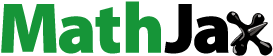
Abstract
To produce large volumes of biomass for implementing its green energy policy, the Government of Indonesia has issued a short rotation energy plantation program for a number of tree species including Calliandra calothyrsus (Meissn.). Optimum density and prompt harvesting are needed to maximise productivity, but information regarding both factors is insufficient. This study aimed to investigate the optimum growth spacing and ideal felling age to produce the largest volumes of biomass both economically and sustainably. Experiments were arranged on one hectare of land, which was divided into four plots with different plant spacing treatments (1 × 1.5 m; 1.5 × 1.5 m; 2 × 1.5 m; and 2 × 2 m). Growth and yield observations were carried out on plants aged 12, 18, and 24 months in each plot, with 30 sample trees left for growth observations and ten sample trees cut at each of the three ages after planting. This resulted in a total of 240 samples from the 2,710 trees planted. Trees were felled at 50 cm from the soil surface, and the resulting stools were left to regrow. A year after felling, resulting coppices, were observed and cut. Growth, productivity and calorific value were studied for both ‘primary’ and ‘coppiced’ wood. Study results demonstrate that competition between plants strongly determines the growth and productivity of ‘primary’ wood. The plot with the densest plant spacing had the lowest nutrient content for almost all soil nutrients. Higher plant density showed higher competition index values, and resulted in fewer and smaller diameter stems emerging from stools, and lower biomass productivity. Competition index values, which increased with plant age, can be used as an indicator for determining harvest timing. Productivity increased by up to 15% following development as a coppice plantation. Optimum wood productivity and greatest economy came from parent stands with plant spacing of 2 × 2 m, harvested at 18 months old and coppiced. This treatment yielded an average biomass/tree of primary wood 7.2 kg and coppice wood 8.22 kg, respectively, with lignin content of approximately 22%, and calorific value of around 18,807 kJ. These values match biomass energy requirements for feedstock for electricity generation. Harvesting at 24 months showed no significant increases in productivity, lignin content, or calorific value.
1. Introduction
Anticipating future energy scarcity due to the depletion of fossil energy deposits, Indonesia issued its National General Energy Plan or Rencana Umum Energi Nasional (RUEN) under Presidential Decree No. 79/2014 (Goverment of Indonesia). Through its National Medium-term Development Plan (RPJMN Citation2020–2024), the National Development Planning Agency (Bappenas) reaffirmed the plan as the National Energy Policy or Kebijakan Energi Nasional (KEN), where renewable energy would make up at least 23% of the national energy mix by 2025, and at least 31% by 2050 (Bappenas Citation2020). The policy was followed up Ministry of Energy and Mineral Resources Decree No. 39.K/20/MEM/2019 on accelerating the use of renewable energy by co-firing coal-fired power plants using biomass, which was outlined in an implementation regulation from state electricity company PLN in the form of Regulation No. 001.P/DIR/2020. To implement the mandate and provide biomass feedstock, the Ministry of Environment and Forestry (MoEF) has established a category of forest estate called Hutan Energi or energy forest for growing biomass for energy production. The United Nations Decade on Ecosystem Restoration 2021–2030 will support Indonesia in achieving its commitments to this initiative.
To guarantee a sustainable supply of biomass feedstock, it is necessary to develop forests for biomass production through short rotation forestry (SRF) (Dimitriou and Rutz Citation2015). SRF involves planting a site and then felling planted trees when they reach a certain size; typically 10–20 cm diameter at breast height (McKay Citation2011). There are two management-based categories of SRF: short rotation plantations (SRP) for harvesting and replanting, and short rotation coppices (SRC) for harvesting and producing coppices (Dimitriou and Rutz Citation2015). In addition to these systems, Moya et al. (Citation2019) introduced short rotation wood coppicing (SRWC), a silvicultural system based on short clear-cutting cycles, generally of 1–15 years, where trees are managed intensively with high-density planting and regular harvests. SRWC plantations are characterized by fast-growing trees of uniform size, planted with a regular spacing. Indications are that SRWC is an ideal biomass management system, as it not only produces renewable energy feedstock for biofuel production, but also provides a plethora of ecological services, including carbon sequestration and wildlife habitat, when planted strategically (Moya et al. Citation2019).
Coppices are characterized by their capacity to regrow with many new sprouts after the primary plant is felled (Dimitriou and Rutz Citation2015). SRC involves cutting trees back to stools to promote the growth of multiple stems in a regular cycle of roughly 2–4 years (McKay Citation2011). SRC has potential as a solution for producing biomass to meet global demand for renewable energy, mainly due to the easy-to-harvest, good-quality feedstock it can produce (Bacenetti et al. Citation2016). In addition, coppice stands are economically efficient due to their short waiting time and simplified management (clear-cut at the end of a rotation with subsequent regeneration by re-sprouting) (Spinelli et al. Citation2016). In establishing SRF, species selected for SRC should have minimum productivity of 30 metric tons per hectare (t/ha) and high coppice productivity for repeated harvesting, and the resulting wood should produce heat of >18,841 kilo joules (kJ) (Widyati et al. Citation2019). One species meeting all necessary requirements for energy forest plantation development in Indonesia is calliandra (Calliandra calothyrsus Meisn.) (Hendrati and Nurrohmah Citation2019).
Crops for harvesting in very short rotations must be planted at high densities in order to achieve their targeted productivity. Spatial arrangement is crucial, both for shaping optimum biomass productivity and calculating investment costs (Fuertes et al. Citation2021). However, high densities limit growth spacing and lead to competition within the population. Growth spacing refers to the availability of all resources required for a tree to grow and exist on a given site (Foli et al. Citation2003), and is defined as the horizontal dimensions of available ground surface area, which are limited by the presence of neighbors. Competition between plants is one of the major determinants of plant community structure and dynamics (Hortal et al. Citation2017). Neighboring plants can be from the same or different species (intraspecific or interspecific interactions), where according to basic principles of community ecology, intraspecific competition is stronger than interspecific competition (Cavalieri et al. Citation2020). Competition occurs as the growth dynamics of plants are affected by the spatial location of surrounding plants, which compete for available resources. Nutrients, water and light are the three main classes of resource that limit plant growth and are considered resources for which individual plants compete (Craine and Dybzinski Citation2013). Competition for these resources involves interactions between individual trees that decrease one or more of these components at individual and population levels. An individual plant can outperform/suppress its neighbors by maximizing its ability to capture resources, and at the same time reduce the availability of these resources for surrounding plants (Simon and Schmidt Citation2017). In addition to density, plant age also determines biomass productivity. The rate of stand biomass accumulation peaks in the early stage of development, usually at the time of canopy closure or peak leaf area of a stand (Xu et al. Citation2012). The age of an SRC plant stands determines its productivity and ability to regrow after pruning. With this information in mind, we hypothesized that in developing SRC stands, plant density and age at initial harvesting can determine coppice productivity and stand sustainability.
Currently there is insufficient information on the optimal growth spacing and ideal age for initial harvesting of calliandra stands to promote optimum productivity in coppices that can be harvested sustainably each year. As such information is limited, scientific guidelines for developers and farmers, and a commercial-scale development standard for this species, have yet to be established. Therefore, the study aimed to investigate: (1) optimum plant density for calliandra cultivated as a high-yield tree through short rotation forestry to produce the highest volumes of biomass; and (2) ideal felling age for calliandra parent stands so post-harvest stools can yield optimum coppice productivity, both economically and sustainably, in subsequent rotations.
2. Materials and methods
Planting trials were carried out from April 2016 to November 2019 in a study site located on state forestry company Perum Perhutani land in the Babakan Jawa village administrative area in Majalengka subdistrict, Majalengka district, West Java province, Indonesia (coordinates 6° 46′ 44.618″ S, 108° 17′ 6.742″ E). Average temperatures at the site range from 22.6–37.3 °C, while average annual rainfall is 3,226.3 mm, with five dry months.
2.1. Establishing SRF plots
The study was designed in randomized blocks with each block having different plant spacing treatments. One hectare of land was divided into four plots. Each plot was planted using a different spacing: 1 × 1.5 meters (density of 1,667 per 2,500 m2); 1.5 × 1.5 meters (density of 1,111); 1.5 × 2 meters (density of 833); and 2 × 2 meters (density of 625).
2.1.1. Growth and survival rate assessments
Approximately 240 tree samples were randomly selected for the study. A total of 120 tree samples were arranged for time series growth assessments, representing the four spacing treatments, and the remaining 120 tree samples were felled at three harvesting age classes (12, 18, and 24 months after planting).
Calliandra calothyrsus Meisn. is a multi-stem tree with no clear leader shoots, so tree diameter measurements were taken for all stems at an average height of 160 cm from the ground, then all values were averaged. Measurements were taken at 6, 12, 18, and 24 months after the planting date. Plant survival rates were determined by comparing numbers of living plants to total numbers of seedlings planted, and expressed as percentages.
2.1.2. Harvesting and establishing SRC plots
A total of 10 trees from each spacing treatment were felled at a height of 50 cm from the soil surface (Abdulah Citation2019). Harvesting was carried out three times, at 12, 18, and 24 months after the planting date. Wood yielded from these felling activities is hereinafter referred to as ‘primary wood’.
The 50-cm stools resulting from felling were left to regrow and designed as SRC plots. After 12 months, survival rates, numbers of stems/coppices, soil quality and volume of harvested wood were assessed. The wood produced from this treatment is hereinafter referred to as ‘coppiced wood’.
2.1.3. Wood productivity and quality determination
Following the felling process, all tree parts that could be classified as wood were separated from leaves and then weighed. Wood productivity was calculated in this study by multiplying the on-site wet weight of the wood together with its density and survival rate. The fresh wood was dried in oven at 105 °C for 3 days, then the wood productivity (biomass) is expressed in dried wood (kg/tree). The difference weight of fresh wood and the dried wood is noted as water content. Quality parameters for primary and coppiced wood measured in this section were calorific value and lignin content. These were determined at the Forestry Research, Development and Innovation Agency (FORDA) Forest Product Chemistry Laboratory.
2.2. Data analysis
A statistical analysis was conducted to investigate the variance and significance effects of the applied treatments. The analysis was followed by an honest significance difference test (HST). If p-value is smaller than 0.5, then the result is significant. Alleged models of competition were analyzed based on the relationship between plant performance and density (Torres and Santos Citation2015) with modified variables to find the competition index (CI) value.
Those were calculated using the equation cited in Maleki et al. (Citation2015).
Where: gi is the tree area base at the Ith observation; S is the plot size.
3. Results
3.1. Survival rates of seedlings and stools
illustrates that all density treatments in this study were able to produce favorable levels of seedling adaptation. The study showed six-month-old SRF seedlings grown at spacing treatments of 1 × 1.5 m, 1.5 × 1.5 m, 2 × 1.5 m, and 2 × 2 m having survival rates of 82.17%, 84.92%, 93.34% and 98.26%, respectively. Meanwhile, SRC stool survival rate was heavily influenced by tree age at the time of harvesting, with older trees proving more resilient after felling.
Table 1. Survival rates (%) of seedlings at six months after planting and stools after cropping.
3.2. Growth, productivity, and competition between individuals
An Analysis of Variance (Anova) test demonstrated primary wood diameter and productivity being affected significantly by tree density and harvesting age. Meanwhile, the number of stems growing from each stool was affected significantly by tree density only ().
Table 2. Summary of Anova test (p= .05) on the SRF plantation.
demonstrated that planting in a narrowest spacing (1 × 1.5 m) always have the highest CI among all ages, however at the widest spacing the CI value increases with age. Study results showed CI ranging from 0.1 to 0.3. With the narrowest spacing (1 × 1.5 m), CI value exceeded 0.2 at the age of 12 months. With the 1.5 × 1.5 m spacing, it did so at the age of 18 months. With the other two spacings (1.5 × 2 m and 2 × 2 m), CI value exceeded 0.2 at the age of 24 months. An anomali occur at the spacing 2 × 1.5 m, at six months age they have higher CI. This spacing also shows an unclear pattern dynamic of CI value.
Figure 1. (a) SRF competition indexes; (b) average number of stems/tree; (c) average diameter of the SRF.
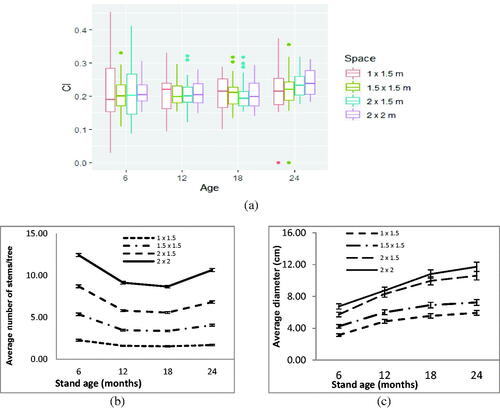
Higher CI values correlated with negative impacts on both numbers of stems per tree () and average stem diameter (). The widest spacing treatment (2 × 2 m) gave the largest stem diameters, with trees having stem diameters of 7, 8, 11, and 12 cm at the ages of 6, 12, 18, and 24 months, respectively. The wider the spacing, the larger the average diameter and number of stems per tree. Calliandra is a multi-stem plant with unclear leader shoots. Planted seeds developed naturally into 2–6 stems and built clumps (), all of which will develop into wood. The higher the plant density the smaller the average stem diameter ().
illustrates that biomas productivity is heavily influenced by growth spacing. When trees were felled at 12 months old, the 1 × 1.5 m spacing yielded 1.8 kg of biomas per tree, while the widest spacing yielded 6.6 kg/tree. A similar tendency also appeared at older ages. At 18 months old, the narrowest spacing yielded 2.1 kg of biomas per tree, while the widest spacing yielded 7.50 kg/tree. Harvesting calliandra trees at 18 months old appeared ideal, as by 24 months old productivity showed a declining trend, with some trees having died.
3.2.1. Stems, sprouts and their diameters
shows numbers of shoots regrown in a year from younger stools were not significantly different to those growing from older stools. However, harvest age of parent stands had a significant effect at the narrowest spacing, with differences evident. This particular spacing treatment caused a slightly different diameter trend when coppices were measured at one year old. Diameters of 1-year-old coppices increased with the age of parent stands at the time of harvesting and with wider spacing.
Table 3. Average numbers and diameters of coppices by spacing and cropping age.
Competition index (CI) among coppices regrown from stools, are shown in and number of coppices in . Spacing of 1 × 1.5 m with a CI value of 0.4 had the lowest number of coppices per stool, while 1.5 × 1.5 m spacing had a CI value of 0.3 and produced 10 stems; 2 × 1.5 m spacing had a CI value of 0.25 and produced 13 stems, and the widest spacing of 2 × 2 m had a CI value of 0.2 and produced 15 stems. illustrates the biomass productivity per tree did not increase significantly with harvesting age delayed from 18 months to 24 months. Since one-year-old coppices had an average yield of 8.22 kg/tree and 8.45 kg/tree, respectively. shows SRF spacing of 2 × 2 m had the highest stand survival rates at all initial harvest ages, and indicates SRF harvesting at 18 months demonstrating the highest survival rate and productivity.
3.2.2. Wood productivity and quality
Coppice wood harvested from stools at one year after cutting yielded biomass of 2–9 kg/tree which is depend on their spacing. Wider spacing has bigger biomass per tree. Similar to the primary wood biomass (), the coppice wood productivity come from stools when plants harvested at 18 and 24 months old, shows a non-significant different (). shows calliandra meeting the necessary requirements as an energy wood commodity because at 18 months old, calliandra wood produced heat energy approaching 18,841 kJ, and already contained approximately 15% lignin.
Table 4. Calorie (kJ) and lignin (%) values for primary and coppiced wood.
3.3. Soil properties
Soil analyses demonstrated the conditions of underground resources. shows soil with the lowest nutrient content coming from the plot where plants were harvested earlier and had the narrowest spacing, with nitrogen content and pH values considered poor, and organic C content categorized as low ().
Table 5. Soil chemical and biological properties at time of primary wood harvesting.
4. Discussion
The high seedling survival rates in this study () indicate the suitability of calliandra for further development as a short rotation energy wood commodity. As stools from plants felled at six months old had a high mortality rate, as indicated by the death of more than a half the population, we do not recommend SRF crops harvested at six months old being continued for SRCs. Plants felled at 12 months old had a better survival rate, but the mortality rate was still above 20%. Stands able to produce optimum coppices were those felled when they were at least 18 months old (). This study found that survival rates of stools remaining after trees had been felled were affected not only by spacing, but also by stand age at the time of harvesting (). The high mortality rates recorded in stands harvested at six and 12 months old () were likely due to strong competition between plants causing many to struggle to survive after harvesting. This finding accords with results of earlier research on the fast-growing energy plant, Miscanthus spp., where monocultures showed heavy intra-species competition with potentially negative effects. The larger miscanthus plants grew, the more they had to compete with weaker plants during the first year (Awty-Carroll et al. Citation2020).
Conversely, when plants were harvested at 18 months old, the survival rate was 95% (). Survival rates were not significantly different for plants harvested at 18 and 24 months old, with the latter having a survival rate of 97.5%. We presumed the number of plant deaths was limited when harvested at 18 months old as calliandra plants demonstrated good adaptability from that age.
The study found that plant density affected numbers of stems produced. There was an inverse relationship; the greater the plant density, the fewer the number of stems (). Plants planted at 2 × 2 m spacing produced higher numbers of stems on each tree than those planted with narrower spacing treatments. It was assumed that as the spacing facilitating plant growth became narrower, more branches would die as each plant had limited growth spacing and strong competition for water and nutrient resources in the soil. The study indicates that the denser the spacing, the greater the number of individual plants fighting for the same resource. Calculating numbers of stems rather than only diameter and basal area minimized error in predicting wood energy biomass productivity (Tahvanainen et al. Citation2007).
Plant mortality rates were 17.83% at 1 × 1.5 m spacing; 15.08% at 1.5 × 1.5 m; 6.66% at 2 × 1.5 m; and 1.76% at 2 × 2 m. As plant age increased and plants grew in size, not all the emerging stems could grow continuously. demonstrates that numbers of clumps tended to decrease as plants grew. However, after reaching 18 months old, there was a tendency to develop more new stems. As plant age increased, numbers of surviving stems decreased gradually until plants were 18 months old. Interestingly, in all plant density treatments, at 24 months old, the plants experienced increases in the numbers of stems per tree (). An earlier study found that calliandra has a unique mechanism, where trees apparently found to experience natural death at 18 months old would recover to produce new stems after 24 months old (Widyati et al. Citation2019). shows that the 24-month-old plants had the smallest average stem diameter (5 cm), in the tightest growth spacing (1 × 1.5 m), where the diameter value was the average of all growing stems. As growth spacing widened, average stem diameter increased, with the widest growth spacing (2 × 2 m) producing trees with average stem diameter of up to 12 cm. The effects of plant density on growth, numbers of stems (), and mean diameter of each stem () were inversely related to survival rate ().
The study indicates the existence of negative interaction with other plants surrounding calliandra. It can be assumed from the CI analysis in this study () that intraspecific competition strongly affected diameter sizes (), numbers of stems (), survival rate (), and primary wood biomass () for calliandra cultivated in a monoculture plantation. The CI, which was developed on the assumption that most interactions occur with nearby plants, was used to predict the size and distribution of a calliandra population that can produce optimum coppices to supply energy feedstock.
shows that a CI value of 0.2, it is assumed that the value is the tolerance level because the number of coppice and productivity is remain good. It means that an individual tree can be tolerant when 20% of either its nutrient occupation zone or its horizontal spacing overlaps with its four neighboring trees. Competition determines the energy uptake of individual plants, since there is a negative correlation between competition index and energy (Yang et al. Citation2019). The higher the plant’s CI, the lower its energy uptake, and the narrower the spacing between plants, the higher the CI (Müllerová et al. Citation2016). Plant spacing of 2 × 2 m is the most ideal for calliandra (). However, at the age of 18 months, even with this spacing the CI value exceeded 0.2. This means that stands need thinning at 18 months old, which can be a signal for initial harvesting. demonstrated that interaction become stronger when stools develop coppices. Calliandra can produce 2–15 coppices per tree after harvesting. This can be understood that they need more space for growing and nutrients foraging. Consequently, narrower space cause more mortality ().
The presence of neighboring plants triggers both aboveground and belowground competition. Neighboring competition can be defined as an interaction between individuals or populations which is negative for both, due to depletion of some limiting resources (Weiner Citation1993). In trees, neighboring competition mainly occurs between neighboring individuals and usually involves competition between crowns for light resources, or between belowground root systems for soil water and nutrients (Song et al. Citation2012). To compete with their neighbors, plants release phytochemicals into the environment, which inhibit the germination and growth of neighboring plants by altering their metabolisms or impacting their soil community mutualists (Fernandez et al. Citation2016). The plants can sense and respond to their competitors’ roots by secreting chemical compounds that may play a role in mediating plant interaction (Weidenhamer et al. Citation2019). The plants produce and release potent phytotoxins through a process called allelopathy, which can reduce the establishment, growth, or survival of susceptible neighboring plants, affecting plant physiology, growth, and survival. The presence of such substances may in turn influence plant and soil community composition and dynamics (Bais et al. Citation2006). A study of marigolds grown under high-density conditions with, both inter or intraspecific competitors, revealed that neighbor identity had an important influence on target plant growth, which may have been influenced by thiophene production (Weidenhamer et al. Citation2019). Unfortunately, our study did not aim to observe chemical substances released by calliandra, and consequently this aspect will require further study. The strength of allelopathic effects decreases as distance between plants widens (Weidenhamer et al. Citation2019), and this study shows that plant survival rates increase with wider spacing ().
The research also shows that plant density affects productivity of coppice wood biomass. At the densest plant spacing, individual trees produced the lowest volumes of biomass. This trend was consistent for plants harvested at almost all ages. At 18 months old, the spacing of 1 × 1.5 m had an average yield of 2.51 kg of biomass per tree (). Productivity was not significantly different for plots with spacings of 2 × 1.5 m and 2 × 2 m, which produced 8.45 kg and 8.92 kg of biomass per tree, respectively.
When extrapolated, at 2 × 2 m spacing, the optimum attainable dry biomass productivity would be 16.5 t/ha, while seed and other input requirements for planting with this spacing are lowest. Therefore, 2 × 2 m is the optimum spacing treatment for calliandra when planted as a short rotation energy wood crop (SRWC). This is corroborated by Erfanifard et al. (Citation2021) who found 2 × 2 m spacing reduced competition between crowns by approximately 15%. This also accords with Proe et al. (Citation1999), who found 2 × 2 m to be the ideal spacing. Narrower spacing will increase CI and investment cost (Hauk et al. Citation2014). This result contrasts with an earlier study by Tenorio et al. (Citation2019), who found the densest plant spacing for Gmelina arborea in their study producing the highest volume of biomass. This is because Gmelina arborea is a single stem species, whereas calliandra produces multiple stems that need more spacing to grow.
The lower biomass productivity of individual plants in narrower growth spacings in this study was presumably due to greater competition between plants. Using a monoculture plantation, competition in this study was intra-species with all plants having the same niche (Yang et al. Citation2019). A further effect of intra-species competition on plant growth and biomass, particularly aboveground biomass (Cavalieri et al. Citation2020), is a decrease in individual plant productivity with increased population density. Earlier studies have shown intra-species competition (in monocultures) being four to five times stronger than interspecies competition due to niche overlapping (Adler et al. Citation2018), and competition inhibiting growth rates and increasing mortality rates of plant parts (leaves, branches) (Weiner Citation1993). Competition decreases growth rates and/or increases the probability of individual mortality due to the presence of neighbors. Several studies have suggested that roots can detect and avoid neighboring roots, and thus segregate spatially in territories. This ability to discriminate the roots of other plants can reduce wasteful allocation to competition and allow greater resource availability for other functions, including greater reproductive output (Wu et al. Citation2016). In this study, however, the calliandra plants grown in a monoculture with narrow spacing were unable to develop such an avoidance mechanism. This confirms that competition with neighboring plants affects tree growth, stem shape, crown shape, and biomass productivity (Cao et al. Citation2014).
This study confirms that plant density seems to generate underground competition. Soil conditions strongly determine the growth of aboveground biomass. There were differences in total N level in soil, which was influenced by spacing, where wider spacing had higher soil N content than narrower spacing. demonstrates that the wider the growth spacing, the better the soil conditions. Almost all soil properties had the lowest values in the plot with the greatest plant density, thus indicating the presence of competition for resources in the soil. In line with earlier research, belowground competition can be stronger than aboveground competition, which primarily involves light as a single resource. This is because plants compete for a broad range of soil resources, including water and at least 20 essential mineral nutrients that differ in molecular size, valence, oxidation state, and mobility within the soil (Casper and Castelli Citation2007). demonstrates that available N for calliandra plants was at its lowest value when they were planted in the smallest growth spacing. Other crucial elements, P and K also showed the same trend. Soil resources reach the root surface through three general processes: root interception, mass flow of water and nutrients, and diffusion (Casper and Castelli Citation2007). Diffusion is especially important for nutrients with large fractions bound to the solid soil matrix, such as potassium and phosphates, whereas mass flow is often more important for nitrogen, particularly nitrates. Supply of the three major nutrients (N, P and K) almost always depends on the collaboration between diffusion and mass flow. Among mechanisms of root interactions, competition via diffusion has received the most attention. Neighboring roots reduce nutrient uptake when nutrient depletion zones overlap. CI also determine soil nutrient content, especially N, P, C, and K (Zhou et al. Citation2018).
also shows the densest plots had the lowest microbial populations. Total microbes increased as growth spacing became larger. One of the plant activities is releasing root exudates to attract soil microbes, thus optimizing nutrient uptake. Root exudates, nutrient availability and soil microbial community, are a closed triangle, simultaneously affecting plant growth and consequently competition (Pierik et al. Citation2012). In this case, the relationship between growth spacing and soil microbial population was that plants growing under tight competition with the greatest plant density per unit area were less able to attract microbial populations than those with more growth spacing (). Plants respond to the presence of neighboring plants through their growth rate and growth form. They also respond physiologically and biochemically, e.g., through changes in root exudate composition (Pierik et al. Citation2012). Alterations in these responses may determine the richness of microbial communities in soil or associated with roots (Huang et al. Citation2014). In intra-species competition, changes in the microbial community in the rhizosphere are more significant (Cavalieri et al. Citation2020).
Soil nutrition is poorer at the narrower spacing and earlier harvest age (). It can be understood that the denser plants need more nutrients from soil. In addition, earlier harvest remove nutrients accumulated in the wood biomass from the site faster. These situations lead to nutrients deficiency. In monoculture plantation, there are the same species with the same requirements of nutrients. It causes deficiency of elements demanded but accumulation of it unneeded. Furthermore, monocultures may prompt shifts in the surrounding environment (soil conditions) and the composition of belowground microbes, potentially inducing plant–soil feedback responses (Pérez-Izquierdo et al. Citation2019). Shifts in the structure of microbial communities have further consequences on ecosystem performance in terms of potential enzyme activities associated with nutrient cycling (Pérez-Izquierdo et al. Citation2019). shows that N, P, and K availability also poor. This study support the evidence that nitrogen availability significantly impacts bacterial and fungal communities (Guo et al. Citation2019).
The lignin content of primary wood was slightly higher when plants were harvested at 24 months old. Coppiced wood produced from stools harvested in the next rotation appeared to have higher calorific values than primary wood. The calorific values of 12-month-old coppiced wood were not significantly different from those of primary wood harvested at 12, 18, and 24 months old. As long as parent stands were at least 12 months old, the calorific value of calliandra wood produced during the following year’s rotation met the criteria necessary for energy wood at harvesting time. However, plant survival rate is an important consideration. When plants were harvested at 12 months old, 77.5% survived and were able to regenerate to produce a further yield. As stand age increased, the survival rate became higher ().
This study shows one-year-old coppices grown from stools harvested at a minimum age of 18 months old could yield up to 30% more biomass compared to the first rotation (). The ideal age for harvesting coppiced wood was deemed to be 12 months old due to its calorific value and lignin content (). This, accords with a previous study showing the most effective pruning interval to be one year (Abdulah Citation2019). The same SRF plantation study also showed plant spacing and age of parent stands at time of harvesting could determine the growth and productivity of calliandra grown as short rotation coppices.
When parent stands were harvested at 12 months old, the mortality rate of plants was high (). However, how defense mechanisms work still requires further study. When parent stands were felled at an age of 18 months old, the average number of resulting coppices ranged from 7%–25% higher than from parent stands felled at 12 months old. At the age of 24 months, the average number only increased by a further 5% (). For stands harvested at 18 months, a growth spacing of 2 × 2 m was the best treatment, providing the highest numbers of coppices and volumes of biomass. This increase was because plants could grow back after harvesting, and with sufficient spacing for growth, the optimum number of coppices would grow. These results accord with a previous study on poplar managed in a similar way (according to planting SOPs), where after coppicing, the single-stem poplar trees re-sprouted in multiple, different numbers of shoots. However, no specific trend in changes in shoot number (in terms of self-thinning) was observed toward the second growth year of the poplar (Verlinden et al. Citation2015).
5. Conclusions
In cultivating Calliandra calothyrsus (Meissn.) in a short rotation forestry (SRF) and short rotation coppice (SRC) system in West Java Indonesia, competition with neighboring plants impacted strongly on numbers of stems and stem diameter sizes. The strongest competition resulting from the densest plant spacing showed the poorest soil nutrient availability for plants, which further limited biomass productivity. To minimize competition and produce optimum volumes of coppiced wood, calliandra should be planted with a spacing of 2 × 2 m. Competition index values increase with plant age.
To ensure sustainable productivity, the initial harvest should take place when parent stands are 18 months old. Harvesting at 12 months led to higher mortality, while productivity, lignin content, and calorific value did not improve when harvesting took place at 24 months. With SRF harvesting at 18 months, the average number of coppices was up to 25% higher than for trees harvested at 12 months. Delaying SRF harvesting to 24 months only increased the average number of coppices by a further 5%, which is not significant enough to preclude harvesting six months earlier.
Recommendations for future calliandra short rotation coppice implementation in sites with characteristics similar to those in West Java:
Calliandra SRC plantations should be arranged with a stand density of 2,500 trees per ha with initial harvesting conducted at 18 months after planting. Subsequent harvesting of coppices should be applied every 12 months when wood quality is most similar to that of primary wood.
Waste (leaves, trunks, etc.) should be left on site as a soil organic matter source for soil amendment.
Acknowledgements
This project is part of the Integrative Research and Development Plan (RPPI) Program 5 entitled “Forest as a Renewable Energy Source” funded by the Indonesian Ministry of Environment and Forestry’s Research and Development Agency (FOERDIA). The authors express our appreciation to the DG of FOERDIA and the Director of Forest Research and Development. The authors also thank the CGIAR research program on Forests, Trees and Agroforestry (FTA) for its support in publishing this paper. The authors also thank Waryono for his assistance in developing the plantation trials, Rukman for data collection, and laboratory staff members for analyzing samples.
Disclosure statement
There are no competing interests that need to be disclosed.
Additional information
Funding
References
- Abdulah L. 2019. The growth and yield of Calliandra callothyrsus trees as biomass-based energy feedstock. IOP Conf Ser: Earth Environ Sci. 308(1):012078.
- Adler PB, Smull D, Beard KH, Choi RT, Furniss T, Kulmatiski A, Meiners JM, Tredennick AT, Veblen KE. 2018. Competition and coexistence in plant communities: intraspecific competition is stronger than interspecific competition. Ecol Lett. 21(9):1319–1329.
- Awty-Carroll D, Hauck B, Clifton-Brown J, Robson P. 2020. Allelopathic and intraspecific growth competition effects establishment of direct sown Miscanthus. Glob Change Biol Bioenergy. 12(6):396–409.
- Bacenetti J, Bergante S, Facciotto G, Fiala M. 2016. Woody biofuel production from short rotation coppice in Italy: environmental-impact assessment of different species and crop management. Biomass Bioenergy. 94:209–219.
- Bais HP, Weir TL, Perry LG, Gilroy S, Vivanco JM. 2006. The role of root exudates in rhizosphere interactions with plants and other organisms. Annu Rev Plant Biol. 57:233–266.
- Cao Y, Wang T, Xiao Y, Zhou B. 2014. The interspecific competition between Humulus scandens and Alternanthera philoxeroides. J Plant Interact. 9(1):194–199.
- Casper BB, Castelli JP. 2007. Evaluating plant-soil feedback together with competition in a serpentine grassland. Ecol Lett. 10(5):394–400.
- Cavalieri A, Bak F, Garcia-Lemos AM, Weiner J, Nicolaisen MH, Nybroe O. 2020. Effects of intra- and interspecific plant density on rhizosphere bacterial communities. Front Microbiol. 11:1045–1014.
- Craine JM, Dybzinski R. 2013. Mechanisms of plant competition for nutrients, water and light. Funct Ecol. 27(4):833–840.
- Dimitriou I, Rutz D. 2015. Sustainable Short Rotation Coppice. A Handbook. D. Rutz (ed.); (Issue November). Munich, Germany:WIP Renewable Energies.
- Erfanifard Y, Pourhashemi M, Alimahmoodi Sarab S. 2021. The impact of coppice management on spatial structure and intraspecific interactions of Brant’s oak (Quercus brantii Lindl.) semi-arid woodlands. Acta Oecologica. 113:103787.
- Fernandez C, Monnier Y, Santonja M, Gallet C, Weston LA, Prévosto B, Saunier A, Baldy V, Bousquet-Mélou A. 2016. The impact of competition and allelopathy on the trade-off between plant defense and growth in two contrasting tree species. Front Plant Sci. 7:1–14.
- Foli EG, Alder D, Miller HG, Swaine MD. 2003. Modelling growth spacing requirements for some tropical forest tree species. For Ecol Manage. 173(1-3):79–88.
- Fuertes A, Oliveira N, Cañellas I, Sixto H, Rodríguez-Soalleiro R. 2021. An economic overview of Populus spp. in short rotation coppice systems under Mediterranean conditions: an assessment tool for decision-making. Renew Sustain Energy Rev. 151:111577.
- Guo Q, Yan L, Korpelainen H, Niinemets Ü, Li C. 2019. Plant-plant interactions and N fertilization shape soil bacterial and fungal communities. Soil Biol Biochem. 128:127–138.
- Hauk S, Knoke T, Wittkopf S. 2014. Economic evaluation of short rotation coppice systems for energy from biomass - a review. Renew Sustain Energy Rev. 29:435–448.
- Hendrati RL, Nurrohmah SH. 2019. Breeding for better growth of Calliandra calothyrsus Meissn., a fast growing legume for wood-energy. JPerbenihanTanHutan. 7(1):13–20.
- Hortal S, Lozano YM, Bastida F, Armas C, Moreno JL, Garcia C, Pugnaire FI. 2017. Plant-plant competition outcomes are modulated by plant effects on the soil bacterial community. Sci Rep. 7(1):1–9.
- Huang X, Chaparro JM, Reardon KF, Zhang R, Shen Q, Vivanco JM. 2014. Rhizosphere interactions: root exudates, microbes, and microbial communities 1. Botany. 92(4):267–275.
- Maleki K, Kiviste A, Korjus H. 2015. Analysis of individual tree competition effect on diameter growth of silver birch in Estonia. Forest Syst. 24(2):e023.
- McKay, H, (ed.), 2011. Short Rotation Forestry: review of growth and environmental impacts. Forest Research Monograph, 2. Forest Research Surrey, 212 pp National Development Plan Council of Indoneisa (Bappenas). Decree Number 11/2020. Strategic plan of Bappenas. year 2020–2024
- Moya R, Tenorio C, Oporto G. 2019. Short rotation wood crops in Latin American: a review on status and potential uses as biofuel. Energies. 12(4):705.
- Müllerová J, Pejcha V, Altman J, Plener T, Dörner P, Doležal J. 2016. Detecting coppice legacies from tree growth. PLoS One. 11(1):e0147205–14.
- National Development Plan Council of Indoneisa (Bappenas). Decree Number 11/2020. Strategic plan of Bappenas. year 2020–2024.
- Peraturan Pemerintah Republik Indonesia Nomor 79 Tahun 2014 tentang Kebijakan Energi Nasional (Government Regulations of the Republic of Indonesia Number 79 Year 2014 on National Energy Policy), Pub. L. No. 79, 1 2014.
- Pérez-Izquierdo L, Zabal-Aguirre M, González-Martínez SC, Buée M, Verdú M, Rincón A, Goberna M. 2019. Plant intraspecific variation modulates nutrient cycling through its below ground rhizospheric microbiome. J Ecol. 107(4):1594–1605.
- Pierik R, Mommer L, Voesenek LACJ. 2012. Molecular mechanisms of plant competition: neighbour detection and response strategies. Funct Ecol. 27(4):841–853.
- Proe MF, Craig J, Gri J, Wilson A, Reid E. 1999. Comparison of biomass production in coppice and single stem woodland management systems on an imperfectly drained gley soil in central Scotland. Biomass Bioenergy. 17(2):141–151.
- Rencana Pembangunan Jangka Menengah Nasional 2020., Pub. L. No. 18, Peraturan Presiden Republik Indonesia Nomor 18 Tahun 2020.
- Simon J, Schmidt S. 2017. Editorial: plant competition in a changing world. Front Plant Sci. 8(April):651–610.
- Song M, Hu Q, Tian Y, Ouyang H. 2012. Seasonal patterns of root and shoot interactions in an alpine meadow on the Tibetan Plateau. J Plant Ecol. 5(2):182–190.
- Spinelli R, Cacot E, Mihelic M, Nestorovski L, Mederski P, Tolosana E. 2016. Techniques and productivity of coppice harvesting operations in Europe: a meta-analysis of available data. Ann for Sci. 73(4):1125–1139.
- Tahvanainen T, Kaartinen K, Pukkala T, Maltamo M. 2007. Comparison of approaches to integrate energy wood estimation into the Finnish compartment inventory system. Silva Fenn. 41(1):123–135.
- Tenorio C, Moya R, Ortiz-Malavassi E, Arias D. 2019. Production and regression models for biomass and carbon captured in Gmelina arborea Roxb. Trees in short rotation coppice plantations in Costa Rica. Forests. 10(7):593.
- Torres NV, Santos G. 2015. The (mathematical) modeling process in biosciences. Front Genet. 6(DEC):354–359.
- Verlinden MS, Broeckx LS, Ceulemans R. 2015. First vs. second rotation of a poplar short rotation coppice: above-ground biomass productivity and shoot dynamics. Biomass Bioenergy. 73:174–185.
- Weidenhamer JD, Montgomery TM, Cipollini DF, Weston PA, Mohney BK. 2019. Plant density and rhizosphere chemistry: does marigold root exudate composition respond to intra- and interspecific competition? J Chem Ecol. 45(5-6):525–533.
- Weiner J. 1993. Competition among plants. Treballs de La SCB. 44:99–109.
- Widyati E, Darwiati W, Yulianti M. 2019. Land management in optimizing productivity of limited land to deal with rural food, feed and fuel (3F) security. IOP Conf Ser: Earth Environ Sci. 394(1):012068–012066.
- Wu J, Liu W, Chen C. 2016. Below-ground interspecific competition for water in a rubber agroforestry system may enhance water utilization in plants. Sci Rep. 6:19502–19513.
- Xu CY, Turnbull MH, Tissue DT, Lewis JD, Carson R, Schuster WSF, Whitehead D, Walcroft AS, Li J, Griffin KL. 2012. Age-related decline of stand biomass accumulation is primarily due to mortality and not to reduction in NPP associated with individual tree physiology, tree growth or stand structure in a Quercus-dominated forest. J Ecol. 100(2):428–440.
- Yang X-z, Zhang W, He Q. 2019. Effects of intraspesific competition on growth, architecture and biomass allocation of Quercus liaotungensis. J Plant Interact. 14(1):284–294.
- Zhou W, Cheng X, Wu R, Han H, Kang F, Zhu J, Tian P. 2018. Effect of intraspecific competition on biomass partitioning of Larix principis-rupprechtii. J Plant Interact. 13(1):1–8.