogene 2004; 23:7906-9; PMID:15489908; http://dx.doi.org/10.1038/sj.onc.1208160
- Turner CE. Paxillin interactions. J Cell Sci 2000; 113:4139-40; PMID:11069756
- Turner CE, West KA, Brown MC. Paxillin-ARF GAP signaling and the cytoskeleton. Curr Opin Cell Biol 2001; 13:593-9; PMID:11544028; http://dx.doi.org/10.1016/S0955-0674(00)00256-8
- Ezratty EJ, Partridge MA, Gundersen GG. Microtubule-induced focal adhesion disassembly is mediated by dynamin and focal adhesion kinase. Nat Cell Biol 2005; 7:581-95; PMID:15895076; http://dx.doi.org/10.1038/ncb1262
- Nader GPF, Ezratty EJ, Gundersen GG. FAK, talin and PIPKI[gamma] regulate endocytosed integrin activation to polarize focal adhesion assembly. Nat Cell Biol 2016; 18:491-503; PMID:27043085; http://dx.doi.org/10.1038/ncb3333
- Wu X, Kodama A, Fuchs E. ACF7 regulates cytoskeletal-focal adhesion dynamics and migration and has ATPase activity. Cell 2008; 135:137-48; PMID:18854161; http://dx.doi.org/10.1016/j.cell.2008.07.045
- Yue J, Xie M, Gou X, Lee P, Schneider MD, Wu X. Microtubules regulate focal adhesion dynamics through MAP4K4. Dev Cell 2014; 31:572-85; PMID:25490267; http://dx.doi.org/10.1016/j.devcel.2014.10.025
- Hamadi A, Bouali M, Dontenwill M, Stoeckel H, Takeda K, Rondé P. Regulation of focal adhesion dynamics and disassembly by phosphorylation of FAK at tyrosine 397. J Cell Sci 2005; 118:4415-25; PMID:16159962; http://dx.doi.org/10.1242/jcs.02565
- Bridgewater RE, Norman JC, Caswell PT. Integrin trafficking at a glance. J Cell Sci 2012; 125:3695-701; PMID:23027580; http://dx.doi.org/10.1242/jcs.095810
- Vicente-Manzanares M, Ma XF, Adelstein RS, Horwitz AR. Non-muscle myosin II takes centre stage in cell adhesion and migration. Nat Rev Mol Cell Biol 2009; 10:778-90; PMID:19851336; http://dx.doi.org/10.1038/nrm2786
- Franco SJ, Rodgers MA, Perrin BJ, Han J, Bennin DA, Critchley DR, Huttenlocher A. Calpain-mediated proteolysis of talin regulates adhesion dynamics. Nat Cell Biol 2004; 6:977-83; PMID:15448700; http://dx.doi.org/10.1038/ncb1175
- Sharifi MN, Mowers EE, Drake LE, Collier C, Chen H, Zamora M, Mui S, Macleod KF. Autophagy Promotes Focal Adhesion Disassembly and Cell Motility of Metastatic Tumor Cells through the Direct Interaction of Paxillin with LC3. Cell Rep 2016; 15:1660-72; PMID:27184837; http://dx.doi.org/10.1016/j.celrep.2016.04.065
- Caswell PT, Vadrevu S, Norman JC. Integrins: masters and slaves of endocytic transport. Nat Revi Mol Cell Biol 2009; 10:843-53; http://dx.doi.org/10.1038/nrm2799
- Rainero E, Norman JC. Late endosomal and lysosomal trafficking during integrin-mediated cell migration and invasion. Bioessays 2013; 35:523-32; PMID:23605698; http://dx.doi.org/10.1002/bies.201200160
- Paul NR, Jacquemet G, Caswell PT. Endocytic Trafficking of Integrins in Cell Migration. Curr Biol 2015; 25:R1092-R105; PMID:26583903; http://dx.doi.org/10.1016/j.cub.2015.09.049
- Caswell PT, Norman JC. Integrin trafficking and the control of cell migration. Traffic 2006; 7:14-21; PMID:16445683; http://dx.doi.org/10.1111/j.1600-0854.2005.00362.x
- Morgan MR, Hamidi H, Bass MD, Warwood S, Ballestrem C, Humphries MJ. Syndecan-4 Phosphorylation Is a Control Point for Integrin Recycling. Dev Cell 2013; 24:472-85; PMID:23453597; http://dx.doi.org/10.1016/j.devcel.2013.01.027
- Kahn RA, Cherfils J, Elias M, Lovering RC, Munro S, Schurmann A. Nomenclature for the human Arf family of GTP-binding proteins: ARF, ARL, and SAR proteins. J Cell Biol 2006; 172:645-50; PMID:16505163; http://dx.doi.org/10.1083/jcb.200512057
- Casalou C, Faustino A, Barral DC. Arf proteins in cancer cell migration. Small GTPases 2016; 7:270-82; PMID:27589148; http://dx.doi.org/10.1080/21541248.2016.1228792
- Donaldson JG, Jackson CL. ARF family G proteins and their regulators: roles in membrane transport, development and disease. Nat Rev Mol Cell Biol 2011; 12:362-75; PMID:21587297; http://dx.doi.org/10.1038/nrm3117
- Gillingham AK, Munro S. The small G proteins of the Arf family and their regulators. Ann Rev Cell Dev Biol 2007; 23:579-611; http://dx.doi.org/10.1146/annurev.cellbio.23.090506.123209
- D'Souza-Schorey C, Chavrier P. ARF proteins: roles in membrane traffic and beyond. Nat Rev Mol Cell Biol 2006; 7:347-58; PMID:16633337; http://dx.doi.org/10.1038/nrm1910
- Kahn RA, Bruford E, Inoue H, Logsdon JM, Nie ZZ, Premont RT, Randazzo PA, Satake M, Theibert AB, Zapp ML, et al. Consensus nomenclature for the human ArfGAP domain-containing proteins. J Cell Biol 2008; 182:1039-44; PMID:18809720; http://dx.doi.org/10.1083/jcb.200806041
- Zhou W, Li XB, Premont RT. Expanding functions of GIT Arf GTPase-activating proteins, PIX Rho guanine nucleotide exchange factors and GIT-PIX complexes. J Cell Sci 2016; 129:1963-74; PMID:27182061; http://dx.doi.org/10.1242/jcs.179465
- Nishiya N, Kiosses WB, Han JW, Ginsberg MH. An alpha(4) integrin-paxillin-Arf-GAP complex restricts Rac activation to the leading edge of migrating cells. Nat Cell Biol 2005; 7:343-52; PMID:15793570; http://dx.doi.org/10.1038/ncb1234
- Frank SR, Adelstein MR, Hansen SH. GIT2 represses Crk- and Rac1-regulated cell spreading and Cdc42-mediated focal adhesion turnover. EMBO J 2006; 25:1848-59; PMID:16628223; http://dx.doi.org/10.1038/sj.emboj.7601092
- Yoon HY, Miura K, Cuthbert EJ, Davis KK, Ahvazi B, Casanova JE, Randazzo PA. ARAP2 effects on the actin cytoskeleton are dependent on Arf6-specific GTPase-activating-protein activity and binding to RhoA-GTP. J Cell Sci 2006; 119:4650-66; PMID:17077126; http://dx.doi.org/10.1242/jcs.03237
- Chen PW, Jian XY, Yoon HY, Randazzo PA. ARAP2 signals through Arf6 and Rac1 to control focal adhesion morphology. J Biol Chem 2013; 288:5849-60; PMID:23295182; http://dx.doi.org/10.1074/jbc.M112.415778
- Randazzo PA, Andrade J, Miura K, Brown MT, Long YQ, Stauffer S, Roller P, Cooper JA. The Arf GTPase-activating protein ASAP1 regulates the actin cytoskeleton. Proc Natl Acad Sci USA 2000; 97:4011-6; http://dx.doi.org/10.1073/pnas.070552297
- Ha VL, Bharti S, Inoue H, Vass WC, Campa F, Nie ZZ, de Gramont A, Ward Y, Randazzo PA. ASAP3 is a focal adhesion-associated Arf GAP that functions in cell migration and invasion. J Biol Chem 2008; 283:14915-26; PMID:18400762; http://dx.doi.org/10.1074/jbc.M709717200
- Winograd-Katz SE, Fassler R, Geiger B, Legate KR. The integrin adhesome: from genes and proteins to human disease. Nat Rev Mol Cell Biol 2014; 15:273-88; PMID:24651544; http://dx.doi.org/10.1038/nrm3769
- Horton ER, Byron A, Askari JA, Ng DH, Millon-Fremillon A, Robertson J, Koper EJ, Paul NR, Warwood S, Knight D, et al. Definition of a consensus integrin adhesome and its dynamics during adhesion complex assembly and disassembly. Nat Cell Biol 2015; 17:1577-87; PMID:26479319; http://dx.doi.org/10.1038/ncb3257
- Chen P-W, Luo R, Jian X, Randazzo PA. The Arf6 GTPase-activating proteins ARAP2 and ACAP1 define distinct endosomal compartments that regulate integrin α5β1 traffic. J Biol Chem 2014; 289:30237-48; PMID:25225293; http://dx.doi.org/10.1074/jbc.M114.596155
- Zhang Q, Calafat J, Janssen H, Greenberg S. ARF6 is required for growth factor- and rac-mediated membrane ruffling in macrophages at a stage distal to rac membrane targeting. Mol Cell Biol 1999; 19:8158-68; PMID:10567541; http://dx.doi.org/10.1128/MCB.19.12.8158
- Donaldson JG. Multiple roles for Arf6: Sorting, structuring, and signaling at the plasma membrane. J Biol Chem 2003; 278:41573-6; PMID:12912991; http://dx.doi.org/10.1074/jbc.R300026200
- Radhakrishna H, Al-Awar O, Khachikian Z, Donaldson JG. ARF6 requirement for Rac ruffling suggests a role for membrane trafficking in cortical actin rearrangements. J Cell Sci 1999; 112 (Pt 6):855-66; PMID:10036235
- Hoefen RJ, Berk BC. The multifunctional GIT family of proteins. J Cell Sci 2006; 119:1469-75; PMID:16598076; http://dx.doi.org/10.1242/jcs.02925
- Zhao ZS, Manser E, Loo TH, Lim L. Coupling of PAK-interacting exchange factor PIX to GIT1 promotes focal complex disassembly. Mol Cell Biol 2000; 20:6354-63; PMID:10938112; http://dx.doi.org/10.1128/MCB.20.17.6354-6363.2000
- Honda A, Nogami M, Yokozeki T, Yamazaki M, Nakamura H, Watanabe H, Kawamoto K, Nakayama K, Morris AJ, Frohman MA, et al. Phosphatidylinositol 4-phosphate 5-kinase alpha is a downstream effector of the small G protein ARF6 in membrane ruffle formation. Cell 1999; 99:521-32; PMID:10589680; http://dx.doi.org/10.1016/S0092-8674(00)81540-8
- Krauss M, Kinuta M, Wenk MR, De Camilli P, Takei K, Haucke V. ARF6 stimulates clathrin/AP-2 recruitment to synaptic membranes by activating phosphatidylinositol phosphate kinase type Igamma. J Cell Biol 2003; 162:113-24; PMID:12847086; http://dx.doi.org/10.1083/jcb.200301006
- Habermann B. The BAR-domain family of proteins: a case of bending and binding? The membrane bending and GTPase-binding functions of proteins from the BAR-domain family. EMBO Rep 2004; 5:250-5; PMID:14993925; http://dx.doi.org/10.1038/sj.embor.7400105
- Tarricone C, Xiao B, Justin N, Walker PA, Rittinger K, Gamblin SJ, Smerdon SJ. The structural basis of Arfaptin-mediated cross-talk between Rac and Arf signalling pathways. Nature 2001; 411:215-9; PMID:11346801; http://dx.doi.org/10.1038/35075620
- Koo TH, Eipper BA, Donaldson JG. Arf6 recruits the Rac GEF Kalirin to the plasma membrane facilitating Rac activation. BMC Cell Biol 2007; 8:29; PMID:17640372; http://dx.doi.org/10.1186/1471-2121-8-29
- Lu MJ, Kinchen JM, Rossman KL, Grimsley C, deBakker C, Brugnera E, Tosello-Trampont AC, Haney LB, Klingele D, Sondek J, et al. PH domain of ELMO functions in trans to regulate Rac activation via Dock180. Nat Struc Mol Biol 2004; 11:756-62; http://dx.doi.org/10.1038/nsmb800
- Koubek EJ, Santy LC. ARF1 and ARF6 regulate recycling of GRASP/Tamalin and the Rac1-GEF Dock180 during HGF-induced Rac1 activation. Small GTPases 2016:1-18; PMID:27562622; http://dx.doi.org/10.1080/21541248.2016.1219186
- Santy LC, Ravichandran KS, Casanova JE. The DOCK180/Elmo complex couples ARNO-mediated Arf6 activation to the downstream activation of Rac1. Curr Biol 2005; 15:1749-54; http://dx.doi.org/10.1016/j.cub.2005.08.052
- Lamorte L, Rodrigues S, Sangwan V, Turner CE, Park M. Crk associates with a multimolecular Paxillin/GIT2/beta-PIX complex and promotes Rac-dependent relocalization of Paxillin to focal contacts. Mol Biol Cell 2003; 14:2818-31; PMID:12857867; http://dx.doi.org/10.1091/mbc.E02-08-0497
- Birge RB, Kalodimos C, Inagaki F, Tanaka S. Crk and CrkL adaptor proteins: networks for physiological and pathological signaling. Cell Communi Signal 2009; 7:13; http://dx.doi.org/10.1186/1478-811X-7-13
- Pasapera AM, Schneider IC, Rericha E, Schlaepfer DD, Waterman CM. Myosin II activity regulates vinculin recruitment to focal adhesions through FAK-mediated paxillin phosphorylation. J Cell Biol 2010; 188:877-90; PMID:20308429; http://dx.doi.org/10.1083/jcb.200906012
- Zhu YJ, Wu YJ, Kim JI, Wang ZM, Daaka Y, Nie ZZ. Arf GTPase-activating protein AGAP2 regulates focal adhesion kinase activity and focal adhesion remodeling. J Biol Chem 2009; 284:13489-96; PMID:19318351; http://dx.doi.org/10.1074/jbc.M900469200
- Dwane S, Durack E, O'Connor R, Kiely PA. RACK1 promotes neurite outgrowth by scaffolding AGAP2 to FAK. Cell Signal 2014; 26:9-18; PMID:24056044; http://dx.doi.org/10.1016/j.cellsig.2013.08.036
- Li J, Peters PJ, Bai M, Dai J, Bos E, Kirchhausen T, Kandror KV, Hsu VW. An ACAP1-containing clathrin coat complex for endocytic recycling. J Cell Biol 2007; 178:453-64; PMID:17664335; http://dx.doi.org/10.1083/jcb.200608033
- Li J, Ballif BA, Powelka AM, Dai J, Gygi SP, Hsu VW. Phosphorylation of ACAP1 by Akt regulates the stimulation-dependent recycling of integrin beta 1 to control cell migration. Dev Cell 2005; 9:663-73; PMID:16256741; http://dx.doi.org/10.1016/j.devcel.2005.09.012
- Yu X, Wang FS, Liu HS, Adams G, Aikhionbare F, Liu D, Cao XW, Fan LB, Hu GH, Chen Y, et al. ACAP4 protein cooperates with Grb2 protein to orchestrate epidermal growth factor-stimulated integrin beta 1 recycling in cell migration. J Bioll Chem 2011; 286:43735-47; http://dx.doi.org/10.1074/jbc.M111.278770
- Onodera Y, Nam JM, Hashimoto A, Norman JC, Shirato H, Hashimoto S, Sabe H. Rab5c promotes AMAP1-PRKD2 complex formation to enhance beta 1 integrin recycling in EGF-induced cancer invasion. J Cell Biol 2012; 197:983-96; PMID:22734003; http://dx.doi.org/10.1083/jcb.201201065
- Randazzo PA, Inoue H, Bharti S. Arf GAPs as regulators of the actin cytoskeleton. Biol Cell 2007; 99:583-600; PMID:17868031; http://dx.doi.org/10.1042/BC20070034
- Manabe R, Kovalenko M, Webb DJ, Horwitz AR. GIT1 functions in a motile, multi-molecular signaling complex that regulates protrusive activity and cell migration. J Cell Sci 2002; 115:1497-510; PMID:11896197
- Matafora V, Paris S, Dariozzi S, de Curtis I. Molecular mechanisms regulating the subcellular localization of p95-APP1 between the endosomal recycling compartment and sites of actin organization at the cell surface. J Cell Sci 2001; 114:4509-20; PMID:11792816
- Paris S, Za L, Sporchia B, de Curtis I. Analysis of the subcellular distribution of avian p95-APP2, an ARF-GAP orthologous to mammalian paxillin kinase linker. Int J Biochemi Cell Biol 2002; 34:826-37; http://dx.doi.org/10.1016/S1357-2725(02)00008-0
- Za L, Albertinazzi C, Paris S, Gagliani M, Tacchetti C, de Curtis I. beta PIX controls cell motility and neurite extension by regulating the distribution of GIT1. J Cell Sci 2006; 119:2654-66; PMID:16787945; http://dx.doi.org/10.1242/jcs.02996
- Valdes JL, Tang JR, McDermott MI, Kuo JC, Zimmerman SP, Wincovitch SM, Waterman CM, Milgram SL, Playford MP. Sorting nexin 27 protein regulates trafficking of a p21-activated Kinase (PAK) Interacting exchange factor (beta-Pix)-G protein-coupled receptor kinase interacting protein (GIT) complex via a PDZ domain interaction. J Biol Chem 2011; 286:39403-16; PMID:21926430; http://dx.doi.org/10.1074/jbc.M111.260802
- Chen PW, Jian X, Heissler SM, Le K, Luo R, Jenkins LM, Nagy A, Moss J, Sellers JR, Randazzo PA. The Arf GTPase-activating protein, ASAP1, binds nonmuscle myosin 2A to control remodeling of the actomyosin network. J BioL Chem 2016; 291:7517-26; PMID:26893376; http://dx.doi.org/10.1074/jbc.M115.701292
- Cook B, Bar-Yaacov M, Ben-Ami HC, Goldstein RE, Paroush Z, Selinger Z, Minke B. Phospholipase C and termination of G-protein-mediated signalling in vivo. Nat Cell Biol 2000; 2:296-301; PMID:10806481; http://dx.doi.org/10.1038/35010571
- Montell C. PLC fills a GAP in G-protein-coupled signalling. Nat Cell Biol 2000; 2:E82-E3; PMID:10806493; http://dx.doi.org/10.1038/35010515