ABSTRACT
We recently identified a key role for SWAP70 as the tethering factor stabilizing F-actin filaments on the surface of phagosomes in human dendritic cells by interacting both with Rho-family GTPases and the lipid phosphatidylinositol (3,4)-bisphosphate. In this study, we aimed to investigate whether this role of SWAP70 was general among immune phagocytes. Our data reveal that SWAP70 is recruited to early phagosomes of macrophages and dendritic cells from both human and mouse. The putative inhibitor of SWAP70 sanguinarine blocked phagocytosis and F-actin polymerization, supporting a key role for SWAP70 in phagocytosis as demonstrated previously with knock-down. Moreover, SWAP70 was recently shown to sequester the F-actin severing protein cofilin and we investigated this relationship in phagocytosis. Our data show an increased activation of cellular cofilin upon siRNA knockdown of SWAP70. Finally, we explored whether SWAP70 would be recruited to the immune synapse between dendritic cells and T cells required for antigen presentation, as the formation of such synapses depends on F-actin. However, we observed that SWAP70 was depleted at immune synapses and specifically was recruited to phagosomes. Our data support an essential and specific role for SWAP70 in tethering and stabilizing F-actin to the phagosomal surface in a wide range of phagocytes.
Introduction
Actin has a well-understood role in the phagocytosis of microbes and tumor cells by immune cells.Citation24,Citation40,Citation50,Citation55 Although the precise course of F-actin formation depends on the phagocytic receptors engaged,Citation37,Citation4 F-actin filaments are generally assembled early during phagocytosis at the site of phagosome formation and F-actin depolymerizes within several minutes after sealing of the phagosome.Citation38,Citation6 We recently showed an essential role for the adaptor protein SWAP70 in phagocytosis by human dendritic cells derived from blood-isolated monocytes.Citation6 In our study, we demonstrated that SWAP70 is transiently recruited to phagosomes ingested by different phagocytic receptors.Citation6 This phagosomal recruitment of SWAP70 coincided with the presence of F-actin on the phagosomal surface, and occurred before recruitment of the early endosomal small GTPase Rab5.Citation6,Citation42 SWAP70 is well known to bind to and stabilize F-actin with its C-terminal region.Citation14,Citation30,Citation34,Citation35,Citation41,Citation46,Citation51 Using 3-dimensional multicolor super-resolution STED microscopy, we showed that SWAP70 organizes F-actin as parallel fibers or concentric rings on the surface of phagosomes.Citation6 In our previous study,Citation6 we also showed that the specific recruitment of SWAP70 to phagosomes is driven by its binding to the small Rho-family GTPase Rac1Citation35,Citation42,Citation45,Citation54 and to phosphatidylinositol (3,4)-bisphosphate (PI(3,4)P2).Citation34,Citation51 Our data indicated that SWAP70 binds to and stabilizes activated Rac1 at the phagosomal surface,Citation6 although it is most likely not a guanine exchange factor (GEF) itself.Citation42 We also showed that SWAP70 was not or less recruited to other F-actin rich cellular regions,Citation6 such as podosomes which are involved in cell migration and trans-epithelial antigen sampling.Citation7,Citation8
In this study, we further explored the role of SWAP70 in phagocytosis. First, we aimed to determine whether the role for SWAP70 in phagocytosis was only present in human dendritic cells or was also observable in other immune phagocytes and other species. Our data show specific recruitment of SWAP70 to phagosomes in murine primary immune cells as well as the murine macrophage cell line RAW264.7. Second, we explored how phagocytosis and F-actin polymerization would be affected by the putative SWAP70 inhibitor sanguinarine.Citation15,Citation26 Corroborating our previous findings that siRNA knockdown of SWAP70 results in phagocytic defects,Citation6 we found that sanguinarine blocked phagocytosis in human dendritic cells. Third, we investigated the link between SWAP70 and cofilin in phagocytosis. Cofilin is an F-actin severing factorCitation18 that is sequestered by SWAP70 and this in turn interferes with its F-actin depolymerizing activity.Citation14 In line with this, we observed increased activation of cellular cofilin upon knockdown of SWAP70 by siRNA. Finally, we addressed the possibility whether SWAP70 would be recruited to the immune synapses of dendritic cells with T cells required for antigen presentation. Similar to phagocytosis, the formation of these immunological synapses relies on cellular rearrangements of the F-actin cytoskeleton, local turnover of phosphoinositides and activation of Rho-GTPases,Citation2,Citation9,Citation10,Citation22,Citation53,Citation58 and all these factors could potentially recruit SWAP70 to the synapse. However, we observed no enrichment but rather a depletion of SWAP70 at the immune synapse between dendritic cells with T cells, both with a murine antigen specific immune synapse model and with the human allogenic mixed leukocyte reaction. Overall, these data support a broad role for SWAP70 as a specific tether and stabilizing factor of the phagosomal F-actin cage.
Results
SWAP70 is recruited to early phagosomes in murine dendritic cells and the macrophage cell line RAW264.7
In our recent study,Citation6 we demonstrated that SWAP70 is essential for phagocytosis by human dendritic cells derived from blood-isolated monocytes (monocyte-derived dendritic cells; moDCs). To determine how general this mechanism is among other phagocytes of the immune system and other species, we first tested for the expression of SWAP70 in mouse dendritic cells and human monocyte-derived macrophages. We also tested for the expression of SWAP70 in the murine macrophage cell line RAW264.7, which is a widely-studied model system for phagocytosis. Mouse dendritic cells were derived from bone marrow stem cells (bone marrow-derived dendritic cells; BMDC) by culturing with granulocyte-macrophage colony-stimulating factor (GM-CSF)Citation39,Citation42 and human macrophages were derived from blood monocytes by culturing with macrophage colony-stimulating factor (M-CSF). Human and mouse SWAP70 amino acid sequences are 95% identical and could both be recognized by our polyclonal antibody by Western blot (). SWAP70 was expressed both in mouse BMDCs and RAW264.7 macrophages, as has been reported previously.Citation42-Citation45,Citation20,Citation29 SWAP70 was expressed in mouse BMDCs at slightly lower levels than in moDCs, whereas the expression of SWAP70 was higher both in human macrophages and the mouse RAW264.7 macrophage cell line (). Expression levels of SWAP70 did not notably change upon exposure of the cells to the fungal adjuvant zymosan (prepared from Saccharomyces cerevisiae). Additionally, we tested SWAP70 expression in the naturally occurring myeloid (mDC; CD1c-positive) and plasmacytoid (pDC; BDCA4-positive) dendritic cell subsets isolated from human blood (). Whereas SWAP70 was expressed by mDCs, we observed almost no expression of SWAP70 in pDCs (). This low expression of SWAP70 in pDCs could relate to their low phagocytic ability.Citation36,Citation49 Stimulation of the mDCs with the bacterial adjuvant lipopolysaccharide (LPS) did not result in a significantly altered expression of SWAP70.
Figure 1. Phagosomal role of SWAP70 is conserved in human and mouse immune cells. (A) SWAP70 expression in human moDCs, human macrophages (MΦ), mouse GM-CSF-differentiated BMDCs, RAW264.7 macrophages and primary human blood-isolated mDCs and pDCs by Western blot. GAPDH: loading control. (B) Quantification of SWAP70 band intensities normalized to GAPDH (statistical significance only observed for unstimulated mDC and pDC; P = 0.0493, 2-sided paired t test). (C) Representative confocal micrographs of zymosan-FITC (green in merge) pulsed GM-CSF-differentiated BMDCs, FLT3L-differentiated BMDCs and RAW264.7 macrophages with immunolabeling for SWAP70 (magenta). Nascent phagocytic cups of zymosan-FITC were labeled with an anti-FITC antibody (cyan) in absence of permeabilization (i.e., only uninternalized portions of zymosan-FITC are labeled). BF: bright-field. The right-hand schemes depict the positions of the cells and phagosomes. Yellow arrowheads: positions of the phagocytic cups. Scale bars: 5 µm. (D) Left-hand graph: the percentages of SWAP70-positive phagocytic cups compared with the total number of all internalized zymosan particles from panel C. Right-hand graph: percentages of SWAP70-positive phagocytic cups to all SWAP70-positive phagosomes (N = 3; >200 cells/time point). (E) Number of fully internalized zymosan particles over time of uptake for the cells from panel C. Statistical significance was assessed for triplicates of the experiment using 1-way ANOVA with post hoc Tukey.
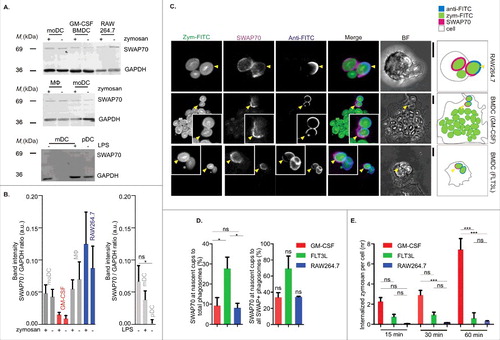
In the next step, we examined whether SWAP70 would be present on phagosomes of mouse BMDCs and RAW264.7 macrophages. In these experiments, we tested BMDCs differentiated both with GM-CSF or with FMS-like tyrosine kinase-3 ligand (FLT3L). Culturing in the presence of FLT3L results mainly in mixed populations of pDCs and conventional dendritic cells (cDCs), compared with the more heterogeneous mixture of macrophages and dendritic cells present in GM-CSF-differentiated BMDCs.Citation39,Citation32 Similar to our previous study,Citation6 we used zymosan particles as phagocytic cargo and selectively searched for early phagosomes containing semi-internalized particles (i.e. nascent phagocytic cups). We loaded the cells with zymosan-FITC and immunolabeled with an anti-FITC antibody in absence of permeabilization, resulting in exclusive labeling of partially internalized and uninternalized zymosan-FITC particles (). In all cell types, endogenous SWAP70 was recruited in a polarized fashion to phagosomes and very often was found at nascent phagocytic cups. In GM-CSF differentiated dendritic cells and RAW264.7 macrophages, on average about ∼10% of all phagosomes was positive for SWAP70 and about ∼35% of all SWAP70-positive phagosomes were phagocytic cups (), which resembles our previous observations with human moDCs.Citation6 Compared to GM-CSF-differentiated dendritic cells and RAW264.7 macrophages, SWAP70 was present at significantly more nascent cups for FLT3L-differentiated dendritic cells, and this probably relates to a lower phagocytic capability and/or altered kinetics of uptake. In the same set of experiments, we compared the phagocytic ability of BMDCs and RAW264.7 macrophages by counting the fully internalized zymosan particles in those cells. Overall, FLT3L-differentiated BMDCs and RAW264.7 were phagocytosing much less zymosan per cell compared with GM-CSF-differentiated BMDCs (). RAW264.7 is an Abelson murine leukemia virus-induced tumor cell line cultured for over 4 decadesCitation47 and perhaps has lower phagocytic capability than primary phagocytes. FLT3L-differentiated BMDCs are substantially smaller (∼30 µm diameter) compared with GM-CSF-differentiated BMDCs (∼70 µm diameter) and perhaps their small cell size limits uptake of zymosan particles (average zymosan particle is ∼3–5 µm in diameter).
In moDCs, we previously showed by live cell imaging transient recruitment of SWAP70 to early phagosomes concomitantly with F-actin.Citation6 We made similar observations for RAW264.7 macrophages transiently co-expressing SWAP70 fused to GFP (SWAP70-GFP) together with the F-actin binding probe LifeAct fused to RFP (LifeAct-RFP), and observed simultaneous recruitment of both proteins to phagosomes engulfing zymosan (, Movie S1). We also performed immunolabeling experiments for Rac1 to determine whether Rac1 was present at SWAP70 and F-actin-rich phagosomes in RAW264.7 macrophages, GM-CSF-differentiated BMDCs and FLT3L-differentiated BMDCs (). In all 3 cell types, we frequently observed the co-localization of Rac1 to SWAP70 and F-actin-positive phagosomes (), very similar to our previous observations with moDCs.Citation6 These data show that SWAP70 is not only recruited to phagosomes in human dendritic cells, but also to phagosomes in murine dendritic cells and macrophages.
Figure 2. SWAP70 in murine cells localizes on the phagosomal surface with F-actin and Rac1. (A) Live cell epi-fluorescence imaging of RAW264.7 macrophages expressing SWAP70-GFP (green in merge) and LifeAct-mRFP (magenta). The inset shows a time series during zymosan uptake (yellow square). See also Movie S1. BF: bright-field. Scale bar: 10 µm. (B) Confocal micrographs of zymosan-pulsed GM-CSF-differentiated BMDCs, FLT3L-differentiated BMDCs and RAW264.7 macrophages with immunolabeling for SWAP70 (red in merge) and Rac1 (green). F-actin was labeled with phalloidin (magenta). Yellow arrowheads: phagosomes positive for SWAP70, Rac1 and F-actin. Scale bars: 5 µm. (C) Venn diagrams and bar graphs from panel B showing phagosomal distributions of Rac1, F-actin and SWAP70 (N = 3; >100 cells per cell type). Statistical significance was assessed for triplicates of the experiment using 1-way ANOVA with post hoc Tukey.
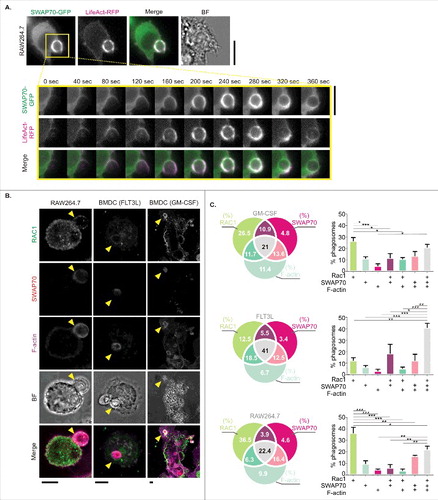
The putative inhibitor of SWAP70 sanguinarine blocks F-actin polymerization and phagocytosis.
In our recent study, we demonstrated that siRNA knockdown of SWAP70 in human moDCs results in a defect in F-actin polymerization and phagocytosis in human moDCs.Citation6 In the current study, we aimed to extent these findings and tested whether pharmacological inhibition of SWAP70 with the putative SWAP70 inhibitor sanguinarineCitation15,Citation26 would result in a similar functional outcome and block phagocytosis and F-actin polymerization. Sanguinarine is a quaternary ammonium compound produced by a variety of plants and an inhibitor of the Na+/K+-ATPase. Although not directly proven, sanguinarine is proposed to inhibit SWAP70 action based on the findings that it can reverse the oncogenic transforming phenotypes of mouse embryo fibroblasts (MEFs) and the COS-7 fibroblast cell line expressing wild-type or an oncogenic mutant of SWAP70.Citation15,Citation26
We tested the effect of sanguinarine on cell viability, phagocytosis and F-actin polymerization. moDCs were precultured for 1 hr with sanguinarine before the 1 hr incubation with zymosan-FITC and phagocytic kinetic was measured by flow cytometry and confocal microscopy. Under these experimental conditions, sanguinarine did not affect viability as measured by the MTT assay (3-(4,5-dimethylthiazol-2-yl)-2,5-diphenyltetrazolium bromide) and co-staining with 7-aminoactinomycin D (7AAD; ). MTT is a substrate for mitochondrial reductases and allows to measure metabolic activity. 7AAD is a DNA intercalating dye that labels necrotic cells. However, we observed a significant increase in staining with the phosphatidylserine-binding probe Annexin-V at the highest concentration of sanguinarine tested (24 µM), suggesting that some moDCs entered the early apoptotic state (). We then tested the effect of sanguinarine on phagocytosis by flow cytometry () and confocal microscopy (). For all donors tested, the fraction of moDCs that took up zymosan-FITC was significantly reduced by sanguinarine and this zymosan-positive fraction took up considerably less particles than without sanguinarine (). Similar phagocytic defects caused by sanguinarine were observed for neutrophils.Citation1 Moreover, immunofluorescence microscopy revealed a reduced recruitment of SWAP70 to phagosomes upon sanguinarine treatment (), in line with a role for SWAP70 in phagocytosis. We also investigated the effect of sanguinarine on the cellular content of F-actin filaments by staining the cells with the F-actin binding probe phalloidin conjugated to Alexa fluor 647. Using both flow cytometry and confocal microscopy, the phalloidin intensity was reduced by sanguinarine in a dose dependent manner (). Thus, sanguinarine blocked phagocytosis and caused a defect in F-actin polymerization. These results mirror our previous observations with siRNA knockdown of SWAP70 in moDCs.Citation6
Figure 3. Putative SWAP70 inhibitor sanguinarine blocks phagocytosis and impairs F-actin polymerization in human moDC. (A) Viability tests of human moDCs by the MTT assay (Abs: absorbance units at 592 nm) and by flow cytometry with AnnexinV and 7AAD staining for the sanguinarine concentrations indicated (0-concentration: methanol control; MeOH). (B–D) MoDCs were pretreated with sanguinarine at the concentrations indicated and stimulated with zymosan-FITC for 1 hour. Shown are the flow cytometry histograms (panel B), the percentages of zymosan-positive cells (panel C; right from the gray dashed lines in panel B), and the mean fluorescence index (MFI) of zymosan-FITC (panel D). (E–G) Similar to panels B–D, but now phagocytic capability was determined by confocal microscopy. (E) representative confocal micrographs of moDCs with internalized zymosan-FITC (green in merge), immunolabeling for SWAP70 (red) and F-actin staining (magenta). BF: bright-field. Scale bar, 10 µm. F: Phagocytic index calculated as the number of internalized zymosan per cell normalized to the condition without sanguinarine (N = 3 donors, >90 cells/condition/donor). (G) Quantification of SWAP70-positive phagosomes per cell. H–I. Same as panels B and D, but now for the phalloidin Alexa Fluor 647 signal (phalloidin-647; F-actin) instead of zymosan-FITC. J. Same as panel G, but now for phalloidin-647-positive phagosomes. Statistical significance was assessed using one-way ANOVA with post hoc Tukey.
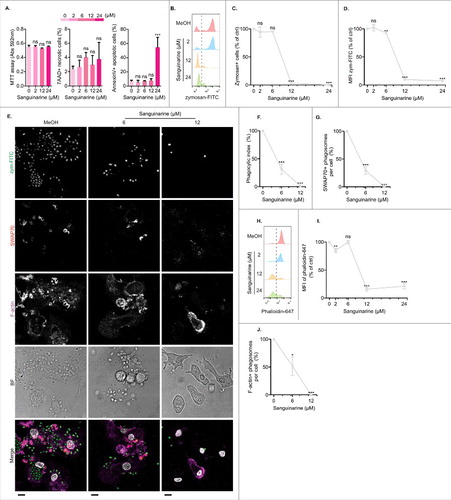
SWAP70 inhibits cofilin activity in dendritic cells
In the next set of experiments, we explored the link between SWAP70 and cofilin in phagocytosis. Recently, SWAP70 was described to directly regulate cofilin activity in vitro.Citation14 The role of cofilin as a severing factor of filamentous actin is well-established,Citation18 although its role in phagocytosis is still controversial.Citation24 Cofilin is tightly spatiotemporally regulated by direct binding to regulatory proteins, phosphorylation and subcellular localization.Citation57,Citation23 Cofilin is also negatively regulated by binding to phosphatidylinositol (4,5)-bisphosphate.Citation11 SWAP70 is reported to directly sequester cofilin and thereby interferes with its F-actin depolymerizing activity.Citation14 In line with this finding, we observed that siRNA knockdown of SWAP70 in moDCs (75% knockdown efficiency by Western blot; ) resulted in a small (∼15%) but significant decrease in the cellular content of inactive cofilin (Serine 3 phosphorylationCitation60,Citation5) (). However, the detection of phosphorylated cofilin by Western blot is a global method that potentially underestimates local effects at the phagosomal surface. Indeed, immunolabeling showed the enrichment of the inactive form of cofilin at actin-rich phagosomes (), suggesting that cofilin was locally sequestered at phagosomes. We were unable to perform co-labeling experiments of inactive cofilin together with SWAP70 due to the incompatibilities of the antibodies (both same host species and isotype). Thus, SWAP70 depletion not only leads to a reduced activation of Rac1 at the phagosomal surface as we have shown previously,Citation6 but also to increased activation of cofilin. Both these effects likely contribute to the formation and stabilization of F-actin at the phagosomal surface by SWAP70.
Figure 4. SWAP70 inhibits the activity of cofilin. (A) Knock-down of SWAP70 in human moDCs (gray; siSWAP70) or non-targeting siRNA (black; siControl) by Western blot (mean ± s.e.m; paired 2-sided t test). GAPDH: loading control. (B) Ser3-phosphorylated (inactive) cofilin (P-cofilin) compared with GAPDH by Western blot upon SWAP70 knockdown. (C) Quantification of panel B for moDCs from 4 and 3 different donors (individual donors shown; paired 2-sided t tests). (D) Representative confocal micrographs of zymosan-pulsed dendritic cells immunostained for P-cofilin (magenta in merge) and F-actin (green). Yellow arrowheads indicate double-positive phagosomes. Scale bar: 5 µm
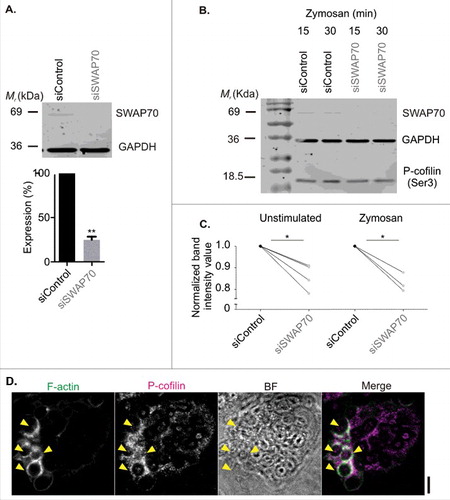
SWAP70 is not enriched at the immunological synapse between dendritic cells and T cells
The phagosome is not the only F-actin-rich structure in dendritic cells and macrophages. We recently showed that although SWAP70 is present at actin-dense mechanosensitive structures called podosomes,Citation31 it is much more enriched at phagosomes.Citation6 This is in line with the findings that phagocytosis depends on SWAP70,Citation6 whereas podosome formation and turnover do not depend on SWAP70.Citation31 Another F-actin-rich region is the immunological synapse formed between dendritic cells and T cells. The immunological synapse is a highly-organized tight cellular interface that provides a platform for the presentation of antigen in major histocompatibility class I and II complexes (MHC class I and II) on the surface of the dendritic cell/macrophage to receptors on the surface of the T cell.Citation58 In T cells, the actin cytoskeleton is well-understood to play an important role in synapse formation, and retrograde transport over F-actin filaments mediates the centripetal motion of T cell receptors and adhesion molecules to the synapse.Citation48 F-actin also plays a key role at the dendritic cell-side of the immunological synapse, because it provides a mechanical counter force that keeps the immunological synapse in a more disorganized state with less clustering of T cell receptors.Citation58,Citation12 Here, the actin cytoskeleton of the dendritic cell polarizes toward the immunological synapse and this polarization is required for complete T cell activation.Citation2,Citation3
We tested whether SWAP70 would be enriched at the immunological synapse between dendritic cells and T cells with 2 model systems for immunological synapse formation. First, we performed a mixed leukocyte reaction, where human moDCs were co-incubated with leukocytes isolated from blood of a different donor. The C-type lectin DC-SIGN was used for labeling the dendritic cells, while the T cells were recognized by labeling for CD3. We did not observe clear localization of SWAP70 to the immunological synapse (). When we included zymosan particles during the dendritic cell incubation with T cells, we observed massive enrichment of SWAP70 to phagosomes, but not to the immunological synapse (). Second, we tested the recruitment of SWAP70 to an antigen-specific immunological synapse in mouse BMDCs. In these experiments, mouse FLT3L-differentiated BMDCs depleted of pDCs (B220+cells) were used as these better resemble the conventional resident dendritic cells than GM-CSF-differentiated BMDCs.Citation33,Citation59 The BMDCs were activated in presence of the adjuvants LPS and 5′-C-phosphate-G-3′ (CpG) and preincubated with a peptide contained in the model antigen ovalbumin (OVA, residues 257–264). These dendritic cells can present the OVA257–264 peptide in MHC class I to CD8+ cytotoxic T cells isolated from OT-I mice which express a T cell receptor specific for this peptide.Citation17 We observed almost complete exclusion of SWAP70 from the immunological synapse, while SWAP70 was detectable in the F-actin-rich region facing away from the immunological synapse (). Interestingly, in these FLT3L-differentiated BMDCs, SWAP70 was recruited to phagosomes containing latex beads of comparable size to T-cells (∼6 µm diameter) (). These data show that SWAP70 is not enriched at the immunological synapse between dendritic cells and T cells, but is specifically recruited to phagosomes.
Figure 5. SWAP70 is not enriched at the immunological synapse between dendritic cells and Tcells. (A) Representative confocal micrographs of human moDCs cultured with allogenic human peripheral-blood leukocytes and immunolabeled for the dendritic cell marker DC-SIGN (magenta in merge) and SWAP70 (red). F-actin was labeled with phalloidin (green). The right-hand scheme depicts the position of the moDC and T cell. Orange arrowhead: position of the immunological synapse. (B) Same as panel A, but now with immunolabeling for the T cell marker CD3 (magenta) instead of DC-SIGN, and exposure of the cells to zymosan for 1 h before fixation. Yellow arrowhead: SWAP70-positive zymosan-containing phagosome (marked with ‘P’ in scheme). (C) Same as panel A, but now with OVA-antigen loaded mouse FLT3L-differentiated BMDCs and OVA-antigen specific CD8+ cytotoxic OT-I T cells. Yellow arrowhead: SWAP70 localization opposite to the immunological synapse. (D) Confocal micrograph of FLT3L-differentiated BMDC pulsed with 6 µm-sized latex beads and immunostained for SWAP70. Yellow arrowhead: SWAP70-positive bead-containing phagosome. Scale bars: 10 µm.
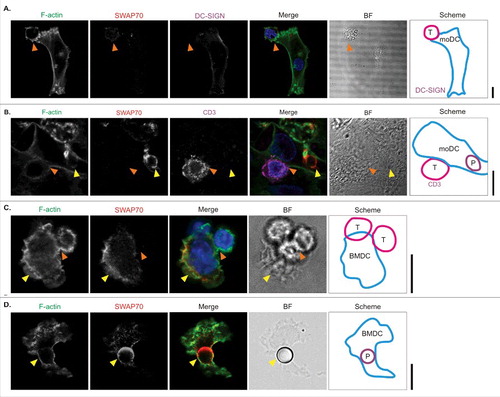
Discussion
In this study, we demonstrate that SWAP70 is recruited to phagosomes in murine dendritic cells and macrophages. This adds to our previous finding of transient recruitment of SWAP70 to phagosomes in human dendritic cells, and supports that SWAP70 could potentially be a general marker of early phagocytosis. We previously showed that phagosomal recruitment of SWAP70 is highly specific, and its recruitment to other F-actin-rich regions such as podosomes is much less compared with phagosomes.Citation6 In the present study, we show that SWAP70 is not enriched at the immunological synapse, even though F-actin has a role in synapse formation and organization.Citation2,Citation3,Citation12,Citation25,Citation58 SWAP70 colocalized at the phagosome with the Rho-GTPase Rac1 and F-actin, and this strengthens our previous conclusion that SWAP70 specifically tethers F-actin at the phagosome via Rac1 and PtdIns(3,4)P2.Citation6 Since we did not observe enrichment of SWAP70 to the immunological synapse, it seems logical that one or both of these factors are not sufficiently present at the synapse. Finally, we showed that SWAP70 promotes the inactivation of cofilin, likely by sequestering the inactive form of cofilin on the phagosomal surface as has been shown in vitro.Citation14 As cofilin is a severing factor of filamentous F-actin,Citation18 this likely contributes to the stabilization of F-actin on the phagosomal surface by SWAP70.Citation6
Our findings are of particular interest given the increasing evidence that SWAP70 has a potential role in cancer. SWAP70 is targeted by microRNAs in prostate cancers, and silencing of SWAP70 exhibited anti-tumor effects.Citation16 In mouse embryo fibroblasts (MEFs), SWAP70 was necessary for acquiring an oncogenic phenotype upon v-Src transformationCitation27,Citation41 as well as for their spontaneous transformation.Citation15 NIH3T3 cells and MEFs overexpressing SWAP70 bearing naturally occurring mutations exhibited increased growth rates and skewed signaling pathways.Citation26,Citation52 Our data now show that besides its previously described role in promoting oncogenesis, SWAP70 could also be required for the clearance of cancer material by phagocytes of the immune system. All these studies put SWAP70 forward as an interesting potential target for future anti-cancer therapeutic intervention.
Materials and methods
Animals
Wild-type female C57Bl/6JRccHsd mice were purchased from Charles River (Sulzfeld, Germany). For experiments including immune synapses of murine cells, the C57BL/6-Tg(TcraTcrb)1100Mjb/J/Thy1 strain (OT-1) was used. Mice were housed at the Central Animal Laboratory of the Radboud University Medical Center, Nijmegen. All animal experiments were performed under approval by the Animal Experimental Committee of the Radboud University Medical Center, and in accordance with European, national and institutional guidelines.
Cells
BMDCs were generated from 7–11 weeks old mice. Briefly, bone marrow cells were isolated and cultured in RPMI 1640 medium (Gibco), supplemented with 10% fetal calf serum (FCS), 1% L-alanyl-L-glutamine, 0.1% β-mercapto-ethanol and 0.5% antibiotics/antimycotics (AA, Gibco). For differentiation, this complete medium was supplemented with either 20 ng/ml GM-CSF or 200 ng/ml FLT3L (both from Peprotech) on the day of culture onset (Day 0). In both cases cells were used on day 8 of culture. MoDCs were generated as described,Citation7 human macrophages were generated from blood-derived adherent monocytes by culturing in presence of recombinant human M-CSF (40 ng/ml) (R&D Systems; 216-MC-005) for 6 d. The fully differentiated human moDCs and macrophages were validated by comparative expression analysis of CD14, CD163 and CD206 with flow cytometry. Primary mDCs and pDCs were isolated from donor's blood by magnetic bead separation with CD1c+ (Miltenyi Biotec; 130–090–506) and BDCA4+ (Miltenyi Biotec; 130–090–532) isolation kits.
SiRNA knockdown procedures are described.Citation6,Citation19 For live cell microscopy of RAW264.7 macrophages, cells were transfected with Fugene HD (Promega; Lot 0000027400; REF E231) following the manufacturer's guidelines. The SWAP70-GFP and LifeAct-RFP plasmids and imaging protocols have been described.Citation6 Cells were pulsed with IgG-opsonized zymosan as described.Citation6 Day 8 FLT3L-differentiated BMDCs (depleted of B220+ cells with mouse CD45R MicroBeads, Miltenyi Biotec; 130–049–501) and activated for 4 h with 1 µg/ml LPS and 1 µg/ml CpG-B 1826) were pulsed with 6.0 µm-sized Polybead Carboxylate beads (Polysciences; Cat. No. 17141) for 1 h before fixation and staining.
Immunological synapse models
For the mixed leukocyte reaction, moDCs cultured on coverslips (50,000 cells) were first activated with LPS (1 µg/ml) for 3 h in complete in RPMI 1640 medium containing 10% FCS, 1% L-alanyl-L-glutamine and AA in a humidified, 5% CO2-containing atmosphere. MoDCs were then co-cultured for another 3 h with peripheral leukocytes (100,000 cells) that were directly isolated from blood of a different donor. Zymosan was added at a 1:10 cell-to-particle ratio during the final 1 hour of incubation.
For the OVA-specific immunological synapses, day 8 FLT3L-differentiated BMDCs (depleted of B220+ cells) were activated for 4 h with LPS and CpG-B. The OVA-derived 257–264 (SIINFEKL) antigenic peptide was added during the last 30 min of incubation. OT-I splenocytes were isolated from a 6 month old OT-I male mouse with a CD8a+ T Cell Isolation Kit (Miltenyi Biotec; 130–104–075). CD8a+ T cells were labeled with CFSE (ThermoFisher; C34554) for 10 min at RT and washed with MACS buffer. They were then resuspended in BMDC medium at a ratio of 2 OT-I cells to 1 FLT3L-differentiated cell. Synapses were allowed to form by culturing for 3 h. Cells were fixed for 20 min in 4% PFA and stained for SWAP70 and F-actin as described.Citation6
Immunoblotting, flow cytometry, MTT and AnnexinV-7AAD viability assays
Detailed immunoblotting procedures are described.Citation6 Cofilin was immunolabeled with a rabbit monoclonal IgG anti-phospho cofilin (Ser3) (Cell Signaling; 3313P; used at 1:500). Immunolabeling protocols, FACS and MTT assays were performed as described.Citation6,Citation19 Cells were treated with sanguinarine at 2, 6, 12, 24 µM concentrations in RPMI medium from a 1 mM stock in methanol similar to as described for the SHIP inhibitors.Citation6 2.4% methanol in RPMI was used as a solvent control (i.e., equivalent to the methanol content at the highest concentration of sanguinarine). AnnexinV-FITC (BD Biosciences; 556419) and 7AAD (eBioscience; 00–6993–50) viability tests were done according to the manufacturer's guidelines. In short, drug-treated cells were stained on ice for 10 min in the presence of 1.5 mM CaCl2.
Microscopy
Protocols for immunofluorescence labeling and staining of the phagocytic cup with zymosan-FITC are described.Citation6 The following primary antibodies were used: rabbit polyclonal anti-SWAP70 (Novus Biologicals; cat. no NBP1–82979) at 1:200 dilution (v/v) for IF and 1:500 for Western blot; mouse monoclonal anti-Rac1 (Cell Biolabs; 240106) at 1:100; mouse IgG1 anti-FITC Alexa fluor 647 (Jackson ImmunoResearch; 200–602–037) at 1:200; mouse IgG2b anti-human DC-SIGN (BD PharMingen;551186; clone DCN46) at 1:100; mouse IgG2a kappa anti-CD3 (BioXcell; clone OKT3) at 500 ng/ml. Alexa fluor 633 labeled phalloidin was from ThermoFisher (A22284) and used at 1:200 (v/v). The following secondary antibodies were used: goat-anti-mouse Alexa fluor 488 (Life Technologies; A11029); goat-anti-rabbit Alexa fluor 568 or 647 (Life Technologies; A11036 and A21245). Samples were imaged on a Leica SP8 confocal microscope as described,Citation6 or on a Zeiss LSM880 confocal microscope with 405 nm and 561 nm diode lasers, a multi-line argon laser and a 633 nm laser for excitation and a 63 × 1.40 NA oil immersion objective. Live cell microscopy was performed on a Leica DMI6000 epi-fluorescence microscope as described.Citation6
Statistics
All data were analyzed for a minimum of 3 independent donors/repeats using 1-way ANOVA (with post hoc Tukey) or with Student's 2-tailed paired t tests (*P < 0.05; **P < 0.01; ***P < 0.001; ns: not significant). Data are plotted as average ± SEM.
Disclosure of potential conflicts of interest
No potential conflicts of interest were disclosed.
Supplemental_materials.zip
Download Zip (3.3 MB)Funding
GvdB is the recipient of an Hypatia fellowship from the Radboud University Medical Center, a Career Development Award from the Human Frontier Science Program, a Starting Grant from the European Research Council (ERC) under the European Union's Seventh Framework Program (Grant agreement number 336479), a grant from the Gravitation Program 2013 from the Netherlands Organization for Scientific Research (NWO ICI-024.002.009), and a VIDI grant from NWO (ALW864.14.001). NHR is the recipient of a VENI grant from NWO (ALW016.Veni.171.097) and a grant from the European Molecular Biology Organization (EMBO ALTF232–2016).
References
- Agarwal S, Reynolds MA, Pou S, Peterson DE, Charon JA, Suzuki JB. The effect of sanguinarine on human peripheral blood neutrophil viability and functions. Oral Microbiol Immunol 1991; 6:51-61; PMID:1945482; https://doi.org/10.1111/j.1399-302X.1991.tb00451.x
- Al-Alwan MM, Liwski RS, Haeryfar SM, Baldridge WH, Hoskin DW, Rowden G, West KA. Cutting edge: dendritic cell actin cytoskeletal polarization during immunological synapse formation is highly antigen-dependent. J Immunol (Baltimore, Md.: 1950) 2003; 171:4479-83; PMID:14568920; https://doi.org/10.4049/jimmunol.171.9.4479
- Al-Alwan MM, Rowden G, Lee TD, West KA. The dendritic cell cytoskeleton is critical for the formation of the immunological synapse. J Immunol (Baltimore, Md.: 1950) 2001; 166:1452-6; PMID:11160183; https://doi.org/10.4049/jimmunol.166.3.1452
- Allen LA, Aderem A. Mechanisms of phagocytosis. Curr Opin Immunol 1996; 8:36-40; PMID:8729444; https://doi.org/10.1016/S0952-7915(96)80102-6
- Arber S, Barbayannis FA, Hanser H, Schneider C, Stanyon CA, Bernard O, Caroni P. Regulation of actin dynamics through phosphorylation of cofilin by LIM-kinase. Nature 1998; 393:805-9; PMID:9655397; https://doi.org/10.1038/31729
- Baranov MV, Revelo NH, Dingjan I, Maraspini R, Ter Beest M, Honigmann A, van den Bogaart G. SWAP70 Organizes the Actin Cytoskeleton and Is Essential for Phagocytosis. Cell Rep 2016; 17:1518-31; PMID:27806292; https://doi.org/10.1016/j.celrep.2016.10.021
- Baranov MV, Ter Beest M, Reinieren-Beeren I, Cambi A, Figdor CG, van den Bogaart G. Podosomes of dendritic cells facilitate antigen sampling. J Cell Sci 2014a; 127:1052-64; https://doi.org/10.1242/jcs.141226
- Baranov MV, Ter Beest M, van den Bogaart G. Reaching for far-flung antigen: How solid-core podosomes of dendritic cells transform into protrusive structures. Commun Integr Biol 2014b; 7:e97069611; https://doi.org/10.4161/cib.29084
- Barreira da Silva R, Graf C, Munz C. Cytoskeletal stabilization of inhibitory interactions in immunologic synapses of mature human dendritic cells with natural killer cells. Blood 2011; 118:6487-98; PMID:21917751; https://doi.org/10.1182/blood-2011-07-366328
- Borg C, Jalil A, Laderach D, Maruyama K, Wakasugi H, Charrier S, Ryffel B, Cambi A, Figdor C, Vainchenker W, et al. NK cell activation by dendritic cells (DCs) requires the formation of a synapse leading to IL-12 polarization in DCs. Blood 2004; 104:3267-75; PMID:15242871; https://doi.org/10.1182/blood-2004-01-0380
- Bravo-Cordero JJ, Magalhaes MA, Eddy RJ, Hodgson L, Condeelis J. Functions of cofilin in cell locomotion and invasion. Nat Rev Mol Cell Biol 2013; 14:405-15; PMID:23778968; https://doi.org/10.1038/nrm3609
- Brossard C, Feuillet V, Schmitt A, Randriamampita C, Romao M, Raposo G, Trautmann A. Multifocal structure of the T cell - dendritic cell synapse. Euro J Immunol 2005; 35:1741-53; PMID:15909310; https://doi.org/10.1002/eji.200425857
- Caron E, Hall A. Identification of two distinct mechanisms of phagocytosis controlled by different Rho GTPases. Science (New York, N.Y.) 1998; 282:1717-21; https://doi.org/10.1126/science.282.5394.1717
- Chacon-Martinez CA, Kiessling N, Winterhoff M, Faix J, Muller-Reichert T, Jessberger R. The switch-associated protein 70 (SWAP-70) bundles actin filaments and contributes to the regulation of F-actin dynamics. J Biol Chem 2013; 288:28687-703; PMID:23921380; https://doi.org/10.1074/jbc.M113.461277
- Chang YT, Shu CL, Lai JY, Lin CY, Chuu CP, Morishita K, Ichikawa T, Jessberger R, Fukui Y. SWAP-70 contributes to spontaneous transformation of mouse embryo fibroblasts. Exp Cell Res 2016; 345:150-7; PMID:26103139; https://doi.org/10.1016/j.yexcr.2015.06.011
- Chiyomaru T, Tatarano S, Kawakami K, Enokida H, Yoshino H, Nohata N, Fuse M, Seki N, Nakagawa M. SWAP70, actin-binding protein, function as an oncogene targeting tumor-suppressive miR-145 in prostate cancer. Prostate 2011; 71:1559-67; PMID: 21360565
- Clarke SR, Barnden M, Kurts C, Carbone FR, Miller JF, Heath WR. Characterization of the ovalbumin-specific TCR transgenic line OT-I: MHC elements for positive and negative selection. Immunol Cell Biol 2000; 78:110-7; PMID:10762410; https://doi.org/10.1046/j.1440-1711.2000.00889.x
- Condeelis J. How is actin polymerization nucleated in vivo? Trends Cell Biol 2001; 11:288-93; PMID:11413039; https://doi.org/10.1016/S0962-8924(01)02008-6
- Dingjan I, Verboogen DR, Paardekooper LM, Revelo NH, Sittig SP, Visser LJ, Mollard GF, Henriet SS, Figdor CG, Ter Beest M, et al. Lipid peroxidation causes endosomal antigen release for cross-presentation. Sci Rep 2016; 6:22064; PMID:26907999; https://doi.org/10.1038/srep22064
- Eichelbaum K, Krijgsveld J. Rapid temporal dynamics of transcription, protein synthesis, and secretion during macrophage activation. Mol Cell Proteomics 2014; 13:792-810; https://doi.org/10.1074/mcp.M113.030916
- Ferrari G, Langen H, Naito M, Pieters J. A coat protein on phagosomes involved in the intracellular survival of mycobacteria. Cell 1999; 97:435-47; PMID:10338208; https://doi.org/10.1016/S0092-8674(00)80754-0
- Fooksman DR, Shaikh SR, Boyle S, Edidin M. Cutting edge: phosphatidylinositol 4,5-bisphosphate concentration at the APC side of the immunological synapse is required for effector T cell function. J Immunol (Baltimore, Md.: 1950) 2009; 182:5179-82; PMID:19380760; https://doi.org/10.4049/jimmunol.0801797
- Frantz C, Barreiro G, Dominguez L, Chen X, Eddy R, Condeelis J, Kelly MJ, Jacobson MP, Barber DL. Cofilin is a pH sensor for actin free barbed end formation: role of phosphoinositide binding. J Cell Biol 2008; 183:865-79; PMID:19029335; https://doi.org/10.1083/jcb.200804161
- Freeman SA, Grinstein S. Phagocytosis: receptors, signal integration, and the cytoskeleton. Immunol Rev 2014; 262:193-215; PMID:25319336; https://doi.org/10.1111/imr.12212
- Friedl P, den Boer AT, Gunzer M. Tuning immune responses: diversity and adaptation of the immunological synapse. Nat Rev Immunol 2005; 5:532-45; PMID:15999094; https://doi.org/10.1038/nri1647
- Fukui Y, Ihara S. A mutant of SWAP-70, a phosphatidylinositoltrisphosphate binding protein, transforms mouse embryo fibroblasts, which is inhibited by sanguinarine. PloS One 2010; 5:e14180; PMID:21152038; https://doi.org/10.1371/journal.pone.0014180
- Fukui Y, Tanaka T, Tachikawa H, Ihara S. SWAP-70 is required for oncogenic transformation by v-Src in mouse embryo fibroblasts. Biochem Biophys Res Commun 2007; 356:512-6; PMID:17367752; https://doi.org/10.1016/j.bbrc.2007.03.011
- Gandhi M, Achard V, Blanchoin L, Goode BL. Coronin switches roles in actin disassembly depending on the nucleotide state of actin. Mol Cell 2009; 34:364-74; PMID:19450534; https://doi.org/10.1016/j.molcel.2009.02.029
- Garbe AI, Roscher A, Schuler C, Lutter AH, Glosmann M, Bernhardt R, Chopin M, Hempel U, Hofbauer LC, Rammelt S, et al. Regulation of bone mass and osteoclast function depend on the F-actin modulator SWAP-70. J Bone Miner Res 2012; 27:2085-96; PMID:22648978; https://doi.org/10.1002/jbmr.1670
- Gomez-Cambronero J. Structure analysis between the SWAP-70 RHO-GEF and the newly described PLD2-GEF. Small GTPases 2012; 3:202-8; PMID:22858691; https://doi.org/10.4161/sgtp.20887
- Gotz A, Jessberger R. Dendritic cell podosome dynamics does not depend on the F-actin regulator SWAP-70. PloS One 2013; 8:e60642; PMID:23544157; https://doi.org/10.1371/journal.pone.0060642
- Guilliams M, Malissen B. A Death Notice for In-Vitro-Generated GM-CSF Dendritic Cells? Immunity 2015; 42:988-90; PMID:26084019; https://doi.org/10.1016/j.immuni.2015.05.020
- Helft J, Bottcher J, Chakravarty P, Zelenay S, Huotari J, Schraml BU, Goubau D, Reis e Sousa C. GM-CSF mouse bone marrow cultures comprise a heterogeneous population of CD11c(+)MHCII(+) macrophages and dendritic cells. Immunity 2015; 42:1197-211; PMID:26084029; https://doi.org/10.1016/j.immuni.2015.05.018
- Hilpela P, Oberbanscheidt P, Hahne P, Hund M, Kalhammer G, Small JV, Bahler M. SWAP-70 identifies a transitional subset of actin filaments in motile cells. Mol Biol Cell 2003; 14:3242-53; PMID:12925760; https://doi.org/10.1091/mbc.E03-01-0043
- Ihara S, Oka T, Fukui Y. Direct binding of SWAP-70 to non-muscle actin is required for membrane ruffling. J Cell Sci 2006; 119:500-7; PMID:16418221; https://doi.org/10.1242/jcs.02767
- Ito T, Inaba M, Inaba K, Toki J, Sogo S, Iguchi T, Adachi Y, Yamaguchi K, Amakawa R, Valladeau J, et al. A CD1a+/CD11c+ subset of human blood dendritic cells is a direct precursor of Langerhans cells. J Immunol (Baltimore, Md. : 1950) 1999; 163:1409-19; PMID: 10415041
- Kuiper JW, Pluk H, Oerlemans F, van Leeuwen FN, de Lange F, Fransen J, Wieringa B. Creatine kinase-mediated ATP supply fuels actin-based events in phagocytosis. PLoS Biol 2008; 6:e51; PMID:18336068; https://doi.org/10.1371/journal.pbio.0060051
- Liebl D, Griffiths G. Transient assembly of F-actin by phagosomes delays phagosome fusion with lysosomes in cargo-overloaded macrophages. J Cell Sci 2009; 122:2935-45; PMID:19638408; https://doi.org/10.1242/jcs.048355
- Lutz MB, Inaba K, Schuler G, Romani N. Still Alive and Kicking: In-Vitro-Generated GM-CSF Dendritic Cells! Immunity 2016; 44:1-2; PMID:26789912; https://doi.org/10.1016/j.immuni.2015.12.013
- May RC, Caron E, Hall A, Machesky LM. Involvement of the Arp2/3 complex in phagocytosis mediated by FcgammaR or CR3. Nat Cell Biol 2000; 2:246-8; PMID:10783245; https://doi.org/10.1038/35008673
- Murugan AK, Ihara S, Tokuda E, Uematsu K, Tsuchida N, Fukui Y. SWAP-70 is important for invasive phenotypes of mouse embryo fibroblasts transformed by v-Src. IUBMB Life 2008; 60:236-40; PMID:18344189; https://doi.org/10.1002/iub.33
- Oberbanscheidt P, Balkow S, Kuhnl J, Grabbe S, Bahler M. SWAP-70 associates transiently with macropinosomes. Euro J Cell Biol 2007; 86:13-24; PMID:17046101; https://doi.org/10.1016/j.ejcb.2006.08.005
- Ocana-Morgner C, Gotz A, Wahren C, Jessberger R. SWAP-70 restricts spontaneous maturation of dendritic cells. J Immunol (Baltimore, Md. : 1950) 2013; 190:5545-58; PMID:23636062; https://doi.org/10.4049/jimmunol.1203095
- Ocana-Morgner C, Reichardt P, Chopin M, Braungart S, Wahren C, Gunzer M, Jessberger R. Sphingosine 1-phosphate-induced motility and endocytosis of dendritic cells is regulated by SWAP-70 through RhoA. J Immunol (Baltimore, Md. : 1950) 2011; 186:5345-55; PMID:21421853; https://doi.org/10.4049/jimmunol.1003461
- Ocana-Morgner C, Wahren C, Jessberger R. SWAP-70 regulates RhoA/RhoB-dependent MHCII surface localization in dendritic cells. Blood 2009; 113:1474-82; PMID:18802007; https://doi.org/10.1182/blood-2008-04-152587
- Pearce G, Audzevich T, Jessberger R. SYK regulates B-cell migration by phosphorylation of the F-actin interacting protein SWAP-70. Blood 2011; 117:1574-84; PMID:21123826; https://doi.org/10.1182/blood-2010-07-295659
- Raschke WC, Baird S, Ralph P, Nakoinz I. Functional macrophage cell lines transformed by Abelson leukemia virus. Cell 1978; 15:261-7; PMID:212198; https://doi.org/10.1016/0092-8674(78)90101-0
- Ritter AT, Angus KL, Griffiths GM. The role of the cytoskeleton at the immunological synapse. Immunol Rev 2013; 256:107-17; PMID:24117816; https://doi.org/10.1111/imr.12117
- Robinson SP, Patterson S, English N, Davies D, Knight SC, Reid CD. Human peripheral blood contains two distinct lineages of dendritic cells. Euro J Immunol 1999; 29:2769-78; PMID:10508251; https://doi.org/10.1002/(SICI)1521-4141(199909)29:09%3c2769::AID-IMMU2769%3e3.0.CO;2-2
- Rohatgi R, Nollau P, Ho HY, Kirschner MW, Mayer BJ. Nck and phosphatidylinositol 4,5-bisphosphate synergistically activate actin polymerization through the N-WASP-Arp2/3 pathway. J Biol Chem 2001; 276:26448-52; PMID:11340081; https://doi.org/10.1074/jbc.M103856200
- Shinohara M, Terada Y, Iwamatsu A, Shinohara A, Mochizuki N, Higuchi M, Gotoh Y, Ihara S, Nagata S, Itoh H, Fukui Y, Jessberger R. SWAP-70 is a guanine-nucleotide-exchange factor that mediates signalling of membrane ruffling. Nature 2002; 416:759-63; PMID:11961559; https://doi.org/10.1038/416759a
- Shu CL, Jing Yang L, Su LC, Chuu CP, Fukui Y. SWAP-70: a new type of oncogene. PloS One 2013; 8:e59245; PMID:23555004; https://doi.org/10.1371/journal.pone.0059245
- Shurin GV, Tourkova IL, Chatta GS, Schmidt G, Wei S, Djeu JY, Shurin MR. Small rho GTPases regulate antigen presentation in dendritic cells. J Immunol (Baltimore, Md. : 1950) 2005; 174:3394-400; PMID:15749872; https://doi.org/10.4049/jimmunol.174.6.3394
- Sivalenka RR, Jessberger R. SWAP-70 regulates c-kit-induced mast cell activation, cell-cell adhesion, and migration. Mol Cell Biol 2004; 24:10277-88; PMID:15542837; https://doi.org/10.1128/MCB.24.23.10277-10288.2004
- Swanson JA. Shaping cups into phagosomes and macropinosomes. Nat Rev Mol Cell Biol 2008; 9:639-49; PMID:18612320; https://doi.org/10.1038/nrm2447
- Toshima J, Toshima JY, Takeuchi K, Mori R, Mizuno K. Cofilin phosphorylation and actin reorganization activities of testicular protein kinase 2 and its predominant expression in testicular Sertoli cells. J Biol Chem 2001; 276:31449-58; PMID:11418599; https://doi.org/10.1074/jbc.M102988200
- van Rheenen J, Song X, van Roosmalen W, Cammer M, Chen X, Desmarais V, Yip SC, Backer JM, Eddy RJ, Condeelis JS. EGF-induced PIP2 hydrolysis releases and activates cofilin locally in carcinoma cells. J Cell Biol 2007; 179:1247-59; PMID:18086920; https://doi.org/10.1083/jcb.200706206
- Verboogen DR, Dingjan I, Revelo NH, Visser LJ, ter Beest M, van den Bogaart G. The dendritic cell side of the immunological synapse. Biomol Concepts 2016; 7:17-28; PMID:26741354; https://doi.org/10.1515/bmc-2015-0028
- Xu Y, Zhan Y, Lew AM, Naik SH, Kershaw MH. Differential development of murine dendritic cells by GM-CSF versus Flt3 ligand has implications for inflammation and trafficking. J Immunol (Baltimore, Md. : 1950) 2007; 179:7577-84; PMID:18025203; https://doi.org/10.4049/jimmunol.179.11.7577
- Yang N, Higuchi O, Ohashi K, Nagata K, Wada A, Kangawa K, Nishida E, Mizuno K. Cofilin phosphorylation by LIM-kinase 1 and its role in Rac-mediated actin reorganization. Nature 1998; 393:809-12; PMID:9655398; https://doi.org/10.1038/31735