ABSTRACT
The Ras homologous (Rho) protein family of GTPases (RhoA, RhoB and RhoC) are the members of the Ras superfamily and regulate cellular processes such as cell migration, proliferation, polarization, adhesion, gene transcription and cytoskeletal structure. Rho GTPases function as molecular switches that cycle between GTP-bound (active state) and GDP-bound (inactive state) forms. Leukaemia-associated RhoGEF (LARG) is a guanine nucleotide exchange factor (GEF) that activates RhoA subfamily GTPases by promoting the exchange of GDP for GTP. LARG is selective for RhoA subfamily GTPases and is an essential regulator of cell migration and invasion. Here, we describe the mechanisms by which LARG is regulated to facilitate the understanding of how LARG mediates functions like cell motility and to provide insight for better therapeutic targeting of these functions.
Introduction and LARG/ARHGEF12 structure
Small GTPases function as membrane-tethered molecular switches that cycle between GTP-bound (active state) and GDP-bound (inactive state) forms. GTP-bound forms of GTPases have an altered conformation that promotes the interaction and activation of effector proteins that can have diverse downstream effects on functions including the regulation of proliferation, migration and transcription. Small GTPases require guanine nucleotide exchange factors (GEFs) to promote the exchange of GDP for GTP, and also they require GTPase-activating proteins (GAPs) to facilitate their intrinsic GTPase enzymatic activity. The Ras homologous (Rho) protein family of GTPases (RhoA, RhoB and RhoC) are the members of the Ras superfamily of small GTPases and regulate cellular processes such as cell migration, proliferation, polarization, adhesion, gene transcription and cytoskeletal structure. Leukaemia-associated RhoGEF (LARG; also known as ARHGEF12) is a GEF that activates RhoA subfamily GTPases. LARG has been shown to play important roles in the progression of several diseases, such as cancer, pulmonary artery hypertension and pathogenic thrombus formation through the activation of RhoA [Citation1–6]. These functions have been identified in platelets, in vascular smooth muscle, and in tumour cells ().
Figure 1. LARG and RhoA Downstream Effectors Mediating Cell Motility. ROCK, Rho-associated protein kinase, mDia, Diaphanous-related formin-1; LIMK, LIM kinases; MLC, Myosin Light Chain.
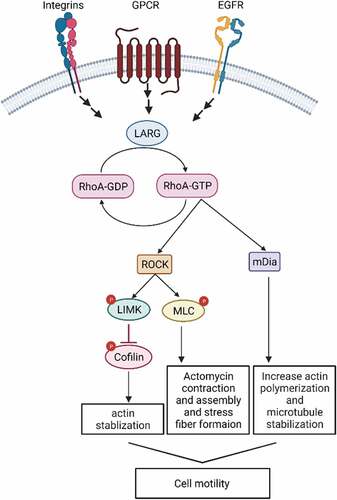
LARG/ARHGEF12 was first identified in acute myeloid leukaemia as a novel mixed-lineage leukaemia fusion partner [Citation7]. The ARHGEF12 gene is located on chromosome 11 and encodes a 1544 amino acid protein with a molecular mass of 173 kDa [Citation8; Citation7]. LARG is a RhoA subfamily-selective RhoGEF and a member of the RH-RhoGEF subfamily, which consists of the three members LARG, PDZ-RhoGEF (PRG) and p115RhoGEF. It is referred to as a RH-RhoGEF due to the presence of a Regulators of G protein Signalling (RGS) homology (RH) domain that is localized at the N-terminal region of LARG () [Citation9,Citation10]. Upon activation of G protein-coupled receptors, the RGS domain interacts with the small G proteins Gα12 and Gα13, which, in turn, activates LARG GEF activity [Citation9,Citation11,Citation12]. The LARG N-terminal domain contains a PDZ (post-synaptic density 95; disk large, zona occludens-1) [Citation13] domain that mediates protein–protein interactions and typically recognizes and binds to short peptide motifs at the carboxyl termini within the target protein [Citation14]. The LARG C-terminal domain contains several conserved regions including a Dbl homology (DH) domain that is responsible for GEF activity followed by a pleckstrin homology (PH) domain (). The DH domain of LARG contains a novel N-terminal extension, which consists of two short helices (residues 733–781) [Citation11]. This extension interacts directly with RhoA and is required for its guanine exchange activity, and the structures that determine LARG specificity for RhoA are primarily within the DH domain [Citation11]. Importantly, in most Rho GEFs, the PH domain regulates GEF activity through direct contact with the Rho GTPase that leads to stabilization of the DH domain and GEF activity [Citation15–17]. In addition, the PH domain is involved in RhoGEF membrane localization [Citation18–21] and in mediating subcellular localization by targeting some RhoGEFs to the cytoskeleton. For example, localization of Dbl family member Lfc to microtubules and Lbc along actin stress fibres where numerous RhoA downstream effectors are found [Citation22,Citation23]. In LARG, the PH domain has been proposed to play an important role in stabilizing the active conformational structure that promotes RhoA binding [Citation11]. Mutational analysis of the PH domain determined that mutations at Ser-1118 and Glu-1023 residues reduce its guanine exchange activity [Citation11]. The Ser-1118 residue of the LARG PH domain interacts with RhoA-Glu-97 whereas Glu-1023, Arg-986 and Asn-983 interact with RhoA-Arg-68. A salt bridge within the PH domain of LARG is formed through Arg-986 and Glu-1023 [Citation11]. In addition, LARG’s PH domain may mediate an interaction with other regulatory proteins or other domains of LARG through its exposed hydrophobic patch (L-1086, F-1098, I-1100, A-1107 and I-1109) [Citation11]. Finally, LARG contains a coiled-coil sequence at the C-terminus (residues 1507–1520) that controls LARG localization and oligomerization [Citation24,Citation25] (see ).
Figure 2. Domain structure of LARG and its regulation. Phosphorylation and sequences important for the regulation of LARG activation and localization are indicated. NLS, nuclear localization signal; PDZ, post-synaptic density 95; disk large, zona occludens-1; RH, RGS homology; DH, Dbl homology; PH, Pleckstrin homology; CC, coiled-coil domain.
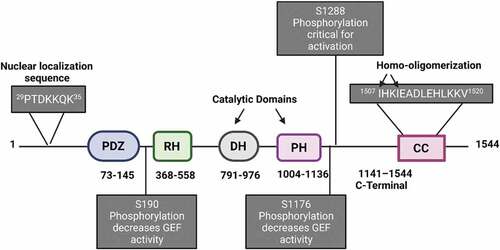
When LARG GEF activity is stimulated, it binds to and activates the RhoA subfamily GTPases, RhoA, RhoB or RhoC. RhoA subfamily GTPases are members of the Rho-family GTPases that mediate signals from several membrane receptors including G protein coupled receptors (GPCRs) [Citation8], growth factor receptors [Citation26], tyrosine kinase receptors and cell-matrix adhesion molecules such as integrin receptors [Citation27]. However, the mechanism by which LARG activates Rho GTPases has not been fully elucidated. As with all GTPases, RhoA proteins cycle between their active GTP-bound state and inactive GDP-bound state. RhoA activation is positively regulated by GEFs that catalyse the release of GDP allowing GTP to bind and activate RhoA. Activated RhoA then interacts with downstream effectors (e.g. the formin family proteins (mDia1), mitogen-activated protein kinase kinase kinase (MEKK1), and Rho-associated protein kinase (or ROCK) recruiting them to specific cellular membranes (see ). This interaction initiates signalling events necessary for controlling cell migration, proliferation, polarization, adhesion, gene transcription and cytoskeletal structure [Citation28; Citation25, Citation27, Citation29]. LARG DH and PH domains are the catalytic domains that interact with the Rho GTPases and mediate the GEF activity [Citation11]. Atomic structures of these LARG catalytic domains alone and in complex with RhoA demonstrate that RhoA directly binds to these domains to induce a conformational change [Citation11].
LARG Oligomerization and Sub-Cellular Localization
The LARG N-terminus contains a nuclear localization sequence (29PTDKKQK35), which is responsible for its nuclear import. Another important structural feature of LARG is a coiled-coil sequence (1507IHKIEADLEHLKKV1520) at the extreme end of the C-terminus that controls LARG cytoplasmic retention and oligomerization [Citation24]. Chikumi and colleagues demonstrated that the LARG C-terminal region (amino acids 1141–1544) regulates the LARG’s GEF activity. Deletion of LARG’s C-terminus increases its GEF activity by inhibiting the formation of homo/hetero LARG oligomers and complex formation [Citation9]. However, this group did not identify, which sequence within the C-terminus mediates oligomerization. A later study investigated the structural requirements for LARG oligomerization and localization [Citation24]. The formation of a LARG oligomer complex is mediated by its coiled-coil domain (amino acids 1507–1520) at the C-terminus (). Indeed, substitution of isoleucines 1507/1510 with alanines blocks homo-oligomerization. However, these substitutions did not affect LARG guanine nucleotide exchange activity, suggesting that an additional event occurs at the C-terminus to regulate its GEF activity. Therefore, LARG oligomerization and its GEF activity are regulated separately through LARG’s C-terminus.
Disrupting oligomerization is important for LARG localization as LARG localizes in the nucleus upon the deletion of the C-terminal domain or when oligomerization is otherwise inhibited [Citation24]. In contrast, full length LARG or mutants that induce dimerization accumulate in the cytoplasm where RhoA is mainly located [Citation24,Citation30]. Furthermore, substitution of isoleucines 1507/1510 with alanines leads to nucleo-cytoplasmic shuttling by the chromosome region maintenance 1 (CRM1) nuclear transport pathway. Thus, oligomerization must be disrupted for LARG to be translocated into the nucleus; a process driven by LARG’s nuclear localization sequence. The presence of LARG, RhoA and mDia2 in the nucleus increases their ability to promote myocardin-related transcription factor (MRTF) activity. Nuclear LARG significantly induced MRTF-B nuclear localization and smooth muscle cell (SMC)-specific gene transcription. The nuclear localization of mDia2, which is a downstream of RhoA, increases nuclear actin polymerization and induces accumulation of MRTF in the nucleus, which, in turn, induces SMC-specific transcription [Citation31]. Interestingly, expression of activated Gα13 promotes translocation to the plasma membrane of both full length LARG or a LARG C-terminal deletion mutant. These findings suggest that LARG’s C-terminal domain may function as a cytoplasmic retention signal and LARG localization to either cytoplasm, nucleus or plasma membrane may regulate specific signalling responses [Citation24].
LARG Activation
An initial step in LARG GEF activation is the recruitment of LARG to plasma membrane-associated proteins. These include receptors such as insulin-like growth factor-1 receptor, Plexin B or LPA-1/2 that recruits LARG through binding to the LARG N-terminal PDZ domain [Citation26,Citation32] as well as G protein-coupled receptors (GPCRs). Upon LARG recruitment to the plasma membrane, it can be activated by several mechanisms. Stimulation by GPCR ligands (such as thrombin, lysophosphatidic acid, sphingosine-1-phosphate and thromboxane A2) promotes the binding of the small G proteins Ga12 and Ga13 to LARG’s RH domain, which stimulates LARG guanine exchange activity and thereby activates the RhoA subfamily GTPases. Thus, LARG provides a direct link from these heterotrimeric G proteins to RhoA subfamily GTPases [Citation9,Citation33] ().
Figure 3. LARG activation and regulation. primary pathways of LARG activation in cell migration and invasion are depicted. Upon GPCR, EGFR/integrin binding to cognate ligands LARG can be activated by either Gα proteins or ERK/RSK respectively. Not shown are undefined tyrosine phosphorylations in the c-terminus that can also mediate activation via FAK or Fyn kinases. Dia1 can alternatively activate LARG GEF activity by binding to the coiled-coil region.
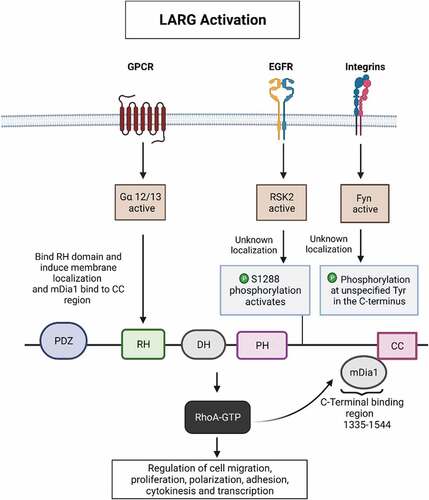
Alternatively, LARG can be activated by Diaphanous-related formin (DRF), or Dia1, which is a GTPase binding protein involved in cell movement, cytokinesis and polarity that are deregulated during metastasis and tumour cell transformation [Citation34–36]. Dia1 associates specifically with LARG and not other members of RH-RhoGEF subfamily. Indeed, Dia1 co-Immunoprecipitates LARG but not PRG or p115RhoGEF. Upon LPA stimulation, active RhoA binds to the Dia1 N-terminus promoting the release of Dia1 autoinhibition. This allows for the Dia1 FH2 domain to bind the C-terminus of LARG and directly stimulate its GEF activity. A LARG, RhoA and Dia1 complex regulates cell invasion through a positive feedback loop [Citation2]. LARG can also be activated by insulin-like growth factor 1 (IGF-1) receptor or Plexin-B1, which binds the LARG PDZ domain [Citation26,Citation37,Citation38]. CD44, a major hyaluronan receptor, can also interact with LARG to activate RhoA signalling [Citation39].
In addition to activation by GPCRs and Dia1, LARG can be activated by growth factor receptors such as Epidermal Growth Factor Receptor (EGFR) or by integrins in response to ligand binding and tension (see ) [Citation3,Citation26,Citation27,Citation37,Citation38]. EGFR and integrins both activate the ERK MAP kinase pathway, and in particular, the ERK substrate p90 Ribosomal S6 kinases (RSKs). In response to upstream activation of EGFR, ERK phosphorylates and thereby activates RSK in many cell types including GBM and human embryonic kidney cells [Citation3,Citation40]. Activated RSK2 then phosphorylates LARG at a recently identified site (serine 1288) that is in the C-terminal (FPRYRTA1288SQGPQTDS) domain, which leads by an unknown mechanism to the activation of RhoA [Citation3]. Integrins can alternatively activate LARG via tyrosine phosphorylation.
Phosphorylation Regulates LARG Nucleotide Exchange Activity
Phosphorylation is a common post-translational modification that regulates RhoGEF localization, interaction and activity. Indeed, phosphorylation at serine and tyrosine residues may either positively or negatively regulate LARG activity. For example, thrombin-mediated activation of GPCRs induces activation of focal adhesion kinase (FAK), which promotes tyrosine phosphorylation in the LARG C-terminal domain at a heretofore unspecified site leading to increased RhoA activation. Deletion of the LARG C-terminal domain inhibits FAK-mediated tyrosine phosphorylation of LARG. Integrin activation by mechanotransduction increases LARG phosphorylation through the Src family tyrosine kinase Fyn and promotes its subsequent recruitment to focal adhesion complexes [Citation37]. Mechanotransduction mediates increased LARG tyrosine phosphorylation even when FAK-mediated signalling is inhibited [Citation37] suggesting other signalling proteins may be involved in regulating LARG phosphorylation. However, the detailed mechanism by which tyrosine kinases regulate LARG activation remains undefined [Citation10].
Recently, RSK2 was shown to phosphorylate serine 1288 (S1288) in the LARG C-terminal domain. This phosphorylation is required for LARG activation downstream of EGFR activation and subsequent activation of RhoA [Citation3]. However, neither EGF nor the activated form of RSK2 can activate RhoA when LARG S1288 is substituted with alanine, indicating that S1288 is required for LARG-mediated activation of RhoA [Citation3]. Integrin cell surface receptors play an important role in controlling cell adhesion and migration and activate both FAK and the ERK/MAP kinase pathway upon binding to extracellular matrix ligands such as fibronectin [Citation41,Citation42]. Integrin activation of ERK and subsequently RSK2, is therefore an alternative potential way for integrins to activate LARG through phosphorylation at S1288 [Citation3,Citation43]. In contrast, phosphorylation of the LARG N-terminal S190 and C-terminal at S1176 by cyclin-dependent kinase 1 leads to LARG inactivation [Citation44].
There are several ways that phosphorylation may regulate LARG activity. One is by inducing a conformational change within the PH/DH domains (the catalytic domains). Another is by inhibiting or stimulating the formation of the LARG oligomeric complex or otherwise affecting localization. Finally, phosphorylation may interfere with LARG’s ability to bind to RhoA or other regulators that are required for RhoA activation. In addition, ubiquitination may also play a role in regulating nucleotide exchange activity of LARG. For example, deubiquitination of LARG by cylindromatosis (CYLD) enhances LARG’s ability to activate RhoA [Citation45]. Although there is now significant accumulated evidence that the phosphorylation of several sites on LARG can control its activity, the precise mechanism by which phosphorylation affects LARG function still remains to be determined.
LARG Physiological Function
LARG has functions in many pathologies through its effects on the actin cytoskeleton. These functions have been identified in platelets, in vascular smooth muscle and in cancer. LARG is one of the top 4% of highly expressed GEFs in platelets, which are important for normal haemostasis and cancer metastasis [Citation46,Citation47]. Platelet production is a result of megakaryocytes cytoplasmic fragmentation [Citation48]. LARG is required for human megakaryocyte maturation as LARG knockdown by lentiviral shRNA blocks megakaryocyte maturation. In addition, LARG promotes platelet aggregation through RhoA activation in response to thrombin and thromboxane. In platelets, LARG transduces signals from Gα subunits to RhoA that lead to RhoA activation. This suggests the inhibition of LARG may reduce the risk of pathogenic thrombus formation [Citation5,Citation6].
In addition, targeting LARG may have therapeutic potential for pulmonary hypertension. Under hypoxic conditions, proliferation and migration of pulmonary artery smooth muscle cells (PASMCs) is increased by upregulating the expression of miR-214-3p, which binds to the 3ʹ-untranslated region of LARG and increases LARG protein expression [Citation4]. LARG activates RhoA and RhoA plays a critical role in the development of pulmonary hypertension by regulating proliferation, migration and apoptosis of PASMCs [Citation4,Citation49]. Moreover, LARG is required for the final step of cytokinesis (abscission). LARG initially colocalizes at the spindle poles with α-tubulin, then localizes to the central spindle and is condensed and colocalized with RhoA in midbody during cytokinesis where it activates RhoA to promote actin contraction that drives cytokinesis. Depletion of LARG in HeLa cells results in increased apoptosis during cytokinesis with unresolved intercellular bridges [Citation50].
Invasion and metastasis are the primary cause of death for patients with cancer. Glioblastoma is the most common malignant brain tumour and can affect any age group. It is associated with poor survival and characterized by resistance to apoptosis, diffuse infiltration and uncontrolled cellular proliferation. The major challenge for effective glioblastoma treatment is the invasion of primary tumour cells into the surrounding normal tissue. Invaded cells are highly resistant to radiotherapy and chemotherapy that follow tumour resection with a high chance for tumour recurrence [Citation51]. Recently, it has become clear that LARG plays a major role in regulating glioblastoma cell migration and invasion [Citation1–3]. In these cells, LARG acts as a guanine nucleotide exchange factor for RhoA, B and C [Citation3,Citation52]. Even though Rho family members have few amino acid differences between their sequences, each Rho plays different roles in cancer cell motility [Citation53,Citation54]. Thus, each Rho family member regulates specific signalling pathways and collaborate in cell migration and remodelling [Citation55–58]. Therefore, the specific Rho GTPases activated by LARG can determine the effect that LARG has in those cells.
RSKs are implicated in metastasis and tumour cell growth in various cancers such as breast, lung and GBM [Citation59,Citation60]. In response to EGF stimulation, ERK activates RSK in many cell types including GBM and human embryonic kidney cells [Citation3,Citation40]. Activated RSK2 then phosphorylates LARG at serine 1288, which activates it [Citation3]. A dominant active mutation of RSK2 (T577E) increases RhoA activation and cell migration and invasion of a GBM-derived cell line. However, alanine mutations at LARG-S1288 impaired this increased cell migration and blocked RhoA activation in response to active RSK2 [Citation3]. Moreover, all RhoA isoforms and LARG were found to interact with RSK2, however RSK2-driven GBM cell migration and invasion relied on RhoA and partly RhoB. RhoC displays unusual function in driving migration that appears to act independently of RSK2 activity. A recent study reported that LARG, RhoA and RhoC protein levels increase in more invasive GBM, but LARG and RhoC expression levels were much higher than RhoA in the tumour core as well as in invading cells [Citation1]. This increased expression of LARG leads to the activation of both RhoA and RhoC. However, RhoC appears to be essential in regulating GBM cell invasion as depletion of RhoA increases cell migration whereas the depletion of RhoC inhibits cell migration [Citation1]. It is unclear how LARG acts distinctly in activating RhoA versus RhoC in the different GBM cells, and it remains to be determined if RSK2 affects RhoC activity. In addition to the regulation of invasion and migration, knockdown of LARG protein levels by siRNA significantly reduces GBM cell survival and increases these cells sensitivity to chemotherapy such as temozolomide [Citation1].
Conclusions and Future Directions
LARG is one of the guanine nucleotide exchange factors belonging to the RH-RhoGEF subfamily and has been associated with the regulation of cell motility, cell shape, cell proliferation and gene transcription. LARG plays an important function in the disease progression of cancer, pulmonary hypertension and pathogenic thrombus formation . Metastasis, the process of spreading the cancer cells from the primary tumour to other sites within the body, is main cause of death for patients with cancer. The activation of RhoA, which is activated by LARG, has been associated with human cancer development and progression through its effect on invasion, migration and metastasis. The deletion of LARG in cancer cells significantly reduces the activation of RhoA and therefore decreased invasion and migration. Thus, LARG regulates cell proliferation, migration, invasion and metastasis through its ability to catalyse the exchange of GDP for GTP on RhoA thereby activating RhoA and its downstream pathways. Given the effects of LARG in cancer cells and pulmonary artery smooth muscle cells motility, it is attractive to hypothesize that disrupting the interaction between LARG and RhoA may provide a new therapeutic approach in blocking migration, invasion and metastasis. To improve LARG targeting agents and to identify potential resistance mechanisms and biomarkers, a more detailed mechanistic understanding of LARG regulation and function in cancer, pulmonary hypertension, and thrombus formation are required.
Figure 4. LARG Physiological Function. Depicted are known physiological functions of LARG/ARHGEF12 and potential therapeutic value of targeting LARG in each.
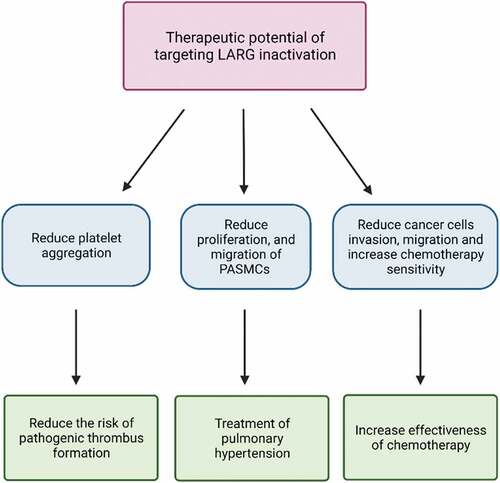
There has been progress in developing inhibitors of GEFs, including LARG, thereby making it an exciting new target [Citation61,Citation62]. For LARG, the Y16 small molecule targets the DH-PH domain junction and thereby suppresses LARG activation of RhoA in a dose-dependent manner with an 80 nM Kd [Citation61,62]. The analysis of LARG activation and activity suggest other potential ways of targeting LARG. The LARG C-terminal domain plays important roles in LARG localization, oligomerization and activation. Disruption of this may be a useful approach. LARG phosphorylation at S1288, which is located at the C-terminus, by RSK2 is required for LARG GEF activity in some cells. Other phosphorylation sites and post-translational modifications have been detected on LARG (www.phosphosite.org) including methylation, ubiquitylation and acetylation and as yet remain uncharacterized. Inhibitors of the kinases, methylases, ubiquitinases and acetylases that mediate these may be additional targets upstream. Thus, further analysis of how LARG is regulated and how it functions at the molecular level may provide viable novel targets for abrogating cancer metastasis and for treating other LARG-dependent pathologies.
Attribution of Authorship
N.Z.G. compiled relevant works and wrote the initial draft. N.Z.G., M.L.M. and J.W.R. wrote and revised the manuscript.
Disclosure statement
No potential conflict of interest was reported by the authors.
Additional information
Funding
References
- Ding Z, Dong Z, Yang Y, et al. Leukemia-Associated Rho Guanine Nucleotide Exchange Factor and Ras Homolog Family Member C Play a Role in Glioblastoma Cell Invasion and Resistance. Am J Pathol. 2020;190(10):2165–2176.
- Kitzing TM, Sahadevan AS, Brandt DT, et al. Positive feedback between Dia1, LARG, and RhoA regulates cell morphology and invasion. Genes Dev. 2007;21(12):1478–1483.
- Shi G-X, Yang WS, Jin L, et al. RSK2 drives cell motility by serine phosphorylation of LARG and activation of Rho GTPases. Proc Natl Acad Sci U S A. 2018a;115(2):E190–E199.
- Xing XQ, Li B, Xu S-L, et al. MicroRNA-214-3p Regulates Hypoxia-Mediated Pulmonary Artery Smooth Muscle Cell Proliferation and Migration by Targeting ARHGEF12. Med Sci Monit. 2019;25:5738–5746.
- Zou S, Teixeira AM, Sanada CD, et al. ARHGEF12 Is Essential for Human Megakaryocyte Differentiation and Plays Critical Roles in Platelet Function. Blood. 2014;124(21):341.
- Zou S, Teixeira AM, Yin M, et al. Leukaemia-associated Rho guanine nucleotide exchange factor (LARG) plays an agonist specific role in platelet function through RhoA activation. Thromb Haemost. 2016;116(3):506–516.
- Kourlas PJ, Strout MP, Becknell B, et al. Identification of a gene at 11q23 encoding a guanine nucleotide exchange factor: evidence for its fusion with MLL in acute myeloid leukemia. Proc Natl Acad Sci U S A. 2000;97(5):2145–2150.
- Fukuhara S, Chikumi H, Gutkind JS. RGS-containing RhoGEFs: the missing link between transforming G proteins and Rho? Oncogene. 2001;20(13):1661–1668.
- Chikumi H, Barac A, Behbahani B, et al. Homo- and hetero-oligomerization of PDZ-RhoGEF, LARG and p115RhoGEF by their C-terminal region regulates their in vivo Rho GEF activity and transforming potential. Oncogene. 2004;23(1):233–240.
- Chikumi H, Fukuhara S, Gutkind JS. Regulation of G protein-linked guanine nucleotide exchange factors for Rho, PDZ-RhoGEF, and LARG by tyrosine phosphorylation: evidence of a role for focal adhesion kinase. J Biol Chem. 2002;277(14):12463–12473.
- Kristelly R, Gao G, Tesmer JJG. Structural determinants of RhoA binding and nucleotide exchange in leukemia-associated Rho guanine-nucleotide exchange factor. J Biol Chem. 2004;279(45):47352–47362.
- O’Brien M, Flynn D, Mullins B, et al. Expression of RHOGTPase regulators in human myometrium. ReprodBiol Endocrinol. 2008;6:1.
- Fanning AS, Anderson JM. Protein–protein interactions: PDZ domain networks. Curr Biol. 1996;6(11):1385–1388.
- Saras J, Heldin C-H. PDZ domains bind carboxy-terminal sequences of target proteins. Trends Biochem Sci. 1996;21(12):455–458.
- Bellanger J-M, Estrach S, Schmidt S, et al. Different regulation of the Trio Dbl-Homology domains by their associated PH domains. Biol Cell. 2003;95(9):625–634.
- Nimnual AS, Yatsula BA, Bar-Sagi D. Coupling of Ras and Rac guanosine triphosphatases through the Ras exchanger Sos. Science. 1998;279(5350):560–563.
- Rossman KL, Worthylake DK, Snyder JT, et al. A crystallographic view of interactions between Dbs and Cdc42: PH domain-assisted guanine nucleotide exchange. EMBO J. 2002;21(6):1315–1326.
- Fuentes EJ, Karnoub AE, Booden MA, et al. Critical Role of the Pleckstrin Homology Domain in Dbs Signaling and Growth Regulation*. J Biol Chem. 2003;278(23):21188–21196.
- Skowronek KR, Guo F, Zheng Y, et al. The C-terminal Basic Tail of RhoG Assists the Guanine Nucleotide Exchange Factor Trio in Binding to Phospholipids*. J Biol Chem. 2004;279(36):37895–37907.
- Whitehead IP, Lambert QT, Glaven JA, et al. Dependence of Dbl and Dbs Transformation on MEK and NF-B Activation. Mol Cell Biol. 1999;19:12.
- Zheng Y, Zangrilli D, Cerione RA, et al. The Pleckstrin Homology Domain Mediates Transformation by Oncogenic Dbl through Specific Intracellular Targeting*. J Biol Chem. 1996;271(32):19017–19020.
- Glaven JA, Whitehead I, Bagrodia S, et al. The Dbl-related Protein, Lfc, Localizes to Microtubules and Mediates the Activation of Rac Signaling Pathways in Cells*. J Biol Chem. 1999;274(4):2279–2285.
- Olson MF, Sterpetti P, Nagata K, et al. Distinct roles for DH and PH domains in the Lbc oncogene. Oncogene. 1997;15(23):2827–2831.
- Grabocka E, Wedegaertner PB. Disruption of oligomerization induces nucleocytoplasmic shuttling of leukemia-associated rho Guanine-nucleotide exchange factor. Mol Pharmacol. 2007;72(4):993–1002.
- Patel M, Karginov AV. Phosphorylation-mediated regulation of GEFs for RhoA. Cell Adh Migr. 2014;8(1):11–18.
- Taya S, Inagaki N, Sengiku H, et al. Direct interaction of insulin-like growth factor-1 receptor with leukemia-associated RhoGEF. J Cell Biol. 2001;155(5):809–820.
- Mikelis CM, Palmby TR, Simaan M, et al. PDZ-RhoGEF and LARG are essential for embryonic development and provide a link between thrombin and LPA receptors and Rho activation. J Biol Chem. 2013;288(17):12232–12243.
- Gallagher ED, Gutowski S, Sternweis PC, et al. RhoA Binds to the Amino Terminus of MEKK1 and Regulates Its Kinase Activity*. J Biol Chem. 2004;279(3):1872–1877.
- Jaffe AB, Hall A. RHO GTPASES: biochemistry and Biology. Annu Rev Cell Dev Biol. 2005;21(1):247–269.
- Michaelson D, Silletti J, Murphy G, et al. Differential localization of Rho GTPases in live cells: regulation by hypervariable regions and RhoGDI binding. J Cell Biol. 2001;152(1):111–126.
- Staus DP, Weise-Cross L, Mangum KD, et al. Nuclear RhoA signaling regulates MRTF-dependent SMC-specific transcription. Am J Physiol Heart Circ Physiol. 2014;307(3):H379–390.
- Yamada T, Ohoka Y, Kogo M, et al. Physical and functional interactions of the lysophosphatidic acid receptors with PDZ domain-containing Rho guanine nucleotide exchange factors (RhoGEFs). J Biol Chem. 2005;280(19):19358–19363.
- Yu OM, Brown JH. G Protein-Coupled Receptor and RhoA-Stimulated Transcriptional Responses: links to Inflammation, Differentiation, and Cell Proliferation. Mol Pharmacol. 2015;88(1):171–180.
- Faix J, Grosse R. Staying in Shape with Formins. Dev Cell. 2006;10(6):693–706.
- Gomez TS, Kumar K, Medeiros RB, et al. Formins regulate the actin-related protein 2/3 complex-independent polarization of the centrosome to the immunological synapse. Immunity. 2007;26(2):177–190.
- Goode BL, Eck MJ. Mechanism and function of formins in the control of actin assembly. Annu Rev Biochem. 2007;76(1):593–627.
- Guilluy C, Swaminathan V, Garcia-Mata R, et al. The Rho GEFs LARG and GEF-H1 regulate the mechanical response to force on integrins. Nat Cell Biol. 2011;13(6):722–727.
- Swiercz JM, Kuner R, Behrens J, et al. Plexin-B1 directly interacts with PDZ-RhoGEF/LARG to regulate RhoA and growth cone morphology. Neuron. 2002;35(1):51–63.
- Bourguignon LYW, Gilad E, Brightman A, et al. Hyaluronan-CD44 interaction with leukemia-associated RhoGEF and epidermal growth factor receptor promotes Rho/Ras co-activation, phospholipase C epsilon-Ca2+ signaling, and cytoskeleton modification in head and neck squamous cell carcinoma cells. J Biol Chem. 2006;281(20):14026–14040.
- Kang S, Dong S, Guo A, et al. Epidermal growth factor stimulates RSK2 activation through activation of the MEK/ERK pathway and src-dependent tyrosine phosphorylation of RSK2 at Tyr-529. J Biol Chem. 2008;283(8):4652–4657.
- Juliano RL, Reddig P, Alahari S, et al. Integrin regulation of cell signalling and motility. Biochem Soc Trans. 2004;32(3):443–446.
- Knapik DM, Perera P, Nam J, et al. Mechanosignaling in bone health, trauma and inflammation. Antioxid Redox Signal. 2014;20(6):970–985.
- Hauge C, Frodin M. RSK and MSK in MAP kinase signalling. J Cell Sci. 2006;119(15):3021–3023.
- Helms MC, Grabocka E, Martz MK, et al. Mitotic-dependent phosphorylation of leukemia-associated RhoGEF (LARG) by Cdk1. Cell Signal. 2016;28(1):43–52.
- Yang Y, Sun L, Tala G, et al. CYLD regulates RhoA activity by modulating LARG ubiquitination. PloS One. 2013;8(2):e55833.
- Karpatkin S, Pearlstein E. Role of Platelets in Tumor Cell Metastases. Ann Intern Med. 1981;95(5):636–641.
- Simon LM, Edelstein LC, Nagalla S, et al. Human platelet microRNA-mRNA networks associated with age and gender revealed by integrated plateletomics. Blood. 2014;123(16):e37–45.
- Bluteau D, Lordier L, Di Stefano A, et al. Regulation of megakaryocyte maturation and platelet formation. J Thromb Haemost. 2009;7(Suppl 1):227–234.
- Xing XQ, Gan Y, Wu S, et al. Statins may ameliorate pulmonary hypertension via RhoA/Rho-kinase signaling pathway. Med Hypotheses. 2006;68(5):1108–1113.
- Martz MK, Grabocka E, Beeharry N, et al. Leukemia-associated RhoGEF (LARG) is a novel RhoGEF in cytokinesis and required for the proper completion of abscission. Mol Biol Cell. 2013;24(18):2785–2794.
- Kesari S. Understanding glioblastoma tumor biology: the potential to improve current diagnosis and treatments. Semin Oncol. 2011;38(Suppl 4):S2–10.
- Jaiswal M, Gremer L, Dvorsky R, et al. Mechanistic insights into specificity, activity, and regulatory elements of the regulator of G-protein signaling (RGS)-containing Rho-specific guanine nucleotide exchange factors (GEFs) p115, PDZ-RhoGEF (PRG), and leukemia-associated RhoGEF (LARG). J Biol Chem. 2011;286(20):18202–18212.
- Ridley AJ. RhoA, RhoB and RhoC have different roles in cancer cell migration. J Microsc. 2013;251(3):242–249.
- Wheeler AP, Ridley AJ. Why three Rho proteins? RhoA, RhoB, RhoC, and cell motility. Exp Cell Res. 2004;301(1):43–49.
- Bravo-Cordero JJ, Sharma VP, Roh-Johnson M, et al. Spatial regulation of RhoC activity defines protrusion formation in migrating cells. J Cell Sci. 2013;126(15):3356–3369.
- Heasman SJ, Ridley AJ. Mammalian Rho GTPases: new insights into their functions from in vivo studies. Nature Reviews Molecular Cell Biology. 2008;9(9):690–701.
- Hirata E, Yukinaga H, Kamioka Y, et al. In vivo fluorescence resonance energy transfer imaging reveals differential activation of Rho-family GTPases in glioblastoma cell invasion. J Cell Sci. 2012;125(4):858–868.
- Pertz O. Spatio-temporal Rho GTPase signaling – where are we now? J Cell Sci. 2010;123(11):1841–1850.
- Sulzmaier FJ, Ramos JW. RSK Isoforms in Cancer Cell Invasion and Metastasis. Cancer Res. 2013;73(20):6099–6105.
- Sulzmaier FJ, Young-Robbins S, Jiang P, et al. RSK2 activity mediates glioblastoma invasiveness and is a potential target for new therapeutics. Oncotarget. 2016;7(48):79869–79884.
- Evelyn CR, Ferng T, Rojas RJ, et al. High-throughput screening for small-molecule inhibitors of LARG-stimulated RhoA nucleotide binding via a novel fluorescence polarization assay. J Biomol Screen. 2009;14(2):161–172.
- Shang X, Marchioni F, Evelyn CR, et al. Small-molecule inhibitors targeting G-protein-coupled Rho guanine nucleotide exchange factors. Proc Natl Acad Sci U S A. 2013;110(8):3155–3160.