ABSTRACT
Skid trails in the Hyrcanian forest of northern Iran are important for moving wood to log landings; however, they can cause soil compaction and alter water infiltration. This research therefore presents an analysis of the impact of ground-based skidding on soil hydrophysical properties in Kuhmiyan forest located in the Hyrcanian forest. Soil infiltration, bulk density, and soil resistance to penetration have been studied at three different depths of soil along the skid trails. Three sampling locations were considered: wheel ruts, center of skid trails, and undisturbed forest (control). The results showed that the total infiltration rate, and also the instantaneous infiltration rate, were significantly higher in the control treatment. The results also showed an increase of 57% and 31% for the soil bulk density at wheel ruts and centers of skid trails compared to the control. Furthermore, there was a significant difference between soil resistance in the control and the other two treatments, with wheel ruts having the highest resistance. According to the results, there was a strong negative correlation between infiltration and soil resistance (r = –0.94), and between infiltration and bulk density (r = –0.93). Using a protective layer of slash and logging debris and residual on skid trails can be highly recommended to moderate soil compaction and mitigate the destructive impact of logging equipment.
Introduction
Preserving forest soil condition and adequately establishing tree regeneration are key factors to achieve sustainable forest productivity. However, long-term productivity of forests may be affected due to trafficking impacts from heavy ground-based harvesting equipment (Zenner et al. Citation2007). The use of heavy logging equipment in log extraction, especially ground-based ones, has been criticized because of soil disturbance and also damage to residual stand caused by such equipment (Han and Kellogg Citation2000). Soil damage includes soil disturbance (Malmer and Grip Citation1990), soil compaction (McNabb et al. Citation2001; Kolka et al. Citation2012; Solgi and Najafi Citation2014), and removal of soil organic matter (Ole-Meiludie and Njau Citation1989). These will result in changes to soil properties which often cause the reduction of tree growth and forest products (Kozlowski Citation1999; Heninger et al. Citation2002; Pimentel Citation2006). The most frequent and depleting damage is soil compaction, which generally leads to an increase in bulk density due to the consolidation of soil particles as air-filled voids are reduced (Lister Citation1999; Craig Citation2004) and decreases in macropore space (Startsev and McNabb Citation2001; Zenner et al. Citation2007). As air-filled porosity falls below 10% of the total soil volume, microbial activity and plant growth can be severely limited in most soils (Brady and Weil Citation2002). Decreases in soil macropore space reduces infiltration capacity due to changes in non-capillary pore space. The increase in soil density is associated with reduced seepage of water, poor aeration, and greater mechanical resistance to root growth (Corns Citation1988; Kozlowski Citation1999; Czyz Citation2004). The increased bulk density can also result in accelerated surface runoff and increased soil erosion (Williamson and Neilsen Citation2000; Christopher and Visser Citation2007; Schmitt Citation2009) due to the reduction of soil infiltration capacity (Startsev and McNabb Citation2000; Horn et al. Citation2004). Unfortunately, these adverse impacts of soil disturbance may persist for several years because of very slow recovery rates (Shepperd Citation1993; Grigal Citation2000; Heninger et al. Citation2002).Topsoil layers which are subject to much more compaction than the below layers are important in terms of water infiltration, particularly during high intensity rainstorms, since they have both high hydraulic conductivity and high water storage capacity which may be significantly reduced by compaction (Jusoff and Majid Citation1986). The topsoil layer also contains 70%–90% of the total root mass and is undoubtedly the most important zone for tree uptake and storage of water and nutrients (Jusoff and Majid Citation1986).
According to the Forests, Range and Watershed Management Organization of Iran (Citation2002), skid trails are permanently located in some parts of the forest for long periods of time. By developing permanent skid trails we may be able to prevent heavy equipment moving into the forest and so limit soil damage to the area where skid trails are located. However, using these permanent skid trails for long periods leads to more soil compaction and thus more runoff and erosion from the skid trails into the undisturbed forest.
The impacts of mechanized forest harvesting on soil physical properties, especially soil compaction, have been widely investigated (Greacen and Sands Citation1980; Shetron et al. Citation1988; Aust et al. Citation1995; McNabb et al. Citation2001; Kolka et al. Citation2012; Solgi and Najafi Citation2014). Aust et al. (Citation1995) found bulk density increases of 20% and decreases in average soil macroporosity (80%) and saturated hydraulic conductivity (12%) following harvesting disturbance on wet pine flats that had been compacted. Similarly, Shetron et al. (Citation1988) showed well over 80% increases in bulk density associated with logging disturbance and 20% decreases in porosity on a hardwood site in Wisconsin. Kolka et al. (Citation2012) studied bulk density and surface soil strength in recent clearcuts in Chippewa National Forest in northern Minnesota. They found higher bulk density and surface soil strength following harvesting compared to adjacent and similar but unharvested stands. Recently, Solgi and Najafi (Citation2014) tested the effect of skid trail slope and traffic frequency on soil compaction, total porosity, and moisture content. Based on their results, the bulk density was 57% higher and the total porosity was 31% lower on the skid trail compared to an undisturbed area. Average moisture content was also lower on the skid trail compared to the undisturbed area. Some researchers have studied the effect of increasing bulk density on soil water permeability. An early study (Steinbrenner and Gessel Citation1955) showed that a 15% increase in the bulk density of the soils of skid roads resulted in a 95% loss in permeability. Later, Malmer and Grip (Citation1990) showed average values of infiltrations to be significantly lower on skid trails (0.28 mm h−1) than in the forest (154 mm h−1). Meek et al. (Citation1992) also reported a reduction in infiltration rates of 54% when soil was compacted from 1.6 Mg m−3 to 1.8 Mg m−3. Jamshidi et al. (Citation2008) reported a significantly higher value of soil bulk density in the tracks of machine skid trails compared to that outside the skid trails.
As one can see, there have been many studies regarding soil compaction following logging operations and it has been an important issue for decades; yet we lack an understanding of the linkage between soil bulk density and soil infiltration, and also between soil penetration resistance and soil infiltration. Having better knowledge of these relationships will help us to estimate the soil infiltration capacity and thus possible runoff from skid trails in the future. The two main objectives of this study were: (1) to measure and compare soil hydrophysical properties in undisturbed forest with different parts of a newly established skid trail (the wheel rut, and center of the trail); and (2) to define the relationship between soil infiltration and soil bulk density and penetration resistance. Conducting this study will help us assess the influence of soil compaction caused by logging equipment on soil infiltration capacity and thus the potential for runoff and overland flow.
Methodology
Study area
The study was conducted in Kuhmiyan forest, located in Hyrcanian forest in northern Iran. The forest is a temperate forest, with mostly deciduous trees growing in the area, situated at 55°14′49″E and 37°56′15″N, and 1400–2000 m above sea level. Forest tree species such as Quercus castaneifolia, Carpinus betulus, and Parrotia persica predominate in this uneven-aged hardwood forest where trees can be found with a mean height of 24 m. Standing volume and tree density are 290 m3 per ha and 190 trees per ha, respectively. The mean annual rainfall is about 700 mm in the study area. Based on the soil analysis carried out in the study site, the soil texture is clay loam. The sand/silt/clay content (%) were 32.1±3.7, 29.2±1.2, and 38.7±1.4, respectively. The harvesting method used in this area is cut-to-length (shortwood) and logs are extracted with a maximum length of 5.2 m and diameter of 80–160 cm by a ground-based skidding system (Timber Jack 450C). Skid trails have been developed in this region with relatively uniform grade (20%) and width of 3.5 m. The skidder used in the study area weighs 12 t (4588 t on the rear axle and 5682 t on the front axle) and has 24.5–32 cm tires. All data were collected in summer 2011.
Materials and method
A 500 m long skid trail with relatively uniform slope (20%) was selected. The trail was 1 year old at the time of the data collection. According to the management plan for the Kuhmiyan forest, the skid trail was used by a wheeled skidder with about a 25 skidding cycle (one skidding turn includes one trip empty and one trip loaded). A sampling transect was selected randomly on the skid trail and other replications were then set along the skid trail every 100 m. Infiltration, compaction, and soil penetration resistance were measured at three different sampling locations: (1) in the center of the skid trail (Richard et al. Citation1999; Han et. al. Citation2009); (2) in the wheel ruts or wheel tracks (Richard et al. Citation1999; Junior et. al. Citation2007; Donagh et al. Citation2010); and (3) 15 m off trail into the forest as an undisturbed reference sample (control) (Han et al. Citation2009; Donagh et. al. Citation2010) (see ). Soil compaction was then evaluated through bulk density and soil penetration resistance. Soil density was evaluated through bulk density in steel cylinders of 10 cm length and 5 cm diameter, in three ranges of soil depths: 0–10 cm; 10–20 cm; and 20–30 cm. Undisturbed soil samples collected in the cylinders were then weighed, dried at 105 °C to constant mass, and finally weighed again to obtain dry bulk density. Soil strength data (soil penetration resistance) were also collected at different depths of soil (10 cm intervals from 0–30 cm), using a handheld recording penetrometer (AgraTronix). The aim was to find a mean value of penetration resistance for a given depth of soil at each sampling point. Finally, the mean values obtained for each depth were taken into account to obtain a total average of penetration resistance for each soil profile. Cumulative infiltration over a 65 min period was measured using a double-ring infiltrometer (Johnson Citation1963). The infiltration and soil penetration resistance were measured a few cm away from the sampling points as the previous sampling for the bulk density measurements had already disturbed the area (Snider and Miller Citation1985). All the measurements were carried out on the same day to make sure the antecedent rainfall and moisture conditions were similar for all the treatments during the data collection. The initial moisture contents were 26.2%, 28.7%, and 46.2% for wheel-rut, center of skid trail, and forest control, respectively.
To compare percentage change the following equation was used (Sutherland et al. Citation2000):(1)
Data analysis
A Kolmogorov–Smirnov test was used to check the normality of the data before proceeding to analysis of variance. The soil properties, infiltration, compaction, and soil penetration resistance were then evaluated by statistical analysis through analysis of variance (SPSS v16). All statistical significances were judged at 1% significance level. The Dunnett multiple comparison test (where equal variances not assumed) and Duncan test were used where the analysis was statistically significant. Linear regressions were fitted to calculate the relationship between compaction and infiltration, and soil penetration resistance and infiltration.
Results
The analysis of variance showed there were significant differences in both different sites and different readings of infiltration during the 65 min period. (). illustrates how the infiltration mean values change during the test period at the three sampling locations. As expected, the mean value of infiltration rate decreased with time over the 65 min period. For all the infiltration measurements a higher mean infiltration was measured for the control treatment than wheel ruts and center of skid trail. However, differences between wheel ruts and the center were significant only in the first and second readings (i.e. the readings at 2 and 4 min).
Table 1. Summary of ANOVA for the effects of treatments on soil properties.
Figure 2. Variation of soil infiltration values at the skid trail and control point (means with different letters a, b and c are statistically different). Vertical bars are standard deviation.
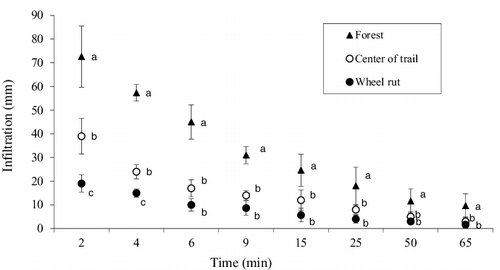
Total values of infiltration at each treatment are shown in and . The total value of infiltration for the control treatment is significantly more than those of the other treatments.
Table 2. Cumulative infiltration value in different sampling locations.
Figure 3. Cumulative infiltration values at the skid trail and control point in the 65 min period (means with different letters a, b and c are statistically different). Vertical bars are standard deviation.
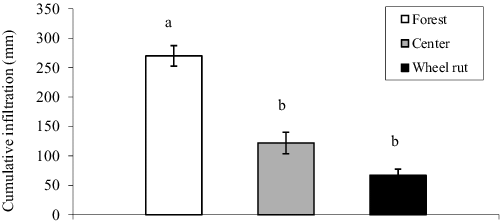
The analysis of variance also revealed that the values of soil compaction and soil penetration resistance are significantly changed in the different treatments (). According to the Dunnett test, the soil bulk density in wheel ruts was more than in the center of skid trail, and the soil bulk density compaction for the center of skid trail was more than the control (). Also, soil penetration resistance was significantly increased by moving from control treatment to center of skid trail and wheel ruts, respectively (). Data measured for soil bulk density and resistance to penetration at different locations and different depths are shown in . Both soil bulk density and soil resistance to penetration were higher at the first depth (0–10 cm) compared to the other two depths (–). As shows, the value of soil penetration resistance increased from soil depth of 0–10 cm to 20–30 cm in treatments. However, a significant increase of soil penetration resistance at the different soil depths was observed only for the control. Indeed, control treatment had a very high coefficient of variation (CV) (1.65) compared to the center of the skid trail (0.67) and wheel ruts (0.43).
Figure 4. Soil bulk density for treatments (mean ± SD) at different depths (means with different letters are statistically different; P < 0.01). The capital letters show the differences among the three locations and the lower case letters show the differences among the different soil depths.
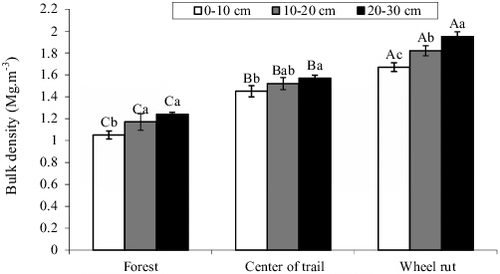
Figure 5. Soil penetration resistance for the treatments (mean ± SD) at different depths (means with different letters are statistically different; P < 0.01). The capital letters show the differences among the three locations and the lower case letters show the differences among the different soil depths.
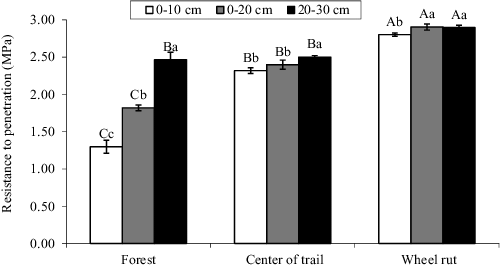
Table 3. Mean value of soil bulk density and resistance to penetration measured in the different sampling locations.
According to the results, both soil bulk density (P = 0.00, r = –0.96) and soil resistance to penetration (P = 0.00, r = –0.97) have strong negative correlation with infiltration ().
Discussion
Human activities make soil exposed and compacted, thus reducing soil infiltration and increasing runoff and sediment yield (Swank et al. Citation1989). This study addressed soil properties (i.e. compaction, infiltration, resistance to penetration) measured on skid trails in the forest. According to the results, there was a significantly different value of infiltration between the control point and the other two treatments. Because soils on skid trails are easily compacted by logging equipment, this can reduce porosity volume and thus reduce infiltration (Rahimi Citation2006). Based on this work, the infiltration rate in the 65 min period of measurement in the center of the skid trail (122 mm) and in wheel ruts (67 mm) was much lower than that of the control treatment (270 mm), with a reduction of 54.8% and 75.2% for infiltration in the center of the trail and in wheel ruts, respectively, compared to the control. Similarly, previous research showed a reduction of 65%–90% of infiltration on secondary forest road (Sutherland et al. Citation2000). However, the value of reduction will be different depending on soil type, moisture content at the skidding time, type and weight of equipment, and traffic level. Cullen et al. (Citation1991) reported a reduction of 68% and 82% for the infiltration on skid trails with medium and heavy traffic, respectively.
A significant difference of infiltration between the center of the trail and wheel ruts can only be observed at the first two readings during the measurement (i.e. at 2 and 4 min) (). This may be caused by the severe impact of the skidder and other heavy logging equipment on the topsoil layer of the wheel tracks. Given the role and effect of the topsoil layer on hydraulic conductivity (Jusoff and Majid Citation1986), there will be a high reduction of infiltration at wheel tracks.
Many researchers have reported an increase in soil bulk density resulting from logging equipment (Snider and Miller Citation1985; McNabb et al. Citation2001; Sakai et al. Citation2008; Kolka et al. Citation2012; Solgi and Najafi Citation2014). In this study, changes in soil bulk density at the center of the skid trail and in wheel ruts ranged from 28% to 58%, well above the value of 15% which Snider and Miller (Citation1985) defined as the threshold of soil compaction. Kolka et al. (Citation2012) found higher bulk density and surface soil strength in recent clearcut stand following harvesting compared to adjacent and similar but unharvested stands. Based on recent research (Solgi and Najafi Citation2014), soil bulk density was 57% higher on the skid trail compared to the undisturbed area. The increase of bulk density resulting from timber skidding on the skid trail makes these tracks more compacted, and thus more trafficable, so helping to speed up log extraction where most of the timbers have to be transported through the skid trails planned and developed for a long-term period. However, such compacted skid trails, which easily facilitate timber skidding, will also result in loss of permeability, increasing runoff and sediment yield.
The main reason for changes in soil infiltration values at wheel ruts and center of the skid trail may result from changes in soil bulk density. As the results showed, 57% and 31% increase in soil bulk density at wheel ruts and center of the skid trail resulted in a reduction of 75% and 54% for the infiltration of wheel ruts and center of the trail, respectively, compared to the control. Previous research has also reported a reduction of 54% for soil infiltration when soil bulk density increased from 1.6 Mg.m−3 to 1.8 Mg.m−3 (13%), (Meek et al. Citation1992).
According to the results of this research, changes in soil penetration resistance at the center of the skid trail and skidder wheel ruts were measured as 79% and 116% for the first depth (0–10 cm), 31% and 59% for the second depth (10–20 cm), and 1.5% and 16.5% for the third depth (20–30 cm), respectively. Other research has similarly indicated a significant increase of soil penetration resistance of skid roads to 50 cm depth (Greacen and Sands Citation1980). Also, Sutherland et al. (Citation2000) reported an increase ranging from 18% to 140% for soil penetration resistance by increasing 9%–16% of bulk density. Nugent et al. (Citation2003) found a 30%–50% increase of the penetration resistance with machine traffic.
As the skid trails are permanent in our study site, damages to the soil will be limited to the skid trails. Although the negative impact of logging equipment on soils of skid trails (e.g. soil compaction, increasing runoff, etc.) is inevitable, it can be mitigated. Considering the importance of skid trails for log transportation in our study area (being the only option for primary transportation) it is, therefore, crucial to use some technical and biological methods to mitigate transport impacts. To do this, developing surface cross drainage and making slash roads by using a cover of slash and logging debris and residual (Labelle and Jaeger Citation2012) on skid trails can be highly recommended to direct overland flow from the skid trails into the forest, and also to moderate soil compaction. Furthermore, using skid trails in dry conditions may help to moderate compaction of soil on the trails.
The result of this research can also be helpful to forest managers to estimate the capability of soil, for absorption of precipitation reaching it. Having this estimates along with the maximum precipitation based on a long-term return period will, in turn, facilitate predicting of runoff and overland flow.
Disclosure statement
No potential conflict of interest was reported by the authors.
Additional information
Funding
References
- Aust WM, Tippett MD, Burger JA, McKee WH. 1995. Compaction and rutting during harvesting affect better drained soils more than poorly drained soils on wet pine flats. South J Appl For. 19:72–77.
- Brady NC, Weil RR. 2002. Elements of the nature and properties of soils. Upper Saddle River: Prentice Hall; p. 624.
- Christopher E, Visser R. 2007. Methodology for evaluating postharvest erosion risk for the protection of water quality. New Zealand J For Sci. 54:20–25. [online] URL:http://www.nzjf.org/free_issues/NZJF52_2_2007/09BD57F4-FF32-4095-A22E-50579B1F1CE4.pdf.
- Craig RF. 2004. Soil mechanics. 7th ed. London (UK): Spon Press. British Library Cataloguing in Publication Data; p. 447.
- Corns IGW. 1988. Compaction by forestry equipment and effects on coniferous seedling growth on four soils in the Alberta foothills. Can J For Res. 18:75–84.
- Cullen J, Montagne C, Fergusen H. 1991. Timber harvest trafficking and soil compaction in Western Montana. Soil Sci Soc Am J. 55:1416–1421.
- Czyz EA. 2004. Effects of traffic on soil aeration, bulk density and growth of spring barley. Soil Till Res. 79:153–166.
- Donagh P, Rivero L, Garibaldi J, Alvez M, Cortez P, Marek M. 2010. Effects of selective harvesting on traffic pattern and soil compaction in a subtropical forest in Guarani, Misiones, Argentine. Sci For Piracicaba. 38(85):115–124.
- Forests, Range and Watershed Management Organization of Iran. 2002. A guideline for locating the landings and the skid trails. 221:35.
- Greacen EL, Sands R. 1980. Compaction of forest soils- a review. Aust J Soils Res. 18:163–189.
- Grigal DF. 2000. Effects of extensive forest management on soil productivity. For Ecol Manag. 138:169–187.
- Han HS, Kellogg LD. 2000. Damage characteristics in young Douglas-fir stands from commercial thinning with four timber harvesting systems. West J Appl For. 15(1):27−33.
- Han SK, Han HS, Page Dumroese DS, Johnson LR. 2009. Soil compaction associated with cut to length and whole tree harvesting of coniferous forest. Can J For Res. 39:976–989.
- Heninger R, Scott W, Dobkowski A, Miller R, Anderson H, Duke S. 2002. Soil disturbance and 10-year growth response of coast Douglas-fir on nontilled skid trails in the Oregon Cascades. Can J For Res. 32:233–246.
- Horn R, Vossbrink J, Becker S. 2004. Modern forestry vehicles and their impacts on soil physical properties. Soil Till Res. 79(2):207–219.
- Jamshidi R, Jaeger D, Raafatnia N, Tabari M. 2008. Influence of two ground-based skidding systems on soil compaction under different slope and gradient conditions. IJFE. 19(1):9–16.
- Johnson AI. 1963. A field method for measurement of Infiltration. US Geol Survey, water supply paper 1544-F; p. 27.
- Junior MSD, Fonseca S, Junior FA, Silva AR. 2007. Soil compaction due to forest harvesting operations. Pesq agropec bras. 4(2):257–264.
- Jusoff K, Majid M. 1986. The impact of skid trails on the physical properties of tropical hill forest soils. Pertanika. 9(3):311–321.
- Kolka R, Steber A, Brooks K, Perry CH, Powers M. 2012. Relationships between soil compaction and harvest season, soil texture, and landscape position for aspen forests. North J Appl For. 29(1):21–25.
- Kozlowski TT. 1999. Soil compaction and growth of woody plants. Scand J For Res. 14:596–619.
- Labelle ER, Jaeger D. 2012. Quantifying the use of brush mats in reducing forwarder peak loads and surface contact pressures. Croat J For Eng. 33(2):249–274.
- Lister TW. 1999. Forest harvesting disturbance and site preparation effects on soil processes and vegetation in a young pine plantation. MSc thesis in the Virginia Polytechnic Institute and State University. p. 104.
- Malmer A, Grip H. 1990. Soil disturbance and loss of infiltrability caused by mechanized and manual extraction of tropical rainforest in Sabah, Malaysia. Forest Ecol Manag. 38:1–12.
- McNabb DH, Startsev AD, Nguyen H. 2001. Soil wetness and traffic level effects on bulk density and air-filled porosity of compacted boreal forest soils. Soil Sci Soc Am J. 65:1238–1247.
- Meek BD, Rechel ER, Carter LM, Detar WR, Urie AL. 1992. Infiltration rate of a sandy loam soil: effects of traffic, tillage, and plant roots. Soil Sci Soc Am J. 56:908–913.
- Nugent C, Kanali C, Owende PMO, Nieuwenhuis M, Ward S. 2003. Characteristic site disturbance due to harvesting and extraction machinery traffic on sensitive forest sites with peat soils. Forest Ecol Manag. 180:85–98.
- Ole-Meiludie REL, Njau WLM. 1989. Impact of logging equipment on water infiltration capacity at Olmotonyi, Tanzania. Forest Ecol Manag. 26:207–213.
- Pimentel D. 2006. Soil erosion: a food and environmental threat. Environ Dev Sustain. 8:119–137.
- Rahimi H. 2006. Soil mechanics. Second ed. Danesh-o-fan Publications; p. 622.
- Richard G, Boizard H, Roger-Estrade J, Boffin J, Guerif J. 1999. Field study of soil compaction due to traffic in northern France: pore space and morphological analysis of the compacted zones. Soil Till Res. 51:151–160.
- Sakai H, Nordfjell T, Saudicani K, Talbot B, Bollenhuus E. 2008. Soil compaction on forest soils from different kinds of tires and tracks and possibility of accurate estimate. Croat J For Eng. 29(1):15–27.
- Schmitt LK. 2009. Developing and applying a soil erosion model in a data-poor context to an island in the rural Philippines. Environ Dev Sustain. 11:19–42.
- Shepperd WD. 1993. The effect of harvesting activities on soil compaction, root damage, and suckering in Colorado aspen. West J Appl For. 8:62–66.
- Shetron SG, Sturos JA, Padley E, Trettin C. 1988. Forest soil compaction: Effect of multiple passes and loadings on wheel track surface soil bulk density. North J Appl For. 5:120–123.
- Snider D, Miller F. 1985. Effects of tractor logging on soils and vegetation in eastern oregon. Soil Sci Soc Am J. 49(5):1280–1282.
- Solgi A, Najafi A. 2014. The impacts of ground-based logging equipment on forest soil. J For Sci. 60(1):28–34.
- Startsev AD, McNabb DH. 2000. Effects of skidding on forest soil infiltration in west-central Alberta. Can J Soil Sci. 80:617–624.
- Startsev AD, McNabb DH. 2001. Skidder traffic effects on water retention, pore size distribution and van Genuchten parameters on boreal forest soils. Soil Sci Soc Am J. 65:224–231.
- Steinbrenner EC, Gessel SP. 1955. The effect of tractor logging on physical properties of some forest soils in southwestern Washington. Soil science America Proc. 19:372–376.
- Sutherland A, Bussen O, Plondke L, Evans M, Ziegler D. 2000. Hydrophysical degradation associated with hiking-trail use: a case study of HAWAI'ILOA ridge trail, O'AHU, HAWAI'I. Land Degrad. Develop. 12:71–86.
- Swank WT, DeBano LF, Nelson D. 1989. Effects of timber management practices on soil and water. In: Burns RL, editor, Technical compiler. National Forest System. General Technical Report WO-55. Washington (DC): U.S. Department of Agriculture, Forest Service; p. 79–106.
- Williamson JR, Neilsen WA. 2000. The influence of soil and forest site on rate and extent of soil compaction and profile disturbance of skidtrails during ground based harvesting. Can J For Res. 30:1196–1205. doi:10.1139/x00-041.
- Zenner EK, Fauskee JT, Berger AL, Puettmann KJ. 2007. Impacts of skidding traffic intensity on soil disturbance, soil recovery, and aspen regeneration in North Central Minnesota. North J Appl For. 24(3):177–183.