ABSTRACT
Autophagy is the process where cytosolic components are digested by the cell. This process is required for cell survival in stressful conditions. It was also shown to control cell division and more recently, cell morphology and migration. We characterized signalling pathways enabling embryonic epidermal cells of the nematode Caenorhabditis elegans to elongate along their antero-posterior axis. Previous studies revealed that epidermal cells can adopt either a RhoA-like or a Rac1-like morphogenic program. We show here that the AMP-activated protein kinase (AMPK) and genes controlling autophagy are required for proper elongation of epidermal cells following the RhoA-like program and are dispensable for other cells. This suggests that AMPK-autophagy is used by the embryo to fuel the most energy-demanding morphogenic processes promoting early elongation.
Introduction
Macroautophagy (hereafter called autophagy) is an autodigestive process induced in response to various metabolic stresses. It acts as a quality control and as a cell survival-enabling system.Citation1 Dysfunction of autophagy has been linked to tumorigenesis, neurodegeneration and the development of immune diseases.Citation2 Recently, autophagy has also been involved in the control of epithelial-mesenchymal transition (EMT) and cancer cell metastasisCitation3 suggesting a function for the autophagy machinery during epithelial morphogenesis. This function remains, however, to be clarified.
During late embryogenesis of the nematode Caenorhabditis elegans, the epidermis (called the hypodermis), which is composed of dorsal, lateral and ventral cells, goes through several morphogenic stages, controlling the overall shape of the embryo.Citation4 During the early elongation stage, myosin contraction at the apical pole of hypodermal cells promotes the elongation of the embryos along their antero-posterior axis.Citation5-Citation8 Two main signalling programs have been shown to control these contraction events in a subset of hypodermal cells. A RhoA-like program, involving the RhoA GTPase's effector kinase ROCK/LET-502, controls the morphogenesis of lateral hypodermal cells,Citation5,7,8 while a Rac1-like program, involving the Rac1/Cdc42-specific Guanine-nucleotide exchange factor β-PIX/PIX-1 and effector PAK1/PAK-1, controls the morphogenesis of dorsal and ventral hypodermis.Citation5,6,8
In C. elegans, autophagy machinery has been involved in the removal of cell corpses by promoting phagosome maturation.Citation9,10 However, the potential involvement of autophagy in controlling epidermal morphogenesis has never been investigated in this system. In this article, we show that genes controlling the autophagy, as well as the ATP-sensor kinase, AMPK/aak-1/2, are required for the morphogenesis of a subset of hypodermal cells as part of the RhoA-like program. These data suggest that cell-to-cell heterogeneity, previously observed within the hypodermis during morphogenesis, is also characterized by pathways providing cells with energy.
Results
Autophagy and apoptosis were previously shown to function in a redundant manner to control morphogenesis of Caenorhabditis elegans embryos.Citation11 However, the specific function of autophagy during epidermal morphogenesis, more specifically during early elongation, has never been investigated. We used embryos expressing both the filamentous actin (F-actin)-binding probe VAB-10(ADB)::mCherry, expressed under the control of the hypodermal specific promoter lin-26p, and the autophagy marker GFP::LGG-1/Atg8/LC3, to test whether autophagy occurs in hypodermal cells. The autophagy marker was observed throughout the embryo during early elongation, including the hypodermal cells (), suggesting that autophagy occurs within these cells during early elongation.
Figure 1. Autophagy is activated in lateral cells and controls early elongation downstream of AMPK. A) Confocal images showing autophagosomes, labelled by GFP::LGG-1 (green), observed in hypodermal cells, identified using the F-actin binding probe ABD::mCherry marker (red), during early elongation. Scale bar: 10 μm. B) Graph bar representing the average number of autophagosome in dorsal and in lateral anterior cells C) Graph bar representing the average ratio of autophagosome number in lateral versus dorsal cells. D) Confocal images showing autophagosome labelled by GFP::LGG-1 in embryo submitted to RNAi against indicated genes. E) Graph bar representing the average number of autophagosome in embryo submitted to RNAi against the indicated genes. F) Graph bar representing average deformation rate (AD in μm/min) of transversal junctions in wt, let-502(RNAi), aak-1/2(RNAi), bec-1(RNAi) and epg-8(RNAi) embryos expressing AJM-1::GFP and VAB-10(ABD)::mCherry. t test p-values only significant difference from wt (*) are indicated. Error bars indicate SEM. N indicates the number of embryos used for the quantifications.
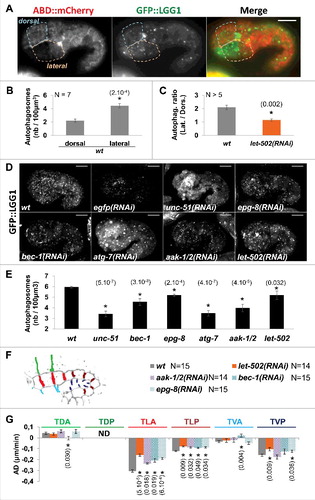
Considering that anterior lateral and dorsal hypodermal cells were shown to follow different morphogenic programs during early elongation,Citation7 we assessed whether these cells displayed different numbers of autophagosomes. This data revealed that anterior lateral cells displayed twice the average number of autophagosomes found in dorsal anterior cells (, and ). Since morphogenesis of lateral cells depends on a RhoA-like program involving the RHO-1 effector kinase LET-502/ROCK, we assessed whether alteration of this program also affects the heterogeneous distribution of autophagosomes in the hypodermis. Interestingly, ABD::mCherry;GFP::LGG-1 embryos submitted to let-502(RNAi) displayed a significant lower number of autophagosomes in anterior lateral cells when compared to dorsal cells (). This suggests, that autophagy is more active in lateral cells than in dorsal cells during early elongation, but only when the RhoA-like program is active in embryos. This also suggests that the RhoA-like morphogenic program controls, to a certain extent, the activation of autophagy in embryos.
The RhoA-like program drives the lateral cell changes during early elongation, and is characterized by the highest shrinking rates of transversal membranes.Citation7 This shrinking is expected to be dependent on the contraction of myosin, which is a highly energy-demanding bioprocess.Citation5 Since, autophagy was shown, in several models, to provide cells with energy, we hypothesized that the prominent activation of autophagy in lateral cells may result from an energetic stress applied on these cells by high level of myosin ATPase activity. Autophagy was also shown to be activated in response to energetic stress downstream of the AMP-activated protein kinase (AMPK) energy sensor.Citation12 While C. elegans homologs of AMPK α-catalytic subunits, aak-1 and aak-2, were shown to regulate germ-line stem cell quiescence and proliferation,Citation13 their function during embryogenesis has never been investigated. We consequently tested whether autophagy may be activated in embryos downstream of AMPK activation. To do so, GFP::LGG-1 embryos were submitted to aak-1(RNAi);aak-2(RNAi) (called aak-1/2(RNAi) hereafter). The aak-1/2(RNAi) embryos had significantly fewer autophagosomes in comparison to GFP::LGG-1 control embryos, when considering the entire embryo ( and ). These data suggest therefore, that an AMPK/autophagy pathway is activated in embryos during early elongation, presumably in response to energetic stress.
To further characterize the function of AMPK/autophagy pathway during early elongation, we first assessed whether we could significantly reduce the number of autophagosome in embryos by knocking-down genes shown to control autophagy in C. elegans. To do so, we submitted nematodes expressing ABD::mCherry;GFP::LGG-1 to RNAi against Beclin/bec-1, Atg14/epg-8, Atg1/unc-51 and Atg7/atg-7, controlling induction of autophagy, autophaosome nucleation and vesicle elongation.Citation14-Citation16 As expected, a significant reduction in the number of autophagosomes was observed in RNAi-treated embryos during early elongation stage in comparison to control embryos ( and ).
We then assessed whether these genes control epidermal morphogenesis, and measured the average deformation rate (AD) of transversal junctions between dorsal anterior cells (TDA), between lateral anterior and posterior cells (TLA and TLP respectively) and between ventral anterior and posterior cells (TVA and TVP respectively) as previously done.Citation7 This was done in nematodes expressing the junctional protein AJM-1::GFP and the F-actin-binding probe VAB-10(ABD)::mCherry and submitted to RNAi against bec-1, epg-8, aak-1/2 and let-502 ( and ). This revealed that genes controlling autophagy and AMPK activity control deformation rate of transversal junction between lateral cells as shown for let-502 (TLA and TLP; and ).
Since the autophagy machinery contributes to cell corpse clearance via phagocytosis during embryogenesis, we also assessed whether the morphogenic defects in bec-1(RNAi) and epg-8(RNAi) may be due to the inefficient clearance of corpses. To do so, we measured the AD of transversal junction in ced-5(RNAi) animals displaying severe alteration of phagocytosis and cell corpse clearance (Fig. S1). AD of transversal junctions was not significantly different in ced-5(RNAi) versus wt animals during early elongation (Fig. S1), suggesting that autophagy controls the deformation of cell-cell junctions during early elongation in a phagocytosis-independent manner.
To further characterize the function of AMPK and autophagy during epidermal morphogenesis, we tested the genetic interactions of aak-1/2 and autophagy genes with genes controlling these programs. To do so, we submitted wt, let-502(sb118ts) upshifted to restrictive temperature, as well as pak-1(ok448) and pix-1(gk416) null mutants to control eGFP (RNAi), aak- 1/2(RNAi), epg-8(RNAi), bec-1(RNAi), unc-51(RNAi) or atg-7(RNAi), and measured the length of embryos at the end of the early elongation using DIC microscopy. All the genes tested were required for early elongation ( and ), supporting that the AMPK/autophagy pathway controls the early elongation of embryos. This also revealed that aak-1/2(RNAi), epg-8(RNAi) and bec-1(RNAi) enhanced the elongation defects of both pix-1(gk416) and pak-1(ok448) but did not enhance those of let-502(sb118ts) ( and ). Similar results were obtained when submitting aak-1(tm1944);aak-2(ok524) animals, which are catalytically null for AMPK,Citation13 to let-502(RNAi) (). These data suggest that aak-1/2 and autophagy genes function in the same pathway as let-502, supporting that the AMPK/autophagy pathway regulates the early elongation of embryos.
Figure 2. aak-1/2, bec-1 and epg-8 control the morphogenesis of lateral hypodermal cells as part of the RhoA-like program. A) DIC images showing embryo at the end of early elongation in wt, epg-8(RNAi), aak-1/2(RNAi), let-502(sb118ts), let-502(sb118ts);epg-8(RNAi) and let-502(sb118ts);aak-1/2(RNAi) animals. B) Graph bar representing the length of the embryos at the end of the early elongation in wt, pak-1(ok448), pix-1(gk416), let-502(sb118ts) at 25.5°C and aak-1(tm1944);aak-2(ok524) animals treated by RNAi against eGFP (control), aak-1;aak-2 or let-502. C) Schematic representation of embryos indicating the L2 transversal junctions between lateral anterior cells. D) Graph bar representing average deformation (AD) of L2 junctions in embryos with indicated genotypes. T-test p-values are indicated in parentheses. *, T-test p-value significantly different from wt (A and C) or egfp(RNAi) (B). Error bars indicate SEM. N indicates the number of embryos used for the quantifications.
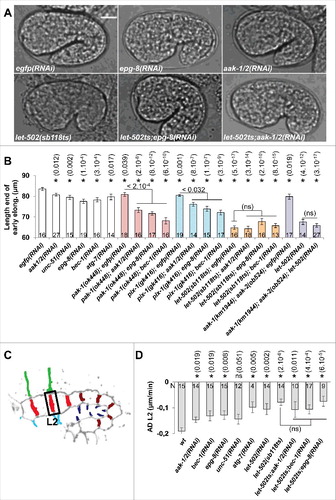
We previously showed that lateral cells adopt a Rac1-like program when ROCK/let-502 is genetically compromised.Citation7 We consequently assessed whether aak-1/2 and autophagy genes controlled the remodelling of junctions between the anterior lateral cells (L2 junction) when the Rac1-like program becomes activated in these cells. Interestingly, aak-1/2(RNAi), epg-8(RNAi) and bec-1(RNAi) did not significantly aggravate the deformation rate defects observed at L2 in let-502(sb118ts) animals at restrictive temperature (). These data suggest that AMPK and autophagy control the remodelling of cell-cell junction between lateral hypodermal cells only when the RhoA-like program is functional in these cells.
Discussion
We have previously shown that the hypodermal cells follow two alternative morphogenic programs during early elongation in C. elegans: a RhoA-like program and a Rac1-like program.Citation7 While controlling embryo morphogenesis in a collaborative manner when observed at the organism level, these programs were shown to control the remodelling of the apical junctions in an antagonistic manner at the single cell level.Citation7 The data presented in the current study suggest that the adoption of any of these programs also impacts on pathways providing energy to the cells. Indeed, epidermal morphogenesis involving the RhoA-like program may rely on the function of the ATP-sensor AMPK/AAK-1/2 and autophagy genes including Beclin/bec-1, ATG14/epg-8, ATG11/unc-51 and ATG7/atg-7 (). Since these genes become dispensable when the Rac1-like program is activated in the absence of ROCK/LET-502-, the Rac1-like program may be less energy-demanding than the RhoA-like program, and may not require the AMPK/autophagy pathway to support morphogenesis (). It may also suggest that the energy required to support the Rac1-like program is provided by an AMPK/autophagy-independent pathway.
Figure 3. Data presented in this study are in agreement with the following model. In wild-type embryos (left panel), high level of myosin contraction downstream of ROCK/LET-502 activation may induce a reduction of the ATP/ADP ratio resulting to the activation of the AMPK/AAK-1/2. Autophagy activation downstream of AMPK may result to the generation of ATP, which supports myosin contraction. Upon let-502 knock-down (right panel), the Rac1-like program involving PIX-1 and PAK-1 controls the remodelling of cell-cell junctions between lateral cells in an AMPK and autophagy-independent manner.
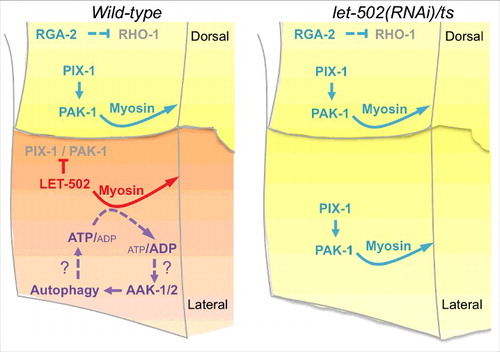
Our data are consistent with a study showing that RhoA and ROCK activate starvation-induced autophagy in Hela cells while, Rac1 was identified as an autophagy inhibitor in this system.Citation17 Functional cross talk between AMPK/autophagy and RhoA/ROCK was observed in several systems, including epithelial MDCK cells,Citation18 vascular smooth muscle cellsCitation19,20 and neurons.Citation21 While RhoA/ROCK appears to induce autophagy in starvation conditions,Citation18 the function of AMPK is less clear.Citation18-Citation20 This suggests a complex relationship between AMPK/autophagy and RhoA/ROCK that may tightly coordinate energy production and consumption in cells undergoing morphogenesis ().
Interestingly, Beclin/bec-1 and other autophagy genes were recently shown to control stem cell proliferation during germ-line development in C. elegans together with the insulin IGF-1 receptor (IIR)/daf-2 pathway.Citation22 This latter pathway was also previously shown to control early elongation of C. elegans embryos as part of the ROCK/let-502 pathway.Citation8 Altogether, these data are consistent with the insulin, AMPK and autophagy pathways controlling early elongation as part of the RhoA-like program in C. elegans embryos.
In conclusion, this study showed that AMPK and genes controlling autophagy contribute to the RhoA-like program controlling the morphogenesis of lateral hypodermal cells during early elongation of C. elegans embryos. This study provides a deeper characterization of the cell-to-cell heterogeneity existing within the hypodermis of C. elegans embryos. It also suggests that the adoption by a cell, of a given morphogenic program, also influences its metabolic behaviour.
Material and methods
Strains and culture methods
Control N2 (wild-type; wt) and other C. elegans strains were grown under standard culture conditions at 20°C (Brenner, 1974). Worm strains carrying the following mutations and marker were obtained from the Caenorhabditis Genetic Center (CGC): pix-1(gk416) X (VC863), pak-1(ok448) X (RB689), jcIs1 [ajm-1::GFP + unc-29(+) + rol-6(su1006)] IV (SU93), mcIs40 [lin-26p::ABDvab-10::mCherry + myo-2p::GFP] (ML916), adIs2122 [lgg-1p::GFP::lgg-1 + rol-6(su1006)] (DA2123). The strain carrying let-502(sb118ts) I and let-502(sb118ts) I; jcIs1 [ajm-1::GFP + unc-29(+) + rol-6(su1006)] IV were kindly and respectively provided by Dr P. Mains (University of Calgary, Calgary, AB, Canada) and Dr A. Piekny (Concordia University, Montreal, QC, Canada). aak-1(tm1944);aak-2(ok524) was kindly provided by Dr Richard Roy (McGill University, Montreal, Qc, Canada). wt, pix-1(gk416) or pak-1(ok448) strains expressing both AJM-1::GFP and VAB-10(ABD)::mCherry were generated as previously described.Citation8 adIs2122 [lgg-1p::GFP::lgg-1 + rol-6(su1006)]; mcIs40 [lin-26p::ABDvab-10::mCherry + myo-2p::GFP] was generated after crossing ML916 males with DA2123 hermaphrodites.
RNA interference treatment
pL4440 constructs containing ced-5, bec-1, epg-8, unc-51, atg-7, aak-1, aak-2, let-502 coding sequences were retrieved from the genome-wide libraryCitation23 and confirmed by sequencing. RNAi treatments were done following the procedure that we previously described.Citation7 Briefly, synchronized L1 animals were grown in S-Basal medium for 60–72 h at 20°C with HT115 bacteria transformed with appropriate pL4440 vector and previously induced for 1h at 37°C for RNAi production by IPTG (30 μg/ml). For double RNAi, bacteria are cultured and induced by IPTG separately, and then mixed in equal proportion prior to be used to feed synchronized L1 larvae. F1 embryos were dissected from young adult hermaphrodites, mounted on agarose pads and observed by microscopy as described below.
Measurement of cell-cell junction deformation
Images were acquired using an A1R confocal microscope (Nikon) equipped with 60X oil objective. Cell-cell junction lengths were measured on three-dimension reconstruction of embryos using Vaa3D software.Citation24 The average deformation rate (AD) was obtained by dividing the difference between junction lengths at t0 and tend by the difference between t0 and tend as previously done.Citation7
Quantification of autophagosomes
Images were acquired using an A1R confocal microscope (Nikon) equipped with 60X oil objective and Z-stacks were pre-treated using the “3D Gaussian blur” function of ImageJ set with an X, Y and Z sigma value of 1.0.
For GFP::LGG-1 animals, treated or no by RNAi, images were treated using the “Spot detector” plugin in Icy. In the detector window of this plugin, boxes to display a binary image, force use of 2D Wavelets for 3D, and compute Wat considering ROIs were checked. A sensitivity value of 150 for the scale 2 and 3 was set. Finally, a filtering size threshold to accept object between 10 and 200 pixels were applied, and objects (autophagosomes) were automatically counted.
For GFP::LGG-1; VAB-10(ABD)::mCherry animals, a mask of the dorsal or lateral anterior hypodermal cells was obtained using an intensity threshold of the red channel (ABD::mCh). This mask was applied on the green channel (gfp::lgg-1) and then, green spots were manually counted.
DIC and quantification
Early elongation was recorded as previously described.Citation25 The full length of embryos was measured at the end of early elongation – identified as the moment when body-wall muscles start contracting – from the tip of the mouth of the embryo up to the tip of its tail. Apoptotic cell corpses were identified by their highly refractive appearance under DIC, and the corpses in the head region of embryos were scored at 1.5-fold stage.
Disclosure of potential conflicts of interest
No potential conflicts of interest were disclosed.
References
- Yu L. Recent progress in autophagy. Cell Res. 2014;24:1-2. https://doi.org/10.1038/cr.2014.1 PMID:24384820
- Huang J, Klionsky DJ. Autophagy and human disease. Cell Cycle. 2007;6:1837-49. https://doi.org/10.4161/cc.6.15.4511 PMID:17671424
- Gugnoni M, Sancisi V, Manzotti G, Gandolfi G, Ciarrocchi A. Autophagy and epithelial-mesenchymal transition: An intricate interplay in cancer. Cell Death Dis. 2016;7:e2520. https://doi.org/10.1038/cddis.2016.415 PMID:27929542
- Vuong-Brender TT, Yang X, Labouesse M. C. elegans embryonic morphogenesis. Curr Top Dev Biol. 2016;116:597-616. https://doi.org/10.1016/bs.ctdb.2015.11.012 PMID:26970644
- Gally C, Wissler F, Zahreddine H, Quintin S, Landmann F, Labouesse M. Myosin II regulation during C. elegans embryonic elongation: LET-502/ROCK, MRCK-1 and PAK-1, three kinases with different roles. Development. 2009;136:3109-19. https://doi.org/10.1242/dev.039412 PMID:19675126
- Martin E, Harel S, Nkengfac B, Hamiche K, Neault M, Jenna S. pix-1 controls early elongation in parallel with mel-11 and let-502 in Caenorhabditis elegans. PloS One. 2014;9:e94684. https://doi.org/10.1371/journal.pone.0094684 PMID:24732978
- Martin E, Ouellette MH, Jenna S. Rac1/RhoA antagonism defines cell-to-cell heterogeneity during epidermal morphogenesis in nematodes. J Cell Biol. 2016;215:483-98. https://doi.org/10.1083/jcb.201604015 PMID:27821782
- Piekny AJ, Wissmann A, Mains PE. Embryonic morphogenesis in Caenorhabditis elegans integrates the activity of LET-502 Rho-binding kinase, MEL-11 myosin phosphatase, DAF-2 insulin receptor and FEM-2 PP2c phosphatase. Genetics. 2000;156:1671-89. PMID:11102366
- Li W, Zou W, Yang Y, Chai Y, Chen B, Cheng S, Tian D, Wang X, Vale RD, Ou G. Autophagy genes function sequentially to promote apoptotic cell corpse degradation in the engulfing cell. J Cell Biol. 2012;197:27-35. https://doi.org/10.1083/jcb.201111053 PMID:22451698
- Zou W, Wang X, Vale RD, Ou G. Autophagy genes promote apoptotic cell corpse clearance. Autophagy. 2012;8:1267-8. https://doi.org/10.4161/auto.20786 PMID:22653037
- Borsos E, Erdelyi P, Vellai T. Autophagy and apoptosis are redundantly required for C. elegans embryogenesis. Autophagy. 2011;7:557-9. https://doi.org/10.4161/auto.7.5.14685 PMID:21285529
- Russell RC, Yuan HX, Guan KL. Autophagy regulation by nutrient signaling. Cell Res. 2014;24:42-57. https://doi.org/10.1038/cr.2013.166 PMID:24343578
- Narbonne P, Hyenne V, Li S, Labbe JC, Roy R. Differential requirements for STRAD in LKB1-dependent functions in C. elegans. Development. 2010;137:661-70. https://doi.org/10.1242/dev.042044 PMID:20110331
- Huang S, Jia K, Wang Y, Zhou Z, Levine B. Autophagy genes function in apoptotic cell corpse clearance during C. elegans embryonic development. Autophagy. 2013;9:138-49. https://doi.org/10.4161/auto.22352 PMID:23108454
- Yang P, Zhang H. The coiled-coil domain protein EPG-8 plays an essential role in the autophagy pathway in C. elegans. Autophagy. 2011;7:159-65. https://doi.org/10.4161/auto.7.2.14223 PMID:21116129
- Cheng S, Wu Y, Lu Q, Yan J, Zhang H, Wang X. Autophagy genes coordinate with the class II PI/PtdIns 3-kinase PIKI-1 to regulate apoptotic cell clearance in C. elegans. Autophagy. 2013;9:2022-32. https://doi.org/10.4161/auto.26323 PMID:24165672
- Aguilera MO, Beron W, Colombo MI. The actin cytoskeleton participates in the early events of autophagosome formation upon starvation induced autophagy. Autophagy. 2012;8:1590-603. https://doi.org/10.4161/auto.21459 PMID:22863730
- Miranda L, Carpentier S, Platek A, Hussain N, Gueuning MA, Vertommen D, Ozkan Y, Sid B, Hue L, Courtoy PJ, et al. AMP-activated protein kinase induces actin cytoskeleton reorganization in epithelial cells. Biochem Biophys Res Commun. 2010;396:656-61. https://doi.org/10.1016/j.bbrc.2010.04.151 PMID:20438708
- Gayard M, Guilluy C, Rousselle A, Viollet B, Henrion D, Pacaud P, Loirand G, Rolli-Derkinderen M. AMPK alpha 1-induced RhoA phosphorylation mediates vasoprotective effect of estradiol. Arterioscler Thromb Vasc Biol. 2011;31:2634-42. https://doi.org/10.1161/ATVBAHA.111.228304 PMID:21852563
- Wang S, Liang B, Viollet B, Zou MH. Inhibition of the AMP-activated protein kinase-alpha2 accentuates agonist-induced vascular smooth muscle contraction and high blood pressure in mice. Hypertension. 2011;57:1010-7. https://doi.org/10.1161/HYPERTENSIONAHA.110.168906 PMID:21464390
- Ban BK, Jun MH, Ryu HH, Jang DJ, Ahmad ST, Lee JA. Autophagy negatively regulates early axon growth in cortical neurons. Mol Cell Biol. 2013;33:3907-19. https://doi.org/10.1128/MCB.00627-13 PMID:23918799
- Ames K, Da Cunha DS, Gonzalez B, Konta M, Lin F, Shechter G, Starikov L, Wong S, Bülow HE, Meléndez A. A non-cell-autonomous role of BEC-1/BECN1/Beclin1 in coordinating cell-cycle progression and stem cell proliferation during germline development. Curr Biol. 2017;27:905-13. https://doi.org/10.1016/j.cub.2017.02.015 PMID:28285998
- Kamath RS, Ahringer J. Genome-wide RNAi screening in Caenorhabditis elegans. Methods. 2003;30:313-21. https://doi.org/10.1016/S1046-2023(03)00050-1 PMID:12828945
- Peng H, Ruan Z, Long F, Simpson JH, Myers EW. V3D enables real-time 3D visualization and quantitative analysis of large-scale biological image data sets. Nat Biotechnol. 2010;28:348-53. https://doi.org/10.1038/nbt.1612 PMID:20231818
- Martin E, Rocheleau-Leclair O, Jenna S. Novel metrics to characterize embryonic elongation of the nematode Caenorhabditis elegans. J Vis Exp. 2016:109:e53712. PMID:27077374