ABSTRACT
Rab GTPases are important regulators of intracellular membrane trafficking in eukaryotes. Both activating and inactivating mutations in Rab genes have been identified and implicated in human diseases ranging from neurological disorders to cancer. In addition, altered Rab expression is often associated with disease prognosis. As such, the study of diseases associated with Rabs or Rab-interacting proteins has shed light on the important role of intracellular membrane trafficking in disease etiology. In this review, we cover recent advances in the field with an emphasis on cellular mechanisms.
Introduction
Intracellular membrane trafficking is key to cell metabolism and survival. In order to maintain cellular homeostasis, vesicular transport of membranes and proteins to proper organelles in the cell is essential. In this regard, Rab GTPases are central regulators of vesicular transport, and they represent the largest branch of the Ras-related monomeric GTPase superfamily [Citation1–5]. There are 66 Rab genes in the human genome [Citation6]. Each Rab regulates multiple events at a specific organelle during vesicular transport, including vesicle formation [Citation7–10], vesicle movement along the cytoskeleton [Citation11–21], and vesicle tethering/docking/fusion with a target compartment [Citation22–27] along endocytic and exocytic pathways ().
Figure 1. Subcellular localization of mammalian Rab GTPases and disease implications. Each Rab specifically targets and localizes to a membrane compartment along the exocytic and endocytic pathways in the cell as indicated. At steady state, each Rab may be found on multiple related organelles, based on and references therein. The association of Rab31 with exocrine granules is based on our unpublished immunohistochemistry data showing Rab31 localization to mucin granules of goblet cells in human and mouse intestinal epithelium. Early endosomes, recycling endosomes and secretory vesicles represent heterogeneous populations of vesicles, and the associated Rabs do not necessarily co-localize on the same vesicles. Also indicated are examples of diseases associated with several Rabs.
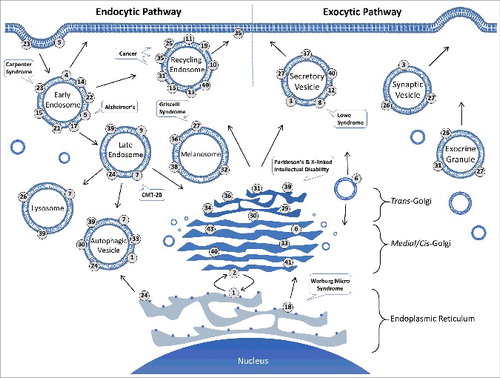
Each Rab is localized to the cytoplasmic surface of a particular membrane compartment or transport vesicle [Citation28–31], and the Rab membrane association requires post-translational isoprenylation (geranylgeranylation) of one or two cysteine residues at or near the C-terminus [Citation32]. Like other GTPases, Rabs function by alternating between active GTP-bound and inactive GDP-bound conformations, with the GTP-bound form interacting with a wide range of effector proteins and promoting multiple steps in vesicular transport. The Rab effectors include cargo proteins to be sorted during vesicle formation, motor proteins for vesicle movement, and tethering factors for vesicle fusion. A single Rab can interact with multiple effectors temporally and spatially. For example, Rab5 has been shown to bind to multiple effectors of different functions in a GTP-dependent fashion in effector pull-down assays, which includes the tethering factor EEA1 and the guanine nucleotide exchange factor (GEF) complex Rabaptin-5/Rabex-5 [Citation33]. The Rab GTPase cycle is facilitated by regulators such as GEFs and GTPase-activating proteins (GAPs) in the cell to accelerate GDP dissociation and GTP hydrolysis [Citation34,Citation35], respectively, since the intrinsic rates are usually very low. The GTPase cycle is coupled with the membrane association and dissociation cycle, which is facilitated by the GDP dissociation inhibitor (GDI) [Citation36,Citation37]. GDI removes inactive GDP-bound Rab from the target membranes and recycles it back to the donor membranes for re-activation and a new round of Rab function in vesicular transport (). While current data are consistent with this model of Rab GTPase cycle, the model should be interpreted with caution in the sense that it is not fully established, especially in the areas of membrane targeting specificity and GDI-mediated recycling in the cell.
Figure 2. Rab GTPase cycle, Rab-interacting proteins and disease implications. Each newly synthesized Rab initially binds to REP-1, which recruits the α and β subunits of the Rab GGTase for post-translational isoprenylation. The prenylated Rab remains complexed with REP-1 and is targeted to the membrane where a cognate GEF facilitates the nucleotide exchange of GDP for GTP and thereby activates the Rab. The GTP-bound Rab can interact with multiple effector proteins temporally and spatially to promote vesicle formation, movement and fusion. Each Rab associates with a particular type of vesicles and interacts with a specific set of effectors, e.g. Rab5 associates with early endosomes and one of its effectors is APPL1 for signaling on endosomes while Rab27 associates with melanosomes and its effectors include Melanophilin and Myosin Va for movement on actin. Upon membrane tethering and docking, the Rab reaches the target membrane to facilitate SNARE-mediated membrane fusion after which a cognate GAP facilitates GTP hydrolysis and converts the Rab to inactive GDP-bound conformation. The GDP-bound Rab is extracted by GDI and delivered back to the donor membrane for a new round of action. Several diseases are known to be associated with the regulators and effectors of the Rab GTPase cycle as indicated.
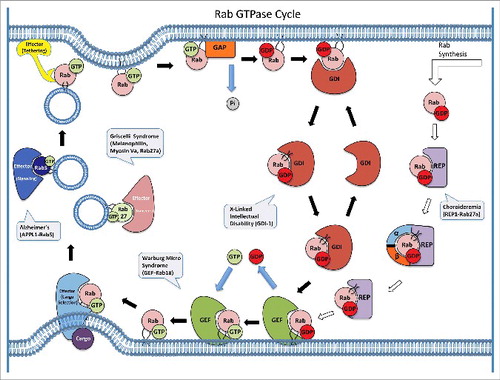
With such a prominent role in membrane trafficking and cell homeostasis, Rabs or Rab-interacting proteins are associated with a multitude of inherited genetic disorders and acquired diseases ranging from neurological diseases to cancer. Depending on the transport pathways, many Rabs have been implicated in various diseases as summarized in and references therein. Here we discuss genetic and acquired diseases associated with Rab mutations or altered expression levels in that order, updating and complementing several previous reviews on the theme [Citation38–40].
Table 1. Rab Functions and Disease Implications.
Charcot-Marie-Tooth Type 2B
Charcot-Marie-Tooth Type 2B (CMT2B) is a hereditary autosomal dominant motor and sensory neuropathy. It represents a subgroup of Charcot-Marie-Tooth (CMT) disease that affects one in every 2,500 individuals and is the most common inherited neurological disease [Citation41]. Phenotypic analyses of CMT2B patients indicate that this disease is an axonal form of peripheral neuropathy characterized by the loss of somatosensory and motor function, which leads to common foot encumbrances such as ulcers and infections with onset of the disease at the age of 20s to 30s [Citation42]. CMT2B is genetically linked to five missense mutations in the RAB7A gene (L129F, K157N, N161I, N161T, V162M) [Citation41,Citation42]. The N161I mutation was the latest addition in 2013 and was the first report of CMT2B in the Asian ethnicity [Citation43]. Because of the later discovery of another Rab7 isoform, Rab7b [Citation44–46], it should be noted that Rab7 in the CMT2B literature refers only to the original isoform (now named Rab7a). Despite the sequence homology and late endosomal localization, Rab7b exhibits different function from Rab7a and promotes transport from late endosomes to the TGN rather than to the lysosome [Citation44,Citation45], which makes it unlikely to compensate for the Rab7a deficiency in CMT2B.
Rab7a localizes to late endosomes and controls late endosome fusion and biogenesis of lysosomes [Citation30,Citation47,Citation48]. Rab7a coordinates with Rab5 in conversion of Rab5-positive early endosomes to Rab7a-positive late endosomes during endosome maturation [Citation49] and has been implicated in multiple cellular functions including apoptosis, neurite growth, and phagocytosis [Citation48]. Interestingly, Rab7a is ubiquitously expressed in all tissues, but the aforementioned Rab7a mutations cause phenotypic consequences only in neuronal cell types, specifically those of the peripheral nervous system [Citation38,Citation50].
Structurally, the five Rab7a missense mutations reside in loops 8 and 10 that coordinate the orientation of guanine base in the bound GTP or GDP molecule [Citation51-53]. Biochemical analyses of these Rab7a missense mutants indicate faster nucleotide exchange rate, leading to constitutive active GTP-bound state due to higher GTP concentration than that of GDP in the cell [Citation51–53]. Along this line, the Rab7a mutants are shown to migrate into the axon and promote premature degradation of neurotrophin receptors, thereby blocking neurotrophin-mediated retrograde signaling from the axon terminal to the soma [Citation54]. With increased Rab7a activity contributing to the phenotypic and morphological attributes of CMT2B, there has been research into the development of Rab7a inhibitors in order to possibly alleviate this neurological disorder. In this regard, one compound (ML282) in the Molecular Libraries Small Molecule Repository (MLSMR) has been found to be proficient inhibitor of Rab7 with higher efficacy towards Rab7a than other Rabs [Citation55]. More recently, however, a loss of Rab7a function appears to be the reason for neurodegeneration in a Drosophila model, and overexpression of the CMT2B Rab7a mutants can actually rescue the neuropathy-like phenotype in a dosage-dependent fashion [Citation56]. This suggests that the CMT2B mutations are loss-of-function mutations rather than gain-of-function mutations as previously thought. If confirmed in humans, a therapeutic approach opposite to the current one would be needed to increase Rab7a function instead.
Warburg Micro syndrome
Warburg Micro syndrome is an autosomal recessive neurological and developmental disorder with phenotypic defects in brain and eye development as well as progressive neurological deterioration in children, which is caused by loss-of-function mutations in Rab18 or the Rab18 GEF (Rab3GAP complex) in about 50% of the cases [Citation57–59]. It is yet to be determined whether the remaining 50% cases of Warburg Micro syndrome are also associated with Rab18 function due to mutations in Rab18 effectors or other factors involved in the Rab18 pathway. While the exact cellular function of Rab18 is unclear, recent studies indicate its localization to the ER and function in maintenance of ER structure [Citation58]. Knockdown of Rab18 by RNAi results in expansion of ER sheets from the perinuclear region towards cell periphery in Hela cells, so does the knockdown of the Rab3GAP complex [Citation58]. Although the Rab3GAP complex was originally discovered and purified for its GAP activity towards Rab3 [Citation60], it was recently found to contain Rab18 GEF activity. This Rab18 GEF activity was abolished by the Rab3GAP mutations (e.g., Rab3GAP1:T18P or E24V and Rab3GAP2:R426C) in Warburg Micro syndrome [Citation58]. Consistently these Rab18 and Rab3GAP mutations produce the same clinical manifestation in brain and eye abnormalities including postnatal microcephaly, developmental delay, congenital bilateral cataracts, microphthalmia and microcornea [Citation57,Citation59].
Carpenter syndrome
Carpenter syndrome is an autosomal recessive neurological disorder of craniosynostosis and limb malformation and is associated with loss-of-function mutations in Rab23 including nonsense and missense mutations [Citation61]. Rab23 is highly expressed in the brain and neurons [Citation62] and is localized to the plasma membrane and early endosomes [Citation63]. Rab23 is shown to regulate the sorting and function of signaling molecules involved in the Sonic Hedgehog signal transduction pathway [Citation64]. Indeed, Rab23 regulates Sonic Hedgehog signaling through controlling the transport and turnover of Hedgehog-associated transmembrane receptor Smoothened in the cilium, which suggests a role in cilia function [Citation65]. Furthermore, the mouse open brain (opb) gene is mapped to Rab23 and blocks Sonic Hedgehog signaling [Citation64]. The opb mutation is a nonsense mutation in Rab23 that leads to premature translation termination and loss of Rab23 function with the phenotype of open neural tube and embryonic lethality [Citation64].
Griscelli Syndrome
Claude Griscelli first described Griscelli Syndrome (GS) in 1978 by characterizing a novel pigment condition paired with immunodeficiency [Citation66]. GS is an autosomal recessive disease characterized by pigment dilution in the hair and skin of patients and by misregulated T lymphocyte and macrophage activation that causes hemophagocytic syndrome [Citation67,Citation68]. The pigment dilution and partial albinism is caused by defective melanosome transport in melanocytes [Citation69,Citation70]. Currently three types of GS are associated with mutations in three different genes, which include MYO5A (GS type 1), RAB27A (GS type 2) and MELANOPHILIN (GS type 3) [Citation71].
It is important to note that Rab27 facilitates melanosome movement and transport along actin cytoskeleton in melanocytes by recruitment of Melanophilin and Myosin Va to the membrane of melanosomes to form a tripartite complex [Citation13,Citation72], which correlates with the mutations of the three genes in the three types of GS. Rab27 has two isoforms (Rab27a and Rab27b) that share 71% amino acid identity. Interestingly, only mutations in the Rab27a gene, which is located on chromosome 15, cause the GS phenotype [Citation73], and these mutations include W73G, L130P, and A152P that inactivate Rab27a and block granule secretion in cytotoxic T lymphocytes [Citation67,Citation68]. In melanocytes, they block melanosome capture by the cortical actin network at the cell periphery and cause melanosome accumulation in the perinuclear region of the cell [Citation69,Citation70,Citation74].
GS is not currently curable. In some cases, hematopoietic stem cell transplantation has been used to rescue the immunodeficiency seen in GS patients, but the defect in melanosome transport remains uncorrected [Citation75]. The ashen mice contain inactivating mutations in Rab27a and constitute an animal model for GS type 2 [Citation73,Citation76]. Transgenic expression of Rab27b in ashen mice can compensate for the Rab27a deficiency and rescue melanosome transport in melanocytes [Citation73], which suggests some degree of functional redundancy between Rab27a and Rab27b and is consistent with the observation that Rab27b is up-regulated in GS type 2 patients [Citation77].
X-linked intellectual disability
X-linked Intellectual Disability (XLID) is a synaptopathy-like disorder caused by mutations in a group of genes on the X chromosome, which mostly encode proteins involved in synaptic plasticity and function [Citation78]. In this regard, one form of XLID is attributed to loss-of-function mutations in Rab39b [Citation79]. Rab39b is expressed in brain neurons and localized to the Golgi complex and endosomes, and inhibition of Rab39b function by shRNA can lead to decreased number of growth cones at the neuronal terminal and synapse formation [Citation79]. Another form of XLID is caused by loss-of-function mutations including both nonsense and missense mutations in a GDI isoform, GDI1 [Citation80,Citation81]. GDI1 encodes GDI-α which is predominantly expressed in the brain. The missense mutant protein (L92P) shows defects in interaction with the synaptic vesicle-associated Rab3a [Citation81,Citation82], and possibly other brain-specific Rabs such as Rab39b, which contributes to the development of XLID.
Choroideremia
Choroideremia (CHM) is an X-linked recessive eye disease that is caused by inactivating mutations in the Rab Escort Protein-1 (REP1) gene [Citation83]. CHM is another example of diseases associated with dysfunction of a Rab regulator or effector, like the aforementioned Melanophilin and Myosin Va in GS. Although CHM is rare only affecting one in 100,000 individuals, this disease develops quickly, and people afflicted exhibit early night blindness by teenage years, which is followed by gradual visual field loss and common blindness by middle age [Citation83]. It is worth noting that CHM is commonly misinterpreted as retinitis pigmentosa or other retinal degeneration diseases, because of similar clinical symptoms [Citation84]. In addition, most females with the mutation are carriers with no symptoms due to the X-linked phylogenetic properties of this gene mutation.
REP1 facilitates the Rab GGTase (geranylgeranyl transferase) reaction by binding to newly synthesized Rab proteins and bringing them into proximity with the catalytic enzyme for post-translational isoprenylation at either one or two cysteine residues located at or near the C terminus of the Rabs [Citation85]. REP1 then delivers the lipid-modified Rabs to each membrane compartment, and the hydrophobicity of the prenyl lipid group anchors the Rabs to the membrane. In CHM, REP1 is non-functional because of inactivating mutations or truncations in the gene sequence [Citation83]. This dysfunction of REP1 causes mislocalization and loss of function of Rab proteins. Through analysis of Rab membrane targeting in lymphocytes, it was discovered that the lack of REP1 expression associated with CHM reduced prenylation of multiple Rabs, and REP2 was shown to compensate for the absence of REP1 and recover the prenylation of several Rabs but not Rab27a [Citation85]. Furthermore, Rab27a is highly expressed in retinal pigment epithelium and choroidal capillaries, in support of the contention that defective Rab27a prenylation is a potential cause of CHM [Citation86]. However, it is worth noting that Rab27a is expressed in multiple tissues and cell types other than retina, e.g., melanocytes and platelets [Citation87], but CHM patients show no clinical defects in these tissues or cell types. Along this line, the aforementioned GS type 2 disease, which is caused by loss-of-function Rab27a mutations, has no known retinal defects suggesting that the REP1 mutations in CHM affects more than Rab27a to induce the retinal degeneration phenotype.
Currently there is no effective treatment for CHM besides symptom management. Recently it has been shown that a significant portion of REP1 non-functional mutations are caused by nonsense mutations, which has led to the development of drugs such as Ataluren (Translarna) to be used as potential counteractive treatments [Citation88]. Retinal gene therapy via recombinant adenoviruses, adeno-associated viruses (AAV) or lentiviruses has also shown promise as another potential avenue for treatment of CHM [Citation89–91]. Importantly, in a 6-patient clinical trial over 6 months, the subretinal delivery of normal REP1 gene via an AAV vector significantly improved the patients' visual acuity and retinal sensitivity [Citation90].
Cancer
Most Rabs show altered expression levels in all cancers except for myeloma based on cancer microarray analysis [Citation92] (). More recently, activating mutations in Rabs have also been identified in human tumor sequencing database, e.g., the A151T and F161L mutations in Rab35 [Citation93]. Like similar mutations in K-Ras, the Rab35 mutants can activate phosphatidylinositol 3-kinase (PI3K) and protein kinase B (AKT) that are essential for cancer cell survival. Along this line, Rab35 is shown to be necessary for growth factor-mediated phosphorylation and activation of AKT in HeLa cells [Citation93]. Rab35 is an endocytic/recycling Rab associated with endosomes and plasma membrane [Citation94,Citation95] and may contribute to growth factor-mediated activation of PI3K/AKT pathway by regulation of endocytosis and recycling, as shown by the endocytosis of PDGFRα driven by the constitutively active Rab35:Q67L mutant [Citation93]. In addition to the activating mutations, increased Rab35 activity can also be achieved by up-regulation of gene expression, which is reported to associate with the onset of ovarian cancer [Citation96]. Interestingly, Rab35 is also shown to interact with p53-related protein kinase (PRPK) in a GTP-dependent manner and suppresses PRPK-induced p53 expression [Citation97]. The p53 protein is a well-known tumor suppressor and down-regulation of p53 is expected to promote tumor cell development by bypassing apoptosis checkpoints.
Altered Rab expression is a common theme in cancer, especially endocytic and recycling Rabs that recycle cell adhesion proteins for cell surface remodeling and are expected to facilitate cell movement and metastasis [Citation10,Citation98–102]. The early endosomal Rabs, such as Rab5 and Rab21, are known to interact with the tails of α subunits of β1 integrins and promote their endocytosis and remodeling at the cell surface, which modulate cell adhesion and migration of breast and prostate cancer cells (MDA-MB-231 and PC3) [Citation10]. Along this line, ablation of Rab5 or Rab21 in carcinoma-associated fibroblasts decreases integrin at the plasma membrane and abrogates the remodeling of extracellular matrix necessary for squamous cell carcinoma invasion [Citation100]. Consistently, Rab5 is over-expressed in highly proliferative and metastatic cancer cells [Citation99,Citation103] while Rab5 activity is suppressed during cell differentiation [Citation104]. Recently, another early endosome-associated Rab (Rab22) was reported to promote microvesicle formation and breast cancer invasion and metastasis and is up-regulated by hypoxia-inducible factors (HIFs), which are often associated with increased risk of metastasis and patient mortality [Citation105]. Along this line, Rab31 (a.k.a. Rab22b) is up-regulated in brain, skin, salivary gland, pancreas, kidney, and gastric cancers but down-regulated in leukemia, lung and colon cancers [Citation38]. Rab31 is highly homologous to Rab22 but targets to TGN instead of early endosomes, and regulates the transport of cargo proteins such as the mannose-6-phosphate receptor (M6PR) to endosomes [Citation106,Citation107] as well as endocytosis of epidermal growth factor receptor (EGFR) [Citation108]. Rab31 overexpression is coupled with unfortunate prognosis in breast cancer patients [Citation109] and is found in estrogen receptor alpha (ERα) positive breast carcinomas [Citation110]. Along with ERα overexpression, MUC1 (mucin 1) which is a heterodimeric transmembrane glycoprotein, is also overexpressed in approximately 90% of breast cancers and forms a complex with ERα that in turn binds to the promoter region of Rab31 and promotes Rab31 expression in an estrogen dependent manner [Citation111,Citation112]. Increased Rab31 levels may up-regulate MUC1 in a positive-feedback loop that influences the prognosis of breast cancers [Citation108].
In addition to endocytic Rabs, exocytic Rabs have also been implicated in cancer progression and malignancy [Citation113,Citation114]. Rab8-containing exocytic vesicles package and deliver matrix metallproteinases (MMP) to the cell surface, and Rab8 is shown to promote MT1-MMP exocytosis, collagen degradation and MDA-MB-231 adenocarcinoma cell invasion [Citation113]. Another exocytic Rab, Rab27B, is also involved in secretion of proinvasive MMPs for degradation of extracellular matrix proteins [Citation114]. In contrast to Rab27A, Rab27B expression level and plasma membrane localization positively correlate with malignant estrogen receptor (ER)-positive breast tumors [Citation114]. Indeed, Rab27B induces G1 to S cell cycle progression and cell proliferation in a lipid modification- and GTP-dependent fashion, and its overexpression increases the invasiveness, tumor size and metastasis of a number of ER-positive breast cancer cell lines both in vitro and in vivo [Citation114].
The same Rab can be up-regulated or down-regulated in different cancers, like the aforementioned Rab31. Rab25 is another well-characterized Rab in this respect and plays a key role in the aggressiveness, prognosis and metastasis of multiple cancer phenotypes including renal cell carcinoma [Citation115], gastric cancer [Citation116], ovarian cancer [Citation117], and lung cancer [Citation118]. Rab25 belongs to the Rab11 subfamily and is also called Rab11c based on sequence homology and is shown to recycle endocytosed membrane proteins [Citation119]. Rab25 can interact with the Rab11 effector Rab coupling protein (RCP) and recycle integrins for plasma membrane remodeling and invasive cell migration [Citation120,Citation121], but its expression is exclusive to the epithelium tissue [Citation122], providing a likely explanation for why Rab25 expression intertwines so closely with epithelium-based cancers. While up-regulated in the aforementioned cancers, interestingly Rab25 is down-regulated in triple-negative breast cancer [Citation123] and colorectal adenocarcinomas [Citation124], which correlates with poor prognosis of patients and displays tumor suppressor property. Whether Rab25 functions as a tumor promoter or a tumor suppressor may be reconciled by the involvement of the chloride intracellular channel protein 3 (CLIC3) in Rab25-mediated integrin recycling and cell invasion [Citation125]. In cell types lacking CLIC3, Rab25 may sort the integrin to lysosomes for degradation and thus inhibit cell invasion behaving like a tumor suppressor, while in cell types with high levels of CLIC3, Rab25 may recycle the integrin to promote cell migration and invasion [Citation125]. In this regard, Rab25 is unique in its GTP-binding motif sequence DTAGLE as opposed to DTAGQE in other Rabs [Citation126], which suggests inhibition of GTP hydrolysis and constitutive activation of Rab25 as a possible mechanism of aggressive metastasis seen in cancers with Rab25 overexpression, similar to the Q61L oncogenic mutation in Ras GTPases.
Alzheimer's Disease
Alzheimer's Disease (AD) is a debilitating form of dementia that affects approximately 5 million new individuals every year and is only treated through symptom management. Increasing evidence has implicated AD as a multifaceted acquired disease caused by multiple factors. In most cases, AD is believed to be initiated by amyloid plaques in the brain tissue that are formed by accumulation of amyloid-beta peptides (Aβ) due to improperly cleaved β-amyloid precursor protein (APP) [Citation127]. The APP gene is located on chromosome 21 and encodes a type 1 integral membrane protein that can be cleaved by proteolytic enzymes (β and γ secretases) upon endocytosis into acidic endosomes to produce Aβ peptides [Citation128–131]. Mutations in these enzymes and the APP gene are shown to have a role in the production of detrimental levels of Aβ associated with AD [Citation127]. In support of the idea of APP processing misregulation in AD pathology, individuals with Down Syndrome (DS) or Trisomy 21 are shown to increase the probability and risk associated with early onset AD, due to an extra copy of the APP gene and elevated levels of APP expression [Citation132,Citation133]. Along this line, in DS mouse models increased expression of APP correlates with decreased retrograde transport of nerve growth factor (NGF) and degeneration of cholinergic neurons [Citation133].
Endosomes are active sites for APP processing in the cell because of the acidic pH favored by the β-secretase, and as a result endosome dysfunction is associated with misregulation of APP processing and AD [Citation134]. In this regard, Rab5 and Rab7 are important regulators of membrane fusion at early and late endosomes, respectively. In AD, the expressions of Rab4, Rab5, Rab7 and Rab27 are up-regulated [Citation135–137] and increased level of Rab5 has been shown to increase APP cleavage and the production of Aβ peptides in fibroblasts, with enlarged endosomes similar to the pathologic endosomal morphology in AD [Citation138]. In DS, a similar enlargement of neuronal endosomes occurs, possibly due to endosomal dysfunction caused by misregulation of APP cleavage and Rab5 activation [Citation132]. In this regard, down-regulation of β−secretase can reduce the production of β-CTF (β−cleaved carboxyl-terminal fragment) as well as the endosomal size [Citation132]. One of the Rab5 effectors APPL1 (adaptor protein, phosphotyrosine interacting with PH domain and leucine zipper 1) that aids in transport of early endosomes containing signaling cargo proteins for gene activation [Citation139–141] can be recruited by β-CTF to endosomes for interaction with and stabilization of Rab5-GTP and up-regulation of Rab5 activity [Citation134], which leads to a positive feedback loop in endosomal dysfunction and Aβ production. Thus, targeting the Rab5 effector protein APPL1 may represent a new therapeutic approach towards stagnation of AD and DS progression.
Conclusion
Rab GTPases are evolutionarily conserved regulators of vesicular transport in all eukaryotic cells. Human cells, in particular, express 66 Rabs some of which are tissue-specific while others show ubiquitous tissue distribution. Both activating and inactivating mutations in Rabs have been identified and implicated in various diseases such as cancer (Rab35 activation), CMT2B (Rab7 activation or inactivation), Warburg Micro syndrome (Rab18 inactivation), Carpenter syndrome (Rab23 inactivation), GS2 (Rab27a inactivation), and XLID (Rab39b inactivation). The investigation of Rab GTPase dysfunction and human diseases has shed light on relative physiological importance of a given Rab and transport pathway in different tissues. For example, the activating Rab7 mutations in CMT2B affect only peripheral neurons, despite its expression in a wide range of tissues and cell types. The mechanism is unclear but it could involve interaction with a tissue-specific effector(s) or represent new Rab function unrelated to its role in membrane transport. This tissue-specific phenotype is also observed in diseases associated with Rab regulators such as CHM, which is caused by mutations in REP-1 that binds Rabs and delivers them to Rab GGTase for prenylation. The Rab GGTase is responsible for post-translational isoprenylation of most Rabs, but the REP-1 mutations in CHM appear to preferentially affect Rab27a and possibly several other Rabs in the retina. In addition to mutations in Rabs and Rab-interacting proteins, increased or decreased Rab expression or activity is associated with devastating diseases like cancer and Alzheimer's. In most cases, however, the cause-effect relationship in the pathogenesis is unclear, which opens new avenues for further research in the field.
Disclosure of potential conflicts of interest
No potential conflicts of interest were disclosed.
Additional information
Funding
References
- Hutagalung AH, Novick PJ. Role of Rab GTPases in membrane traffic and cell physiology. Physiol Rev. 2011;91(1):119–49. https://doi.org/10.1152/physrev.00059.2009
- Li G, Marlin MC. Rab family of GTPases. Methods Mol Biol. 2015;1298:1–15. https://doi.org/10.1007/978-1-4939-2569-8_1
- Pfeffer SR. Rab GTPase localization and Rab cascades in Golgi transport. Biochem Soc Trans. 2012;40(6):1373–7. https://doi.org/10.1042/BST20120168
- Stenmark H. Rab GTPases as coordinators of vesicle traffic. Nat Rev Mol Cell Biol. 2009;10(8):513–25. https://doi.org/10.1038/nrm2728
- Wandinger-Ness A, Zerial M. Rab proteins and the compartmentalization of the endosomal system. Cold Spring Harb Perspect Biol. 2014;6(11):a022616. https://doi.org/10.1101/cshperspect.a022616
- Klopper TH, Kienle N, Fasshauer D, et al. Untangling the evolution of Rab G proteins: implications of a comprehensive genomic analysis. BMC Biol. 2012;10:71. https://doi.org/10.1186/1741-7007-10-71
- Carroll KS, Hanna J, Simon I, et al. Role of Rab9 GTPase in facilitating receptor recruitment by TIP47. Science. 2001;292(5520):1373–6. https://doi.org/10.1126/science.1056791
- McLauchlan H, Newell J, Morrice N, et al. A novel role for Rab5-GDI in ligand sequestration into clathrin-coated pits. Curr Biol. 1998;8(1):34–45. https://doi.org/10.1016/S0960-9822(98)70018-1
- Miserey-Lenkei S, Chalancon G, Bardin S, et al. Rab and actomyosin-dependent fission of transport vesicles at the Golgi complex. Nat Cell Biol. 2010;12(7):645–54. https://doi.org/10.1038/ncb2067
- Pellinen T, Arjonen A, Vuoriluoto K, et al. Small GTPase Rab21 regulates cell adhesion and controls endosomal traffic of beta1-integrins. J Cell Biol. 2006;173(5):767–80. https://doi.org/10.1083/jcb.200509019
- Casavola EC, Catucci A, Bielli P, et al. Ypt32p and Mlc1p bind within the vesicle binding region of the class V myosin Myo2p globular tail domain. Mol Microbiol. 2008;67(5):1051–66. https://doi.org/10.1111/j.1365-2958.2008.06106.x
- Echard A, Jollivet F, Martinez O, et al. Interaction of a Golgi-associated kinesin-like protein with Rab6. Science. 1998;279(5350):580–5. https://doi.org/10.1126/science.279.5350.580
- Fukuda M, Kuroda TS, Mikoshiba K. Slac2-a/melanophilin, the missing link between Rab27 and myosin Va: implications of a tripartite protein complex for melanosome transport. J Biol Chem. 2002;277(14):12432–6. https://doi.org/10.1074/jbc.C200005200
- Govindan B, Bowser R, Novick P. The role of Myo2, a yeast class V myosin, in vesicular transport. J Cell Biol. 1995;128(6):1055–68. https://doi.org/10.1083/jcb.128.6.1055
- Guo X, Farias GG, Mattera R, et al. Rab5 and its effector FHF contribute to neuronal polarity through dynein-dependent retrieval of somatodendritic proteins from the axon. Proc Natl Acad Sci U S A. 2016;113(36):E5318–27. https://doi.org/10.1073/pnas.1601844113
- Hoepfner S, Severin F, Cabezas A, et al. Modulation of receptor recycling and degradation by the endosomal kinesin KIF16B. Cell. 2005;121(3):437–50. https://doi.org/10.1016/j.cell.2005.02.017
- Johansson M, Rocha N, Zwart W, et al. Activation of endosomal dynein motors by stepwise assembly of Rab7-RILP-p150Glued, ORP1L, and the receptor betalll spectrin. J Cell Biol. 2007;176(4):459–71. https://doi.org/10.1083/jcb.200606077
- Jordens I, Fernandez-Borja M, Marsman M, et al. The Rab7 effector protein RILP controls lysosomal transport by inducing the recruitment of dynein-dynactin motors. Curr Biol. 2001;11(21):1680–5. https://doi.org/10.1016/S0960-9822(01)00531-0
- Roland JT, Kenworthy AK, Peranen J, et al. Myosin Vb interacts with Rab8a on a tubular network containing EHD1 and EHD3. Mol Biol Cell. 2007;18(8):2828–37. https://doi.org/10.1091/mbc.E07-02-0169
- Schlager MA, Kapitein LC, Grigoriev I, et al. Pericentrosomal targeting of Rab6 secretory vesicles by Bicaudal-D-related protein 1 (BICDR-1) regulates neuritogenesis. EMBO J. 2010;29(10):1637–51. https://doi.org/10.1038/emboj.2010.51
- Wu XS, Rao K, Zhang H, et al. Identification of an organelle receptor for myosin-Va. Nat Cell Biol. 2002;4(4):271–8. https://doi.org/10.1038/ncb760
- Hickey CM, Wickner W. HOPS initiates vacuole docking by tethering membranes before trans-SNARE complex assembly. Mol Biol Cell. 2010;21(13):2297–305. https://doi.org/10.1091/mbc.E10-01-0044
- Nielsen E, Christoforidis S, Uttenweiler-Joseph S, et al. Rabenosyn-5, a novel Rab5 effector, is complexed with hVPS45 and recruited to endosomes through a FYVE finger domain. J Cell Biol. 2000;151(3):601–12. https://doi.org/10.1083/jcb.151.3.601
- Ohya T, Miaczynska M, Coskun U, et al. Reconstitution of Rab- and SNARE-dependent membrane fusion by synthetic endosomes. Nature. 2009;459(7250):1091–7. https://doi.org/10.1038/nature08107
- Price A, Seals D, Wickner W, et al. The docking stage of yeast vacuole fusion requires the transfer of proteins from a cis-SNARE complex to a Rab/Ypt protein. J Cell Biol. 2000;148(6):1231–8. https://doi.org/10.1083/jcb.148.6.1231
- Simonsen A, Lippe R, Christoforidis S, et al. EEA1 links PI(3)K function to Rab5 regulation of endosome fusion. Nature. 1998;394(6692):494–8. https://doi.org/10.1038/28879
- TerBush DR, Maurice T, Roth D, et al. The Exocyst is a multiprotein complex required for exocytosis in Saccharomyces cerevisiae. EMBO J. 1996;15(23):6483–94.
- Ali BR, Wasmeier C, Lamoreux L, et al. Multiple regions contribute to membrane targeting of Rab GTPases. J Cell Sci. 2004;117(Pt 26):6401–12. https://doi.org/10.1242/jcs.01542
- Blumer J, Rey J, Dehmelt L, et al. RabGEFs are a major determinant for specific Rab membrane targeting. J Cell Biol. 2013;200(3):287–300. https://doi.org/10.1083/jcb.201209113
- Chavrier P, Parton RG, Hauri HP, et al. Localization of low molecular weight GTP binding proteins to exocytic and endocytic compartments. Cell. 1990;62(2):317–29. https://doi.org/10.1016/0092-8674(90)90369-P
- Pfeffer S, Aivazian D. Targeting Rab GTPases to distinct membrane compartments. Nat Rev Mol Cell Biol. 2004;5(11):886–96. https://doi.org/10.1038/nrm1500
- Leung KF, Baron R, Seabra MC. Thematic review series: lipid posttranslational modifications. geranylgeranylation of Rab GTPases. J Lipid Res. 2006;47(3):467–75. https://doi.org/10.1194/jlr.R500017-JLR200
- Christoforidis S, McBride HM, Burgoyne RD, et al. The Rab5 effector EEA1 is a core component of endosome docking. Nature. 1999;397(6720):621–5. https://doi.org/10.1038/17618
- Barr F, Lambright DG. Rab GEFs and GAPs. Curr Opin Cell Biol. 2010;22(4):461–70. https://doi.org/10.1016/j.ceb.2010.04.007
- Novick P. Regulation of membrane traffic by Rab GEF and GAP cascades. Small GTPases. 2016;7(4):252–6. https://doi.org/10.1080/21541248.2016.1213781
- Soldati T, Riederer MA, Pfeffer SR. Rab GDI: a solubilizing and recycling factor for rab9 protein. Mol Biol Cell. 1993;4(4):425–34. https://doi.org/10.1091/mbc.4.4.425
- Ullrich O, Stenmark H, Alexandrov K, et al. Rab GDP dissociation inhibitor as a general regulator for the membrane association of rab proteins. J Biol Chem. 1993;268(24):18143–50.
- Li G. Rab GTPases, membrane trafficking and diseases. Curr Drug Targets. 2011;12(8):1188–93. https://doi.org/10.2174/138945011795906561
- Mitra S, Cheng KW, Mills GB. Rab GTPases implicated in inherited and acquired disorders. Semin Cell Dev Biol. 2011;22(1):57–68. https://doi.org/10.1016/j.semcdb.2010.12.005
- Seixas E, Barros M, Seabra MC, et al. Rab and Arf proteins in genetic diseases. Traffic. 2013;14(8):871–85. https://doi.org/10.1111/tra.12072
- Szigeti K, Lupski JR. Charcot-Marie-Tooth disease. Eur J Hum Genet. 2009;17(6):703–10. https://doi.org/10.1038/ejhg.2009.31
- Ponomareva OY, Eliceiri KW, Halloran MC. Charcot-Marie-Tooth 2b associated Rab7 mutations cause axon growth and guidance defects during vertebrate sensory neuron development. Neural Dev. 2016;11:2. https://doi.org/10.1186/s13064-016-0058-x
- Wang X, Han C, Liu W, et al. A novel RAB7 mutation in a Chinese family with Charcot-Marie-Tooth type 2B disease. Gene. 2014;534(2):431–4. https://doi.org/10.1016/j.gene.2013.10.023
- Bucci C, Bakke O, Progida C. Rab7b and receptors trafficking. Commun Integr Biol. 2010;3(5):401–4. https://doi.org/10.4161/cib.3.5.12341
- Progida C, Cogli L, Piro F, et al. Rab7b controls trafficking from endosomes to the TGN. J Cell Sci. 2010;123(Pt 9):1480–91. https://doi.org/10.1242/jcs.051474
- Yang M, Chen T, Han C, et al. Rab7b, a novel lysosome-associated small GTPase, is involved in monocytic differentiation of human acute promyelocytic leukemia cells. Biochem Biophys Res Commun. 2004;318(3):792–9. https://doi.org/10.1016/j.bbrc.2004.04.115
- Feng Y, Press B, Wandinger-Ness A. Rab 7: an important regulator of late endocytic membrane traffic. J Cell Biol. 1995;131(6 Pt 1):1435–52. https://doi.org/10.1083/jcb.131.6.1435
- Guerra F, Bucci C. Multiple Roles of the Small GTPase Rab7. Cells. 2016;5(3):https://doi.org/10.3390/cells5030034
- Rink J, Ghigo E, Kalaidzidis Y, et al. Rab conversion as a mechanism of progression from early to late endosomes. Cell. 2005;122(5):735–49. https://doi.org/10.1016/j.cell.2005.06.043
- Bucci C, De Luca M. Molecular basis of Charcot-Marie-Tooth type 2B disease. Biochem Soc Trans. 2012;40(6):1368–72. https://doi.org/10.1042/BST20120197
- Cogli L, Progida C, Lecci R, et al. CMT2B-associated Rab7 mutants inhibit neurite outgrowth. Acta Neuropathol. 2010;120(4):491–501. https://doi.org/10.1007/s00401-010-0696-8
- De Luca A, Progida C, Spinosa MR, et al. Characterization of the Rab7K157N mutant protein associated with Charcot-Marie-Tooth type 2B. Biochem Biophys Res Commun. 2008;372(2):283–7. https://doi.org/10.1016/j.bbrc.2008.05.060
- Spinosa MR, Progida C, De Luca A, et al. Functional characterization of Rab7 mutant proteins associated with Charcot-Marie-Tooth type 2B disease. J Neurosci. 2008;28(7):1640–8. https://doi.org/10.1523/JNEUROSCI.3677-07.2008
- Zhang K, Fishel Ben Kenan R, Osakada Y, et al. Defective axonal transport of Rab7 GTPase results in dysregulated trophic signaling. J Neurosci. 2013;33(17):7451–62. https://doi.org/10.1523/JNEUROSCI.4322-12.2013
- Hong L, Simons P, Waller A, et al. A small molecule pan-inhibitor of Ras-superfamily GTPases with high efficacy towards Rab7. Bethesda (MD): Probe Reports from the NIH Molecular Libraries Program; 2010.
- Cherry S, Jin EJ, Ozel MN, et al. Charcot-Marie-Tooth 2B mutations in rab7 cause dosage-dependent neurodegeneration due to partial loss of function. Elife. 2013;2:e01064. https://doi.org/10.7554/eLife.01064
- Bem D, Yoshimura S, Nunes-Bastos R, et al. Loss-of-function mutations in RAB18 cause Warburg micro syndrome. Am J Hum Genet. 2011;88(4):499–507. https://doi.org/10.1016/j.ajhg.2011.03.012
- Gerondopoulos A, Bastos RN, Yoshimura S, et al. Rab18 and a Rab18 GEF complex are required for normal ER structure. J Cell Biol. 2014;205(5):707–20. https://doi.org/10.1083/jcb.201403026
- Handley MT, Morris-Rosendahl DJ, Brown S, et al. Mutation spectrum in RAB3GAP1, RAB3GAP2, and RAB18 and genotype-phenotype correlations in warburg micro syndrome and Martsolf syndrome. Hum Mutat. 2013;34(5):686–96. https://doi.org/10.1002/humu.22296
- Fukui K, Sasaki T, Imazumi K, et al. Isolation and characterization of a GTPase activating protein specific for the Rab3 subfamily of small G proteins. J Biol Chem. 1997;272(8):4655–8. https://doi.org/10.1074/jbc.272.8.4655
- Jenkins D, Seelow D, Jehee FS, et al. RAB23 mutations in Carpenter syndrome imply an unexpected role for hedgehog signaling in cranial-suture development and obesity. Am J Hum Genet. 2007;80(6):1162–70. https://doi.org/10.1086/518047
- Olkkonen VM, Peterson JR, Dupree P, et al. Isolation of a mouse cDNA encoding Rab23, a small novel GTPase expressed predominantly in the brain. Gene. 1994;138(1-2):207–11. https://doi.org/10.1016/0378-1119(94)90809-5
- Evans TM, Ferguson C, Wainwright BJ, et al. Rab23, a negative regulator of hedgehog signaling, localizes to the plasma membrane and the endocytic pathway. Traffic. 2003;4(12):869–84. https://doi.org/10.1046/j.1600-0854.2003.00141.x
- Eggenschwiler JT, Espinoza E, Anderson KV. Rab23 is an essential negative regulator of the mouse Sonic hedgehog signalling pathway. Nature. 2001;412(6843):194–8. https://doi.org/10.1038/35084089
- Boehlke C, Bashkurov M, Buescher A, et al. Differential role of Rab proteins in ciliary trafficking: Rab23 regulates smoothened levels. J Cell Sci. 2010;123(Pt 9):1460–7. https://doi.org/10.1242/jcs.058883
- Griscelli C, Prunieras M. Pigment dilution and immunodeficiency: a new syndrome. Int J Dermatol. 1978;17(10):788–91. https://doi.org/10.1111/j.1365-4362.1978.tb05980.x
- Menasche G, Pastural E, Feldmann J, et al. Mutations in RAB27A cause Griscelli syndrome associated with haemophagocytic syndrome. Nat Genet. 2000;25(2):173–6. https://doi.org/10.1038/76024
- Stinchcombe JC, Barral DC, Mules EH, et al. Rab27a is required for regulated secretion in cytotoxic T lymphocytes. J Cell Biol. 2001;152(4):825–34. https://doi.org/10.1083/jcb.152.4.825
- Bahadoran P, Aberdam E, Mantoux F, et al. Rab27a: A key to melanosome transport in human melanocytes. J Cell Biol. 2001;152(4):843–50. https://doi.org/10.1083/jcb.152.4.843
- Hume AN, Collinson LM, Rapak A, et al. Rab27a regulates the peripheral distribution of melanosomes in melanocytes. J Cell Biol. 2001;152(4):795–808. https://doi.org/10.1083/jcb.152.4.795
- Van Gele M, Dynoodt P, Lambert J. Griscelli syndrome: a model system to study vesicular trafficking. Pigment Cell Melanoma Res. 2009;22(3):268–82. https://doi.org/10.1111/j.1755-148X.2009.00558.x
- Wu X, Wang F, Rao K, et al. Rab27a is an essential component of melanosome receptor for myosin Va. Mol Biol Cell. 2002;13(5):1735–49. https://doi.org/10.1091/mbc.01-12-0595
- Barral DC, Ramalho JS, Anders R, et al. Functional redundancy of Rab27 proteins and the pathogenesis of Griscelli syndrome. J Clin Invest. 2002;110(2):247–57. https://doi.org/10.1172/JCI0215058
- Wu X, Bowers B, Rao K, et al. Visualization of melanosome dynamics within wild-type and dilute melanocytes suggests a paradigm for myosin V function In vivo. J Cell Biol. 1998;143(7):1899–918. https://doi.org/10.1083/jcb.143.7.1899
- Lipsker D. Haemophagocytic lymphohistiocytosis and silvery hair in Griscelli syndrome. Br J Haematol. 2016;175(1):11. https://doi.org/10.1111/bjh.14252
- Wilson SM, Yip R, Swing DA, et al. A mutation in Rab27a causes the vesicle transport defects observed in ashen mice. Proc Natl Acad Sci U S A. 2000;97(14):7933–8. https://doi.org/10.1073/pnas.140212797
- Westbroek W, Lambert J, De Schepper S, et al. Rab27b is up-regulated in human Griscelli syndrome type II melanocytes and linked to the actin cytoskeleton via exon F-Myosin Va transcripts. Pigment Cell Res. 2004;17(5):498–505. https://doi.org/10.1111/j.1600-0749.2004.00173.x
- Boda B, Mas C, Muller D. Activity-dependent regulation of genes implicated in X-linked non-specific mental retardation. Neuroscience. 2002;114(1):13–7. https://doi.org/10.1016/S0306-4522(02)00218-X
- Giannandrea M, Bianchi V, Mignogna ML, et al. Mutations in the small GTPase gene RAB39B are responsible for X-linked mental retardation associated with autism, epilepsy, and macrocephaly. Am J Hum Genet. 2010;86(2):185–95. https://doi.org/10.1016/j.ajhg.2010.01.011
- Bienvenu T, des Portes V, Saint Martin A, et al. Non-specific X-linked semidominant mental retardation by mutations in a Rab GDP-dissociation inhibitor. Hum Mol Genet. 1998;7(8):1311–5. https://doi.org/10.1093/hmg/7.8.1311
- D'Adamo P, Menegon A, Lo Nigro C, et al. Mutations in GDI1 are responsible for X-linked non-specific mental retardation. Nat Genet. 1998;19(2):134–9. https://doi.org/10.1038/487
- Sasaki T, Kikuchi A, Araki S, et al. Purification and characterization from bovine brain cytosol of a protein that inhibits the dissociation of GDP from and the subsequent binding of GTP to smg p25A, a ras p21-like GTP-binding protein. J Biol Chem. 1990;265(4):2333–7.
- van den Hurk JA, Schwartz M, van Bokhoven H, et al. Molecular basis of choroideremia (CHM): mutations involving the Rab escort protein-1 (REP-1) gene. Hum Mutat. 1997;9(2):110–7. https://doi.org/10.1002/(SICI)1098-1004(1997)9:2%3c110::AID-HUMU2%3e3.0.CO;2-D
- Lee TK, McTaggart KE, Sieving PA, et al. Clinical diagnoses that overlap with choroideremia. Can J Ophthalmol. 2003;38(5):364–72. quiz 72. https://doi.org/10.1016/S0008-4182(03)80047-9
- Pereira-Leal JB, Hume AN, Seabra MC. Prenylation of Rab GTPases: molecular mechanisms and involvement in genetic disease. FEBS Lett. 2001;498(2-3):197–200. https://doi.org/10.1016/S0014-5793(01)02483-8
- Seabra MC, Ho YK, Anant JS. Deficient geranylgeranylation of Ram/Rab27 in choroideremia. J Biol Chem. 1995;270(41):24420–7. https://doi.org/10.1074/jbc.270.41.24420
- Chen D, Guo J, Miki T, et al. Molecular cloning and characterization of rab27a and rab27b, novel human rab proteins shared by melanocytes and platelets. Biochem Mol Med. 1997;60(1):27–37. https://doi.org/10.1006/bmme.1996.2559
- Moosajee M, Tracey-White D, Smart M, et al. Functional rescue of REP1 following treatment with PTC124 and novel derivative PTC-414 in human choroideremia fibroblasts and the nonsense-mediated zebrafish model. Hum Mol Genet. 2016;https://doi.org/10.1093/hmg/ddw184
- Anand V, Barral DC, Zeng Y, et al. Gene therapy for choroideremia: in vitro rescue mediated by recombinant adenovirus. Vision Res. 2003;43(8):919–26. https://doi.org/10.1016/S0042-6989(02)00389-9
- MacLaren RE, Groppe M, Barnard AR, et al. Retinal gene therapy in patients with choroideremia: initial findings from a phase 1/2 clinical trial. Lancet. 2014;383(9923):1129–37. https://doi.org/10.1016/S0140-6736(13)62117-0
- Tolmachova T, Tolmachov OE, Wavre-Shapton ST, et al. CHM/REP1 cDNA delivery by lentiviral vectors provides functional expression of the transgene in the retinal pigment epithelium of choroideremia mice. J Gene Med. 2012;14(3):158–68. https://doi.org/10.1002/jgm.1652
- Rodrigues ML, Pereira-Leal JB. Novel Rab GTPases. In: Li G, Segev N, editors. Rab GTPases and Membrane Trafficking. Bentham Science Publishers; 2012. p. 155–68.
- Wheeler DB, Zoncu R, Root DE, et al. Identification of an oncogenic RAB protein. Science. 2015;350(6257):211–7. https://doi.org/10.1126/science.aaa4903
- Klinkert K, Echard A. Rab35 GTPase: A Central Regulator of Phosphoinositides and F-actin in Endocytic Recycling and Beyond. Traffic. 2016;17(10):1063–77. https://doi.org/10.1111/tra.12422
- Zhu AX, Zhao Y, Flier JS. Molecular cloning of two small GTP-binding proteins from human skeletal muscle. Biochem Biophys Res Commun. 1994;205(3):1875–82. https://doi.org/10.1006/bbrc.1994.2889
- Sheach LA, Adeney EM, Kucukmetin A, et al. Androgen-related expression of G-proteins in ovarian cancer. Br J Cancer. 2009;101(3):498–503. https://doi.org/10.1038/sj.bjc.6605153
- Abe Y, Takeuchi T, Imai Y, et al. A Small Ras-like protein Ray/Rab1c modulates the p53-regulating activity of PRPK. Biochem Biophys Res Commun. 2006;344(1):377–85. https://doi.org/10.1016/j.bbrc.2006.03.071
- Cheng KW, Lahad JP, Kuo WL, et al. The RAB25 small GTPase determines aggressiveness of ovarian and breast cancers. Nat Med. 2004;10(11):1251–6. https://doi.org/10.1038/nm1125
- Fukui K, Tamura S, Wada A, et al. Expression of Rab5a in hepatocellular carcinoma: Possible involvement in epidermal growth factor signaling. Hepatol Res. 2007;37(11):957–65. https://doi.org/10.1111/j.1872-034X.2007.00143.x
- Hooper S, Gaggioli C, Sahai E. A chemical biology screen reveals a role for Rab21-mediated control of actomyosin contractility in fibroblast-driven cancer invasion. Br J Cancer. 2010;102(2):392–402. https://doi.org/10.1038/sj.bjc.6605469
- Hou Q, Wu YH, Grabsch H, et al. Integrative genomics identifies RAB23 as an invasion mediator gene in diffuse-type gastric cancer. Cancer Res. 2008;68(12):4623–30. https://doi.org/10.1158/0008-5472.CAN-07-5870
- Kanda I, Nishimura N, Nakatsuji H, et al. Involvement of Rab13 and JRAB/MICAL-L2 in epithelial cell scattering. Oncogene. 2008;27(12):1687–95. https://doi.org/10.1038/sj.onc.1210812
- Li Y, Meng X, Feng H, et al. Over-expression of the RAB5 gene in human lung adenocarcinoma cells with high metastatic potential. Chin Med Sci J. 1999;14(2):96–101.
- Liu J, Lamb D, Chou MM, et al. Nerve growth factor-mediated neurite outgrowth via regulation of Rab5. Mol Biol Cell. 2007;18(4):1375–84. https://doi.org/10.1091/mbc.E06-08-0725
- Wang T, Gilkes DM, Takano N, et al. Hypoxia-inducible factors and RAB22A mediate formation of microvesicles that stimulate breast cancer invasion and metastasis. Proc Natl Acad Sci U S A. 2014;111(31):E3234–42. https://doi.org/10.1073/pnas.1410041111
- Ng EL, Wang Y, Tang BL. Rab22B's role in trans-Golgi network membrane dynamics. Biochem Biophys Res Commun. 2007;361(3):751–7. https://doi.org/10.1016/j.bbrc.2007.07.076
- Rodriguez-Gabin AG, Yin X, Si Q, et al. Transport of mannose-6-phosphate receptors from the trans-Golgi network to endosomes requires Rab31. Exp Cell Res. 2009;315(13):2215–30. https://doi.org/10.1016/j.yexcr.2009.03.020
- Chua CE, Tang BL. The role of the small GTPase Rab31 in cancer. J Cell Mol Med. 2015;19(1):1–10. https://doi.org/10.1111/jcmm.12403
- Grismayer B, Solch S, Seubert B, et al. Rab31 expression levels modulate tumor-relevant characteristics of breast cancer cells. Mol Cancer. 2012;11:62. https://doi.org/10.1186/1476-4598-11-62
- Abba MC, Hu Y, Sun H, et al. Gene expression signature of estrogen receptor alpha status in breast cancer. BMC Genomics. 2005;6:37. https://doi.org/10.1186/1471-2164-6-37
- Jin C, Rajabi H, Pitroda S, et al. Cooperative interaction between the MUC1-C oncoprotein and the Rab31 GTPase in estrogen receptor-positive breast cancer cells. PLoS One. 2012;7(7):e39432. https://doi.org/10.1371/journal.pone.0039432
- Wei X, Xu H, Kufe D. MUC1 oncoprotein stabilizes and activates estrogen receptor alpha. Mol Cell. 2006;21(2):295–305. https://doi.org/10.1016/j.molcel.2005.11.030
- Bravo-Cordero JJ, Marrero-Diaz R, Megias D, et al. MT1-MMP proinvasive activity is regulated by a novel Rab8-dependent exocytic pathway. EMBO J. 2007;26(6):1499–510. https://doi.org/10.1038/sj.emboj.7601606
- Hendrix A, Maynard D, Pauwels P, et al. Effect of the secretory small GTPase Rab27B on breast cancer growth, invasion, and metastasis. J Natl Cancer Inst. 2010;102(12):866–80. https://doi.org/10.1093/jnci/djq153
- Li Y, Jia Q, Zhang Q, et al. Rab25 upregulation correlates with the proliferation, migration, and invasion of renal cell carcinoma. Biochem Biophys Res Commun. 2015;458(4):745–50. https://doi.org/10.1016/j.bbrc.2015.01.144
- Cao C, Lu C, Xu J, et al. Expression of Rab25 correlates with the invasion and metastasis of gastric cancer. Chin J Cancer Res. 2013;25(2):192–9.
- Gomez-Roman N, Sahasrabudhe NM, McGregor F, et al. Hypoxia-inducible factor 1 alpha is required for the tumourigenic and aggressive phenotype associated with Rab25 expression in ovarian cancer. Oncotarget. 2016;7(16):22650–64. https://doi.org/10.18632/oncotarget.7998
- Ma YF, Yang B, Li J, et al. Expression of Ras-related protein 25 predicts chemotherapy resistance and prognosis in advanced non-small cell lung cancer. Genet Mol Res. 2015;14(4):13998–4008. https://doi.org/10.4238/2015.October.29.19
- Casanova JE, Wang X, Kumar R, et al. Association of Rab25 and Rab11a with the apical recycling system of polarized Madin-Darby canine kidney cells. Mol Biol Cell. 1999;10(1):47–61. https://doi.org/10.1091/mbc.10.1.47
- Caswell PT, Chan M, Lindsay AJ, et al. Rab-coupling protein coordinates recycling of alpha5beta1 integrin and EGFR1 to promote cell migration in 3D microenvironments. J Cell Biol. 2008;183(1):143–55. https://doi.org/10.1083/jcb.200804140
- Caswell PT, Spence HJ, Parsons M, et al. Rab25 associates with alpha5beta1 integrin to promote invasive migration in 3D microenvironments. Dev Cell. 2007;13(4):496–510. https://doi.org/10.1016/j.devcel.2007.08.012
- Goldenring JR, Shen KR, Vaughan HD, et al. Identification of a small GTP-binding protein, Rab25, expressed in the gastrointestinal mucosa, kidney, and lung. J Biol Chem. 1993;268(25):18419–22.
- Cheng JM, Volk L, Janaki DK, et al. Tumor suppressor function of Rab25 in triple-negative breast cancer. Int J Cancer. 2010;126(12):2799–812.
- Nam KT, Lee HJ, Smith JJ, et al. Loss of Rab25 promotes the development of intestinal neoplasia in mice and is associated with human colorectal adenocarcinomas. J Clin Invest. 2010;120(3):840–9. https://doi.org/10.1172/JCI40728
- Dozynkiewicz MA, Jamieson NB, Macpherson I, et al. Rab25 and CLIC3 collaborate to promote integrin recycling from late endosomes/lysosomes and drive cancer progression. Dev Cell. 2012;22(1):131–45. https://doi.org/10.1016/j.devcel.2011.11.008
- Lall P, Horgan CP, Oda S, et al. Structural and functional analysis of FIP2 binding to the endosome-localised Rab25 GTPase. Biochim Biophys Acta. 2013;1834(12):2679–90. https://doi.org/10.1016/j.bbapap.2013.09.005
- Krstic D, Knuesel I. Deciphering the mechanism underlying late-onset Alzheimer disease. Nat Rev Neurol. 2013;9(1):25–34. https://doi.org/10.1038/nrneurol.2012.236
- Kimberly WT, Esler WP, Ye W, et al. Notch and the amyloid precursor protein are cleaved by similar gamma-secretase(s). Biochemistry. 2003;42(1):137–44. https://doi.org/10.1021/bi026888g
- Lin X, Koelsch G, Wu S, et al. Human aspartic protease memapsin 2 cleaves the beta-secretase site of beta-amyloid precursor protein. Proc Natl Acad Sci U S A. 2000;97(4):1456–60. https://doi.org/10.1073/pnas.97.4.1456
- Sinha S, Anderson JP, Barbour R, et al. Purification and cloning of amyloid precursor protein beta-secretase from human brain. Nature. 1999;402(6761):537–40. https://doi.org/10.1038/990114
- Vassar R, Bennett BD, Babu-Khan S, et al. Beta-secretase cleavage of Alzheimer's amyloid precursor protein by the transmembrane aspartic protease BACE. Science. 1999;286(5440):735–41. https://doi.org/10.1126/science.286.5440.735
- Jiang Y, Mullaney KA, Peterhoff CM, et al. Alzheimer's-related endosome dysfunction in Down syndrome is Abeta-independent but requires APP and is reversed by BACE-1 inhibition. Proc Natl Acad Sci U S A. 2010;107(4):1630–5. https://doi.org/10.1073/pnas.0908953107
- Salehi A, Delcroix JD, Belichenko PV, et al. Increased App expression in a mouse model of Down's syndrome disrupts NGF transport and causes cholinergic neuron degeneration. Neuron. 2006;51(1):29–42. https://doi.org/10.1016/j.neuron.2006.05.022
- Kim S, Sato Y, Mohan PS, et al. Evidence that the rab5 effector APPL1 mediates APP-betaCTF-induced dysfunction of endosomes in Down syndrome and Alzheimer's disease. Mol Psychiatry. 2016;21(5):707–16. https://doi.org/10.1038/mp.2015.97
- Cataldo AM, Peterhoff CM, Troncoso JC, et al. Endocytic pathway abnormalities precede amyloid beta deposition in sporadic Alzheimer's disease and Down syndrome: differential effects of APOE genotype and presenilin mutations. Am J Pathol. 2000;157(1):277–86. https://doi.org/10.1016/S0002-9440(10)64538-5
- Ginsberg SD, Mufson EJ, Alldred MJ, et al. Upregulation of select rab GTPases in cholinergic basal forebrain neurons in mild cognitive impairment and Alzheimer's disease. J Chem Neuroanat. 2011;42(2):102–10. https://doi.org/10.1016/j.jchemneu.2011.05.012
- Ginsberg SD, Mufson EJ, Counts SE, et al. Regional selectivity of rab5 and rab7 protein upregulation in mild cognitive impairment and Alzheimer's disease. J Alzheimers Dis. 2010;22(2):631–9. https://doi.org/10.3233/JAD-2010-101080
- Grbovic OM, Mathews PM, Jiang Y, et al. Rab5-stimulated up-regulation of the endocytic pathway increases intracellular beta-cleaved amyloid precursor protein carboxyl-terminal fragment levels and Abeta production. J Biol Chem. 2003;278(33):31261–8. https://doi.org/10.1074/jbc.M304122200
- Kalaidzidis I, Miaczynska M, Brewinska-Olchowik M, et al. APPL endosomes are not obligatory endocytic intermediates but act as stable cargo-sorting compartments. J Cell Biol. 2015;211(1):123–44. https://doi.org/10.1083/jcb.201311117
- Miaczynska M, Christoforidis S, Giner A, et al. APPL proteins link Rab5 to nuclear signal transduction via an endosomal compartment. Cell. 2004;116(3):445–56. https://doi.org/10.1016/S0092-8674(04)00117-5
- Schenck A, Goto-Silva L, Collinet C, et al. The endosomal protein Appl1 mediates Akt substrate specificity and cell survival in vertebrate development. Cell. 2008;133(3):486–97. https://doi.org/10.1016/j.cell.2008.02.044
- Bacon RA, Salminen A, Ruohola H, et al. The GTP-binding protein Ypt1 is required for transport in vitro: the Golgi apparatus is defective in ypt1 mutants. J Cell Biol. 1989;109(3):1015–22. https://doi.org/10.1083/jcb.109.3.1015
- Cooper AA, Gitler AD, Cashikar A, et al. Alpha-synuclein blocks ER-Golgi traffic and Rab1 rescues neuron loss in Parkinson's models. Science. 2006;313(5785):324–8. https://doi.org/10.1126/science.1129462
- Coune PG, Bensadoun JC, Aebischer P, et al. Rab1A over-expression prevents Golgi apparatus fragmentation and partially corrects motor deficits in an alpha-synuclein based rat model of Parkinson's disease. J Parkinsons Dis. 2011;1(4):373–87.
- Nikoshkov A, Broliden K, Attarha S, et al. Expression pattern of the PRDX2, RAB1A, RAB1B, RAB5A and RAB25 genes in normal and cancer cervical tissues. Int J Oncol. 2015;46(1):107–12. https://doi.org/10.3892/ijo.2014.2724
- Quan Y, Song Q, Wang J, et al. MiR-1202 functions as a tumor suppressor in glioma cells by targeting Rab1A. Tumour Biol. 2017;39(4):1010428317697565. https://doi.org/10.1177/1010428317697565
- Sannerud R, Marie M, Nizak C, et al. Rab1 defines a novel pathway connecting the pre-Golgi intermediate compartment with the cell periphery. Mol Biol Cell. 2006;17(4):1514–26. https://doi.org/10.1091/mbc.E05-08-0792
- Saraste J, Lahtinen U, Goud B. Localization of the small GTP-binding protein rab1p to early compartments of the secretory pathway. J Cell Sci. 1995;108(Pt 4):1541–52.
- Segev N, Mulholland J, Botstein D. The yeast GTP-binding YPT1 protein and a mammalian counterpart are associated with the secretion machinery. Cell. 1988;52(6):915–24. https://doi.org/10.1016/0092-8674(88)90433-3
- Shimada K, Uzawa K, Kato M, et al. Aberrant expression of RAB1A in human tongue cancer. Br J Cancer. 2005;92(10):1915–21. https://doi.org/10.1038/sj.bjc.6602594
- Thomas JD, Zhang YJ, Wei YH, et al. Rab1A is an mTORC1 activator and a colorectal oncogene. Cancer Cell. 2014;26(5):754–69. https://doi.org/10.1016/j.ccell.2014.09.008
- Tisdale EJ, Bourne JR, Khosravi-Far R, et al. GTP-binding mutants of rab1 and rab2 are potent inhibitors of vesicular transport from the endoplasmic reticulum to the Golgi complex. J Cell Biol. 1992;119(4):749–61. https://doi.org/10.1083/jcb.119.4.749
- Wang X, Liu F, Qin X, et al. Expression of Rab1A is upregulated in human lung cancer and associated with tumor size and T stage. Aging (Albany NY). 2016;8(11):2790–8. https://doi.org/10.18632/aging.101087
- Webster CP, Smith EF, Bauer CS, et al. The C9orf72 protein interacts with Rab1a and the ULK1 complex to regulate initiation of autophagy. EMBO J. 2016;35(15):1656–76. https://doi.org/10.15252/embj.201694401
- Wilson BS, Nuoffer C, Meinkoth JL, et al. A Rab1 mutant affecting guanine nucleotide exchange promotes disassembly of the Golgi apparatus. J Cell Biol. 1994;125(3):557–71. https://doi.org/10.1083/jcb.125.3.557
- Winslow AR, Chen CW, Corrochano S, et al. alpha-Synuclein impairs macroautophagy: implications for Parkinson's disease. J Cell Biol. 2010;190(6):1023–37. https://doi.org/10.1083/jcb.201003122
- Wu G, Yussman MG, Barrett TJ, et al. Increased myocardial Rab GTPase expression: a consequence and cause of cardiomyopathy. Circ Res. 2001;89(12):1130–7. https://doi.org/10.1161/hh2401.100427
- Xu H, Qian M, Zhao B, et al. Inhibition of RAB1A suppresses epithelial-mesenchymal transition and proliferation of triple-negative breast cancer cells. Oncol Rep. 2017;37(3):1619–26. https://doi.org/10.3892/or.2017.5404
- Zenner HL, Yoshimura S, Barr FA, et al. Analysis of Rab GTPase-activating proteins indicates that Rab1a/b and Rab43 are important for herpes simplex virus 1 secondary envelopment. J Virol. 2011;85(16):8012–21. https://doi.org/10.1128/JVI.00500-11
- Connor MG, Pulsifer AR, Price CT, et al. Yersinia pestis Requires Host Rab1b for Survival in Macrophages. PLoS Pathog. 2015;11(10):e1005241. https://doi.org/10.1371/journal.ppat.1005241
- Dugan JM, deWit C, McConlogue L, et al. The Ras-related GTP-binding protein, Rab1B, regulates early steps in exocytic transport and processing of beta-amyloid precursor protein. J Biol Chem. 1995;270(18):10982–9. https://doi.org/10.1074/jbc.270.18.10982
- Halberg N, Sengelaub CA, Navrazhina K, et al. PITPNC1 Recruits RAB1B to the Golgi Network to Drive Malignant Secretion. Cancer Cell. 2016;29(3):339–53. https://doi.org/10.1016/j.ccell.2016.02.013
- He H, Dai F, Yu L, et al. Identification and characterization of nine novel human small GTPases showing variable expressions in liver cancer tissues. Gene Expr. 2002;10(5-6):231–42. https://doi.org/10.3727/000000002783992406
- Jiang HL, Sun HF, Gao SP, et al. Loss of RAB1B promotes triple-negative breast cancer metastasis by activating TGF-beta/SMAD signaling. Oncotarget. 2015;6(18):16352–65. https://doi.org/10.18632/oncotarget.3877
- Kakuta S, Yamaguchi J, Suzuki C, et al. Small GTPase Rab1B is associated with ATG9A vesicles and regulates autophagosome formation. FASEB J. 2017;31(9):3757–73. https://org/10.1096/fj.201601052
- Mihai Gazdag E, Streller A, Haneburger I, et al. Mechanism of Rab1b deactivation by the Legionella pneumophila GAP LepB. EMBO Rep. 2013;14(2):199–205. https://doi.org/10.1038/embor.2012.211
- Mochizuki Y, Ohashi R, Kawamura T, et al. Phosphatidylinositol 3-phosphatase myotubularin-related protein 6 (MTMR6) is regulated by small GTPase Rab1B in the early secretory and autophagic pathways. J Biol Chem. 2013;288(2):1009–21. https://doi.org/10.1074/jbc.M112.395087
- Muller MP, Peters H, Blumer J, et al. The Legionella effector protein DrrA AMPylates the membrane traffic regulator Rab1b. Science. 2010;329(5994):946–9. https://doi.org/10.1126/science.1192276
- Yadav V, Panganiban AT, Honer Zu Bentrup K, et al. Influenza infection modulates vesicular trafficking and induces Golgi complex disruption. Virusdisease. 2016;27(4):357–68. https://doi.org/10.1007/s13337-016-0347-3
- Yamayoshi S, Neumann G, Kawaoka Y. Role of the GTPase Rab1b in ebolavirus particle formation. J Virol. 2010;84(9):4816–20. https://doi.org/10.1128/JVI.00010-10
- Yang XZ, Cui SZ, Zeng LS, et al. Overexpression of Rab1B and MMP9 predicts poor survival and good response to chemotherapy in patients with colorectal cancer. Aging (Albany NY). 2017;9(3):914–31.
- Dey KK, Pal I, Bharti R, et al. Identification of RAB2A and PRDX1 as the potential biomarkers for oral squamous cell carcinoma using mass spectrometry-based comparative proteomic approach. Tumour Biol. 2015;36(12):9829–37. https://doi.org/10.1007/s13277-015-3758-7
- Fujita N, Huang W, Lin TH, et al. Genetic screen in Drosophila muscle identifies autophagy-mediated T-tubule remodeling and a Rab2 role in autophagy. Elife. 2017;6:https://doi.org/10.7554/eLife.23367
- Lomnytska MI, Becker S, Hellman K, et al. Diagnostic protein marker patterns in squamous cervical cancer. Proteomics Clin Appl. 2010;4(1):17–31. https://doi.org/10.1002/prca.200900086
- Lorincz P, Toth S, Benko P, et al. Rab2 promotes autophagic and endocytic lysosomal degradation. J Cell Biol. 2017;216(7):1937–47. https://doi.org/10.1083/jcb.201611027
- Ortiz Sandoval C, Simmen T. Rab proteins of the endoplasmic reticulum: functions and interactors. Biochem Soc Trans. 2012;40(6):1426–32. https://doi.org/10.1042/BST20120158
- Saraste J. Spatial and Functional Aspects of ER-Golgi Rabs and Tethers. Front Cell Dev Biol. 2016;4:28. https://doi.org/10.3389/fcell.2016.00028
- Tisdale EJ, Jackson MR. Rab2 protein enhances coatomer recruitment to pre-Golgi intermediates. J Biol Chem. 1998;273(27):17269–77. https://doi.org/10.1074/jbc.273.27.17269
- White JA, 2nd, Anderson E, Zimmerman K, et al. Huntingtin differentially regulates the axonal transport of a sub-set of Rab-containing vesicles in vivo. Hum Mol Genet. 2015;24(25):7182–95. https://doi.org/10.1093/hmg/ddv415
- Aizawa M, Fukuda M. Small GTPase Rab2B and Its Specific Binding Protein Golgi-associated Rab2B Interactor-like 4 (GARI-L4) Regulate Golgi Morphology. J Biol Chem. 2015;290(36):22250–61. https://doi.org/10.1074/jbc.M115.669242
- Ni X, Ma Y, Cheng H, et al. Molecular cloning and characterization of a novel human Rab (Rab2B) gene. J Hum Genet. 2002;47(10):548–51. https://doi.org/10.1007/s100380200083
- Bereczki E, Francis PT, Howlett D, et al. Synaptic proteins predict cognitive decline in Alzheimer's disease and Lewy body dementia. Alzheimers Dement. 2016;12(11):1149–58. https://doi.org/10.1016/j.jalz.2016.04.005
- Bustos MA, Lucchesi O, Ruete MC, et al. Small GTPases in acrosomal exocytosis. Methods Mol Biol. 2015;1298:141–60. https://doi.org/10.1007/978-1-4939-2569-8_12
- Chen RH, Wislet-Gendebien S, Samuel F, et al. alpha-Synuclein membrane association is regulated by the Rab3a recycling machinery and presynaptic activity. J Biol Chem. 2013;288(11):7438–49. https://doi.org/10.1074/jbc.M112.439497
- Encarnacao M, Espada L, Escrevente C, et al. A Rab3a-dependent complex essential for lysosome positioning and plasma membrane repair. J Cell Biol. 2016;213(6):631–40. https://doi.org/10.1083/jcb.201511093
- Fischer von Mollard G, Mignery GA, Baumert M, et al. rab3 is a small GTP-binding protein exclusively localized to synaptic vesicles. Proc Natl Acad Sci U S A. 1990;87(5):1988–92. https://doi.org/10.1073/pnas.87.5.1988
- Hong Y, Zhao T, Li XJ, et al. Mutant Huntingtin Impairs BDNF Release from Astrocytes by Disrupting Conversion of Rab3a-GTP into Rab3a-GDP. J Neurosci. 2016;36(34):8790–801. https://doi.org/10.1523/JNEUROSCI.0168-16.2016
- Kang HJ, Voleti B, Hajszan T, et al. Decreased expression of synapse-related genes and loss of synapses in major depressive disorder. Nat Med. 2012;18(9):1413–7. https://doi.org/10.1038/nm.2886
- Kim JK, Lee SY, Park CW, et al. Rab3a promotes brain tumor initiation and progression. Mol Biol Rep. 2014;41(9):5903–11. https://doi.org/10.1007/s11033-014-3465-2
- Lodhi SS, Farmer R, Singh AK, et al. 3D structure generation, virtual screening and docking of human Ras-associated binding (Rab3A) protein involved in tumourigenesis. Mol Biol Rep. 2014;41(6):3951–9. https://doi.org/10.1007/s11033-014-3263-x
- Pavlos NJ, Jahn R. Distinct yet overlapping roles of Rab GTPases on synaptic vesicles. Small GTPases. 2011;2(2):77–81. https://doi.org/10.4161/sgtp.2.2.15201
- Raiborg C, Stenmark H. Plasma membrane repairs by small GTPase Rab3a. J Cell Biol. 2016;213(6):613–5. https://doi.org/10.1083/jcb.201606006
- Saetre P, Jazin E, Emilsson L. Age-related changes in gene expression are accelerated in Alzheimer's disease. Synapse. 2011;65(9):971–4. https://doi.org/10.1002/syn.20933
- Chung CY, Koprich JB, Hallett PJ, et al. Functional enhancement and protection of dopaminergic terminals by RAB3B overexpression. Proc Natl Acad Sci U S A. 2009;106(52):22474–9. https://doi.org/10.1073/pnas.0912193106
- Liu Q, Tang H, Liu X, et al. miR-200b as a prognostic factor targets multiple members of RAB family in glioma. Med Oncol. 2014;31(3):859. https://doi.org/10.1007/s12032-014-0859-x
- Nishioka H, Haraoka J. Significance of immunohistochemical expression of Rab3B and SNAP-25 in growth hormone-producing pituitary adenomas. Acta Neuropathol. 2005;109(6):598–602. https://doi.org/10.1007/s00401-005-1008-6
- Piper Hanley K, Hearn T, Berry A, et al. In vitro expression of NGN3 identifies RAB3B as the predominant Ras-associated GTP-binding protein 3 family member in human islets. J Endocrinol. 2010;207(2):151–61. https://doi.org/10.1677/JOE-10-0120
- Schluter OM, Khvotchev M, Jahn R, et al. Localization versus function of Rab3 proteins. Evidence for a common regulatory role in controlling fusion. J Biol Chem. 2002;277(43):40919–29. https://doi.org/10.1074/jbc.M203704200
- Schluter OM, Schmitz F, Jahn R, et al. A complete genetic analysis of neuronal Rab3 function. J Neurosci. 2004;24(29):6629–37. https://doi.org/10.1523/JNEUROSCI.1610-04.2004
- Schonn JS, van Weering JR, Mohrmann R, et al. Rab3 proteins involved in vesicle biogenesis and priming in embryonic mouse chromaffin cells. Traffic. 2010;11(11):1415–28. https://doi.org/10.1111/j.1600-0854.2010.01107.x
- Tsetsenis T, Younts TJ, Chiu CQ, et al. Rab3B protein is required for long-term depression of hippocampal inhibitory synapses and for normal reversal learning. Proc Natl Acad Sci U S A. 2011;108(34):14300–5. https://doi.org/10.1073/pnas.1112237108
- van ISC, Tuvim MJ, Weimbs T, et al. Direct interaction between Rab3b and the polymeric immunoglobulin receptor controls ligand-stimulated transcytosis in epithelial cells. Dev Cell. 2002;2(2):219–28. https://doi.org/10.1016/S1534-5807(02)00115-6
- Ye F, Tang H, Liu Q, et al. miR-200b as a prognostic factor in breast cancer targets multiple members of RAB family. J Transl Med. 2014;12:17. https://doi.org/10.1186/1479-5876-12-17
- Zou L, Zhou J, Zhang J, et al. The GTPase Rab3b/3c-positive recycling vesicles are involved in cross-presentation in dendritic cells. Proc Natl Acad Sci U S A. 2009;106(37):15801–6. https://doi.org/10.1073/pnas.0905684106
- Carroll K, Ray K, Helm B, et al. Differential expression of Rab3 isoforms in high- and low-secreting mast cell lines. Eur J Cell Biol. 2001;80(4):295–302. https://doi.org/10.1078/0171-9335-00161
- Cheng H, Ma Y, Ni X, et al. Cloning, mapping, and characterization of the human Rab3C gene. Biochem Genet. 2002;40(7-8):263–72. https://doi.org/10.1023/A:1019834901190
- Fischer von Mollard G, Stahl B, Khokhlatchev A, et al. Rab3C is a synaptic vesicle protein that dissociates from synaptic vesicles after stimulation of exocytosis. J Biol Chem. 1994;269(15):10971–4.
- Uphues I, Chern Y, Eckel J. Insulin-dependent translocation of the small GTP-binding protein rab3C in cardiac muscle: studies on insulin-resistant Zucker rats. FEBS Lett. 1995;377(2):109–12. https://doi.org/10.1016/0014-5793(95)01315-6
- Larkin JM, Woo B, Balan V, et al. Rab3D, a small GTP-binding protein implicated in regulated secretion, is associated with the transcytotic pathway in rat hepatocytes. Hepatology. 2000;32(2):348–56. https://doi.org/10.1053/jhep.2000.9110
- Luo Y, Ye GY, Qin SL, et al. High expression of Rab3D predicts poor prognosis and associates with tumor progression in colorectal cancer. Int J Biochem Cell Biol. 2016;75:53–62. https://doi.org/10.1016/j.biocel.2016.03.017
- Martelli AM, Baldini G, Tabellini G, et al. Rab3A and Rab3D control the total granule number and the fraction of granules docked at the plasma membrane in PC12 cells. Traffic. 2000;1(12):976–86.
- Millar AL, Pavios NJ, Xu J, et al. Rab3D: a regulator of exocytosis in non-neuronal cells. Histol Histopathol. 2002;17(3):929–36.
- Pavlos NJ, Xu J, Riedel D, et al. Rab3D regulates a novel vesicular trafficking pathway that is required for osteoclastic bone resorption. Mol Cell Biol. 2005;25(12):5253–69. https://doi.org/10.1128/MCB.25.12.5253-5269.2005
- Yang J, Liu W, Lu X, et al. High expression of small GTPase Rab3D promotes cancer progression and metastasis. Oncotarget. 2015;6(13):11125–38. https://doi.org/10.18632/oncotarget.3575
- Zhang J, Kong R, Sun L. Silencing of Rab3D suppresses the proliferation and invasion of esophageal squamous cell carcinoma cells. Biomed Pharmacother. 2017;91:402–7. https://doi.org/10.1016/j.biopha.2017.04.010
- Zhu S, Chim SM, Cheng T, et al. Calmodulin interacts with Rab3D and modulates osteoclastic bone resorption. Sci Rep. 2016;6:37963. https://doi.org/10.1038/srep37963
- Arjonen A, Alanko J, Veltel S, et al. Distinct recycling of active and inactive beta1 integrins. Traffic. 2012;13(4):610–25. https://doi.org/10.1111/j.1600-0854.2012.01327.x
- Barbarin A, Frade R. Procathepsin L secretion, which triggers tumour progression, is regulated by Rab4a in human melanoma cells. Biochem J. 2011;437(1):97–107. https://doi.org/10.1042/BJ20110361
- Chen Y, Wang Y, Zhang J, et al. Rab10 and myosin-Va mediate insulin-stimulated GLUT4 storage vesicle translocation in adipocytes. J Cell Biol. 2012;198(4):545–60. https://doi.org/10.1083/jcb.201111091
- Do MT, Chai TF, Casey PJ, et al. Isoprenylcysteine carboxylmethyltransferase function is essential for RAB4A-mediated integrin beta3 recycling, cell migration and cancer metastasis. Oncogene. 2017;36(14):5757–67. https://doi.org/10.1038/onc.2017.183
- Lazzarino DA, Blier P, Mellman I. The monomeric guanosine triphosphatase rab4 controls an essential step on the pathway of receptor-mediated antigen processing in B cells. J Exp Med. 1998;188(10):1769–74. https://doi.org/10.1084/jem.188.10.1769
- Uphues I, Kolter T, Goud B, et al. Failure of insulin-regulated recruitment of the glucose transporter GLUT4 in cardiac muscle of obese Zucker rats is associated with alterations of small-molecular-mass GTP-binding proteins. Biochem J. 1995;311(Pt 1):161–6. https://doi.org/10.1042/bj3110161
- van der Sluijs P, Hull M, Webster P, et al. The small GTP-binding protein rab4 controls an early sorting event on the endocytic pathway. Cell. 1992;70(5):729–40. https://doi.org/10.1016/0092-8674(92)90307-X
- Van Der Sluijs P, Hull M, Zahraoui A, et al. The small GTP-binding protein rab4 is associated with early endosomes. Proc Natl Acad Sci U S A. 1991;88(14):6313–7. https://doi.org/10.1073/pnas.88.14.6313
- Hou L, Cai MJ, Liu W, et al. Small GTPase Rab4b participates in the gene transcription of 20-hydroxyecdysone and insulin pathways to regulate glycogen level and metamorphosis. Dev Biol. 2012;371(1):13–22. https://doi.org/10.1016/j.ydbio.2012.06.015
- Kaddai V, Gonzalez T, Keslair F, et al. Rab4b is a small GTPase involved in the control of the glucose transporter GLUT4 localization in adipocyte. PLoS One. 2009;4(4):e5257. https://doi.org/10.1371/journal.pone.0005257
- Krawczyk M, Leimgruber E, Seguin-Estevez Q, et al. Expression of RAB4B, a protein governing endocytic recycling, is co-regulated with MHC class II genes. Nucleic Acids Res. 2007;35(2):595–605. https://doi.org/10.1093/nar/gkl980
- Perrin L, Lacas-Gervais S, Gilleron J, et al. Rab4b controls an early endosome sorting event by interacting with the gamma-subunit of the clathrin adaptor complex 1. J Cell Sci. 2013;126(Pt 21):4950–62. https://doi.org/10.1242/jcs.130575
- Thean LF, Wong YH, Lo M, et al. Chromosome 19q13 disruption alters expressions of CYP2A7, MIA and MIA-RAB4B lncRNA and contributes to FAP-like phenotype in APC mutation-negative familial colorectal cancer patients. PLoS One. 2017;12(3):e0173772. https://doi.org/10.1371/journal.pone.0173772
- Gorvel JP, Chavrier P, Zerial M, et al. rab5 controls early endosome fusion in vitro. Cell. 1991;64(5):915–25. https://doi.org/10.1016/0092-8674(91)90316-Q
- Lu Y, Dong S, Hao B, et al. Vacuolin-1 potently and reversibly inhibits autophagosome-lysosome fusion by activating RAB5A. Autophagy. 2014;10(11):1895–905. https://doi.org/10.4161/auto.32200
- Saitoh S, Maruyama T, Yako Y, et al. Rab5-regulated endocytosis plays a crucial role in apical extrusion of transformed cells. Proc Natl Acad Sci U S A. 2017;114(12):E2327–E36. https://doi.org/10.1073/pnas.1602349114
- Silva P, Soto N, Diaz J, et al. Down-regulation of Rab5 decreases characteristics associated with maintenance of cell transformation. Biochem Biophys Res Commun. 2015;464(2):642–6. https://doi.org/10.1016/j.bbrc.2015.06.176
- Xu Y, An Y, Wang Y, et al. miR-101 inhibits autophagy and enhances cisplatin-induced apoptosis in hepatocellular carcinoma cells. Oncol Rep. 2013;29(5):2019–24. https://doi.org/10.3892/or.2013.2338
- Yu MH, Luo Y, Qin SL, et al. Increased expression of Rab5A predicts metastasis and poor prognosis in colorectal cancer patients. Int J Clin Exp Pathol. 2015;8(6):6974–80.
- Arnett AL, Bayazitov I, Blaabjerg M, et al. Antisense oligonucleotide against GTPase Rab5b inhibits metabotropic agonist DHPG-induced neuroprotection. Brain Res. 2004;1028(1):59–65. https://doi.org/10.1016/j.brainres.2004.08.064
- Bucci C, Lutcke A, Steele-Mortimer O, et al. Co-operative regulation of endocytosis by three Rab5 isoforms. FEBS Lett. 1995;366(1):65–71. https://doi.org/10.1016/0014-5793(95)00477-Q
- Yun HJ, Kim H, Ga I, et al. An early endosome regulator, Rab5b, is an LRRK2 kinase substrate. J Biochem. 2015;157(6):485–95. https://doi.org/10.1093/jb/mvv005
- Onodera Y, Nam JM, Hashimoto A, et al. Rab5c promotes AMAP1-PRKD2 complex formation to enhance beta1 integrin recycling in EGF-induced cancer invasion. J Cell Biol. 2012;197(7):983–96. https://doi.org/10.1083/jcb.201201065
- Tan YS, Kim M, Kingsbury TJ, et al. Regulation of RAB5C is important for the growth inhibitory effects of MiR-509 in human precursor-B acute lymphoblastic leukemia. PLoS One. 2014;9(11):e111777. https://doi.org/10.1371/journal.pone.0111777
- Vizoso M, Ferreira HJ, Lopez-Serra P, et al. Epigenetic activation of a cryptic TBC1D16 transcript enhances melanoma progression by targeting EGFR. Nat Med. 2015;21(7):741–50. https://doi.org/10.1038/nm.3863
- Bardin S, Miserey-Lenkei S, Hurbain I, et al. Phenotypic characterisation of RAB6A knockout mouse embryonic fibroblasts. Biol Cell. 2015;107(12):427–39. https://doi.org/10.1111/boc.201400083
- Elfrink HL, Zwart R, Cavanillas ML, et al. Rab6 is a modulator of the unfolded protein response: implications for Alzheimer's disease. J Alzheimers Dis. 2012;28(4):917–29.
- Goud B, Akhmanova A. Rab6 GTPase. In: Li G, Segev N, editors. Rab GTPases and Membrane Trafficking. Bentham Science Publishers; 2012. p. 34–46.
- Lee PL, Ohlson MB, Pfeffer SR. Rab6 regulation of the kinesin family KIF1C motor domain contributes to Golgi tethering. Elife. 2015;4:https://doi.org/10.7554/eLife.06029
- Peeters K, Litvinenko I, Asselbergh B, et al. Molecular defects in the motor adaptor BICD2 cause proximal spinal muscular atrophy with autosomal-dominant inheritance. Am J Hum Genet. 2013;92(6):955–64. https://doi.org/10.1016/j.ajhg.2013.04.013
- Seifert W, Kuhnisch J, Maritzen T, et al. Cohen syndrome-associated protein COH1 physically and functionally interacts with the small GTPase RAB6 at the Golgi complex and directs neurite outgrowth. J Biol Chem. 2015;290(6):3349–58. https://doi.org/10.1074/jbc.M114.608174
- White J, Johannes L, Mallard F, et al. Rab6 coordinates a novel Golgi to ER retrograde transport pathway in live cells. J Cell Biol. 1999;147(4):743–60. https://doi.org/10.1083/jcb.147.4.743
- Opdam FJ, Echard A, Croes HJ, et al. The small GTPase Rab6B, a novel Rab6 subfamily member, is cell-type specifically expressed and localised to the Golgi apparatus. J Cell Sci. 2000;113(Pt 15):2725–35.
- Wanschers BF, van de Vorstenbosch R, Schlager MA, et al. A role for the Rab6B Bicaudal-D1 interaction in retrograde transport in neuronal cells. Exp Cell Res. 2007;313(16):3408–20. https://doi.org/10.1016/j.yexcr.2007.05.032
- Shan J, Mason JM, Yuan L, et al. Rab6c, a new member of the rab gene family, is involved in drug resistance in MCF7/AdrR cells. Gene. 2000;257(1):67–75. https://doi.org/10.1016/S0378-1119(00)00395-4
- Young J, Menetrey J, Goud B. RAB6C is a retrogene that encodes a centrosomal protein involved in cell cycle progression. J Mol Biol. 2010;397(1):69–88. https://doi.org/10.1016/j.jmb.2010.01.009
- Chi ZL, Akahori M, Obazawa M, et al. Overexpression of optineurin E50K disrupts Rab8 interaction and leads to a progressive retinal degeneration in mice. Hum Mol Genet. 2010;19(13):2606–15. https://doi.org/10.1093/hmg/ddq146
- Hagemann N, Hou X, Goody RS, et al. Crystal structure of the Rab binding domain of OCRL1 in complex with Rab8 and functional implications of the OCRL1/Rab8 module for Lowe syndrome. Small GTPases. 2012;3(2):107–10. https://doi.org/10.4161/sgtp.19380
- Huber LA, Pimplikar S, Parton RG, et al. Rab8, a small GTPase involved in vesicular traffic between the TGN and the basolateral plasma membrane. J Cell Biol. 1993;123(1):35–45. https://doi.org/10.1083/jcb.123.1.35
- Kim MJ, Deng HX, Wong YC, et al. The Parkinson's disease-linked protein TMEM230 is required for Rab8a-mediated secretory vesicle trafficking and retromer trafficking. Hum Mol Genet. 2017;26(4):729–41.
- Lai YC, Kondapalli C, Lehneck R, et al. Phosphoproteomic screening identifies Rab GTPases as novel downstream targets of PINK1. EMBO J. 2015;34(22):2840–61. https://doi.org/10.15252/embj.201591593
- Ao X, Zou L, Wu Y. Regulation of autophagy by the Rab GTPase network. Cell Death Differ. 2014;21(3):348–58. https://doi.org/10.1038/cdd.2013.187
- Goncalves SA, Outeiro TF. Traffic jams and the complex role of alpha-Synuclein aggregation in Parkinson disease. Small GTPases. 2017;8(2):78–84. https://doi.org/10.1080/21541248.2016.1199191
- Heidrych P, Zimmermann U, Bress A, et al. Rab8b GTPase, a protein transport regulator, is an interacting partner of otoferlin, defective in a human autosomal recessive deafness form. Hum Mol Genet. 2008;17(23):3814–21. https://doi.org/10.1093/hmg/ddn279
- Kobayashi S, Suzuki T, Kawaguchi A, et al. Rab8b Regulates Transport of West Nile Virus Particles from Recycling Endosomes. J Biol Chem. 2016;291(12):6559–68. https://doi.org/10.1074/jbc.M115.712760
- Sato T, Iwano T, Kunii M, et al. Rab8a and Rab8b are essential for several apical transport pathways but insufficient for ciliogenesis. J Cell Sci. 2014;127(Pt 2):422–31. https://doi.org/10.1242/jcs.136903
- Ganley IG, Pfeffer SR. Cholesterol accumulation sequesters Rab9 and disrupts late endosome function in NPC1-deficient cells. J Biol Chem. 2006;281(26):17890–9. https://doi.org/10.1074/jbc.M601679200
- Hirota Y, Yamashita S, Kurihara Y, et al. Mitophagy is primarily due to alternative autophagy and requires the MAPK1 and MAPK14 signaling pathways. Autophagy. 2015;11(2):332–43. https://doi.org/10.1080/15548627.2015.1023047
- Kaptzan T, West SA, Holicky EL, et al. Development of a Rab9 transgenic mouse and its ability to increase the lifespan of a murine model of Niemann-Pick type C disease. Am J Pathol. 2009;174(1):14–20. https://doi.org/10.2353/ajpath.2009.080660
- Lombardi D, Soldati T, Riederer MA, et al. Rab9 functions in transport between late endosomes and the trans Golgi network. EMBO J. 1993;12(2):677–82.
- Murray JL, Mavrakis M, McDonald NJ, et al. Rab9 GTPase is required for replication of human immunodeficiency virus type 1, filoviruses, and measles virus. J Virol. 2005;79(18):11742–51. https://doi.org/10.1128/JVI.79.18.11742-11751.2005
- Seki N, Azuma T, Yoshikawa T, et al. cDNA cloning of a new member of the Ras superfamily, RAB9-like, on the human chromosome Xq22.1-q22.3 region. J Hum Genet. 2000;45(5):318–22. https://doi.org/10.1007/s100380070025
- Bruno J, Brumfield A, Chaudhary N, et al. SEC16A is a RAB10 effector required for insulin-stimulated GLUT4 trafficking in adipocytes. J Cell Biol. 2016;214(1):61–76. https://doi.org/10.1083/jcb.201509052
- Isabella AJ, Horne-Badovinac S. Rab10-Mediated Secretion Synergizes with Tissue Movement to Build a Polarized Basement Membrane Architecture for Organ Morphogenesis. Dev Cell. 2016;38(1):47–60. https://doi.org/10.1016/j.devcel.2016.06.009
- Jiang W, Liu J, Xu T, et al. MiR-329 suppresses osteosarcoma development by downregulating Rab10. FEBS Lett. 2016;590(17):2973–81. https://doi.org/10.1002/1873-3468.12337
- Li Z, Schulze RJ, Weller SG, et al. A novel Rab10-EHBP1-EHD2 complex essential for the autophagic engulfment of lipid droplets. Sci Adv. 2016;2(12):e1601470. https://doi.org/10.1126/sciadv.1601470
- Sano H, Eguez L, Teruel MN, et al. Rab10, a target of the AS160 Rab GAP, is required for insulin-stimulated translocation of GLUT4 to the adipocyte plasma membrane. Cell Metab. 2007;5(4):293–303. https://doi.org/10.1016/j.cmet.2007.03.001
- Vazirani RP, Verma A, Sadacca LA, et al. Disruption of Adipose Rab10-Dependent Insulin Signaling Causes Hepatic Insulin Resistance. Diabetes. 2016;65(6):1577–89. https://doi.org/10.2337/db15-1128
- Bruce EA, Digard P, Stuart AD. The Rab11 pathway is required for influenza A virus budding and filament formation. J Virol. 2010;84(12):5848–59. https://doi.org/10.1128/JVI.00307-10
- Kelly EE, Horgan CP, McCaffrey MW. Rab11 proteins in health and disease. Biochem Soc Trans. 2012;40(6):1360–7. https://doi.org/10.1042/BST20120157
- Li J, Kanekiyo T, Shinohara M, et al. Differential regulation of amyloid-beta endocytic trafficking and lysosomal degradation by apolipoprotein E isoforms. J Biol Chem. 2012;287(53):44593–601. https://doi.org/10.1074/jbc.M112.420224
- Li X, Valencia A, Sapp E, et al. Aberrant Rab11-dependent trafficking of the neuronal glutamate transporter EAAC1 causes oxidative stress and cell death in Huntington's disease. J Neurosci. 2010;30(13):4552–61. https://doi.org/10.1523/JNEUROSCI.5865-09.2010
- Roberts RC, Peden AA, Buss F, et al. Mistargeting of SH3TC2 away from the recycling endosome causes Charcot-Marie-Tooth disease type 4C. Hum Mol Genet. 2010;19(6):1009–18. https://doi.org/10.1093/hmg/ddp565
- Rzomp KA, Scholtes LD, Briggs BJ, et al. Rab GTPases are recruited to chlamydial inclusions in both a species-dependent and species-independent manner. Infect Immun. 2003;71(10):5855–70. https://doi.org/10.1128/IAI.71.10.5855-5870.2003
- Ullrich O, Reinsch S, Urbe S, et al. Rab11 regulates recycling through the pericentriolar recycling endosome. J Cell Biol. 1996;135(4):913–24. https://doi.org/10.1083/jcb.135.4.913
- Grimsey NJ, Coronel LJ, Cordova IC, et al. Recycling and Endosomal Sorting of Protease-activated Receptor-1 Is Distinctly Regulated by Rab11A and Rab11B Proteins. J Biol Chem. 2016;291(5):2223–36. https://doi.org/10.1074/jbc.M115.702993
- Tarafder AK, Bolasco G, Correia MS, et al. Rab11b mediates melanin transfer between donor melanocytes and acceptor keratinocytes via coupled exo/endocytosis. J Invest Dermatol. 2014;134(4):1056–66. https://doi.org/10.1038/jid.2013.432
- Efergan A, Azouz NP, Klein O, et al. Rab12 Regulates Retrograde Transport of Mast Cell Secretory Granules by Interacting with the RILP-Dynein Complex. J Immunol. 2016;196(3):1091–101. https://doi.org/10.4049/jimmunol.1500731
- Matsui T, Fukuda M. Small GTPase Rab12 regulates transferrin receptor degradation: Implications for a novel membrane trafficking pathway from recycling endosomes to lysosomes. Cell Logist. 2011;1(4):155–8. https://doi.org/10.4161/cl.1.4.18152
- Xu J, Fotouhi M, McPherson PS. Phosphorylation of the exchange factor DENND3 by ULK in response to starvation activates Rab12 and induces autophagy. EMBO Rep. 2015;16(6):709–18. https://doi.org/10.15252/embr.201440006
- Yoshida T, Kobayashi T, Itoda M, et al. Clinical omics analysis of colorectal cancer incorporating copy number aberrations and gene expression data. Cancer Inform. 2010;9:147–61. https://doi.org/10.4137/CIN.S3851
- Ioannou MS, McPherson PS. Regulation of Cancer Cell Behavior by the Small GTPase Rab13. J Biol Chem. 2016;291(19):9929–37. https://doi.org/10.1074/jbc.R116.715193
- Morimoto S, Nishimura N, Terai T, et al. Rab13 mediates the continuous endocytic recycling of occludin to the cell surface. J Biol Chem. 2005;280(3):2220–8. https://doi.org/10.1074/jbc.M406906200
- Nokes RL, Fields IC, Collins RN, et al. Rab13 regulates membrane trafficking between TGN and recycling endosomes in polarized epithelial cells. J Cell Biol. 2008;182(5):845–53. https://doi.org/10.1083/jcb.200802176
- Ohira M, Oshitani N, Hosomi S, et al. Dislocation of Rab13 and vasodilator-stimulated phosphoprotein in inactive colon epithelium in patients with Crohn's disease. Int J Mol Med. 2009;24(6):829–35.
- Zahraoui A, Joberty G, Arpin M, et al. A small rab GTPase is distributed in cytoplasmic vesicles in non polarized cells but colocalizes with the tight junction marker ZO-1 in polarized epithelial cells. J Cell Biol. 1994;124(1-2):101–15. https://doi.org/10.1083/jcb.124.1.101
- Brewer PD, Habtemichael EN, Romenskaia I, et al. Rab14 limits the sorting of Glut4 from endosomes into insulin-sensitive regulated secretory compartments in adipocytes. Biochem J. 2016;473(10):1315–27. https://doi.org/10.1042/BCJ20160020
- Guo B, Wang W, Zhao Z, et al. Rab14 Act as Oncogene and Induce Proliferation of Gastric Cancer Cells via AKT Signaling Pathway. PLoS One. 2017;12(1):e0170620. https://doi.org/10.1371/journal.pone.0170620
- Junutula JR, De Maziere AM, Peden AA, et al. Rab14 is involved in membrane trafficking between the Golgi complex and endosomes. Mol Biol Cell. 2004;15(5):2218–29. https://doi.org/10.1091/mbc.E03-10-0777
- Kim EA, Kim TG, Sung EG, et al. miR-148a increases the sensitivity to cisplatin by targeting Rab14 in renal cancer cells. Int J Oncol. 2017;50(3):984–92. https://doi.org/10.3892/ijo.2017.3851
- Linford A, Yoshimura S, Nunes Bastos R, et al. Rab14 and its exchange factor FAM116 link endocytic recycling and adherens junction stability in migrating cells. Dev Cell. 2012;22(5):952–66. https://doi.org/10.1016/j.devcel.2012.04.010
- Lu R, Johnson DL, Stewart L, et al. Rab14 regulation of claudin-2 trafficking modulates epithelial permeability and lumen morphogenesis. Mol Biol Cell. 2014;25(11):1744–54. https://doi.org/10.1091/mbc.E13-12-0724
- Yu J, Wang L, Yang H, et al. Rab14 Suppression Mediated by MiR-320a Inhibits Cell Proliferation, Migration and Invasion in Breast Cancer. J Cancer. 2016;7(15):2317–26. https://doi.org/10.7150/jca.15737
- Nishimura N, Van Huyen Pham T, Hartomo TB, et al. Rab15 expression correlates with retinoic acid-induced differentiation of neuroblastoma cells. Oncol Rep. 2011;26(1):145–51.
- Strick DJ, Elferink LA. Rab15 effector protein: a novel protein for receptor recycling from the endocytic recycling compartment. Mol Biol Cell. 2005;16(12):5699–709. https://doi.org/10.1091/mbc.E05-03-0204
- Zuk PA, Elferink LA. Rab15 differentially regulates early endocytic trafficking. J Biol Chem. 2000;275(35):26754–64.
- Beaumont KA, Hamilton NA, Moores MT, et al. The recycling endosome protein Rab17 regulates melanocytic filopodia formation and melanosome trafficking. Traffic. 2011;12(5):627–43. https://doi.org/10.1111/j.1600-0854.2011.01172.x
- Haobam B, Nozawa T, Minowa-Nozawa A, et al. Rab17-mediated recycling endosomes contribute to autophagosome formation in response to Group A Streptococcus invasion. Cell Microbiol. 2014;16(12):1806–21. https://doi.org/10.1111/cmi.12329
- Hunziker W, Peters PJ. Rab17 localizes to recycling endosomes and regulates receptor-mediated transcytosis in epithelial cells. J Biol Chem. 1998;273(25):15734–41. https://doi.org/10.1074/jbc.273.25.15734
- Mori Y, Fukuda M, Henley JM. Small GTPase Rab17 regulates the surface expression of kainate receptors but not alpha-amino-3-hydroxy-5-methyl-4-isoxazolepropionic acid (AMPA) receptors in hippocampal neurons via dendritic trafficking of Syntaxin-4 protein. J Biol Chem. 2014;289(30):20773–87. https://doi.org/10.1074/jbc.M114.550632
- von Thun A, Birtwistle M, Kalna G, et al. ERK2 drives tumour cell migration in three-dimensional microenvironments by suppressing expression of Rab17 and liprin-beta2. J Cell Sci. 2012;125(Pt 6):1465–77.
- Wang K, Mao Z, Liu L, et al. Rab17 inhibits the tumourigenic properties of hepatocellular carcinomas via the Erk pathway. Tumour Biol. 2015;36(8):5815–24. https://doi.org/10.1007/s13277-015-3251-3
- Zacchi P, Stenmark H, Parton RG, et al. Rab17 regulates membrane trafficking through apical recycling endosomes in polarized epithelial cells. J Cell Biol. 1998;140(5):1039–53. https://doi.org/10.1083/jcb.140.5.1039
- Feldmann A, Bekbulat F, Huesmann H, et al. The RAB GTPase RAB18 modulates macroautophagy and proteostasis. Biochem Biophys Res Commun. 2017;486(3):738–43. https://doi.org/10.1016/j.bbrc.2017.03.112
- Li C, Luo X, Zhao S, et al. COPI-TRAPPII activates Rab18 and regulates its lipid droplet association. EMBO J. 2017;36(4):441–57. https://doi.org/10.15252/embj.201694866
- Martin S, Driessen K, Nixon SJ, et al. Regulated localization of Rab18 to lipid droplets: effects of lipolytic stimulation and inhibition of lipid droplet catabolism. J Biol Chem. 2005;280(51):42325–35. https://doi.org/10.1074/jbc.M506651200
- You X, Liu F, Zhang T, et al. Hepatitis B virus X protein upregulates oncogene Rab18 to result in the dysregulation of lipogenesis and proliferation of hepatoma cells. Carcinogenesis. 2013;34(7):1644–52. https://doi.org/10.1093/carcin/bgt089
- Zhong K, Chen K, Han L, et al. MicroRNA-30b/c inhibits non-small cell lung cancer cell proliferation by targeting Rab18. BMC Cancer. 2014;14:703. https://doi.org/10.1186/1471-2407-14-703
- Gillingham AK, Sinka R, Torres IL, et al. Toward a comprehensive map of the effectors of rab GTPases. Dev Cell. 2014;31(3):358–73. https://doi.org/10.1016/j.devcel.2014.10.007
- Amillet JM, Ferbus D, Real FX, et al. Characterization of human Rab20 overexpressed in exocrine pancreatic carcinoma. Hum Pathol. 2006;37(3):256–63. https://doi.org/10.1016/j.humpath.2005.10.017
- Egami Y, Araki N. Rab20 regulates phagosome maturation in RAW264 macrophages during Fc gamma receptor-mediated phagocytosis. PLoS One. 2012;7(4):e35663. https://doi.org/10.1371/journal.pone.0035663
- Ho JR, Chapeaublanc E, Kirkwood L, et al. Deregulation of Rab and Rab effector genes in bladder cancer. PLoS One. 2012;7(6):e39469. https://doi.org/10.1371/journal.pone.0039469
- Pei G, Schnettger L, Bronietzki M, et al. Interferon-gamma-inducible Rab20 regulates endosomal morphology and EGFR degradation in macrophages. Mol Biol Cell. 2015;26(17):3061–70. https://doi.org/10.1091/mbc.E14-11-1547
- Seto S, Tsujimura K, Koide Y. Rab GTPases regulating phagosome maturation are differentially recruited to mycobacterial phagosomes. Traffic. 2011;12(4):407–20. https://doi.org/10.1111/j.1600-0854.2011.01165.x
- Hognas G, Tuomi S, Veltel S, et al. Cytokinesis failure due to derailed integrin traffic induces aneuploidy and oncogenic transformation in vitro and in vivo. Oncogene. 2012;31(31):3597–606. https://doi.org/10.1038/onc.2011.527
- Simpson JC, Griffiths G, Wessling-Resnick M, et al. A role for the small GTPase Rab21 in the early endocytic pathway. J Cell Sci. 2004;117(Pt 26):6297–311. https://doi.org/10.1242/jcs.01560
- Kauppi M, Simonsen A, Bremnes B, et al. The small GTPase Rab22 interacts with EEA1 and controls endosomal membrane trafficking. J Cell Sci. 2002;115(Pt 5):899–911.
- Mesa R, Salomon C, Roggero M, et al. Rab22a affects the morphology and function of the endocytic pathway. J Cell Sci. 2001;114(Pt 22):4041–9.
- Su F, Chen Y, Zhu S, et al. RAB22A overexpression promotes the tumor growth of melanoma. Oncotarget. 2016;7(44):71744–53. https://doi.org/10.18632/oncotarget.12329
- Wang L, Liang Z, Li G. Rab22 controls NGF signaling and neurite outgrowth in PC12 cells. Mol Biol Cell. 2011;22(20):3853–60. https://doi.org/10.1091/mbc.E11-03-0277
- Weigert R, Yeung AC, Li J, et al. Rab22a regulates the recycling of membrane proteins internalized independently of clathrin. Mol Biol Cell. 2004;15(8):3758–70. https://doi.org/10.1091/mbc.E04-04-0342
- Yang D, Liu G, Wang K. miR-203 Acts as a Tumor Suppressor Gene in Osteosarcoma by Regulating RAB22A. PLoS One. 2015;10(9):e0132225. https://doi.org/10.1371/journal.pone.0132225
- Yu H, Yang W. MiR-211 is epigenetically regulated by DNMT1 mediated methylation and inhibits EMT of melanoma cells by targeting RAB22A. Biochem Biophys Res Commun. 2016;476(4):400–5. https://doi.org/10.1016/j.bbrc.2016.05.133
- Zhang Y, Zhao FJ, Chen LL, et al. MiR-373 targeting of the Rab22a oncogene suppresses tumor invasion and metastasis in ovarian cancer. Oncotarget. 2014;5(23):12291–303. https://doi.org/10.18632/oncotarget.2577
- Chen Y, Ng F, Tang BL. Rab23 activities and human cancer-emerging connections and mechanisms. Tumour Biol. 2016;37(10):12959–67. https://doi.org/10.1007/s13277-016-5207-7
- Zheng LQ, Chi SM, Li CX. Rab23's genetic structure, function and related diseases: a review. Biosci Rep. 2017;37(2):https://doi.org/10.1042/BSR20160410
- Agler C, Nielsen DM, Urkasemsin G, et al. Canine hereditary ataxia in old english sheepdogs and gordon setters is associated with a defect in the autophagy gene encoding RAB24. PLoS Genet. 2014;10(2):e1003991. https://doi.org/10.1371/journal.pgen.1003991
- Amaya C, Militello RD, Calligaris SD, et al. Rab24 interacts with the Rab7/Rab interacting lysosomal protein complex to regulate endosomal degradation. Traffic. 2016;17(11):1181–96. https://doi.org/10.1111/tra.12431
- Igci M, Baysan M, Yigiter R, et al. Gene expression profiles of autophagy-related genes in multiple sclerosis. Gene. 2016;588(1):38–46. https://doi.org/10.1016/j.gene.2016.04.042
- Jacobsen M, Repsilber D, Gutschmidt A, et al. Ras-associated small GTPase 33A, a novel T cell factor, is down-regulated in patients with tuberculosis. J Infect Dis. 2005;192(7):1211–8. https://doi.org/10.1086/444428
- Munafo DB, Colombo MI. Induction of autophagy causes dramatic changes in the subcellular distribution of GFP-Rab24. Traffic. 2002;3(7):472–82. https://doi.org/10.1034/j.1600-0854.2002.30704.x
- Olkkonen VM, Dupree P, Killisch I, et al. Molecular cloning and subcellular localization of three GTP-binding proteins of the rab subfamily. J Cell Sci. 1993;106(Pt 4):1249–61.
- Yla-Anttila P, Eskelinen EL. Roles for RAB24 in autophagy and disease. Small GTPases. 2017;1–9. https://doi.org/10.1080/21541248.2017.1317699
- Yla-Anttila P, Mikkonen E, Happonen KE, et al. RAB24 facilitates clearance of autophagic compartments during basal conditions. Autophagy. 2015;11(10):1833–48. https://doi.org/10.1080/15548627.2015.1086522
- Binotti B, Pavlos NJ, Riedel D, et al. The GTPase Rab26 links synaptic vesicles to the autophagy pathway. Elife. 2015;4:e05597. https://doi.org/10.7554/eLife.05597
- Jin RU, Mills JC. RAB26 coordinates lysosome traffic and mitochondrial localization. J Cell Sci. 2014;127(Pt 5):1018–32. https://doi.org/10.1242/jcs.138776
- Yoshie S, Imai A, Nashida T, et al. Expression, characterization, and localization of Rab26, a low molecular weight GTP-binding protein, in the rat parotid gland. Histochem Cell Biol. 2000;113(4):259–63. https://doi.org/10.1007/s004180000130
- Chang C, Liu T, Huang Y, et al. MicroRNA-134-3p is a novel potential inhibitor of human ovarian cancer stem cells by targeting RAB27A. Gene. 2017;605:99–107. https://doi.org/10.1016/j.gene.2016.12.030
- Haddad EK, Wu X, Hammer JA, 3rd, et al. Defective granule exocytosis in Rab27a-deficient lymphocytes from Ashen mice. J Cell Biol. 2001;152(4):835–42. https://doi.org/10.1083/jcb.152.4.835
- Li Y, Chen S, Shan Z, et al. miR-182-5p improves the viability, mitosis, migration, and invasion ability of human gastric cancer cells by down-regulating RAB27A. Biosci Rep. 2017;37(3):https://doi.org/10.1042/BSR20170136
- Wang Q, Ni Q, Wang X, et al. High expression of RAB27A and TP53 in pancreatic cancer predicts poor survival. Med Oncol. 2015;32(1):372. https://doi.org/10.1007/s12032-014-0372-2
- Worst TS, Meyer Y, Gottschalt M, et al. RAB27A, RAB27B and VPS36 are downregulated in advanced prostate cancer and show functional relevance in prostate cancer cells. Int J Oncol. 2017;50(3):920–32. https://doi.org/10.3892/ijo.2017.3872
- Wu X, Rao K, Bowers MB, et al. Rab27a enables myosin Va-dependent melanosome capture by recruiting the myosin to the organelle. J Cell Sci. 2001;114(Pt 6):1091–100.
- Zhang X, Zhang Y, Yang J, et al. Upregulation of miR-582-5p inhibits cell proliferation, cell cycle progression and invasion by targeting Rab27a in human colorectal carcinoma. Cancer Gene Ther. 2015;22(10):475–80. https://doi.org/10.1038/cgt.2015.44
- Chen Y, Samaraweera P, Sun TT, et al. Rab27b association with melanosomes: dominant negative mutants disrupt melanosomal movement. J Invest Dermatol. 2002;118(6):933–40. https://doi.org/10.1046/j.1523-1747.2002.01754.x
- Huang D, Bian G, Pan Y, et al. MiR-20a-5p promotes radio-resistance by targeting Rab27B in nasopharyngeal cancer cells. Cancer Cell Int. 2017;17:32. https://doi.org/10.1186/s12935-017-0389-7
- Munoz I, Danelli L, Claver J, et al. Kinesin-1 controls mast cell degranulation and anaphylaxis through PI3K-dependent recruitment to the granular Slp3/Rab27b complex. J Cell Biol. 2016;215(2):203–16. https://doi.org/10.1083/jcb.201605073
- Ren P, Yang XQ, Zhai XL, et al. Overexpression of Rab27B is correlated with distant metastasis and poor prognosis in ovarian cancer. Oncol Lett. 2016;12(2):1539–45.
- Shen YT, Gu Y, Su WF, et al. Rab27b is Involved in Lysosomal Exocytosis and Proteolipid Protein Trafficking in Oligodendrocytes. Neurosci Bull. 2016;32(4):331–40. https://doi.org/10.1007/s12264-016-0045-6
- Zhao H, Wang Q, Wang X, et al. Correlation Between RAB27B and p53 Expression and Overall Survival in Pancreatic Cancer. Pancreas. 2016;45(2):204–10. https://doi.org/10.1097/MPA.0000000000000453
- Jensen VL, Carter S, Sanders AA, et al. Whole-Organism Developmental Expression Profiling Identifies RAB-28 as a Novel Ciliary GTPase Associated with the BBSome and Intraflagellar Transport. PLoS Genet. 2016;12(12):e1006469. https://doi.org/10.1371/journal.pgen.1006469
- Roosing S, Rohrschneider K, Beryozkin A, et al. Mutations in RAB28, encoding a farnesylated small GTPase, are associated with autosomal-recessive cone-rod dystrophy. Am J Hum Genet. 2013;93(1):110–7. https://doi.org/10.1016/j.ajhg.2013.05.005
- Aoki Y, Manzano R, Lee Y, et al. C9orf72 and RAB7L1 regulate vesicle trafficking in amyotrophic lateral sclerosis and frontotemporal dementia. Brain. 2017;140(4):887–97. https://doi.org/10.1093/brain/awx024
- Guo XY, Chen YP, Song W, et al. An association analysis of the rs1572931 polymorphism of the RAB7L1 gene in Parkinson's disease, amyotrophic lateral sclerosis and multiple system atrophy in China. Eur J Neurol. 2014;21(10):1337–43. https://doi.org/10.1111/ene.12490
- Khaligh A, Goudarzian M, Moslem A, et al. RAB7L1 promoter polymorphism and risk of Parkinson's disease; a case-control study. Neurol Res. 2017;39(5):468–71. https://doi.org/10.1080/01616412.2017.1297558
- Onnis A, Finetti F, Patrussi L, et al. The small GTPase Rab29 is a common regulator of immune synapse assembly and ciliogenesis. Cell Death Differ. 2015;22(10):1687–99. https://doi.org/10.1038/cdd.2015.17
- Spano S, Liu X, Galan JE. Proteolytic targeting of Rab29 by an effector protein distinguishes the intracellular compartments of human-adapted and broad-host Salmonella. Proc Natl Acad Sci U S A. 2011;108(45):18418–23. https://doi.org/10.1073/pnas.1111959108
- Wang S, Ma Z, Xu X, et al. A role of Rab29 in the integrity of the trans-Golgi network and retrograde trafficking of mannose-6-phosphate receptor. PLoS One. 2014;9(5):e96242. https://doi.org/10.1371/journal.pone.0096242
- Kelly EE, Giordano F, Horgan CP, et al. Rab30 is required for the morphological integrity of the Golgi apparatus. Biol Cell. 2012;104(2):84–101. https://doi.org/10.1111/boc.201100080
- Oda S, Nozawa T, Nozawa-Minowa A, et al. Golgi-Resident GTPase Rab30 Promotes the Biogenesis of Pathogen-Containing Autophagosomes. PLoS One. 2016;11(1):e0147061. https://doi.org/10.1371/journal.pone.0147061
- Bultema JJ, Boyle JA, Malenke PB, et al. Myosin vc interacts with Rab32 and Rab38 proteins and works in the biogenesis and secretion of melanosomes. J Biol Chem. 2014;289(48):33513–28. https://doi.org/10.1074/jbc.M114.578948
- Gerondopoulos A, Langemeyer L, Liang JR, et al. BLOC-3 mutated in Hermansky-Pudlak syndrome is a Rab32/38 guanine nucleotide exchange factor. Curr Biol. 2012;22(22):2135–9. https://doi.org/10.1016/j.cub.2012.09.020
- Li Q, Wang J, Wan Y, et al. Depletion of Rab32 decreases intracellular lipid accumulation and induces lipolysis through enhancing ATGL expression in hepatocytes. Biochem Biophys Res Commun. 2016;471(4):492–6. https://doi.org/10.1016/j.bbrc.2016.02.047
- Li Y, Wang Y, Zou L, et al. Analysis of the Rab GTPase Interactome in Dendritic Cells Reveals Anti-microbial Functions of the Rab32 Complex in Bacterial Containment. Immunity. 2016;44(2):422–37. https://doi.org/10.1016/j.immuni.2016.01.027
- Niyogi S, Jimenez V, Girard-Dias W, et al. Rab32 is essential for maintaining functional acidocalcisomes, and for growth and infectivity of Trypanosoma cruzi. J Cell Sci. 2015;128(12):2363–73. https://doi.org/10.1242/jcs.169466
- Pham TM, Tran SC, Lim YS, et al. Hepatitis C Virus-Induced Rab32 Aggregation and Its Implications for Virion Assembly. J Virol. 2017;91(3):https://doi.org/10.1128/JVI.01662-16
- Solano-Collado V, Rofe A, Spano S. Rab32 restriction of intracellular bacterial pathogens. Small GTPases. 2016;1–8. https://doi.org/10.1080/21541248.2016.1219207
- Spano S. Host restriction in Salmonella: insights from Rab GTPases. Cell Microbiol. 2014;16(9):1321–8. https://doi.org/10.1111/cmi.12327
- Wasmeier C, Romao M, Plowright L, et al. Rab38 and Rab32 control post-Golgi trafficking of melanogenic enzymes. J Cell Biol. 2006;175(2):271–81. https://doi.org/10.1083/jcb.200606050
- Zhang F, Liu H, Chen S, et al. Identification of two new loci at IL23R and RAB32 that influence susceptibility to leprosy. Nat Genet. 2011;43(12):1247–51. https://doi.org/10.1038/ng.973
- Imai A, Tsujimura M, Yoshie S, et al. The small GTPase Rab33A participates in regulation of amylase release from parotid acinar cells. Biochem Biophys Res Commun. 2015;461(3):469–74. https://doi.org/10.1016/j.bbrc.2015.04.022
- Moller RS, Jensen LR, Maas SM, et al. X-linked congenital ptosis and associated intellectual disability, short stature, microcephaly, cleft palate, digital and genital abnormalities define novel Xq25q26 duplication syndrome. Hum Genet. 2014;133(5):625–38. https://doi.org/10.1007/s00439-013-1403-3
- Nakazawa H, Sada T, Toriyama M, et al. Rab33a mediates anterograde vesicular transport for membrane exocytosis and axon outgrowth. J Neurosci. 2012;32(37):12712–25. https://doi.org/10.1523/JNEUROSCI.0989-12.2012
- Alshammari MJ, Al-Otaibi L, Alkuraya FS. Mutation in RAB33B, which encodes a regulator of retrograde Golgi transport, defines a second Dyggve–Melchior–Clausen locus. J Med Genet. 2012;49(7):455–61. https://doi.org/10.1136/jmedgenet-2011-100666
- Doring T, Prange R. Rab33B and its autophagic Atg5/12/16L1 effector assist in hepatitis B virus naked capsid formation and release. Cell Microbiol. 2015;17(5):747–64. https://doi.org/10.1111/cmi.12398
- Dupuis N, Lebon S, Kumar M, et al. A novel RAB33B mutation in Smith-McCort dysplasia. Hum Mutat. 2013;34(2):283–6. https://doi.org/10.1002/humu.22235
- Itoh T, Fujita N, Kanno E, et al. Golgi-resident small GTPase Rab33B interacts with Atg16L and modulates autophagosome formation. Mol Biol Cell. 2008;19(7):2916–25. https://doi.org/10.1091/mbc.E07-12-1231
- Itoh T, Kanno E, Uemura T, et al. OATL1, a novel autophagosome-resident Rab33B-GAP, regulates autophagosomal maturation. J Cell Biol. 2011;192(5):839–53. https://doi.org/10.1083/jcb.201008107
- Pusapati GV, Luchetti G, Pfeffer SR. Ric1-Rgp1 complex is a guanine nucleotide exchange factor for the late Golgi Rab6A GTPase and an effector of the medial Golgi Rab33B GTPase. J Biol Chem. 2012;287(50):42129–37. https://doi.org/10.1074/jbc.M112.414565
- Salian S, Cho TJ, Phadke SR, et al. Additional three patients with Smith-McCort dysplasia due to novel RAB33B mutations. Am J Med Genet A. 2017;173(3):588–95. https://doi.org/10.1002/ajmg.a.38064
- Starr T, Sun Y, Wilkins N, et al. Rab33b and Rab6 are functionally overlapping regulators of Golgi homeostasis and trafficking. Traffic. 2010;11(5):626–36. https://doi.org/10.1111/j.1600-0854.2010.01051.x
- Zheng JY, Koda T, Fujiwara T, et al. A novel Rab GTPase, Rab33B, is ubiquitously expressed and localized to the medial Golgi cisternae. J Cell Sci. 1998;111(Pt 8):1061–9.
- Goldenberg NM, Grinstein S, Silverman M. Golgi-bound Rab34 is a novel member of the secretory pathway. Mol Biol Cell. 2007;18(12):4762–71. https://doi.org/10.1091/mbc.E06-11-0991
- Kasmapour B, Gronow A, Bleck CK, et al. Size-dependent mechanism of cargo sorting during lysosome-phagosome fusion is controlled by Rab34. Proc Natl Acad Sci U S A. 2012;109(50):20485–90. https://doi.org/10.1073/pnas.1206811109
- Wang HJ, Gao Y, Chen L, et al. RAB34 was a progression- and prognosis-associated biomarker in gliomas. Tumour Biol. 2015;36(3):1573–8. https://doi.org/10.1007/s13277-014-2732-0
- Zougman A, Mann M, Wisniewski JR. Identification and characterization of a novel ubiquitous nucleolar protein ‘NARR’ encoded by a gene overlapping the rab34 oncogene. Nucleic Acids Res. 2011;39(16):7103–13. https://doi.org/10.1093/nar/gkr273
- Chen L, Hu J, Yun Y, et al. Rab36 regulates the spatial distribution of late endosomes and lysosomes through a similar mechanism to Rab34. Mol Membr Biol. 2010;27(1):23–30. https://doi.org/10.3109/09687680903417470
- Matsui T, Ohbayashi N, Fukuda M. The Rab interacting lysosomal protein (RILP) homology domain functions as a novel effector domain for small GTPase Rab36: Rab36 regulates retrograde melanosome transport in melanocytes. J Biol Chem. 2012;287(34):28619–31. https://doi.org/10.1074/jbc.M112.370544
- Mori T, Fukuda Y, Kuroda H, et al. Cloning and characterization of a novel Rab-family gene, Rab36, within the region at 22q11.2 that is homozygously deleted in malignant rhabdoid tumors. Biochem Biophys Res Commun. 1999;254(3):594–600. https://doi.org/10.1006/bbrc.1998.9968
- Ljubicic S, Bezzi P, Brajkovic S, et al. The GTPase Rab37 Participates in the Control of Insulin Exocytosis. PLoS One. 2013;8(6):e68255. https://doi.org/10.1371/journal.pone.0068255
- Masuda ES, Luo Y, Young C, et al. Rab37 is a novel mast cell specific GTPase localized to secretory granules. FEBS Lett. 2000;470(1):61–4. https://doi.org/10.1016/S0014-5793(00)01288-6
- Mori R, Ikematsu K, Kitaguchi T, et al. Release of TNF-alpha from macrophages is mediated by small GTPase Rab37. Eur J Immunol. 2011;41(11):3230–9. https://doi.org/10.1002/eji.201141640
- Tsai CH, Cheng HC, Wang YS, et al. Small GTPase Rab37 targets tissue inhibitor of metalloproteinase 1 for exocytosis and thus suppresses tumour metastasis. Nat Commun. 2014;5:4804. https://doi.org/10.1038/ncomms5804
- Xu X, Guan X, Tao H, et al. An association study on genetic polymorphisms of Rab37 gene with the risk of esophageal squamous cell carcinoma in a Chinese Han population. Int J Med Sci. 2013;10(3):235–42. https://doi.org/10.7150/ijms.5524
- Di Pietro SM, Dell'Angelica EC. The cell biology of Hermansky-Pudlak syndrome: recent advances. Traffic. 2005;6(7):525–33. https://doi.org/10.1111/j.1600-0854.2005.00299.x
- Osanai K, Higuchi J, Oikawa R, et al. Altered lung surfactant system in a Rab38-deficient rat model of Hermansky-Pudlak syndrome. Am J Physiol Lung Cell Mol Physiol. 2010;298(2):L243–51. https://doi.org/10.1152/ajplung.00242.2009
- Becker CE, Creagh EM, O'Neill LA. Rab39a binds caspase-1 and is required for caspase-1-dependent interleukin-1beta secretion. J Biol Chem. 2009;284(50):34531–7. https://doi.org/10.1074/jbc.M109.046102
- Chen T, Han Y, Yang M, et al. Rab39, a novel Golgi-associated Rab GTPase from human dendritic cells involved in cellular endocytosis. Biochem Biophys Res Commun. 2003;303(4):1114–20. https://doi.org/10.1016/S0006-291X(03)00482-0
- Gambarte Tudela J, Capmany A, Romao M, et al. The late endocytic Rab39a GTPase regulates the interaction between multivesicular bodies and chlamydial inclusions. J Cell Sci. 2015;128(16):3068–81. https://doi.org/10.1242/jcs.170092
- Seto S, Sugaya K, Tsujimura K, et al. Rab39a interacts with phosphatidylinositol 3-kinase and negatively regulates autophagy induced by lipopolysaccharide stimulation in macrophages. PLoS One. 2013;8(12):e83324. https://doi.org/10.1371/journal.pone.0083324
- Cheng H, Ma Y, Ni X, et al. Isolation and characterization of a human novel RAB (RAB39B) gene. Cytogenet Genome Res. 2002;97(1-2):72–5. https://doi.org/10.1159/000064047
- Corbier C, Sellier C. C9ORF72 is a GDP/GTP exchange factor for Rab8 and Rab39 and regulates autophagy. Small GTPases. 2016;1–6.
- Lesage S, Bras J, Cormier-Dequaire F, et al. Loss-of-function mutations in RAB39B are associated with typical early-onset Parkinson disease. Neurol Genet. 2015;1(1):e9. https://doi.org/10.1212/NXG.0000000000000009
- Tang BL. Rabs, Membrane Dynamics, and Parkinson's Disease. J Cell Physiol. 2017;232(7):1626–33. https://doi.org/10.1002/jcp.25713
- Wilson GR, Sim JC, McLean C, et al. Mutations in RAB39B cause X-linked intellectual disability and early-onset Parkinson disease with alpha-synuclein pathology. Am J Hum Genet. 2014;95(6):729–35. https://doi.org/10.1016/j.ajhg.2014.10.015
- Lee RH, Iioka H, Ohashi M, et al. XRab40 and XCullin5 form a ubiquitin ligase complex essential for the noncanonical Wnt pathway. EMBO J. 2007;26(15):3592–606. https://doi.org/10.1038/sj.emboj.7601781
- Pereira-Leal JB, Seabra MC. The mammalian Rab family of small GTPases: definition of family and subfamily sequence motifs suggests a mechanism for functional specificity in the Ras superfamily. J Mol Biol. 2000;301(4):1077–87. https://doi.org/10.1006/jmbi.2000.4010
- Bedoyan JK, Schaibley VM, Peng W, et al. Disruption of RAB40AL function leads to Martin–Probst syndrome, a rare X-linked multisystem neurodevelopmental human disorder. J Med Genet. 2012;49(5):332–40. https://doi.org/10.1136/jmedgenet-2011-100575
- Oldak M, Ruszkowska E, Pollak A, et al. A note of caution on the diagnosis of Martin-Probst syndrome by the detection of the p.D59G mutation in the RAB40AL gene. Eur J Pediatr. 2015;174(5):693–6. https://doi.org/10.1007/s00431-014-2452-x
- Jacob A, Jing J, Lee J, et al. Rab40b regulates trafficking of MMP2 and MMP9 during invadopodia formation and invasion of breast cancer cells. J Cell Sci. 2013;126(Pt 20):4647–58. https://doi.org/10.1242/jcs.126573
- Jacob A, Linklater E, Bayless BA, et al. The role and regulation of Rab40b-Tks5 complex during invadopodia formation and cancer cell invasion. J Cell Sci. 2016;129(23):4341–53. https://doi.org/10.1242/jcs.193904
- Li Y, Jia Q, Wang Y, et al. Rab40b upregulation correlates with the prognosis of gastric cancer by promoting migration, invasion, and metastasis. Med Oncol. 2015;32(4):126. https://doi.org/10.1007/s12032-015-0562-6
- Rodriguez-Gabin AG, Almazan G, Larocca JN. Vesicle transport in oligodendrocytes: probable role of Rab40c protein. J Neurosci Res. 2004;76(6):758–70. https://doi.org/10.1002/jnr.20121
- Tan R, Wang W, Wang S, et al. Small GTPase Rab40c associates with lipid droplets and modulates the biogenesis of lipid droplets. PLoS One. 2013;8(4):e63213. https://doi.org/10.1371/journal.pone.0063213
- Yang Q, Jie Z, Cao H, et al. Low-level expression of let-7a in gastric cancer and its involvement in tumorigenesis by targeting RAB40C. Carcinogenesis. 2011;32(5):713–22. https://doi.org/10.1093/carcin/bgr035
- Liu S, Hunt L, Storrie B. Rab41 is a novel regulator of Golgi apparatus organization that is needed for ER-to-Golgi trafficking and cell growth. PLoS One. 2013;8(8):e71886. https://doi.org/10.1371/journal.pone.0071886
- Kohnke M, Delon C, Hastie ML, et al. Rab GTPase prenylation hierarchy and its potential role in choroideremia disease. PLoS One. 2013;8(12):e81758. https://doi.org/10.1371/journal.pone.0081758
- Cox JV, Kansal R, Whitt MA. Rab43 regulates the sorting of a subset of membrane protein cargo through the medial Golgi. Mol Biol Cell. 2016;27(11):1834–44. https://doi.org/10.1091/mbc.E15-03-0123
- Dejgaard SY, Murshid A, Erman A, et al. Rab18 and Rab43 have key roles in ER-Golgi trafficking. J Cell Sci. 2008;121(Pt 16):2768–81. https://doi.org/10.1242/jcs.021808
- Haas AK, Yoshimura S, Stephens DJ, et al. Analysis of GTPase-activating proteins: Rab1 and Rab43 are key Rabs required to maintain a functional Golgi complex in human cells. J Cell Sci. 2007;120(Pt 17):2997–3010. https://doi.org/10.1242/jcs.014225
- Han MZ, Huang B, Chen AJ, et al. High expression of RAB43 predicts poor prognosis and is associated with epithelial-mesenchymal transition in gliomas. Oncol Rep. 2017;37(2):903–12. https://doi.org/10.3892/or.2017.5349
- Kretzer NM, Theisen DJ, Tussiwand R, et al. RAB43 facilitates cross-presentation of cell-associated antigens by CD8alpha+ dendritic cells. J Exp Med. 2016;213(13):2871–83. https://doi.org/10.1084/jem.20160597
- Srikanth S, Woo JS, Gwack Y. A large Rab GTPase family in a small GTPase world. Small GTPases. 2017;8(1):43–8. https://doi.org/10.1080/21541248.2016.1192921
- Nakamura S, Takemura T, Tan L, et al. Small GTPase RAB45-mediated p38 activation in apoptosis of chronic myeloid leukemia progenitor cells. Carcinogenesis. 2011;32(12):1758–72. https://doi.org/10.1093/carcin/bgr205
- Shintani M, Tada M, Kobayashi T, et al. Characterization of Rab45/RASEF containing EF-hand domain and a coiled-coil motif as a self-associating GTPase. Biochem Biophys Res Commun. 2007;357(3):661–7. https://doi.org/10.1016/j.bbrc.2007.03.206
- Liew GM, Ye F, Nager AR, et al. The intraflagellar transport protein IFT27 promotes BBSome exit from cilia through the GTPase ARL6/BBS3. Dev Cell. 2014;31(3):265–78. https://doi.org/10.1016/j.devcel.2014.09.004