Abstract
New approaches in probing for antimicrobial metabolites require genome and chemical investigation. The rapid identification of known compounds, i.e. dereplication, is strategically crucial in bioprospecting microbes for novel metabolites. An endophytic fungal strain, CBR-18, was isolated from Cryptolepis buchanani Roem. and identified as Phomopsis liquidambaris by molecular analysis. Biosynthetic polyketide synthase (PKS) genes of CBR-18 were investigated using three sets of degenerate primers, amplified with LC1–LC2c primers. Type I PKS gene-based and chemical investigation by a chromatography-guided approach furnished a broad-spectrum antimicrobial metabolite which was identified as oblongolide Y. Minimum inhibitory concentration values of the compound against test pathogens ranged between 25 and 100 µg/ml. The present study highlights the utility of strain CBR-18 as a promising source of potential polyketide antimicrobial agents which could be exploited for industrial purposes. This approach enabled different ketosynthase domains to be targeted for the isolation of previously uncharacterized bioactive polyketides, and could be used as a method for the rapid screening of fungal endophytes capable of producing potential polyketide antimicrobial agents.
Introduction
Although natural products from microbes contribute a broad spectrum of chemical diversity, increasing the diversity of natural compounds is still necessary to achieve enough new hits for drug discovery (Zhao Citation2011). Despite the present focus on synthetic products, natural products serve as a continuing source of novel bioactive metabolites, having an immense impact on modern medicine (Wang et al. Citation2012). Meanwhile, the emergence of antibiotic-resistant pathogens calls for inventive research and development strategies (Sadrati et al. Citation2013). Therefore, new advanced techniques are crucial to enhance the diversity of natural products for the discovery of therapeutic agents, not only to increase the efficacy and decrease the toxicity, but also to combat the emergence of drug resistance.
Accordingly, natural products and drugs derived from natural sources increasingly represent a significant proportion of newly developed drugs (Knight et al. Citation2003; Demain & Sanchez Citation2009; Newman & Cragg 2012). Antimicrobial metabolites from endophytic fungi isolated from higher plants are the major focus of natural products research. Bioactive natural metabolites produced by endophytic fungi may provide a promising therapeutic approach to address the problem of drug resistance by human pathogens and multidrug-resistant microorganisms. Endophytic fungi residing in medicinal plants can survive in extreme conditions such as drought, salinity, heat or cold, and with a variety of competingorganisms which live in their natural environments (Aly et al. Citation2010). These facts make endophytic microbial consortia from such environments promising targets in the search for potential antimicrobial metabolites (Rateb & Ebel Citation2011; Blunt et al. Citation2012; Debbab et al. Citation2012; Newman & Cragg Citation2012).
Advanced approaches to searching for bioactive secondary metabolites from microbes involve chemical and biological screening (Rakshith et al. Citation2013a). Polyketides constitute a diverse group of secondary metabolites that play an important role in drug discovery (Schumann & Hertweck Citation2006). They are widely used in human and animal drugs (antibacterials, e.g. erythromycin and tetracyclines; anticholesteraemics, e.g. statins; antiparasitics, e.g. avermictin; immunosuppressors, e.g. rapamycin; and antitumorals, e.g. daunorubicin and doxorubicin), and also used in agriculture and chemical industries, some of them having a high commercial value (Cox Citation2007; Scherlach and Hertweck Citation2009). Biosynthesis of fungal polyketides is governed by multidomain enzymes, which are termed type I polyketide synthases (PKS). The application of molecular screening methods may provide information to predict the nature of bioactive compounds during the bioprospecting of microbes.
Cryptolepis buchanani Roem. and Schult. is a climbing tree which has medicinal value in Ayurveda as an anti-diarrhoeal, anti-inflammatory and blood-purifying agent (Kaul et al. Citation2003). In the course of an investigation into the dereplication of antimicrobial metabolites of polyketide biosynthetic origin from an endophytic fungus, an antimicrobial metabolite from strain CBR-18 was isolated and identified through the simultaneous implementation of genomic and chromatographic approaches.
Materials and methods
Source of endophytic fungus
Endophytic fungus was isolated from the stem of an invasive plant, C. buchanani Roem., collected in the campus of Mysore University, India. Collected tissue samples were sealed with parafilm to maintain the endophytic nature. Samples were brought to the laboratory for isolation of the endophytic fungus within 24 h.
Surface sterilization and isolation of endophytic fungus
The tissue samples were washed in running tap water followed by double-distilled water before processing. To remove the epiphytic microorganisms, samples were rinsed with 70% ethanol for 2 min, surface sterilized with sodium hypochlorite (4%) for 5 min and again rinsed with 70% ethanol for 30 s. Later, the samples were rinsed with sterile double-distilled water and left for surface drying. To confirm the success of the surface disinfection process, aliquots of the sterile distilled water from the final rinse were inoculated on the isolation media plates. The plant material was cut into small segments (5 mm size) and placed on water agar plates (distilled water, 1.5% agar) amended with chloramphenicol (250 ppm) and incubated at 30°C for 3–4 days to a few weeks until growth was initiated (Wang et al. Citation2012). The hyphal tips that emerged from the plant tissues were picked and maintained on potato dextrose agar plates for further studies.
Molecular identification of endophytic fungus using internal transcribed spacer region
Genomic DNA extraction
Strain CBR-18 was cultured in potato dextrose broth (PDB) for 7 days at 30°C and the mycelium was harvested by vacuum filtration. The chilled mycelia were ground with a pestle and mortar under liquid nitrogen, transferred into microcentrifuge tubes with 1 ml of 2 × cetyltrimethyl ammonium bromide (CTAB) extraction buffer and incubated at 65°C for 30 min with gentle swirling. After centrifugation, the aqueous phase of the mixture containing total DNA was extracted with an equal volume of phenol/chloroform/isoamyl alcohol (25:24:1). Residual phenol was removed with the addition of chloroform/isoamyl alcohol (24:1) twice. Two volumes of ethanol and 0.1 volume of 3 M sodium acetate were added to precipitate DNA in the aqueous phase, which was then incubated at −20°C overnight (Kim et al. Citation2010). The DNA pellet was washed twice with 70% ethanol and suspended in 15 µl of Tris–EDTA (TE) buffer.
Polymerase chain reaction amplification
Internal transcribed spacer-1 (ITS1; 5′-TCCGTAGGTG AACCTGCGG-3′) and ITS4 (5′-TCCTCCGCTTATTGA TATGC-3′) (White et al. Citation1990) universal primers were used for the amplification of ITS regions. The polymerase chain reaction (PCR) mixture (50 µl) contained 5 µl 10 × PCR buffer containing 15 mM MgCl2, 5 µl 2 mM each deoxynucleoside triphosphates, 2 µl of each primer (5 pmol/µl), 4 µl template DNA, 2 µl (1 U/µl) Taq polymerase and deionized water (30 µl). Thermal cycling conditions were as follows: initial denaturation (4 min at 95°C), followed by 30 cycles of denaturation (94°C for 50 s), annealing (51°C for 1 min) and primer extension (72°C for 1 min), followed by final extension for 10 min at 72°C (Bhagat et al. Citation2012).
Taxon sampling and phylogenetic affiliation
ITS sequence data obtained for strain CBR-18 were annotated using Geneious 6.1.6 (2013) software and submitted to the National Centre for Biotechnology Information (NCBI) GenBank database. ITS rDNA sequences with maximum identity to that of Phomopsis spp. were retrieved from the NCBI nucleotide database using BLASTN. ITS sequences were filter-searched and the closest sequences related to the same taxon were selected for phylogenetic analysis. The multiple sequence alignments were performed using CLUSTALW software utilizing default settings, and dendrograms were generated by MEGA 4.0 software with a bootstrap consensus of 1000 replicates (Padhi & Tayung Citation2013).
Polyketide synthase gene amplification of strain CBR-18
LC1 and LC2c, LC3 and LC5c (Bingle et al. Citation1999), and KS3 and KS4c (Nicholson et al. 2011), which are ketosynthase domain degenerate primers, were used to amplify PKS genes of Phomopsis liquidambaris. PCRs (50 µl) contained 4 µl DNA template, 5 µl 10 × PCR buffer, 4 µl 2.5 mM of each dNTPs, 3 µl of each primer, 1 µl of 5 U/µl rTaq DNA polymerase and 30 µl deionized water. The thermal cycling programme was 5 min at 94°C, 34 cycles of 1 min at 94°C, 1.5 min at 55°C, 3 min at 72°C and 10 min at 72°C.
Accession numbers
The GenBank/DNA Data Bank of Japan (DDBJ)/European Molecular Biology Laboratory (EMBL)/NCBI accession numbers for the ITS rDNA sequence and PKS gene sequence of the endophytic fungus in the present study are KJ372757 and KM215685, respectively.
Fermentation and extraction of secondary metabolites
For metabolite production, the fungus was cultured in 2 litre Erlenmeyer flasks containing 500 ml of PDB at 28°C under static conditions for 8 weeks. The culture broth was then filtered through double-layer sterile muslin cloth to separate the filtrate and mycelium. The filtrate was blended thoroughly and centrifuged at 4000 rpm for 5 min. The aqueous supernatant phase was extracted three times with an equal volume of ethyl acetate and evaporated to dryness under reduced pressure at 45°C using a rotary flash evaporator (Buatong et al. Citation2011).
Evaluation of antimicrobial activity
Antimicrobial susceptibility testing was carried out by the disc diffusion assay (Sadrati et al. Citation2013). Sterile discs (6 mm diameter) impregnated with 20 µl of ethyl acetate extract were dried in a laminar hood and placed on the surface of the media already seeded with test organisms in Petri plates. The plates were incubated at 37 ± 2°C and the diameter of the zone of inhibition was recorded.
Determination of minimum inhibitory concentration
The minimum inhibitory concentration (MIC) was determined by a microbroth dilution assay in sterile 96-well plates (Padhi and Tayung Citation2013). The purified compound was tested in two-fold dilution in the concentration range 200–0.3906 µg/ml. 2,3,5-Triphenyltetrazolium chloride (TTC) and 3-(4,5-dimethylthiazol-2-yl)-2,5- diphenyltetrazolium bromide (MTT) (Sigma-Aldrich Bengaluru, India) were added to each well as microbial growth indicators for test bacteria and fungi, respectively. Standard drugs, gentamicin and nystatin, were used as positive controls, whereas broth alone was used as a negative and sterility control. The MIC was determined as the lowest concentration of the compound with no visible growth as indicated by the growth indicators. All the determinations were conducted in duplicate.
Thin-layer chromatography–bioautography
Antibacterial activity of ethyl acetate extract was evaluated by analytical thin-layer chromatography (TLC) using the bioautographic agar overlay method (Valgas et al. Citation2007). Ten microlitres of the extract was spotted on TLC silica gel plates (TLC, Alugram® SIL G/UV254; Machereye-Nagel, Duren, Germany) in an optimized solvent system of petroleum ether/ethyl acetate (1:2). TLC sheets were air dried, ultraviolet (UV) sterilized and then placed on a sterile Petri plate overlaid with Muller–Hinton medium incorporating 1 mg/ml TTC, inoculated with 1% standardized Staphylococcus aureus. After 8 h of diffusion at 8°C, plates were incubated for 24 h at 37°C. The areas of inhibition on the active spot were compared with the Rf value of the related spots on the reference TLC plate.
Fractionation and purification of the antimicrobial metabolite
Ethyl acetate extract of culture filtrate was fractionated by column chromatography (50 cm × 1 cm) using silica gel (60–200 mesh; Hi Media, Mumbai, India), eluted successively with petroleum ether/ethyl acetate (100:0 to 0:100) in a stepwise gradient to obtain the increased polarity. Bioactive fractions were pooled on the basis of similar UV/visible absorption patterns and TLC–bioautography analysis (Rakshith et al. Citation2013a). The pooled bioactive fractions were further subjected to preparative TLC to increase the purity of the compound.
Preparative thin-layer chromatography for further purification of compound
A streak of the pooled bioactive fraction was applied manually on a preparative TLC glass plate (20 cm × 20 cm; 1500 µm thickness) with inorganic fluorescent indicator binder (Analtech; Sigma Aldrich, Steinheim, Germany). After air drying, TLC plates were developed using the same mobile phase as used above in a presaturated glass chamber. Bands that showed antimicrobial activity were scraped off carefully from the plate in each set of experiments. The scratched sample was dissolved in high-performance liquid chromatography (HPLC) grade methanol and centrifuged at 10,000 rpm for 15 min to remove silica. The supernatant was collected, filtered through a 0.22 µm filter and dried under reduced pressure. All the dried samples were passed under nitrogen gas for 10 min and then dissolved in methanol for characterization and bioactivity analysis (Rajauria and Abu-Ghannam Citation2013).
Identification of antimicrobial metabolite
Electrospray ionization time-of-flight mass spectrometry (ESI-TOF-MS) was carried out using an LCT liquid chromatogram–mass spectrometer (Micromass). Optical rotation was measured on a JASCO P-1020 polarimeter.
Results and discussion
Isolation of endophytic fungus and evaluation of surface sterilization protocol
Endophytic fungi which colonize inner plant tissues have been found to be associated with every plant species so far investigated. Considering the myriad of plants in the world, there is a great opportunity to find novel and interesting endophytic fungi (Padhi and Tayung Citation2013). In the present work, an endophytic fungus was isolated from stem tissue of C. buchanani Roem. In addition, media plates spread with the final rinse of sterile water showed no microbial growth even after 10 days of incubation at 28°C. This indicates that the surface sterilization protocol was effective at killing the epiphytic microorganisms. Thus, subsequent isolates can be considered true endophytic fungi. The growth of bacterial endophytes was effectively inhibited by amending the media with chloramphenicol (250 ppm). The present surface sterilization method was effective in helping to acquire endophytic fungi.
Molecular identification of strain CBR-18 and phylogenetic analysis
Molecular analysis was carried out based on the ITS region of rDNA and intervening 5.8S rRNA gene to identify the strain at species level (). Phylogenetic affiliation showed that the ITS sequence of strain CBR-18 closely resembles that of P. liquidambaris. A dendrogram generated by neighbour-joining analysis placed the isolate in the clade with P. liquidambaris KF032029 and P. liquidambaris KC895530, indicating that isolate CBR-18 belongs to the P. liquidambaris taxa ().
Figure 1. Polymerase chain reaction (PCR) amplification of rDNA by internal transcribed spacer (ITS) universal primers and polyketide synthase gene by the LC1–LC2c set of degenerate primers from Phomopsis liquidambaris CBR-18. Lanes: M = 100 bp DNA ladder; ITS = ITS1 and ITS4 universal primers; LC = LC1 and LC2c degenerate primers.
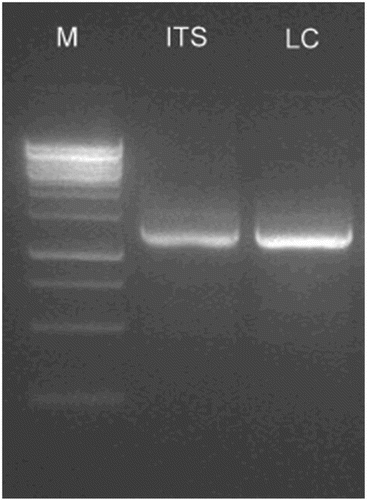
Figure 2. Internal transcribed spacer (ITS) sequence-based neighbour joining tree of Phomopsis sp. isolates: consensus neighbour joining dendrogram with bootstrap values (1000 replications) based on multiple sequence alignment. The scale bar indicates nucleotide substitutions per nucleotide position.
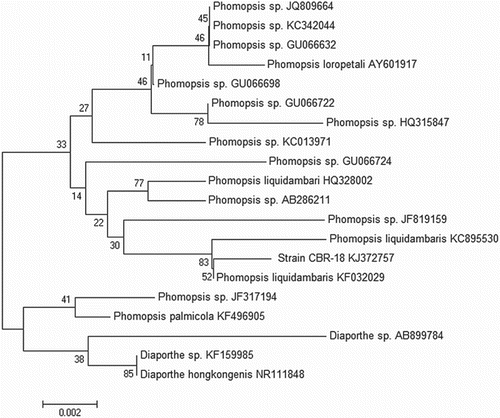
Detection of ketosynthase domain of fungal polyketide synthase genes in Phomopsis liquidambaris CBR-18
To detect the ketosynthase domain of strain CBR-18, PCR was employed using three pairs of degenerate primers, LC1–LC2c, LC3–LC5c and KS3–KS4c, which are specific for the particular types of non-reduced, partially reduced and highly reduced ketosynthase domains of endophytic fungal polyketide metabolites. Genomic DNA of CBR-18 was amplified with LC1–LC2c pairs of degenerate primers (). Lin et al. (Citation2011) also reported on some endophytic fungi amplified with the LC1–LC2c set of degenerate primers. This encodes uncharacterized functional enzyme systems, which may suggest a novel function for polyketide production (Jumpathong et al. Citation2011). Fungal type I polyketides are synthesized by multidomain enzymes using an iterative strategy to build up a polyketide molecule (Fujii et al. Citation2001). Fungal endophytes have been genetically screened for the presence of PKS genes as indicators of bioactivity.
Antimicrobial activity
Ethyl acetate extract of CBR-18 was evaluated for antimicrobial activity against test pathogens by disc diffusion assays. Metabolites displayed moderate to strong antimicrobial activity (), with the highest activity observed against Escherichia coli (19.33 ± 0.66 mm), followed by Bacillus subtilis (18.66 ± 0.66 mm) and Salmonella typhi (18.00 ± 0.00 mm). The diameters of inhibition zones for the test pathogens are given in . Similarly, an endophytic Phomopsis sp. ED2 residing in the medicinal plant Orthosiphon stamineus Benth. has also shown significant biological activity against a wide range of bacterial and fungal pathogens (Tong et al. 2014). The capacity to inhibit both bacterial and fungal pathogens shows that metabolites have broad-spectrum antimicrobial activity and this fungus, as an endophyte, may protect the host against invading pathogens. Thus, the ability of C. buchanani to grow better and to have greater competitiveness in its invaded range may be due to the association of this fungal endophyte, which that produces antimicrobial metabolites.
Figure 3. Antimicrobial activity of ethyl acetate extract of Phomopsis liquidambaris CBR-18 against (a) Escherichia coli, (b) Staphylococcus aureus, (c) Salmonella typhi, and (d) Bacillus subtilis by disc diffusion assay. +ve = positive control; –ve = ethyl acetate as negative control.
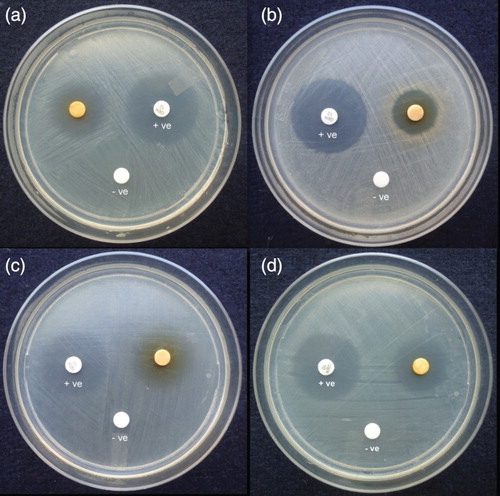
Table 1. Antimicrobial activity of ethyl acetate extract of Phomopsis liquidambaris CBR-18 (100 µg/disc) isolated from Cryptolepis buchanani Roem.
Thin-layer chromatography–bioautography
In this study, the compound present in the ethyl acetate extract was confirmed by analytical TLC. The chromatographic profile of ethyl acetate extract indicated the presence of bands under 254 nm and 365 nm. The band at Rf = 0.56 was more intense than the rest of the bands, which may be due to the increased production of antimicrobial metabolites.
Regarding the antimicrobial activity by TLC–bioautography, developed TLC sheets were preinoculated with S. aureus culture and TTC agent. A clear zone of inhibition was observed at Rf = 0.56 against the red background. TLC–bioautographic screening of antimicrobial activity of compounds in the crude extract is a simple, fast and reproducible method (Marston Citation2011; Rao et al. Citation2014). The antimicrobial activity of ethyl acetate extract of Phomopsis sp. FPSP-25 was determined using the same approach (Rakshith et al. Citation2013b). A TLC band at Rf = 0.56 was observed as an active metabolite of P. liquidambaris CBR-18; therefore, this extract component was analysed further to identify the metabolite.
Purification and identification of compound
Eleven fractions derived from silica gel column chromatography of culture extract were evaporated to dryness and analysed by TLC–bioautography. Fraction 5 was found to be active against S. aureus in the initial antibacterial assay. The active fraction was further purified by preparative TLC to obtain high-purity metabolite using petroleum ether/ethyl acetate (1:2) as the eluant ().
Figure 4. (a) Thin-layer chromatogram of column purified fractions showing an active band at an Rf value of 0.56. (b) Chromatogram of purified compound using preparative thin-layer chromatography (TLC). (c) TLC–bioautography assay of purified compound showing a zone of inhibition against Staphylococcus aureus.
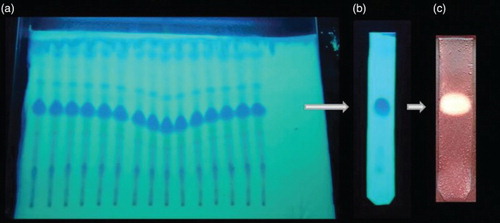
Figure 5. Electrospray ionization time-of-flight mass spectrometry (ESI-TOF-MS) of oblongolide Y showing a major molecular ion peak at 301.1607 [M+Na]+.
![Figure 5. Electrospray ionization time-of-flight mass spectrometry (ESI-TOF-MS) of oblongolide Y showing a major molecular ion peak at 301.1607 [M+Na]+.](/cms/asset/d3ef5efa-6318-4692-8dee-845202ecf6e6/tfls_a_1033768_f0005_c.jpg)
Crystallization of metabolite from MeOH furnished the compound as a white powder. ESI-TOF-MS of the metabolite revealed a molecular ion peak at m/z 301.1607 [M+Na]+ corresponding to the molecular formula C17H26O3 (). MIC values of the purified compound are given in . Purified metabolite significantly inhibited S. aureus, E. coli, Pseudomonas aeruginosa and Candida albicans with MIC values of 25–100 µg/ml when gentamicin and nystatin were used as the positive control for test bacteria and fungi, respectively. In ESI-TOF-MS, the metabolite exhibited a molecular ion peak at m/z 301.1607 [M+Na]+, which closely resembles the mass of oblongolide Y. A literature comparison of ESI-TOF-MS spectra with that of oblongolide indicated that the metabolite belongs to the derivatives of oblongolides (Bunyapaiboonsri et al. Citation2010). The structure of the compound was deduced from comparison of the TOF-MS spectroscopic data with those reported in the literature (). To avoid wasting effort on the isolation and identification of known compounds, TOF-MS is a powerful tool for the rapid identification of known compounds, i.e. dereplication, which is crucial for the rapid discovery of novel metabolites (Nielsen et al. Citation2011).
Table 2. Minimum inhibitory concentration (MIC) of purified compound of endophytic Phomopsis liquidambaris CBR-18.
The biosynthetic pathway of oblongolides was proposed by Shen and co-workers (Lin et al. Citation2009). The putative biosynthetic pathway of oblongolides suggested that these compounds could be classified as polyketides (Dai et al. Citation2005). Oblongolide is a hexaketide γ-lactone, first isolated from Phomopsis oblonga found in the outer bark of an elm tree, Ulmus sp. (Begley & Grove Citation1985). Many oblongolides are reported to have an identical fusion pattern of two six-membered rings, with an octahydronaphthalene skeleton (Lin et al. Citation2009). This type of carbon skeleton has been found in other fungal metabolites such as decumbenones A and B (Fujii et al. Citation2002); polyketide dihydromonacolin, which is a nonaketide and the first recognized intermediate in lovastatin biosynthesis, also has the same octahydronaphthalene skeleton (Endo et al. 1985). Polyketides have gained great commercial interest for drug discovery and account for medicinal sales exceeding $20 billion per year (Cheng et al. Citation2009). The study of PKS genes and natural polyketide products may provide important ecological insights in addition to opportunities for antimicrobial drug development (Zhao et al. Citation2008).
To the authors' knowledge, no reports are available on the assessment of the biosynthetic potential of endophytic fungi for polyketide metabolites via a comprehensive genomic and TLC-guided approach; therefore, this is the first report of polyketide production by this method. Phomopsis spp. are known to produce oblongolides B, C, C1, N, O, P, P1, Q, R, S, T, U, V, R, X, W1 and W2 (Lin et al. Citation2009, Citation2011; Bunyapaiboonsri et al. Citation2010). The anti-cancer activity of oblongolides C1, P1 and X1 (Lin et al. Citation2011), and the anti-tuberculosis, anti-herpes simplex virus type 1 and cytotoxic activities of a few oblongalides have been authenticated (Bunyapaiboonsri et al. Citation2010). Apart from these, the ecological benefits of a few other oblongolides have also been reported. Hence, from the present study, it can be understood that the antimicrobial activities of this compound play a significant role in mutualistic benefits of this endophyte to the host. Besides the enhanced range of bioactive products of this widespread species, the ability of isolate CBR-18 to yield previously known compounds provides additional support to the evidence that endophytic fungi are implicated in the biosynthesis of antimicrobial metabolites. Thus, the present study indicates that P. liquidambaris strain CBR-18 is a promising source of potential antimicrobial agents and could be used for industrial purposes.
Conclusions
PKS type I is a functional PKS gene of P. liquidambaris which may perform an important role in the fungal metabolite synthesis. This study indicates that genomic and chemical investigation by a chromatographic approach is a useful strategy for the discovery of polyketides that could be employed for initiating pilot studies. This approach enabled the targeting of different ketosynthase domains for the isolation of polyketide antimicrobials and could be used for the rapid screening of fungal endophytes capable of producing polyketide agents. Strain CBR-18 is a promising source of oblongolide Y polyketide antimicrobials, which could be exploited for industrial purposes.
Acknowledgements
The authors are very thankful to the University Grand Commission (UGC), New Delhi, and University of Mysore, India, for providing a PhD fellowship to the first author. We also thank the Institution of Excellence (IOE), University of Mysore, for providing instrumentation facilities.
Disclosure statement
The authors declare that they have no conflict of interest.
References
- Aly AH, Debbab A, Kjer J, Proksch P. 2010. Fungal endophytes from higher plants: a prolific source of phytochemicals and other bioactive natural products. Fungal Divers. 41(1):1–16. doi: 10.1007/s13225-010-0034-4
- Begley MJ, Grove JF. 1985. Metabolic products of Phomopsis oblonga. Part 1. 3a,5a,6,7,8,9,9a,9b-Octahydro-7,9b-dimethylnaphtho[1,2-c]furan-1(3H)one (oblongolide). J. Chem. Soc., Perkin Trans. 1:861–863. doi: 10.1039/p19850000861
- Bhagat J, Kaur A, Sharma M, Saxena AK, Chadha BS. 2012. Molecular and functional characterization of endophytic fungi from traditional medicinal plants. World J Microbiol Biotechnol. 28:963–971. doi: 10.1007/s11274-011-0894-0
- Bingle LE, Simpson TJ, Lazarus CM. 1999. Ketosynthase domain probes identify two subclasses of fungal polyketide synthase genes. Fungal Genet Biol. 26:209–223. doi: 10.1006/fgbi.1999.1115
- Blunt JW, Copp BR, Keyzers RA, Munro MHG, Prinsep MR. 2012. Marine natural products. Nat. Prod. Rep. 29:144–222. doi: 10.1039/C2NP00090C
- Buatong J, Phongpaichit S, Rukachaisirikul V, Sakayaroj J. 2011. Antimicrobial activity of crude extracts from mangrove fungal endophytes. World J Microbiol Biotechnol. 27:3005–3008. doi: 10.1007/s11274-011-0765-8
- Bunyapaiboonsri T, Yoiprommarat S, Srikitikulchai P, Srichomthong K, Lumyong S. 2010. Oblongolides from the endophytic fungus Phomopsis sp. BCC 9789. J. Nat. Prod. 73:55–59. doi: 10.1021/np900650c
- Cheng YQ, Coughlin LM, Lim SK, Shen B. 2009. Type I polyketide synthases that require discrete acyltransferases. Methods Enzymol. 459:165–186. doi: 10.1016/S0076-6879(09)04608-4
- Cox RJ. 2007. Polyketides, proteins and genes in fungi: programmed nano-machines begin to reveal their secrets. Org Biomol Chem. 5:2010–2026. doi: 10.1039/b704420h
- Dai CC, Yu BY, Zhao YT, Jiang JH, Yang QY. 2005. Correlation between inhibition activity of endophytic fungus from Euphorbia pekinensis and its host. J. Chin. Appl. Ecol. 16(7):1290–1294.
- Debbab A, Aly AH, Proksch P. 2012. Endophytes and associated marine derived fungi – ecological and chemical perspectives. Fungal Divers. 57:45–83. doi: 10.1007/s13225-012-0191-8
- Demain AL, Sanchez S. 2009. Microbial drug discovery: 80 years of progress. J. Antibiot. 62:5–16. doi: 10.1038/ja.2008.16
- Endo A, Negishi Y, Iwashita T, Mizukawa K, Hirama M. 1985. Biosynthesis of ML-236B (compactin) and monacolin K. J Antibiot (Tokyo). 38: 444–448.
- Fujii I, Watanabe A, Sankawa U, Ebizuka Y. 2001. Identification of a Claisen cyclase domain in fungal polyketide synthase WA, a naphthopyrone synthase of Aspergillus nidulans. Chem Biol. 8:189–197. doi: 10.1016/S1074-5521(00)90068-1
- Fujii Y, Asahara M, Ichinoe M, Nakajima H. 2002. Fungal melanin inhibitor and related compounds from Penicillium decumbens. Phytochem. 60(7):703–708. doi: 10.1016/S0031-9422(02)00196-6
- Jumpathong J, Seshime Y, Fujii I, Peberdy J, Lumyong S. 2011. Genome screening for reducing type I polyketide synthase genes in tropical fungi associated with medicinal plants. World J. Microb. Biot. 27:1989–1995. doi: 10.1007/s11274-011-0659-9
- Kaul A, Bani S, Zutshi U, Suri KA, Satti NK, Suri OP. 2003. Immunopotentiating properties of Cryptolepis buchanani root extract. Phytother Res. 17:1140–1144. doi: 10.1002/ptr.1186
- Kim JS, Seo SG, Jun KB, Kim JW, Kim SH. 2010. Simple and reliable DNA extraction method for the dark pigmented fungus, Cercospora sojina. Plant Pathol J. 26(3):289–292. doi: 10.5423/PPJ.2010.26.3.289
- Knight V, Sanglier JJ, DiTullio D, Braccili S, Bonner P, Waters J, Hughes D, Zhang L. 2003. Diversifying microbial natural products for drug discovery. Appl Microbiol Biotechnol. 62:446–458. doi: 10.1007/s00253-003-1381-9
- Komaki H, Fudou R, Iizuka T, Nakajima D, Okazaki K, Shibata D, Ojika M, Harayama S. 2008. PCR detection of type I polyketide synthase genes in myxobacteria. Appl Environ Microb. 74(17):5571–5574. doi: 10.1128/AEM.00224-08
- Lin T, Lin X, Lu C, Hu Z, Huang W, Huang Y, Shen Y. 2009. Secondary metabolites of Phomopsis sp. XZ-26, an endophytic fungus from Camptotheca acuminate. Eur. J. Org. Chem. 18:2975–2982. doi: 10.1002/ejoc.200801021
- Lin Y, Wang HG, Lin X, Hu ZY, Chen QC, Xu Y, Zhang XK, Chen HF. 2011. Three new oblongolides from Phomopsis sp. XZ-01, an endophytic fungus from Camptotheca acuminate. Molecules. 16:3351–3359. doi: 10.3390/molecules16043351
- Marston A. 2011. Thin-layer chromatography with biological detection in phytochemistry. Planar Chromatogr. 1218:2676–2683. doi: 10.1016/j.chroma.2010.12.068
- Newman DJ, Cragg GM. 2007. Natural products as sources of new drugs over the last 25 years. J. Nat. Prod. 70:461–477. doi: 10.1021/np068054v
- Newman DJ, Cragg GM. 2012. Natural products as sources of new drugs over the 30 years from 1981 to 2010. J. Nat. Prod. 75:311–335. doi: 10.1021/np200906s
- Nicholson TP, Rudd BA, Dawson M, Lazarus CM, Simpson TJ, Cox RJ. 2001. Design and utility of oligo nucleotide gene probes for fungal polyketide synthases. Chem Biol. 8:157–178. doi: 10.1016/S1074-5521(00)90064-4
- Nielsen KF, Mansson M, Rank C, Frisvad JC, Larsen TO. 2011. Dereplication of Microbial Natural Products by LC-DAD-TOFMS. J. Nat. Prod. 74:2338–2348. doi: 10.1021/np200254t
- Padhi S, Tayung K. 2013. Antimicrobial activity and molecular characterization of an endophytic fungus, Quambalaria sp. isolated from Ipomoea carnea. Ann Microbiol. 63:793–800. doi: 10.1007/s13213-012-0534-4
- Rajauria G, Abu-Ghannam N. 2013. Isolation and partial characterization of bioactive fucoxanthin from Himanthalia elongate brown seaweed: a TLC-based approach. International Journal of Analytical Chemistry. http://dx.doi.org/10.1155/2013/802573http://dx.doi.org/10.1155/2013/802573
- Rakshith D, Santosh P, Satish S. 2013a. Isolation and characterization of antimicrobial metabolite producing endophytic Phomopsis sp. from Ficus pumila Linn. (Moraceae). Inter. J. Chem. Anal. Sci. 4:156–160. doi: 10.1016/j.ijcas.2013.08.006
- Rakshith D, Santosh P, Tarman K, Gurudatt DM, Satish S. 2013b. Dereplication strategy for antimicrobial metabolite using thin-layer chromatography–bioautography and LC–PDA–MS analysis. J. Planar. Chromatogr. – Mod. TLC. 26(6):470–474. doi: 10.1556/JPC.26.2013.6.2
- Rao HCY, Santosh P, Rakshith D, Satish S. 2014. Molecular characterization of an endophytic Phomopsis liquidambaris CBR-15 from Cryptolepis buchanani Roem. and impact of culture media on biosynthesis of antimicrobial metabolites. 3Biotech. doi:10.1007/s13205-014-0204-2
- Rateb ME, Ebel R. 2011. Secondary metabolites of fungi from marine habitats. Nat. Prod. Rep. 28:290–344. doi: 10.1039/c0np00061b
- Sadrati N, Daoud H, Zerroug A, Dahamna S, Bouharati S. 2013. Screening of antimicrobial and antioxidant secondary metabolites from endophytic fungi isolated from wheat (Triticum durum). J. Plant Prot. Res. 53(2):128–136. doi: 10.2478/jppr-2013-0019
- Scherlach K, Hertweck C. 2009. Triggering cryptic natural product biosynthesis in microorganisms. Org Biomol Chem. 7: 1753–1760. doi: 10.1039/b821578b
- Schumann J, Hertweck C. 2006. Advances in cloning, functional analysis and heterologous expression of fungal polyketide synthase genes. Journal of Biotechnology. 124:690–703. doi: 10.1016/j.jbiotec.2006.03.046
- Tong WY, Zaadah NJ, Nurhaida, Tan WN, Melati K, Latiffah Z, Darah I. 2014. Antimicrobial activity of Phomopsis sp. ED2 residing in medicinal plant Orthosiphon stamineus Benth. Annu Rev Res Biol. 4(9). doi: 10.9734/ARRB/2014/8060
- Valgas C, de Souza SM, Smania EFA, Smania AJ. 2007. Screening methods to determine antibacterial activity of natural products. Braz J Microbiol. 38:369–380. doi: 10.1590/S1517-83822007000200034
- Wang LW, Xu G, Wang JY, Su ZZ, Lin FC, Zhang CL, Kubicek CP. 2012. Bioactive metabolites from Phoma species, an endophytic fungus from the Chinese medicinal plant Arisaema erubescens. Appl Microbiol Biotechnol. 93:1231–1239. doi: 10.1007/s00253-011-3472-3
- White TJ, Bruns T, Lee S, Taylor JW. 1990. PCR Protocols: A Guide to Methods and Applications Amplification and direct sequencing of fungal genes for phylogenetics. In: Innis M, Gelfand DH, Sninsky JJ, White TJ, editor. San Diego: Academic Press; p. 315–322.
- Zhao J, Yang N, Zeng R. 2008. Phylogenetic analysis of type I polyketide synthase and non-ribosomal peptide synthetase genes in Antarctic sediment. Extremophiles. 12:97–105. doi: 10.1007/s00792-007-0107-9
- Zhao XQ. 2011. Genome-Based Studies of Marine Microorganisms to Maximize the Diversity of Natural Products Discovery for Medical Treatments. Evid. Based Complement. Alternat. Med. doi:10.1155/2011/384572